1. Introduction
The Rhodope Massif is a major tectonic zone of the Alpine orogen in the northern Aegean region of the eastern Mediterranean (Fig. 1a). A thick marble-dominated succession is interpreted as representing a carbonate platform involved in the lower Rhodope thrust system of Bulgaria and Greece (Burg et al. Reference Burg, Ricou, Ivanov, Godfriaux, Dimov and Klain1996; Fig. 1b). It is known as the Pangeon (Pangaion, Boz Dağ) unit (Jordan, Reference Jordan1969; Kronberg, Reference Kronberg1969; Papanikolaou & Panagopoulos, Reference Papanikolaou and Panagopoulos1981; Papanikolaou, Reference Papanikolaou, Robertson and Dixon1984; Dimadis & Zachos, Reference Dimadis and Zachos1989) or Pirin–Pangaion zone, which includes the Bulgarian Pirin unit and the Greek Pangaion and Thasos Island units (Zagorčev, Reference Zagorčev1994). This regional Pirin–Pangeon–Thasos (PPT) composite unit is considered as a separate crustal terrane in the tectonic framework of the Hellenides (Burg, Reference Burg2012; Papanikolaou, Reference Papanikolaou2013). It is of critical importance for understanding the organization of the terranes involved in the Mesozoic tectonic evolution of the region.

Fig. 1. (a) Tectonic framework of the Alpine orogen in the northern Aegean region of the eastern Mediterranean. Abbreviations: SMM, Serbo-Macedonian Massif; Sf, Sofia; At, Athens; Is, Istanbul. (b) Synthetic map of the Rhodope and Serbo-Macedonian massifs in southern Bulgaria and northern Greece (compiled after Ricou et al. Reference Ricou, Burg, Godfriaux and Ivanov1998; Bonev et al. Reference Bonev, Ovtcharova-Schaltegger, Moritz, Marchev and Ulianov2013). U–Pb zircon geochronology: 1, Himmerkus et al. (Reference Himmerkus, Zachariadis, Reischmann and Kostopoulos2012); 2, Himmerkus et al. (Reference Himmerkus, Reischmann and Kostopoulos2009a); 3, Himmerkus et al. (Reference Himmerkus, Reischmann and Kostopoulos2009b); 4, Bonev et al. (Reference Bonev, Moritz, Borisova and Filipov2019c); 5, Turpaud & Reischmann (Reference Turpaud and Reischmann2010); 6, Macheva et al. (Reference Macheva, Peytcheva, Von Quadt, Zidarov and Tarassova2006); 7, Peytcheva et al. (Reference Peytcheva, Von Quadt, Titorenkova, Zidarov and Tarassova2005); 8, Christofides et al. (Reference Christofides, Koroneos, Liati and Kral2007); 9, Peytcheva et al. (Reference Peytcheva, Von Quadt, Tarassov, Zidarov, Tarassova and Andreichev2009a); 10, Peytcheva et al. (Reference Peytcheva, Von Quadt, Sarov, Voinova and Kolcheva2009b); 11, Kounov et al. (Reference Kounov, Graf, Von Quadt, Bernoulli, Burg, Seward, Ivanov and Fanning2012); 12, Peytcheva et al. (Reference Peytcheva, Von Quadt, Ovtcharova, Handler, Neubauer, Salnikova, Kostitsin, Sarov and Kolcheva2004); 13, Liati et al. (Reference Liati, Gebauer, Fanning, Dobrzhinetskaya, Faryad, Wallis and Cuthbert2011); 14, Arkadakskiy et al. (Reference Arkadakskiy, Bohm, Heaman, Cherneva, Stancheva and Ovtcharova2003).
There are two contrasting Late Permian palaeogeographic interpretations for the PPT unit. One interpretation links it as the Drama terrane to the Pelagonia microcontinent derived from the northern passive margin of Gondwana (Ricou, Reference Ricou1994; Fig. 2a). A second interpretation considers it as a Rhodope unit next to Pelagonia along the Eurasian active margin and associated north-directed subduction of the Palaeotethys (Ziegler & Stampfli, Reference Ziegler, Stampfli and Cassins2001; Fig. 2b). Both interpretations commonly account for the Neotethys rifting episode along Gondwana’s northern margin following the Late Palaeozoic Variscan orogeny in Europe. The age of the PPT carbonate platform is interpreted as Silurian to Carboniferous based on a single coral find (reefal Rugosa determined by R.Wolfart in Jordan, Reference Jordan1969), or Ordovician to Carboniferous as inferrred from brachiopod (Atrypida?) remains (Ancirev et al. Reference Ancirev, Gorozanin, Velichkov and Bogoyavlenskaya1980). Therefore, constraining the protracted Palaeozoic depositional age of the PPT carbonate platform is critical for a better understanding of the Rhodope palaeogeography. Furthermore, this carbonate platform, which records the geodynamic evolution of the Tethyan margins in the Aegean region, allows constraining the Gondwana–Eurasia crustal organization and interaction at the Palaeozoic–Mesozoic transition.

