Introduction
Tapeworms (Cestoda) are parasitic flatworms (Platyhelminthes: Neodermata) that occur as adults almost exclusively in the intestinal tract of all major vertebrate groups, including elasmobranchs (Chondrichthyes: Holocephali and Elasmobranchii; here called elasmobranchs for simplicity) and ray-finned fishes (Actinopterygii; here called teleosts for simplicity). They are characterized by (1) the absence of a digestive tract (intestine), (2) the presence of hair-like structures called microtriches on their surface, (3) the anterior end of the body called the scolex and (4) a usually long, dorsoventrally flattened body (strobila) that contains multiple sets of genital organs (proglottids) in most groups (except for the earliest diverged orders called monozoic, i.e. with a single set of genital organs) (Khalil et al., Reference Khalil, Bray and Jones1994; Caira and Jensen, Reference Caira and Jensen2017). Adult fish tapeworms vary in overall length from less than a millimetre up to 2 m (Eubothrium crassum), but their larvae (metacestodes) are usually much smaller (Williams and Jones, Reference Williams and Jones1994; Chervy, Reference Chervy2002). ‘Fish tapeworms’ here refer to all tapeworms that mature in elasmobranchs and teleosts.
Caira and Jensen (Reference Caira and Jensen2014) and Scholz and Kuchta (Reference Scholz and Kuchta2017a) provided an overview of the current knowledge on elasmobranch and teleost tapeworms, respectively. Caira et al. (Reference Caira, Jensen, Waeschenbach, Olson and Littlewood2014) provided evidence for the unexpected radiation of elasmobranch tapeworms and its importance in deciphering the evolutionary history of all tapeworms, and finally Caira and Jensen (Reference Caira and Jensen2017) summarized updated information on all tapeworms in a comprehensive monograph. Data on nominal species of all tapeworms, including hosts, localities and authorities not listed herein, are available (and continually updated) in the freely available on-line Global Cestode Database (Caira et al., Reference Caira, Jensen and Barbeau2022).
Here we briefly review the history of fish tapeworm research, focusing on the recent application of molecular tools, diversity of fish tapeworms, their systematics, host–parasite associations and distribution.
Pre-molecular era
Research on tapeworms has a long tradition, mainly because some species infecting humans (such as the pork tapeworm Taenia solium and beef tapeworm Taenia saginata or the broad fish tapeworm Dibothriocephalus latus) were known since the Middle Ages and were already known to Linnaeus (Reference Linnaeus1758). However, intensive research on tapeworms began much later, especially in the 20th century, with relatively few fish tapeworms described in the 18th and 19th centuries (Fig. 1).

Fig. 1. Species diversity of fish tapeworms (elasmobranch tapeworms in blue, teleost tapeworms in green): (A) cumulative curve of species of fish tapeworms described since 1776; (B) numbers of species of fish tapeworms described every year since 1970; note steady or even slowly lowering rate of description of new species of teleost tapeworms within the last 50 years.
The first fish tapeworm – larvae of trypanorhynchs (now Tetrarhynchus argentinae; see Southwell, Reference Southwell1929) – was recorded as early as in the 17th century by Redi (Reference Redi1684), who isolated its larvae from various organs of the herring smelt Argentina sphyraena and was probably the first helminthologist who studied fish tapeworms. The first adult fish tapeworm described was Bothriocephalus scorpii by Müller (Reference Müller1776) from the sculpin Myoxocephalus scorpius off Denmark, followed by the description of the largest fish tapeworm E. crassum by Bloch (Reference Bloch1779) from the Atlantic salmon Salmo salar in Germany. The first adult elasmobranch tapeworm was Gilquinia squali, described by Fabricius (Reference Fabricius1794) from the dogfish Squalus acanthias off Denmark (see Caira et al., Reference Caira, Jensen and Barbeau2022).
Fish tapeworms have been most intensively studied in temperate regions, including Europe, the former USSR, Japan and North America (Dubinina, Reference Dubinina and Bauer1987; Williams and Jones, Reference Williams and Jones1994; Hoffman, Reference Hoffman1999). The life cycles of most pathogenic fish tapeworms were elucidated in the 20th century, but this almost exclusively involves freshwater taxa (mainly those of bothriocephalideans, caryophyllideans and proteocephalids [= Onchoproteocephalidea I; for simplicity and to avoid confusion, called thereafter as proteocephalideans]; see below). In contrast, little attention has been paid to the life cycles and ecology of marine fish tapeworms, mainly because of obstacles of laboratory experiments with marine animals (Jensen and Bullard, Reference Jensen and Bullard2010). The first elasmobranch tapeworm life cycle elucidated was that of the trypanorhynch Grillotia erinaceus, which involves 2 intermediate hosts, copepods and fish (Ruszkowski, Reference Ruszkowski1934).
The original concept of higher-level classification of tapeworms dates back to Van Beneden (Reference Van Beneden1850) and van Beneden in Carus (Reference Carus and Carus1863), who recognized several groups that are now considered orders, such as Diphyllidea, Caryophyllidea or Tetraphyllidea, followed by the monographs of Wardle and McLeod (Reference Wardle and McLeod1952) and Yamaguti (Reference Yamaguti1959) which set important milestones in terms of tapeworm systematics. Classification of cestodes at a higher taxonomic level was based mainly on the scolex morphology (Fig. 2) and the characteristics of the genital system (see Schmidt, Reference Schmidt1986; Khalil et al., Reference Khalil, Bray and Jones1994). The most important taxonomic categories are the orders and their delimitation was relatively uncontroversial (Wardle and McLeod, Reference Wardle and McLeod1952; Yamaguti, Reference Yamaguti1959; Schmidt, Reference Schmidt1986), with 14 orders recognized in the most comprehensive monograph published in the pre-molecular era (Khalil et al., Reference Khalil, Bray and Jones1994).

Fig. 2. Scanning electron micrographs of scoleces of representatives of orders of fish tapeworms: (A) Archigetes vadosus (Caryophyllidea) from Ictiobus bubalus, USA; (B) Diplocotyle olrikii (Spathebothriidea) from Myoxocephalus scorpius, off Svalbard Archipelago, Norway; (C) Haplobothrium globuliforme (Haplobothriidea) from Amia calva, USA; (D) plerocercoid of Dibothriocephalus latus (Diphyllobothriidea) from Perca fluviatilis, Italy; (E) Halysioncum reginae (Diphyllidea) from Pastinachus ater, off Madagascar; (F) Pterobothrium sp. (Trypanorhyncha) from Styracura schmardae, off Belize; (G) Kirstenella gordoni (Bothriocephalidea) from Heterobranchus bidorsalis, Kenya; (H) plerocercoid of Clistobothrium sp. 3 of Caira et al. (Reference Caira, Jensen, Hayes and Ruhnke2020a, Reference Caira, Jensen, Pickering, Ruhnke and Gallagher2020b) (Phyllobothriidea) from Oncorhynchus gorbuscha, off Alaska; (I) Gangesia bengalensis (Onchoproteocephalidea I – Proteocephalidae) from Wallago attu, India; (J) Nippotaenia perccotti (Nippotaeniidea) from Perccottus glenii, Slovakia; (K) Ichthyolepis africana (Cyclophyllidea: Dilepididae) from Marcusenius macrolepidotus, South Africa; (L) merocercoid of Neogryporhynchus lasiopeius (Cyclophyllidea: Gryporhynchidae) from Tilapia sparrmanii, Zimbabwe.
Onset of the molecular era
Molecular phylogenetic studies
Molecular tools, particularly sequences of 18S rDNA, were used in tapeworms beginning in the late 1990s (Králová et al., Reference Králová, Van de Peer, Jirků, van Ranst, Scholz and Lukeš1997; Liu et al., Reference Liu, Kato and Sugane1997), but the study of Mariaux (Reference Mariaux1998) should be considered the starting point of the molecular phylogeny era in tapeworms, followed by studies of Olson and Caira (Reference Olson and Caira1999) and Kodedová et al. (Reference Kodedová, Doležel, Broučková, Jirků, Hypša, Lukeš and Scholz2000), which included 16 and 33 species of fish tapeworms, respectively.
At the beginning of the 21st century, intensive efforts were made to verify the reliability of the existing classification of tapeworms. The initial studies, based primarily on partial 18S rDNA sequences, surprisingly identified the internally proglottized Spathebothriidea as the most basal ‘true’ tapeworms (Eucestoda) (Olson and Caira, Reference Olson and Caira1999; Kodedová et al., Reference Kodedová, Doležel, Broučková, Jirků, Hypša, Lukeš and Scholz2000). However, more comprehensive studies later confirmed the transition of cestode body plan from monozoy (Caryophyllidea) to polyzoy, i.e. the consequent evolution of strobilization and the formation of attachment organs on the scolex from simple bothria (bothriate groups) to sucker-like attachment organs (acetabulate groups) (Olson et al., Reference Olson, Littlewood, Bray and Mariaux2001).
Waeschenbach et al. (Reference Waeschenbach, Webster, Bray and Littlewood2007, Reference Waeschenbach, Webster and Littlewood2012) and Caira et al. (Reference Caira, Jensen, Waeschenbach, Olson and Littlewood2014) have provided the most robust phylogenetic dataset for all tapeworm orders to date. These studies confirmed unexpectedly high radiation of elasmobranch tapeworms (Caira et al., Reference Caira, Jensen, Waeschenbach, Olson and Littlewood2014; Fig. 3; see section ‘Remarkable achievements’). They also provided strong support for the previous ordinal classification of tapeworms, with only a few exceptions, such as the non-monophyly of the Pseudophyllidea (divided now into 2 unrelated orders, Bothriocephalidea and Diphyllobothriidea – Kuchta et al., Reference Kuchta, Scholz, Brabec and Bray2008a) and the existence of several independent lineages of elasmobranch tapeworms that merit elevation to the ordinal level (see below and Waeschenbach and Littlewood, Reference Waeschenbach, Littlewood, Caira and Jensen2017).