Fig. 2. Gondwana–Eurasia palaeotectonic reconstructions for the Late Permian: (a) according to Ricou (Reference Ricou1994) (DR, Drama; Moe, Moesia; PL, Pelagonian), (b) according to Ziegler & Stampfli (Reference Ziegler, Stampfli and Cassins2001) (PL, Pelagonian; Rh, Rhodope).
In this paper, we combine U–Pb laser ablation inductively coupled plasma mass spectrometry (LA-ICP-MS) dating of detrital zircons from schist layers interstratified with marble and Sr isotopic compositions of marble from the Pirin unit in Bulgaria. The aim is to constrain the deposition of the regional PPT carbonate platform, having in mind its critical position and its role during the Mesozoic evolution of the Alpine orogen in the Aegean region.
2. Geological background
The Pangeon unit belongs to the metamorphic basement of the Rhodope Massif (Fig. 1b). It consists of a lower gneiss sequence underlying a marble–gneiss sequence, which is overlain by a thick marble sequence, and an upper sequence of interlayered two-mica schist, calc-schist and marble (Dimadis & Zachos, Reference Dimadis and Zachos1989). The thickness of the marble sequence in the Greek Rhodope varies between 700 and 2800 m and generally becomes thinner to the east (e.g. Dimadis & Kosmas, Reference Dimadis and Kosmas1989). In Bulgaria, the Pirin unit exposes the Pangeon-type marble sequence, which is c. 1000 m thick in the north, and c. 1600 m thick in the south (Zagorčev, Reference Zagorčev1994). On Thasos Island, the Pangeon-type metamorphic section contains two schist sequences alternating with two marble sequences, which altogether reach a thickness of c. 2000 m (Dimitriadis, Reference Dimitriadis1989).
To the northeast, along the late Alpine SW-directed Nestos syn-metamorphic ductile thrust, the Pirin–Pangeon unit is overlain by the intermediate unit of the Rhodope thrust stack called the Sideronero unit (Papanikolaou & Panagopoulos, Reference Papanikolaou and Panagopoulos1981) or Mesta–Sideronero unit (Burg et al. Reference Burg, Ricou, Ivanov, Godfriaux, Dimov and Klain1996; Fig. 1). Multiple-phased isoclinal folding of the Pirin–Pangeon metamorphic section is associated with inverted metamorphic zones ranging from upper greenschist facies to the south, to sillimanite-bearing amphibolite facies to the north, against the Nestos thrust (Kronberg & Raith, Reference Kronberg and Raith1977; Zachos & Dimadis, Reference Zachos and Dimadis1983; Mposkos & Liati, Reference Mposkos and Liati1993). Similar sillimanite-bearing schists have been described in Thasos Island (Dimitriadis, Reference Dimitriadis1989). The thickness of 7000 m initially proposed by Kronberg (Reference Kronberg1969) for the Pangeon unit marble was subsequently questioned, and attributed to an overestimated section due to folding and thrusting (Papanikolaou & Panagopoulos, Reference Papanikolaou and Panagopoulos1981; Zachos & Dimadis, Reference Zachos and Dimadis1983; Dimadis & Kosmas, Reference Dimadis and Kosmas1989). To the SW, the Pirin–Pangeon unit is bounded by the Strymon thrust (Kockel & Walther, Reference Kockel and Walther1965) reworked by the Cenozoic Strymon extensional detachment (Dinter, Reference Dinter1998) from the Kerdilion unit of the Serbo-Macedonian Massif (Kockel et al. Reference Kockel, Mollat and Walther1977). The Strymon detachment was recently put under question (Brun & Sokoutis, Reference Brun and Sokoutis2018). The Kerdilion unit is considered as a gneissic complex similar to the Rhodope Massif and representing its integral crustal component (Burg et al. Reference Burg, Godfriaux and Ricou1995; Kydonakis et al. Reference Kydonakis, Moulas, Chatzitheodoridis, Brun and Kostopoulos2015).
U–Pb zircon geochronological studies of the gneissic units of the Serbo-Macedonian Massif have identified Late Neoproterozoic meta-granitoids (Himmerkus et al. Reference Himmerkus, Reischmann, Kostopoulos, Robertson and Mountrakis2006). They are coeval with the meta-granitoids of the Carpathian–Balkan units located to the north (Kounov et al. Reference Kounov, Graf, Von Quadt, Bernoulli, Burg, Seward, Ivanov and Fanning2012). They probably also have their age counterparts in the Rhodope (Arkadakskiy et al. Reference Arkadakskiy, Bohm, Heaman, Cherneva, Stancheva and Ovtcharova2003; Fig. 1). Ordovician–Silurian meta-granitoids (Macheva et al. Reference Macheva, Peytcheva, Von Quadt, Zidarov and Tarassova2006; Himmerkus et al. Reference Himmerkus, Reischmann and Kostopoulos2009a; Kounov et al. Reference Kounov, Graf, Von Quadt, Bernoulli, Burg, Seward, Ivanov and Fanning2012; Bonev et al. Reference Bonev, Ovtcharova-Schaltegger, Moritz, Marchev and Ulianov2013) and voluminous Late Carboniferous – Permian meta-granitoids (Peytcheva & von Quadt, Reference Peytcheva and Von Quadt1995; Peytcheva et al. Reference Peytcheva, Von Quadt, Ovtcharova, Handler, Neubauer, Salnikova, Kostitsin, Sarov and Kolcheva2004; Cornelius, Reference Cornelius2008); Turpaud & Reischmann, Reference Turpaud and Reischmann2010; Himmerkus et al. Reference Himmerkus, Zachariadis, Reischmann and Kostopoulos2012) are common in the Serbo-Macedonian and Rhodope massifs. They provide the age of the igneous components involved in the continental build-up of both massifs (Fig. 1b). Early–Middle Triassic meta-granitoids and gabbroids (Christofides et al. Reference Christofides, Koroneos, Liati and Kral2007; Himmerkus et al. Reference Himmerkus, Reischmann and Kostopoulos2009b; Peytcheva et al. Reference Peytcheva, Von Quadt, Tarassov, Zidarov, Tarassova and Andreichev2009a; Drakoulis et al. Reference Drakoulis, Koroneos, Poli, Soldatos, Papadopoulou, Murata and Eliwa2013; Bonev et al. Reference Bonev, Moritz, Borisova and Filipov2019c) in the Serbo-Macedonian and Rhodope massifs record Triassic rifting during opening of the Neotethys (Fig. 1b). Jurassic – Early Cretaceous meta-granitoids and granitoids in the Serbo-Macedonian and Rhodope massifs (c. 163–134 Ma) are attributed to an arc/back-arc system (not shown in Fig. 1), which subsequently developed in the Neotethys during its Mesozoic evolution (Turpaud & Reischmann, Reference Turpaud and Reischmann2010; Himmerkus et al. Reference Himmerkus, Zachariadis, Reischmann and Kostopoulos2012; Bonev et al. Reference Bonev, Marchev, Moritz and Collings2015a, b).
Further geological components adjacent to the Pirin–Pangeon unit are Late Cretaceous to Oligocene granitoids (e.g. Marchev et al. Reference Marchev, Georgiev, Raicheva, Peytcheva, Von Quadt, Ovtcharova and Bonev2013, and references therein) and Eocene to Quaternary sedimentary rocks (e.g. Zagorchev, Reference Zagorchev1998, and references therein), which represent a syn- and post-tectonic Cenozoic cover and magmatic units related to the extensional evolution of the Rhodope Massif in Bulgaria and Greece (e.g. Burg et al. Reference Burg, Ricou, Ivanov, Godfriaux, Dimov and Klain1996; Bonev et al. Reference Bonev, Burg and Ivanov2006; Bonev & Beccaletto, Reference Bonev, Beccaletto, Taymaz, Yilmaz and Dilek2007; Burchfiel et al. Reference Burchfiel, Nakov, Dumurdzanov, Papanikolaou, Tzankov, Serafimovski, King and Nurce2008; Brun & Sokoutis, Reference Brun and Sokoutis2018 and references therein).
3. Field data, samples and their composition
Thin schist layers are interstratified with marble of the Pirin unit near the villages of Ilindentsi and Petrovo (Fig. 3; Supplementary Table S1 available online at https://doi.org/10.1017/S0016756819001183). Their structures were characterized during mapping at a scale of 1:1000 conducted for marble quary exploration (Dabovski et al. Reference Dabovski, Karagjuleva, Kostadinov, Stojanov, Haidutov and Tsankov1980).