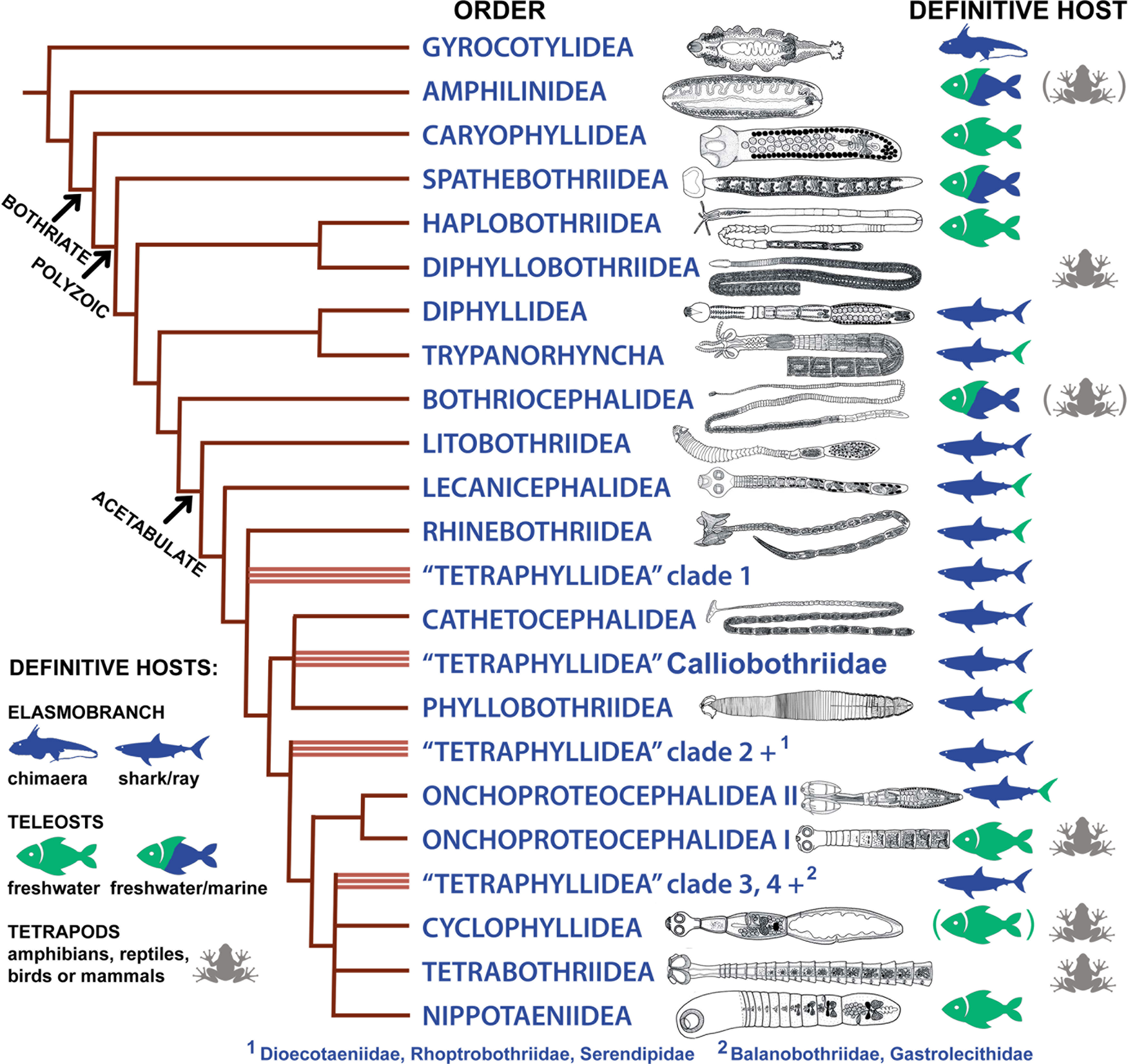
Fig. 3. Phylogenetic relationships of tapeworms (Cestoda); modified from Caira et al. (Reference Caira, Jensen, Waeschenbach, Olson and Littlewood2014) and updated from Caira and Jensen (Reference Caira and Jensen2017). A single species of adult cyclophyllideans was found in teleosts (host pictogram in parentheses). Freshwater hosts in green, marine hosts in blue (combined if tapeworms occur in both environments considering proportion of their species).
Molecular markers used
The molecular markers used in studies of tapeworms depend on the taxonomic level studied. For higher level classification (ordinal and familiar), only partial 18S rDNA sequences were originally used, with the addition of partial 28S rDNA sequences as proposed by Olson et al. (Reference Olson, Littlewood, Bray and Mariaux2001). Domains D1–D3 of 28S rDNA have become the gold standard in most taxonomic and phylogenetic studies of fish tapeworms and a high number of their sequences are available, usually supplemented with other markers suitable for lower-level classification (genus and species levels), such as internal transcribed spacers (ITS-1, 5S rDNA, ITS-2) of the nuclear ribosomal DNA region. This region became popular some 15–20 years ago for taxonomic studies at the species level to discriminate closely related taxa. However, these markers can cause artefacts in some groups, such as Bothriocephalidea, Caryophyllidea and Diphyllobothriidea (Bouzid et al., Reference Bouzid, Štefka, Hypša, Lek, Scholz, Legal, Hassine and Loot2008; Brabec et al., Reference Brabec, Scholz, Králová-Hromadová, Baszalovicsová and Olson2012, Reference Brabec, Kuchta, Scholz and Littlewood2016), which partly concerns also some mitochondrial genes, especially the complete cytochrome c oxidase subunit I (cox1). The last marker is most commonly used in recent lower-level phylogenetic and population genetical studies of tapeworms. The most sequenced fish tapeworm to date is the invasive Asian fish tapeworm Schyzocotyle acheilognathi (syn. Bothriocephalus acheilognathi), with more than 100 sequences each of 18S, 28S and ITS. Details of currently available molecular markers of fish tapeworms can be found in the GenBank database and Table 1.
Table 1. Number of DNA sequences of tapeworms, including species parasitizing fish, i.e. teleosts and elasmobranchs (data as of 31 May 2022)

a Elasmobranch tapeworms.
Mitogenomics (mtDNA) and next-generation sequencing (NGS)
In contrast to the Cyclophyllidea and Diphyllobothriidea, which may parasitize humans, very few data are available on mitochondrial genomes of fish tapeworms. To date, very few mitochondrial genomes of fish tapeworms are available. Waeschenbach et al. (Reference Waeschenbach, Webster and Littlewood2012) used large fragments of mtDNA (more than 4000 bp) together with 18S and 28S rDNA to reconstruct the relationships of 23 tapeworm species from all 18 orders. Brabec et al. (Reference Brabec, Kuchta, Scholz and Littlewood2016) characterized complete mitochondrial genomes and nuclear rRNA operons of 8 geographically distinct isolates of the bothriocephalidean S. acheilognathi, representing the parasite's global diversity spanning 4 continents. These authors also demonstrated the limited utility of nuclear rRNA sequences, including ITS, which likely misled previous phylogenetic and population genetic studies of S. acheilognathi because of the presence of considerable intragenomic sequence variation (i.e. the presence of multiple paralogous sequences of these genomic loci within an individual) within the rDNA and ITS, as well as the other rRNA operon loci of S. acheilognathi (Brabec et al., Reference Brabec, Kuchta, Scholz and Littlewood2016). In addition, Brabec et al. (Reference Brabec, Scholz and Štefka2018) developed a set of 15 polymorphic microsatellite markers for future population genetic and phylogeographic studies of S. acheilognathi. Moreover, Li et al. (Reference Li, Zhang, Boyce, Xi, Zou, Wu, Li and Wang2017) sequenced mitochondrial genomes of 3 caryophyllidean tapeworms and S. acheilognathi. A comparative analysis of 54 cestode mitogenomes of 52 species, which included 40 cyclophyllideans (16 species of Taenia and 9 species of Echinococcus), revealed that Atractolytocestus huronensis had the longest mitogenome of all tapeworms (15 130 bp long) and that mitogenomes of all 3 caryophyllideans sequenced (A. huronensis, Breviscolex orientalis and Khawia sinensis) had the lowest A–T content (58.6–65.6%) of all cestodes (Li et al., Reference Li, Zhang, Boyce, Xi, Zou, Wu, Li and Wang2017). An unpublished sequence of the mitogenome of another caryophyllidean, Caryophyllaeus brachycollis from China, is deposited in the GenBase database (KT028770).
In the case of elasmobranch tapeworms, Trevisan et al. (Reference Trevisan, Alcantara, Machado, Marques and Lahr2019) sequenced the mitogenome of Anindobothrium anacolum and Rhinebothrium reydai. Trevisan et al. (Reference Trevisan, Jacob Machado, Lahr and Marques2021) obtained complete mtDNA sequences of 86 specimens from 5 orders. At that time, these data nearly doubled the mtDNA dataset available for cestodes and an expansion of the representation of the cestode orders by about 1/3. The complete mtDNA of fish tapeworms is around 13 500 bp long and contains 12 protein-coding genes, 22 tRNA (trn) genes and 2 rRNA genes, following the pattern of flatworms (Brabec et al., Reference Brabec, Kuchta, Scholz and Littlewood2016; Trevisan et al., Reference Trevisan, Jacob Machado, Lahr and Marques2021).
NGS data are scarce for fish tapeworms (e.g. Brabec et al., Reference Brabec, Kuchta, Scholz and Littlewood2016, Reference Brabec, Scholz and Štefka2018; Trevisan et al., Reference Trevisan, Alcantara, Machado, Marques and Lahr2019, Reference Trevisan, Jacob Machado, Lahr and Marques2021). To date, there are only 6 transcriptomes, all diphyllobothriideans that use fish as intermediate hosts (Dibothriocephalus dendriticus, D. latus, D. nihonkaiensis, Ligula intestinalis, Schistocephalus solidus, and Spirometra mansoni), and 3 published genomes for D. latus, S. solidus and S. mansoni compared with at least 13 available genomes for cyclophyllideans (International Helminth Genomes Consortium, 2019). However, new high-throughput data are currently being generated for several fish tapeworms (J. N. Caira, University of Connecticut, Storrs, USA; J. Brabec, Biology Centre CAS, České Budějovice, Czech Republic, personal communication, 2022).
Remarkable achievements
Species diversity
The last 2 decades have seen unprecedented progress in the study of the diversity of fish tapeworms (Caira et al., Reference Caira, Jensen, Georgiev, Kuchta, Littlewood, Mariaux, Scholz, Tkach, Waeschenbach, Caira and Jensen2017a). Several monographs have been published on individual elasmobranch tapeworm orders, namely Trypanorhyncha – Palm (Reference Palm2004), Lecanicephalidea – Jensen (Reference Jensen2005), Diphyllidea – Tyler (Reference Tyler2006) and Phyllobothriidea – Ruhnke (Reference Ruhnke2011). Caira and Jensen (Reference Caira and Jensen2014) and Scholz and Kuchta (Reference Scholz and Kuchta2017a) presented concise reviews (‘digest’) of elasmobranch and teleost tapeworms, respectively, but the major achievements were summarized in a monograph by Caira and Jensen (Reference Caira and Jensen2017) that provides detailed information on all cestode orders.
Another milestone is the establishment of the Global Cestode Database (www.tapewormdb.uconn.edu; Caira et al., Reference Caira, Jensen and Barbeau2022), which contains taxonomic and nomenclatural data on most of the described cestode species with their original hosts and localities, availability of type material and in most cases also with their original descriptions and images. To date (as of 1 June 2022), there are 12 790 records, including 1334 nominal generic names (804 valid) and 11 456 nominal species names (3400 valid species have been included to date, representing 68% of ~5000 known valid tapeworm species, with the fish tapeworm orders almost fully represented – Caira and Jensen, Reference Caira and Jensen2017; Caira et al., Reference Caira, Jensen and Barbeau2022). Moreover, the database also contains extensive literature, particularly original descriptions of new species that are available electronically (a total of 4779 papers and monographs is available online in the Global Cestode Database).
Since 2000, the pace of descriptions of new cestode taxa from fish has accelerated, with an increase of 527 new species that represent almost 1/2 of known species of elasmobranch tapeworms and 75 new species of teleost tapeworms, as shown by comparing the data of Caira and Jensen (Reference Caira and Jensen2017) with the current status (Fig. 1, Table 2). The highest increase in species richness since 2017 is seen in the Rhinebothriidea (38 new species, including 15 spp. of Rhinebothrium), Phyllobothriidea (37 new species, including 7 spp. of Scyphophyllidium), Onchoproteocephalidea II (22 new species, including 15 spp. of Acanthobothrium), Trypanorhyncha (20 new spp.), ‘Tetraphyllidea’ (19 new spp.), Caryophyllidea (13 new spp.) and Onchoproteocephalidea I (12 new spp.) (Caira et al., Reference Caira, Jensen and Barbeau2022).
Table 2. Number of valid species of tapeworms in 2022

Numbers from Caira and Jensen (Reference Caira and Jensen2017) in parentheses. Total numbers in bold.
a Including orders using fish as final (definitive) host, with the exception of Diphyllobothriidea (71 spp.) and Tetrabothriidea (70 spp.).
Currently, nearly 1200 species of elasmobranch tapeworms and nearly 500 species of teleost tapeworms are recognized as valid (Fig. 4, Table 2; Caira et al., Reference Caira, Jensen and Barbeau2022). Elasmobranch diversity hot spots are indeed restricted to regions where recent sampling has occurred, with tropical marine regions predominating (Caira and Jensen, Reference Caira and Jensen2017). For teleost tapeworms, the main hot spot of diversity is South American fresh water, i.e. the Amazon and Paraná river basins (de Chambrier et al., Reference de Chambrier, Scholz, Mariaux, Kuchta, Caira and Jensen2017), where 7 new species have been described since 2017 (Alves et al., Reference Alves, de Chambrier, Luque and Scholz2020, Reference Alves, de Chambrier, Luque, Takemoto, Tavares and Scholz2021), but there are still many undescribed taxa (unpublished data). Another hot spot is North America, especially the southern USA, where 10 new caryophyllidean species have been described since 2017 (Scholz and Oros, Reference Scholz, Oros, Caira and Jensen2017). In contrast, very few new species have been described from other regions, including the Afrotropical region (3 new spp.), and only 2 valid species from the Oriental region (Scholz and de Chambrier, Reference Scholz and de Chambrier2012; Scholz et al., Reference Scholz, Biswas, Patra and Ash2022a).
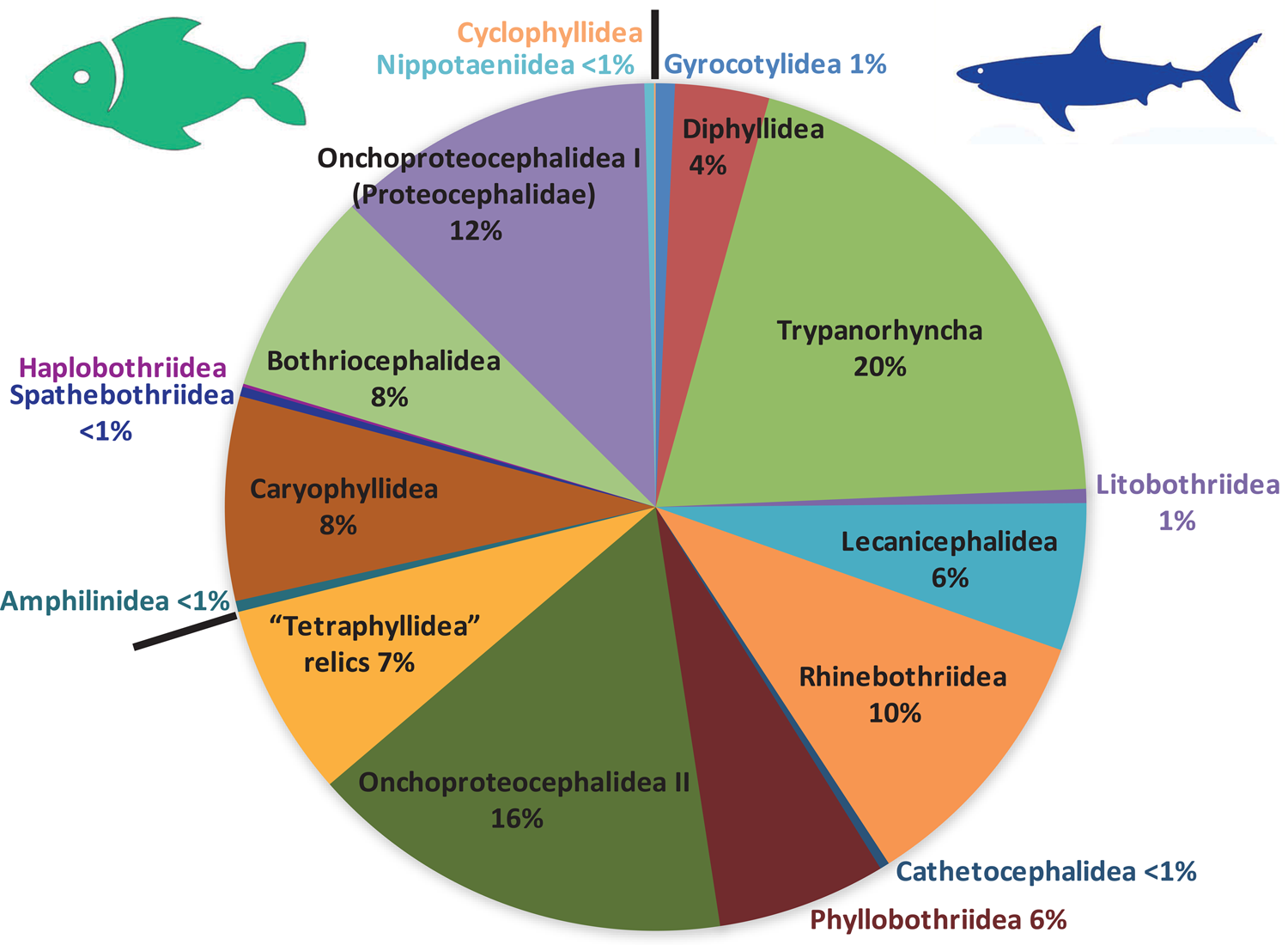
Fig. 4. Pie chart documenting species diversity of fish tapeworms, i.e. proportion of species numbers of individual orders of fish tapeworms.
Molecular tools also allowed us to discover cryptic species diversity in some groups, such as caryophyllideans of the genus Paracaryophyllaeus in loaches (Cobitoidei) in the Palaearctic region and Bothriocephalus in the Nearctic region (Scholz et al., Reference Scholz, Oros, Bazsalovicsová, Brabec, Waeschenbach, Xi, Aydogdu, Besprozvannykh, Shimazu, Králová-Hromadová and Littlewood2014; Choudhury and Scholz, Reference Choudhury and Scholz2020). However, the number of cryptic species of fish tapeworms discovered is rather low, most likely due to the predominant use of 28S rDNA sequences and the small number of specimens studied, which prevents the discovery of genetically similar but distinct taxa.
Taxonomic revisions of fish tapeworms in the Oriental region have shown that the actual number of valid species is much lower. Our recent studies of freshwater teleost tapeworms, based on new material from Bangladesh, Cambodia, India, Indonesia and Vietnam, have substantially reduced the number of valid species of teleost tapeworms in this region, where dozens of species and many ‘new’ genera have been inadequately and often invalidly described (nomina nuda or species inquirendae), mostly based on only 1 or 2 poorly fixed specimens found in a few teleost species, such as catfishes Clarias batrachus, Heteropneustes fossilis, Wallago attu, Sperata seenghala, snakeheads Channa spp. and zig-zag eels Mastacembelus spp. (Kuchta and Scholz, Reference Kuchta and Scholz2007, Reference Kuchta, Scholz, Caira and Jensen2017a). Similarly, Ash et al. (Reference Ash, Scholz, Oros and Kar2011a, Reference Ash, Scholz, Oros, Levron and Kar2011b) recognized only 9 of 78 caryophyllidean species described from C. batrachus and H. fossilis, or only 4 of 48 species of proteocephalideans described from W. attu and S. seenghala (Ash et al., Reference Ash, Scholz, de Chambrier, Brabec, Oros, Kar, Chavan and Mariaux2012, Reference Ash, de Chambrier, Shimazu, Ermolenko and Scholz2015). A similar situation also occurs in bothriocephalideans, where at least 80 species (57 since 2000) from 7 genera (Apicobothrium, Circumoncobothrium, Mastalobothrium, Polyonchobothrium, Probothriocephalus, Ptychobothrium and Senga) have been described very superficially and often in violation of the International Code of Zoological Nomenclature (Ride et al., Reference Ride, Cogger, Dupuis, Kraus, Minelli, Thompson and Tubbs1999) from a single host species, the zig-zag eel Mastacembelus armatus, in India and southeast Asia. The first and probably only valid species (and genus) from M. armatus was described by Johri (Reference Johri1956) as Senga lucknowensis (see Kuchta and Scholz, Reference Kuchta and Scholz2007; Kuchta et al., Reference Kuchta, Scholz and Bray2008b). The situation is even worse for the notorious genus Senga (and its synonyms), where no less than 152 (!) species have been described from 36 species of snakeheads and zig-zag eels in India, Pakistan and other southeast Asian countries. Provisionally, only 14 species are considered valid (Kuchta and Scholz, Reference Kuchta and Scholz2007, Reference Kuchta, Scholz, Caira and Jensen2017a; Caira et al., Reference Caira, Jensen and Barbeau2022), but the actual number of valid taxa may be even lower. A similar situation exists for S. acheilognathi, where 24 species from 5 genera are considered synonyms (see Kuchta et al., Reference Kuchta, Scholz and Bray2008b, Reference Kuchta, Choudhury and Scholz2018). Moreover, all these taxonomic changes proposed more than 10 years ago are constantly ignored by local authors who continue to describe ‘new’ taxa, including genera, from the same host species. The same problem, but to a somewhat lesser extent, exists also with the elasmobranch tapeworms of the Oriental region. Editors of scientific journals are strongly urged to avoid publishing these invalid and scientifically unsound descriptions of ‘new’ taxa, which only pollute the literature.
Phylogenetic interrelationships and new classification
Recent collaborative efforts of cestodologists have allowed us to greatly improve our understanding of the interrelationships between all groups of tapeworms and their evolutionary history. New molecular phylogenetic data have confirmed the crucial role of fish tapeworms in the evolution of all tapeworms and also led to major changes in their higher classification. These new data revealed (or confirmed) the following:
(1) Transition from monozoy to polyzoy in cestode evolution and consecutive development of the scolex from simple attachment organs to bothriate structures (1, 2 or 4 attachment grooves), followed by the development of acetabulate attachment organs (usually with 4 suckers or bothridia) (Fig. 3; Olson et al., Reference Olson, Littlewood, Bray and Mariaux2001; Waeschenbach et al., Reference Waeschenbach, Webster, Bray and Littlewood2007, Reference Waeschenbach, Webster and Littlewood2012; Caira et al., Reference Caira, Jensen, Waeschenbach, Olson and Littlewood2014). New data collected in the last 2 decades suggest the evolutionary scenario that the non-strobilate orders (Gyrocotylidea, Amphilinidea and Caryophyllidea) form the basal lineages of cestodes followed by the bothriate orders, while the acetabulate orders are the most derived (Fig. 3; Caira et al., Reference Caira, Jensen, Waeschenbach, Olson and Littlewood2014).
(2) Non-monophyly of fish tapeworms, i.e. independent, repeated colonization of elasmobranchs and teleosts with different, distantly related lineages of cestodes during their evolution (Caira et al., Reference Caira, Jensen, Waeschenbach, Olson and Littlewood2014). Molecular data confirmed at least 8 colonizations of freshwater environments (Fig. 3). Chimaeras, as members of an apparently relict vertebrate group, were most likely colonized by tapeworm progenitor represented recently only by gyrocotylideans (Kuchta et al., Reference Kuchta, Scholz, Hansen, Caira and Jensen2017; Barčák et al., Reference Barčák, Fan, Sonko, Kuchta, Scholz, Orosová, Chen and Oros2021). However, chimaeras also harbour, albeit rarely, derived tapeworms, namely Prochristianella clarkeae (broad host range) (Trypanorhyncha) and Chimaerocestos prudhoei (specific) (Phyllobothriidea).
In contrast, further colonization of elasmobranchs (rays and sharks) originally occurred in more recently diverging groups, such as trypanorhynchs and diphyllideans, but surprisingly after the colonization of marine and even freshwater teleosts (Amphilinidea, Caryophyllidea, Spathebothriidea) and probably also tetrapods (Diphyllobothriidea) and ancient freshwater bowfin fishes (Haplobothriidea) (Caira et al., Reference Caira, Jensen, Waeschenbach, Olson and Littlewood2014) (Fig. 3). However, the interrelationships between the individual orders (especially the basal ones) are not well supported by any available molecular data (Waeschenbach and Littlewood, Reference Waeschenbach, Littlewood, Caira and Jensen2017). Furthermore, reliable dating of individual colonization events is problematic because fossils of flatworms, including parasitic Neodermata, are completely lacking. However, it is assumed that the Neodermata must have parasitized the stem group Gnathostomata between the Cambrian and Ordovician (Littlewood, Reference Littlewood, Maule and Marks2006).
(3) Probably most surprising is the extraordinary diversity of elasmobranch tapeworms (Caira and Jensen, Reference Caira and Jensen2014, Reference Caira and Jensen2017; Caira et al., Reference Caira, Jensen, Waeschenbach, Olson and Littlewood2014), which belong to 8 orders and at least 9 other independent lineages that may represent new independent orders (Fig. 3).
(4) The taxonomic significance of some previously used traditional morphological characters has been questioned. The position of the internal longitudinal musculature relative to the reproductive organs used in higher classification of Caryophyllidea and Proteocephalidae (Onchoproteocephalidea I) is not congruent with molecular data (de Chambrier et al., Reference de Chambrier, Zehnder, Vaucher and Mariaux2004, Reference de Chambrier, Waeschenbach, Fisseha, Scholz and Mariaux2015; Brabec et al., Reference Brabec, Scholz, Králová-Hromadová, Baszalovicsová and Olson2012; Scholz et al., Reference Scholz, Waeschenbach, Oros, Brabec and Littlewood2021a). It is therefore obvious that this apparently homoplastic character is not suitable to define (sub)families. Also, the shape of the scolex seems to represent a homoplastic character that does not reflect the interrelationships at the level of families and even genera of some groups, such as bothriocephalideans, lecanicephalideans, proteocephalideans and ‘tetraphyllideans’ (Brabec et al., Reference Brabec, Waeschenbach, Scholz, Littlewood and Kuchta2015; de Chambrier et al., Reference de Chambrier, Waeschenbach, Fisseha, Scholz and Mariaux2015; Jensen et al., Reference Jensen, Caira, Cielocha, Littlewood and Waeschenbach2016; Caira et al., Reference Caira, Jensen, Ruhnke, Caira and Jensen2017b).
(5) New molecular phylogenetic data have led to significant changes in the classification and nomenclature of fish tapeworms at different taxonomic levels (ordinal, familial and generic). At the ordinal level, not all groups deserved changes and their ordinal status has been confirmed (see below). In contrast, taxonomic changes were made in some groups, based on the presence of independent lineages of fish tapeworms that deserve to be elevated to ordinal or subordinal rank (Fig. 3; Caira et al., Reference Caira, Jensen, Waeschenbach, Olson and Littlewood2014; Caira and Jensen, Reference Caira and Jensen2017).
Monozoic groups
The 2 most basal groups (orders) of tapeworms, namely the Gyrocotylidea (spiral intestine of the chimaeras) and the Amphilinidea (body cavities of freshwater and marine teleosts, including sturgeons, and turtles), called Cestodaria and sometimes classified as a subclass (Schmidt, Reference Schmidt1986), have a 10-hooked lycophore larva (decacanth) and a non-proglottized body without scolex, and have been considered sister groups to all other ‘true’ tapeworms (Eucestoda), which have a 6-hooked larva called a hexacanth (Conn and Świderski, Reference Conn and Świderski2008). However, the available molecular data do not support the monophyly of the ‘Cestodaria’, as the Gyrocotylidea most likely forms a sister group to all other tapeworms, including the Amphilinidea (Waeschenbach et al., Reference Waeschenbach, Webster and Littlewood2012; Caira et al., Reference Caira, Jensen, Waeschenbach, Olson and Littlewood2014). However, the interrelationships of these most basal tapeworms are not yet sufficiently resolved (Waeschenbach et al., Reference Waeschenbach, Webster and Littlewood2012; Caira and Jensen, Reference Caira and Jensen2017).
Caryophyllidea (parasites of cypriniform and siluriform teleosts, intestines, exclusively freshwater). Scholz et al. (Reference Scholz, Waeschenbach, Oros, Brabec and Littlewood2021a) presented the most comprehensive multi-gene molecular phylogeny of this group, encompassing ~50 and 75% of the known species and genus diversity, respectively (Scholz and Oros, Reference Scholz, Oros, Caira and Jensen2017). Phylogenetic reconstructions provided high support for 3 major lineages that were only partially consistent with previously recognized families. In contrast, host association and biogeographical distribution play key roles in circumscribing the 3 well-supported clades revealed by molecular data (Scholz et al., Reference Scholz, Waeschenbach, Oros, Brabec and Littlewood2021a). The most basal caryophyllideans seem to be species of Balanotaenia from catfishes in Australasia and/or Lytocestoides tanganyikae from cichlids in Africa (Scholz et al., Reference Scholz, Waeschenbach, Oros, Brabec and Littlewood2021a).
Polyzoic bothriate groups
Spathebothriidea (parasites of marine and freshwater teleosts including sturgeons) are a small group with a relatively low host specificity. Phylogenetic interrelationships have been studied by Kuchta et al. (Reference Kuchta, Pearson, Scholz, Ditrich and Olson2014), and the most basal species seems to be the marine Spathebothrium simplex with a simple scolex without specialized attachment organs.
Pseudophyllidea (parasites of a wide range of vertebrates; share the presence of bothria as attachment organs). Based on molecular, morphological and ecological evidence, the long recognized order Pseudophyllidea proposed by Carus (Reference Carus and Carus1863) was suppressed by Kuchta et al. (Reference Kuchta, Scholz, Brabec and Bray2008a) and 2 new, not closely related orders, Diphyllobothriidea and Bothriocephalidea, were proposed (see below).
Diphyllobothriidea, so-called ‘broad tapeworms’ (parasites of marine, freshwater and terrestrial tetrapods; teleosts usually serve as a second-intermediate host; formerly part of the Pseudophyllidea). The molecular interrelationships of more than 30 of 70 known species have been studied using multigene and mitogenome analyses (Waeschenbach et al., Reference Waeschenbach, Brabec, Scholz, Littlewood and Kuchta2017; Fraija-Fernández et al., Reference Fraija-Fernández, Waeschenbach, Briscoe, Hocking, Kuchta, Nyman and Littlewood2021). Three major lineages were recognized that correspond well to the 3 recognized families that include parasites of frogs (the most basal lineage), reptiles and the most derived group with parasites of birds and mammals, including humans (causing diphyllobothriosis or sparganosis). Humans were found to have been accidental hosts at least 4 times during the diphyllobothriidean evolution (Waeschenbach et al., Reference Waeschenbach, Brabec, Scholz, Littlewood and Kuchta2017). Fraija-Fernández et al. (Reference Fraija-Fernández, Waeschenbach, Briscoe, Hocking, Kuchta, Nyman and Littlewood2021) sequenced the complete mitochondrial genome (mtDNA) of 13 representatives and added nodal resolution to major branches (families) of the order.
Diphyllidea (parasites of marine batoids and rarely of sharks). This small group of small tapeworms was revised and the sequences of 31 of 59 recognized species were analysed. Based on molecular and morphological evidence, 4 new genera were proposed, with Halysioncum to be the most basal genus (Caira et al., Reference Caira, Marques, Jensen, Kuchta and Ivanov2013, Reference Caira, Ivanov, Jensen, Marques, Caira and Jensen2017c; Abbott and Caira, Reference Abbott and Caira2014).
Trypanorhyncha (most species-rich order of elasmobranch tapeworms; larvae in invertebrates and teleosts; with 2 species, Paroncomegas araya and Paroncomegas baeri, specific parasites of freshwater stingrays in South America). Two robust molecular phylogenetic studies based on extensive datasets (66 of 335 known species) have been published (Palm et al., Reference Palm, Waeschenbach, Olson and Littlewood2009; Olson et al., Reference Olson, Caira, Jensen, Overstreet, Palm and Beveridge2010). Two new, well-supported suborders (lineages) have been erected, Trypanobatoida and Trypanoselachoida, which parasitize primarily rays and sharks, respectively (Olson et al., Reference Olson, Caira, Jensen, Overstreet, Palm and Beveridge2010). The interrelationships of this species-rich order are not yet adequately understood, because available molecular data represent only about one-fifth of the known taxa (Beveridge et al., Reference Beveridge, Haseli, Ivanov, Menoret, Schaeffner, Caira and Jensen2017).
Bothriocephalidea (parasites of marine and freshwater teleosts and rarely of amphibians; formerly part of the Pseudophyllidea). Brabec et al. (Reference Brabec, Waeschenbach, Scholz, Littlewood and Kuchta2015) evaluated the interrelationships of this order using multi-gene molecular phylogenetic analyses that included 59 of 135 recognized species, covering 70% of recognized genera. The order proved to be monophyletic, but 3 of 4 recognized families appeared non-monophyletic. Only the family Bothriocephalidae is monophyletic, and forms the most derived lineage of the order which comprises a single freshwater clade and several marine clades (including the most species-rich and polyphyletic genus Bothriocephalus). Biogeographical patterns within the freshwater clade indicate that monophyletic lineages have evolved in Africa and North America (Brabec et al., Reference Brabec, Waeschenbach, Scholz, Littlewood and Kuchta2015). The most basal genera seem to be fresh water (Marsipometra and/or Bathybothrium) or marine (Abothrium or Parabothrium) (Brabec et al., Reference Brabec, Waeschenbach, Scholz, Littlewood and Kuchta2015).
Polyzoic acetabulate groups
Litobothriidea (small group in 1 genus; parasitize exclusively pelagic sharks). With one exception (enigmatic Litobothrium aenigmaticum), all possess a scolex consisting of a single apical sucker followed by a series of pseudosegments, part of which is cruciform (Caira et al., Reference Caira, Gallagher, Jensen, Caira and Jensen2017d). In contrast, the scolex of L. aenigmaticum consists of a simple dome-shaped scolex proper and an extended cephalic peduncle housing 4 distinct tissue types (Caira et al., Reference Caira, Gallagher, Jensen, Caira and Jensen2017d). The order Lithobothriidea is considered a sister taxon to all acetabulate tapeworms (Fig. 3; Caira and Jensen, Reference Caira and Jensen2014; Caira et al., Reference Caira, Jensen, Waeschenbach, Olson and Littlewood2014, Reference Caira, Gallagher, Jensen, Caira and Jensen2017d).
Lecanicephalidea (parasites of elasmobranchs, mainly rays; with only a few freshwater species). Jensen et al. (Reference Jensen, Caira, Cielocha, Littlewood and Waeschenbach2016) presented the first assessment of the phylogenetic interrelationships of the order and confirmed its monophyly. However, 61 species representing 22 of the 25 valid genera were found to have an apparent conflict between scolex morphology and proglottid anatomy, complicating the assignment of many of these genera to families. Proglottid anatomy was found to be much more conserved and informative of phylogenetic relationships than scolex morphology, and genera lacking apical structures (such as the Paraberrapecidae) were confirmed to be the earliest diverging lineages (Jensen et al., Reference Jensen, Caira, Cielocha, Littlewood and Waeschenbach2016). The order is divided into 8 families based on molecular data (Jensen et al., Reference Jensen, Caira, Cielocha, Littlewood and Waeschenbach2016, Reference Jensen, Cielocha, Herzog, Caira, Caira and Jensen2017).
Rhinebothriidea (formerly part of the Tetraphyllidea; parasites of elasmobranchs, with 15 known species in freshwater rays). The order was erected by Healy et al. (Reference Healy, Caira, Jensen, Webster and Littlewood2009) based on molecular data and is characterized by the possession of a scolex consisting of 4 bothridia borne on stalks. Ruhnke et al. (Reference Ruhnke, Caira and Cox2015, Reference Ruhnke, Caira, Pickering, Caira and Jensen2017) proposed 4 families of the order and erected several new genera. The order contains the second most species-rich genus of elasmobranch tapeworms, Rhinebothrium, with 63 valid species, including 11 species from freshwater rays in South America that form a monophyletic group with species parasitizing potamotrygonid stingrays (Reyda and Marques, Reference Reyda and Marques2011), and 2 species from freshwater whipray in Borneo (Healy, Reference Healy2006) that are not closely related to the Neotropical taxa (Ruhnke et al., Reference Ruhnke, Caira, Pickering, Caira and Jensen2017). Moreover, 1 Anindobothrium species from Amazonian potamotrygonids and Sungaicestus kinabatanganensis from Bornean giant freshwater whipray have been recently discovered (Ruhnke et al., Reference Ruhnke, Caira, Pickering, Caira and Jensen2017, Trevisan et al., Reference Trevisan, Primon and Marques2017). Rhinebothriideans adapted to a freshwater environment at least 4 times, with the most basal species appearing to be new genus 11 sensu Ruhnke et al. (Reference Ruhnke, Caira and Cox2015) (Ruhnke et al., Reference Ruhnke, Caira, Pickering, Caira and Jensen2017).
Cathetocephalidea (formerly part of the Tetraphyllidea; parasites of pelagic sharks). This is a very small but peculiar order characterized by a scolex consisting of an anterior globose or transversely extended region and a posterior rugose base with or without a papillary band (Caira and Jensen, Reference Caira and Jensen2014). Caira et al. (Reference Caira, Jensen, Waeschenbach, Olson and Littlewood2014) found this small order deeply nested among the acetabulate orders of cestodes. Sanguilevator yearsleyi from the broadfin shark Lamiopsis tephrodes off Borneo has a unique scolex with internal chambers and channels that appear to sequester host blood cells, making it possibly the only known tapeworm species that ingests blood (Caira et al., Reference Caira, Mega and Ruhnke2005).
Phyllobothriidea (formerly part of the Tetraphyllidea; parasites of elasmobranchs; 1 species in fresh water). Based on their molecular phylogenetic analyses, Caira et al. (Reference Caira, Jensen, Waeschenbach, Olson and Littlewood2014) elevated the family Phyllobothriidae to ordinal status. Caira et al. (Reference Caira, Bueno and Jensen2021a, Reference Caira, Otto, Fernando and Jensen2021b) provided the most comprehensive phylogenetic analysis of this order; their dataset included species from 15 of the 18 genera, as well as 3 new genera. Members of the order have simple, undivided bothridia, each bearing an apical sucker. The most species-rich genus is now Scyphophyllidium with 45 species, as 6 genera have been synonymized with this genus, and the most basal genus appears to be Clistobothrium (Caira et al., Reference Caira, Jensen, Hayes and Ruhnke2020a, Reference Jensen, Pen and Caira2021). Surprisingly, 1 species of Scyphophyllidium, originally described as Anindobothrium guariticus and later transferred to Nandocestus, was described from freshwater potamotrygonid stingrays in South America (Caira et al., Reference Caira, Bueno and Jensen2021a).
Onchoproteocephalidea (a highly controversial order consisting of members of the long recognized order Proteocephalidea parasitizing freshwater teleosts and tetrapods – Onchoproteocephalidea I, and taxa of the former ‘tetraphyllidean’ family Onchobothriidae from elasmobranchs – Onchoproteocephalidea II). Caira et al. (Reference Caira, Jensen, Waeschenbach, Olson and Littlewood2014) proposed this new order based on molecular data, but no morphological, biological or ecological synapomorphies for the order have been identified to characterize this rather heterogeneous assemblage of tapeworms.
Tapeworms of the former order Proteocephalidea (Onchoproteocephalidea I) are parasites of freshwater teleosts (60% of species) and some tetrapods (amphibians, reptiles and 1 in mammals) that are currently placed in a single family, Proteocephalidae (see de Chambrier et al., Reference de Chambrier, Scholz, Mariaux, Kuchta, Caira and Jensen2017). The most comprehensive phylogenetic study included more than 100 of 334 now recognized species from 54 genera (80% of all recognized genera) (de Chambrier et al., Reference de Chambrier, Waeschenbach, Fisseha, Scholz and Mariaux2015). The results confirmed the origin of proteocephalideans in the Old World with the most basal groups appearing in catfishes (Gangesiinae) or reptiles (Acanthotaeniinae), with more recent dispersal followed by radiation in teleosts, particularly pimelodid catfishes in South America. Most of the subfamilies and species-rich genera proved to be non-monophyletic, requiring a profound systematic reorganization of this cestode group, abandoning the traditional subfamily system (de Chambrier et al., Reference de Chambrier, Waeschenbach, Fisseha, Scholz and Mariaux2015, Reference de Chambrier, Scholz, Mariaux, Kuchta, Caira and Jensen2017).
Onchoproteocephalidea II of Caira and Jensen (Reference Caira and Jensen2017) consists of hooked cestodes parasitizing rays and sharks. They encompass the most species-rich genus of elasmobranch tapeworms Acanthobothrium with 211 valid species (Caira et al., Reference Caira, Jensen and Barbeau2022; Van der Spuy et al., Reference Van Der Spuy, Smit and Schaeffner2022), including 11 species described from giant freshwater whipray in Borneo and potamotrygonid stingrays in South America (Fyler and Caira, Reference Fyler and Caira2006; Caira et al., Reference Caira, Jensen and Barbeau2022). Moreover, all 7 species of Potamotrygonocestus with unique acetabula and unipronged hooks are specific parasites of potamotrygonid stingrays in South America (Marques et al., Reference Marques, Brooks and Araujo2003) and may represent elasmobranch tapeworms most closely related to proteocephalideans (Caira et al., Reference Caira, Jensen, Ivanov, Caira and Jensen2017e).
Nippotaeniidea (small group, exclusive in freshwater teleosts). They have only been found in freshwater gobiids (Perciformes) and osmeroids (Osmeriformes) in the Palaearctic region and New Zealand (Scholz et al., Reference Scholz, Brabec, Kuchta, Caira and Jensen2017a). They are closely related to the Tetrabothriidea, Cyclophyllidea (both groups parasitize tetrapods) and some tetraphyllidean relicts, but their relationships are still not clearly resolved (Caira et al., Reference Caira, Jensen, Waeschenbach, Olson and Littlewood2014; Waeschenbach and Littlewood, Reference Waeschenbach, Littlewood, Caira and Jensen2017).
Cyclophyllidea (mature in tetrapods; 1 species has recently been described from freshwater teleosts). This is the most species-rich order, comprising over 3000 species (more than 60% of tapeworm diversity) that are parasites of tetrapods, especially birds and mammals, including humans (Mariaux et al., Reference Mariaux, Tkach, Vasileva, Waeschenbach, Beveridge, Dimitrova, Haukisalmi, Greiman, Littlewood, Makarikov, Phillips, Razafiarisolo, Widmer, Georgiev, Caira and Jensen2017). However, adults had never been found in bony fishes until Scholz et al. (Reference Scholz, Tavakol and Luus-Powell2020) described the dilepidid Ichthyolepis africana in 6 species of elephantfishes (Mormyriformes) across Africa. The new species belongs to the dilepidid lineage consisting of tapeworms of African swifts (Apodidae) (Scholz et al., Reference Scholz, Tavakol and Luus-Powell2020).
‘Tetraphyllidea’ relicts. This group tentatively includes more than 100 species of acetabulate elasmobranch tapeworms with highly diverse morphologies that form at least 9 independent lineages of acetabulate tapeworms that may represent new orders (Caira et al., Reference Caira, Jensen, Ruhnke, Caira and Jensen2017b) (Fig. 3). Recently, numerous morphological and molecular data have been added to these groups, including descriptions of new genera such as Ambitalveolus (Eudy et al., Reference Eudy, Caira and Jensen2019; Caira et al., Reference Jensen, Pen and Caira2021; Caira and Jensen, Reference Caira and Jensen2021, Reference Caira and Jensen2022; Jensen et al., Reference Jensen, Pen and Caira2021; Sadeghi Kamachali and Haseli, Reference Sadeghi Kamachali and Haseli2022; Stephan and Caira, Reference Stephan and Caira2022).
(6) Several genera of fish tapeworms, including taxa of medical and veterinary importance, were found to be non-monophyletic, resulting in nomenclatural changes at the genus level. For example, the bothriocephalidean genus Schyzocotyle was resurrected by Brabec et al. (Reference Brabec, Waeschenbach, Scholz, Littlewood and Kuchta2015) to accommodate the important fish pathogen and invasive Asian fish tapeworm S. acheilognathi (see Scholz et al., Reference Scholz, Kuchta, Williams, Woo and Buchmann2012; Kuchta et al., Reference Kuchta, Choudhury and Scholz2018).
A molecular phylogenetic study by Waeschenbach et al. (Reference Waeschenbach, Brabec, Scholz, Littlewood and Kuchta2017) resulted in several taxonomic changes in the genera containing human-infecting broad tapeworms (Diphyllobothriidea), especially resurrection of the genus Dibothriocephalus that accommodates freshwater and terrestrial species, including human parasites, such as D. latus, D. nihonkaiensis and D. dendriticus, and the genus Adenocephalus with the Pacific broad tapeworm that infects humans on the Pacific coast of South America, with marine teleosts serving as a source of human infections (Kuchta et al., Reference Kuchta, Serrano-Martinez and Scholz2015), and the synonymy of Diplogonoporus (including parasites of humans) with Diphyllobothrium by Waeschenbach et al. (Reference Waeschenbach, Brabec, Scholz, Littlewood and Kuchta2017).
Host associations
As noted above, both elasmobranchs and teleosts were independently colonized several times during the evolution of tapeworms, including the most basal gyrocotylideans, amphilinideans and caryophyllideans, but also recently by divergent acetabulate groups such as nippotaeniideans (Caira et al., Reference Caira, Jensen, Waeschenbach, Olson and Littlewood2014; Fig. 3). In elasmobranchs, the next colonization event after the establishment of gyrocotylidean–holocephalan association occurred much later, most likely by ancestors of the recent Trypanorhyncha and Diphyllidea, which expanded in both sharks and rays (Caira et al., Reference Caira, Jensen, Waeschenbach, Olson and Littlewood2014, Reference Caira, Ivanov, Jensen, Marques, Caira and Jensen2017c; Beveridge et al., Reference Beveridge, Haseli, Ivanov, Menoret, Schaeffner, Caira and Jensen2017). Using a relaxed molecular clock model calibrated by host fossil data, the Trypanobatoida of rays was estimated to have diversified around the Jurassic–Cretaceous boundary, whereas the Trypanoselachoida of sharks was estimated to have diversified later, in the mid-Cretaceous (Olson et al., Reference Olson, Caira, Jensen, Overstreet, Palm and Beveridge2010).
Teleosts have also been colonized independently several times (Fig. 3). Tapeworms occur in their most basal groups, i.e. the 2 orders of the most basal superorder Chondrostei, namely Polypteriformes and Acipenseriformes (proteocephalideans, bothriocephalideans and amphilinideans – Kuchta et al., Reference Kuchta, Burianová, Jirků, de Chambrier, Oros, Brabec and Scholz2012; de Chambrier et al., Reference de Chambrier, Scholz, Mariaux, Kuchta, Caira and Jensen2017; Scholz and Kuchta, Reference Scholz, Kuchta, Caira and Jensen2017b). Fishes of both orders of another ancient superorder Neopterygii (orders Amiiformes and Lepisosteiformes) have also been colonized by relatively derived proteocephalidean tapeworms, and bowfin (Amia calva) also by haplobothriideans (Brabec et al., Reference Brabec, Waeschenbach, Scholz, Littlewood and Kuchta2015; Scholz et al., Reference Scholz, Biswas, Patra and Ash2022a, Reference Scholz, Choudhury and McAllister2022b). Adult tapeworms also occur in the most basal superorder Teleostei, as in the Osteoglossiformes (2 amphilinideans in Arapaima gigas), Elopiformes (bothriocephalideans) and Anguilliformes (1 proteocephalidean and 2 bothriocephalideans) (de Chambrier et al., Reference de Chambrier, Scholz, Mariaux, Kuchta, Caira and Jensen2017; Kuchta and Scholz, Reference Kuchta, Scholz, Caira and Jensen2017a; Scholz and Kuchta, Reference Scholz, Kuchta, Caira and Jensen2017b). With the exception of amphilinideans, all of the above-mentioned tapeworms belong to more derived groups, so their colonization of ancient fish groups was apparently the result of host switching.
Bothriocephalideans have spread in marine and freshwater teleosts, with a few taxa switching to amphibians (Kuchta and Scholz, Reference Kuchta, Scholz, Caira and Jensen2017a). Further colonization of freshwater teleosts has occurred much later by members of acetabulate tapeworms (Fig. 3), particularly proteocephalideans, which have adapted to an extraordinarily wide range of distantly related orders, including basal ray-finned species such as the Polypteriformes, Amiiformes and Lepisosteiformes, as well as the recently divergent orders Centrarchiformes, Cichliformes, Gobiiformes and ‘Perciformes’, but also other vertebrate groups (de Chambrier et al., Reference de Chambrier, Scholz, Mariaux, Kuchta, Caira and Jensen2017).
Species of 4 orders (Caryophyllidea, Haplobothriidea, Onchoproteocephalidea I and Nippotaeniidea) live exclusively in fresh water. In the Spathebothriidea, the most basal extant species (S. simplex) is a parasite of marine snailfish, while the most basal bothriocephalideans may occur in freshwater hosts (see above; Brabec et al., Reference Brabec, Waeschenbach, Scholz, Littlewood and Kuchta2015). Interestingly, the number of marine species among teleost tapeworms accounts only for 22% of known species (Scholz and Kuchta, Reference Scholz and Kuchta2017a; Table 1).
There are 1200 described species of elasmobranch fishes (Last et al., Reference Last, White, de Carvalho, Séret, Stehmann and Naylor2016), of which an estimated 40% have been examined for tapeworms from which almost 1200 species have been described (Table 2; Caira et al., Reference Caira, Jensen, Georgiev, Kuchta, Littlewood, Mariaux, Scholz, Tkach, Waeschenbach, Caira and Jensen2017a). Nearly all elasmobranch species examined for parasites harbour at least 1 tapeworm species (Caira et al., Reference Caira, Jensen, Healy, Littlewood and Bray2001), but each elasmobranch species may harbour an average of 6 tapeworm species, 4 of which have strict host specificity (Randhawa and Poulin, Reference Randhawa and Poulin2010). In addition, it is estimated that there are at least 3600 undescribed tapeworm species from ‘known’ elasmobranchs (Randhawa and Poulin, Reference Randhawa and Poulin2010; Caira et al., Reference Caira, Jensen, Georgiev, Kuchta, Littlewood, Mariaux, Scholz, Tkach, Waeschenbach, Caira and Jensen2017a). Most species of elasmobranch tapeworms were described after 1986 (Fig. 1; Randhawa and Poulin, Reference Randhawa and Poulin2019).
The number of known teleosts is much higher, with at least 35 000 described species (10 000 in fresh water and 15 000–20 000 marine) (Reis et al., Reference Reis, Albert, Di Dario, Mincarone, Petry and Rocha2016). To date, only a small number of them has been examined for parasites. A large sample of more than 8000 freshwater teleosts of at least 300 species from 5 bioregions revealed an overall prevalence of 16%, with the highest species diversity in the Neotropical realm (Kuchta and Scholz, Reference Kuchta, Scholz, Caira and Jensen2017a).
In total 101 proteocephalidean species from 81 catfish species have been reported in South America, representing an average of 1.2 proteocephalidean species per host species (Alves et al., Reference Alves, de Chambrier, Scholz and Luque2017; present data). Catfishes are probably the most suitable teleost group in which new tapeworms are to be discovered, but the known species diversity is still very low when compared to the diversity of elasmobranch tapeworms. The diversity of catfishes in South America alone is much higher than the total diversity of elasmobranchs in the whole world (estimated at 1915 spp. by Reis et al., Reference Reis, Albert, Di Dario, Mincarone, Petry and Rocha2016). When extrapolated to the currently recognized species of proteocephalideans, the total diversity of proteocephalideans in South American siluriforms could reach 2400 species. However, this is almost certainly overestimation because proteocephalideans are mainly found in members of a single family Pimelodidae, and 85 proteocephalideans have been reported from 38 pimelodid species, i.e. 2.2 tapeworm species per host. Considering the existence of at least 113 pimepodid species (based on Froese and Pauly, Reference Froese and Pauly2022), there could be at least 250 proteocephalidean species just in fishes of this single family alone. In contrast, species diversity of proteocephalideans in other catfishes is much lower (they occur in only another 8 families). Overall, rough estimates of proteocephalidean diversity in South America vary between 1000 and 2000 species, suggesting that we have described only 5–10% of the freshwater teleost tapeworms of South America.
The prevalence and diversity of tapeworms in marine teleosts is low and also varies among different ocean depths. The shelf, epipelagic (up to 200 m) and mesopelagic fauna (up to 1000 m) is relatively poor, whereas the cestode fauna of deep-sea teleosts appears to be much richer. Kuchta and Scholz (Reference Kuchta and Scholz2007) reported that only 4 of 500 teleosts of 37 species from 11 sites in the epipelagic Atlantic Ocean off Scotland were infected with only 2 species of adult tapeworms. In contrast, 20 of 286 teleosts from 42 species in the deep sea off the Outer Hebrides were infected with 5 species of bothriocephalideans (Kuchta and Scholz, Reference Kuchta and Scholz2007).
Among marine teleosts, only the family Centrolophidae (Scombriformes) represents a ‘hot spot’ of tapeworm diversity. To date, 16 bothriocephalid species have been reported from 9 centrolophid species, corresponding to 1.8 tapeworm species per host species. Considering the existence of 32 species of centrolophids (Froese and Pauly, Reference Froese and Pauly2022), there could be as many as 57 species of bothriocephalid tapeworms.
Caira and Jensen (Reference Caira and Jensen2014, Reference Caira and Jensen2017) reviewed all groups of elasmobranch tapeworms, which are generally strictly host-specific (oioxenous; strict specialists), with the exception of Trypanorhyncha, where strict host specificity at the species level is not the general pattern (Palm, Reference Palm2004; Caira and Jensen, Reference Caira and Jensen2014). A remarkable case of suprageneric specialist or even low generalist (euryxeny) has also been reported for some species of Rhinebothrium by Golzarianpour et al. (Reference Golzarianpour, Malek, Golestaninasab, Sarafrazi and Kochmann2021). In general, tapeworms are parasites of all groups of elasmobranchs (with the greatest diversity in rays), including recent freshwater lineages in South America and Southeast Asia (Caira and Jensen, Reference Caira and Jensen2014, Reference Caira and Jensen2017).
Scholz and Kuchta (Reference Scholz and Kuchta2017a) found no general patterns in host use at the level of definitive hosts of teleost tapeworms, as they are found in distantly related fish groups, particularly proteocephalideans (see above). They parasitize mainly fishes of 3 teleost orders, namely Siluriformes, Cypriniformes and ‘Perciformes’ (=sensu lato). More than 60% of teleost tapeworms are specialists (Scholz and Kuchta, Reference Scholz and Kuchta2017a). Extremely low host specificity (euryxenous; high generalists) was rarely observed (8%), mainly among bothriocephalideans, including the most opportunistic helminth, S. acheilognathi, which has been reported from more than 300 freshwater actinopterygian species as well as axolotls, snakes and birds (Kuchta et al., Reference Kuchta, Choudhury and Scholz2018).
Kuchta et al. (Reference Kuchta, Řehulková, Francová, Scholz, Morand and Šimková2020) critically revised tapeworms of cypriniform fishes known in Europe and North America and found that their host specificity is more strict than previously hypothesized by Scholz and Kuchta (Reference Scholz and Kuchta2017a), primarily due to the predominance of highly specific caryophyllideans in Nearctic catostomids (Uhrovič et al., Reference Uhrovič, Oros, Kudlai, Choudhury and Scholz2021a, Reference Uhrovič, Oros, Reyda, Kudlai and Scholz2021b, Reference Uhrovič, Oros, Kudlai, Kuchta and Scholz2022).
Life cycles
The life cycles and transmission patterns of fish tapeworms, including marine taxa, are poorly understood (Williams and Jones, Reference Williams and Jones1994; Beveridge, Reference Beveridge, Littlewood and Bray2001; Poulin et al., Reference Poulin, Blasco-Costa and Randhawa2016). To date, at least 1 complete cycle is known for representatives of only 11 of the 18 tapeworm orders (Table 3). Life cycles are best known in 2 freshwater groups, proteocephalideans and caryophyllideans (Table 3). In elasmobranch tapeworms, life cycles are clarified only for a few species of the Trypanorhyncha and Rhinebothriidea. The first-intermediate hosts are mainly copepods (confirmed in 7 orders), less frequently amphipods or crayfish (Amphlinidea, Spathebothriidea) or oligochaetes (Caryophyllidea). The second-intermediate host is not required in fish tapeworms of at least 6 orders, but may be involved in at least 7 orders, with teleost fish (including the cyclophyllidean family Gryporhynchidae) or rarely crustaceans and cephalopods serving as second-intermediate hosts (Table 3). Tetrapods, including humans, may also serve as intermediate or accidental hosts in some taxa of the Cyclophyllidea (Mesocestoidae, Taeniidae) and in the Diphyllobothriidea (Spirometra).
Table 3. Survey of life cycles by tapeworm orders

?, no cycle known.
a Number of species with known complete life cycle.
b Jensen and Bullard (Reference Jensen and Bullard2010).
The main obstacles to studying life cycles are the lack of diagnostic features in larval stages and the difficulty of maintaining experimental hosts in the laboratory, particularly marine invertebrates and fish. The application of DNA sequencing allowed the assignment of different ontogenetic stages of tapeworms, which helped to elucidate their development (Aznar et al., Reference Aznar, Agustí, Littlewood, Raga and Olson2007; Jensen and Bullard, Reference Jensen and Bullard2010; Caira et al., Reference Caira, Jensen, Pickering, Ruhnke and Gallagher2020b). For example, Caira et al. (Reference Caira, Jensen, Pickering, Ruhnke and Gallagher2020b) confirmed molecularly the systematic position of well-known larvae infecting marine mammals tentatively designated as Monorygma grimaldii and Phyllobothrium delphini, as well as several larvae from marine teleosts that are molecularly related to the phyllobothriidean genus Clistobothrium.
Recently, Appy et al. (Reference Appy, Goffredi, Pernet and Latino2019) experimentally investigated the life cycle of Rhinebothrium urobatidium (Rhinebothriidea) from the round stingray Urobatis halleri off California and found that the copepod Tigriopus californicus (Harpacticoidea) and gobies (Clevelandia ios) serve as the first- and second-intermediate hosts, respectively. This is the first completed life cycle of a rhinebothriidean tapeworm and the fourth known cycle of elasmobranch tapeworms (Table 3).
Zoogeographical distribution
The biogeographical data collected in the last 2 decades also made it possible to significantly expand the distributional ranges of many fish tapeworms. The distributional ranges of marine tapeworms are more extensive compared to those of freshwater taxa and even span most of the globe. Overall, tropical and subtropical areas are richest in elasmobranchs (Naylor et al., Reference Naylor, Caira, Jensen, Rosana, Straube, Lakner, Carrier, Musick and Heithaus2012a, Reference Naylor, Caira, Jensen, Rosana, White and Last2012b) as well as their tapeworms (Caira and Jensen, Reference Caira and Jensen2014, Reference Caira and Jensen2017; Randhawa and Poulin, Reference Randhawa and Poulin2019). However, for some species of trypanorhynchs or phyllobothriideans, a near cosmopolitan distribution including Arctic areas has been demonstrated (Palm et al., Reference Palm, Waeschenbach and Littlewood2007; Beveridge et al., Reference Beveridge, Haseli, Ivanov, Menoret, Schaeffner, Caira and Jensen2017; Caira and Jensen, Reference Caira and Jensen2017).
The distribution of teleost tapeworms differs markedly from one another, with no obvious general pattern (see Scholz and Kuchta, Reference Scholz and Kuchta2017a). However, individual lineages are generally restricted to a single continent or biogeographical region (Amphilinidea, Bothriocephalidea, Caryophyllidea, Nippotaeniidea and Proteocephalidae). Particularly little is known about the distribution of ‘deep-sea’ fish tapeworms (Kuchta et al., Reference Kuchta, Scholz and Bray2008b; Klimpel et al., Reference Klimpel, Busch, Kellermanns, Kleinertz and Palm2009; Brabec et al., Reference Brabec, Waeschenbach, Scholz, Littlewood and Kuchta2015). Most tapeworms of freshwater teleosts (and those of freshwater rays) have much more restricted distributions, usually confined to 1, at most 2, zoogeographical regions. Teleost tapeworms are more diverse in the temperate zones of the Northern Hemisphere, i.e. Nearctic and Palaearctic regions, than in the tropics, with the exception of proteocephalideans in the Neotropical region, where they account for up to 95% of all tapeworm species (with the exception of 2 amphilinideans and 4 bothriocephalideans) (Scholz and Kuchta, Reference Scholz and Kuchta2017a). Caryophyllideans dominate in the Nearctic region, along with proteocephalideans also in the Ethiopian and Palaearctic realms.
Unnatural distributions as a result of human activities are exhibited by invasive species that include caryophyllideans (A. huronensis, Khawia japonica, K. sinensis), bothriocephalideans (S. acheilognathi) and nippotaeniideans (Nippotaenia perccotti) (Scholz and Kuchta, Reference Scholz and Kuchta2017a). Caryophyllideans and S. acheilognathi have been introduced to Europe, North America, Australia and Africa probably from East Asia by infected carps (Cyprinus carpio and Ctenopharyngodon idella) (Oros et al., Reference Oros, Hanzelová and Scholz2004, Reference Oros, Scholz and Hanzelová2009; Scholz et al., Reference Scholz, Tavakol, Halajian and Luus-Powell2015, Reference Scholz, Barčák and Oros2018a; Kuchta et al., Reference Kuchta, Choudhury and Scholz2018), but N. perccotti was introduced relatively recently from Asia to Central and Eastern Europe by the invasive Chinese sleeper Perccottus glenii (Mierzejewska et al., Reference Mierzejewska, Kvach, Woźniak and Kosowska2012; Kvach et al., Reference Kvach, Drobiniak, Kutsokon and Hoch2013).
Tapeworms as fish pathogens and causative agents of human fish-borne zoonoses
There are relatively few fish tapeworms that can be transmitted to humans. The eggs of invasive S. acheilognathi have been detected once in the stool of a human, but most likely as an incidental infection (Yera et al., Reference Yera, Kuchta, Brabec, Peyron and Dupouy-Camet2013). Moreover, some trypanorhynch larvae such as Hepatoxylon trichiuri and Nybelinia surmenicola have been reported (as accidental pseudoparasites) in 4 cases, apparently after accidentally ingesting an infected second-intermediate host (Heinz, Reference Heinz1954; Grimmo and Buckley, Reference Grimmo and Buckley1961; Ikikuchi et al., Reference Ikikuchi, Takenouchi, Kamiya and Ozaki1981; Fripp and Mason, Reference Fripp and Mason1983). In fact, only the larvae (plerocercoids) of a few fish diphyllobothriideans (mainly D. latus, D. nihonkaiensis and Adenocephalus pacificus) can cause the disease called diphyllobothriosis in humans (see Scholz and Kuchta, Reference Scholz and Kuchta2016; Scholz et al., Reference Scholz, Kuchta and Brabec2019; Králová-Hromadová et al., Reference Králová-Hromadová, Radačovská, Čisovská Bazsalovicsová and Kuchta2021).
There are also relatively few cestodes, especially their larvae (metacestodes), that are actually pathogenic to fish (Williams and Jones, Reference Williams and Jones1994; Dick et al., Reference Dick, Chambers, Isinguzo and Woo2006; Scholz et al., Reference Scholz, Kuchta and Oros2021b, Reference Scholz, Davidovich, Aflalo, Hadar, Mazuz and Yasur-Landau2021c). Plerocercoids of species of Dibothriocephalus are commonly found in the viscera and musculature of freshwater and marine teleosts, especially salmonids, including those in aquaculture, and can adversely affect heavily infected fish (Williams and Jones, Reference Williams and Jones1994; Scholz et al., Reference Scholz, Kuchta and Oros2021b). Plerocercoids of Proteocephalus ambloplitis in North American centrarchids and larvae of trypanorhynch tapeworms in marine teleosts may also pose a threat to local fish populations because of their pathogenicity (Williams and Jones, Reference Williams and Jones1994).
Invasive parasites are a major threat to biodiversity and non-native parasites introduced to new regions can also negatively affect the health of cultured fish in newly colonized regions. Parasites introduced into new areas with their non-native hosts alter natural host–parasite interactions, which can lead to the emergence of disease in native species (Šimková et al., Reference Šimková, Řehulková, Rasoloariniaina, Jorissen, Scholz, Faltýnková, Mašová and Vanhove2019). Most invasive tapeworms are found in cyprinids, particularly common carp, which have contributed to the spread of several successful invaders among tapeworms (see above).
Unresolved problems and prospects of future research
Despite vast progress over the past 2 decades, it is evident that only a small fraction of the global cestode diversity, including fish tapeworms, has been adequately described. The following obstacles should be overcome in future research to better characterize the true diversity of extant tapeworms in elasmobranchs and teleosts:
(1) Limited accessibility of potential fish hosts. Serious barriers include restrictions related to the availability of less common or protected hosts, and restrictions on the export of biological samples based on the Nagoya Protocol (Nagoya Protocol on Access to Genetic Resources and the Fair and Equitable Sharing of Benefits Arising from their Utilization to the Convention on Biological Diversity), which seriously impair or even prevent any international collaboration in research. Limited accessibility to biological samples, both hosts and their parasites, can have negative consequences for the advancement of biodiversity studies, especially in countries with the strictest Nagoya Protocol regulations.
(2) Methodological obstacles. A seemingly very trivial issue, proper processing of tapeworm samples, remains one of the major obstacles in tapeworm studies. The unfortunate practice of studying long dead, non-fresh or frozen fish hosts, and the use of inappropriate fixation or inadequate processing (flattening, relaxation or use of fixatives in the case of teleost tapeworms, lack of ethanol-fixed vouchers for molecular studies, etc.), as well as headless and/or fragmented specimens, lead to poor and incomplete morphological descriptions of new taxa, including where internal structures are often misinterpreted or not adequately characterized (Oros et al., Reference Oros, Scholz, Hanzelová and Mackiewicz2010; Scholz et al., Reference Scholz, Vanhove, Smit, Jayasundera and Gelnar2018b). It is strongly recommended to collect only complete tapeworms with the scolex from freshly killed fish after they have been gently cleaned in saline and fixed in hot fixative (for teleost tapeworms), including preservation of molecular vouchers, i.e. hologenophores/paragenophores (Pleijel et al., Reference Pleijel, Jondelius, Norlinder, Nygren, Oxelman, Schander, Sundberg and Thollesson2008; Scholz et al., Reference Scholz, Vanhove, Smit, Jayasundera and Gelnar2018b).
(3) Correct host identification. Host taxonomy and nomenclature are constantly changing (especially for elasmobranchs with over 300 new species described in the last 20 years), which can complicate their correct identification and thus assessment of the range of cestode host specificity. It is strongly recommended that tapeworm hosts always be adequately documented by photographs and tissue samples for DNA genotyping for each infected host should be deposited (Caira and Jensen, Reference Caira and Jensen2014; Scholz et al., Reference Scholz, Vanhove, Smit, Jayasundera and Gelnar2018b).
(4) Taxonomic crisis. The recent biodiversity crisis is accompanied by a taxonomic crisis, i.e. a continuous loss of taxonomic expertise, including limited funding of taxonomic studies. Factors that have led to this crisis include unfavourable evaluation of taxonomy by other scientists, insufficient funding, declining effort to properly identify specimens in biodiversity studies, methods of global diversity assessment, penalization of large, long-term work and faunal surveys and bureaucratic obstacles that hinder fieldwork (Löbl, Reference Löbl2018).
(5) International collaborations. The exceptional achievements of the international cestode consortium of the projects funded by NSF-PBI (Caira and Jensen, Reference Caira and Jensen2017) are strong evidence of the need for international scientific collaboration in future studies on fish tapeworms. The greater the number of authors per study, the higher its quality, suggesting that more collaborations between taxonomists and experts from other fields are needed to produce more comprehensive species characterizations (Poulin and Presswell, Reference Poulin and Presswell2016).
Our understanding of tapeworm evolution and phylogenetic relationships has improved considerably, especially for fish tapeworms. However, several important questions still remain unanswered, in part because of the lack of resolution of tapeworm relationships at the order level, such as in the so-called ‘Tetraphyllidea relicts’, whose members represent at least 9 independent lineages that may be established as new orders (see above; Fig. 3). The prevailing practice of deriving taxonomic conclusions and proposals for new classification, even at the ordinal level, from single-gene phylogenies (especially short sequences of the 28S rRNA gene) is somewhat problematic because phylogenies of cestodes based on a single marker, including mitochondrial genes, may not capture their evolutionary history (Trevisan et al., Reference Trevisan, Jacob Machado, Lahr and Marques2021).
A serious problem is also the limited suitability of some of the molecular markers widely used so far, especially ITS, since its paralogues have been detected in some species of the Caryophyllidea, Bothriocephalidea and Diphyllobothriidea (Bouzid et al., Reference Bouzid, Štefka, Hypša, Lek, Scholz, Legal, Hassine and Loot2008; Králová-Hromadová et al., Reference Králová-Hromadová, Štefka, Špakulová, Orosová, Bombarová, Hanzelová, Bazsalovicsová and Scholz2010, Reference Králová-Hromadová, Baszalovicsová, Oros and Scholz2012; Bazsalovicsová et al., Reference Bazsalovicsová, Králová-Hromadová, Štefka, Scholz, Hanzelová, Vávrová, Szemek and Kirk2011; Brabec et al., Reference Brabec, Kuchta, Scholz and Littlewood2016). The situation is particularly unfavourable with respect to the use of common molecular markers in caryophyllideans, one of the most basal groups of all cestodes. Brabec et al. (Reference Brabec, Scholz, Králová-Hromadová, Baszalovicsová and Olson2012) evaluated the utility of 2 nuclear and 2 mitochondrial molecular markers (ssrDNA and lsrDNA, nad3 and cox1) for estimating caryophyllidean interrelationships. They found that these markers did not contain sufficient phylogenetic signal. Moreover, Brabec et al. (Reference Brabec, Scholz, Králová-Hromadová, Baszalovicsová and Olson2012) detected multiple trnK + nad3 + trnS + trnW + cox1 haplotypes within individuals, suggesting gene exchange between the mitochondrial and nuclear genomes. The presence of such nuclear paralogues (i.e. numts) makes the search for informative markers to estimate their evolution unusually problematic, especially compared to other major tapeworm lineages (Brabec et al., Reference Brabec, Scholz, Králová-Hromadová, Baszalovicsová and Olson2012).
Multigene phylogenies are largely absent in fish tapeworms, with exception of phylogenetic studies on individual orders (see Waeschenbach and Littlewood, Reference Waeschenbach, Littlewood, Caira and Jensen2017), which were based on sequences of 2 nuclear and 2 mitochondrial gene sequences. Phylogenomic approaches have not been applied at all, even to the most ‘problematic’ groups with poor resolve position within the cestode tree, such as the Gyrocotylidea, Amphilinidea, Onchoproteocephalidea and ‘Tetraphyllidea-like’ lineages. Trevisan et al. (Reference Trevisan, Jacob Machado, Lahr and Marques2021) call for a comprehensive evidence framework to be conducted in tapeworm phylogeny studies and consider the characterization of the new mitochondrial genomes a good step to provide a valuable resource for future studies on the evolutionary relationships of tapeworms. Another problem with some groups, particularly Trypanorhyncha, is the insufficient representation of the sequenced taxa to cover most of the diversity of a given group (Beveridge et al., Reference Beveridge, Haseli, Ivanov, Menoret, Schaeffner, Caira and Jensen2017).
More molecular data are of course also needed for a better understanding of the actual host associations of fish tapeworms and their geographical distribution, including deciphering the most plausible scenario of colonization of individual fish groups by tapeworms and colonization of individual zoogeographical regions. Last but not least, molecular tools should be increasingly used in ecologically oriented research of fish tapeworms and in studies of their life cycles. The eDNA metabarcoding of ontogenetic stages of fish tapeworms also represents a promising tool that should be increasingly used in ecological and epidemiological studies and in screening the occurrence and species diversity of tapeworms in aquatic ecosystems. It is evident that quite good progress has been made in cestodology, but much remains to be unravelled and many interesting evolutionary and ecological questions to be answered.
Acknowledgements
The authors are indebted to Janine N. Caira, University of Connecticut in Storrs, and Kirsten Jensen, University of Kansas, Lawrence, both USA, for inviting them to work together in their NSF-funded projects on tapeworms, and to Blanka Škoríková and Jan Brabec from the Institute of Parasitology, BC, CAS for help with generating of data. Two anonymous reviewers provided helpful suggestions.
Author contributions
T. S. conceived and designed the study. T. S. and R. K. conducted data gathering and wrote the article.
Financial support
This study was partly supported by the Czech Science Foundation (project no. 19-28399X) and the Institute of Parasitology (RVO 60077344).
Conflict of interest
The authors declare there are no conflicts of interest.