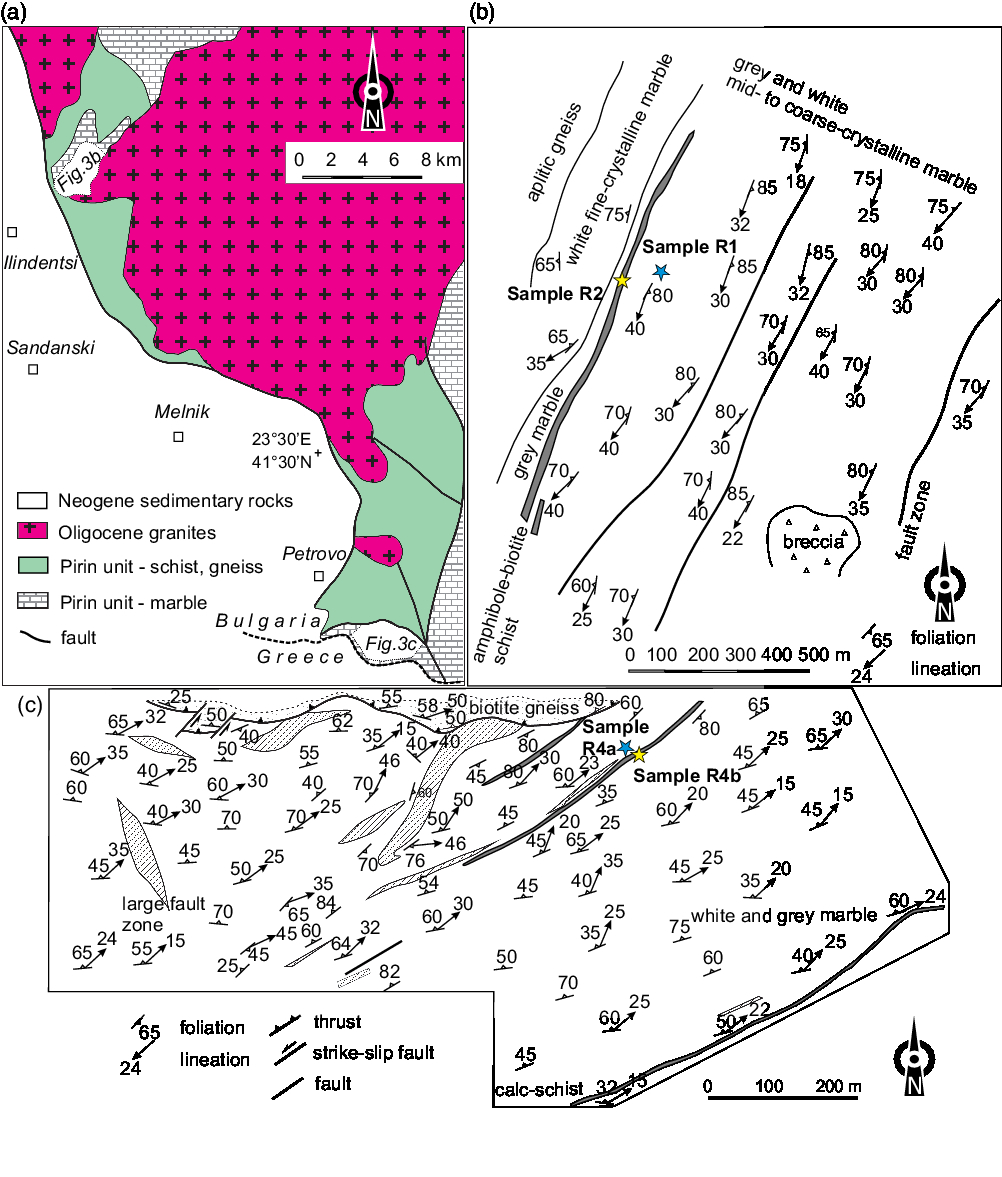
Fig. 3. Simplified geological maps for (a) Pirin unit (location in Fig. 1b), (b) Ilindentsi locality and (c) Petrovo locality (locations in (a)). Modified after Dabovski et al. (Reference Dabovski, Karagjuleva, Kostadinov, Stojanov, Haidutov and Tsankov1980).
At Ilindentsi, a 30 m thick, weathered biotite schist layer is overlain by grey massive marble and underlain by impure white mica-rich marble that passes down-section into a white crystalline marble. In thin-section, the biotite schist consists of modally decreasing quartz, biotite, plagioclase and garnet. Grain size of quartz and plagioclase is variable and is attributed to its detrital origin. Biotite is strongly chloritized, and epidote replaces the plagioclase. Both minerals indicate retrogression. Garnet occurs as small unaltered porphyroblasts. Zircon and apatite are accessory minerals. A layer of biotite schist (sample R2) was sampled for U–Pb dating of detrital zircons (see Fig. 4), and the underlying white fine-grained crystalline marble (sample R1) was collected to determine its Sr isotopic composition. The whole-rock chemical composition of sample R1 indicates that it is a dolomitic marble (Supplementary Table S1, available online at https://doi.org/10.1017/S0016756819001183).

Fig. 4. Selected cathodoluminescence (CL) images of dated zircons in the schist layers interstratified with marble of the Pirin unit, and diagrams of U–Pb LA-ICP-MS zircon analyses. Circles represent the location of spot analyses with corresponding ages given with 2σ error. (a) CL image of the youngest zircon in sample R2; (b) density distribution diagram of zircons from sample R2; (c) concordia diagram for the two youngest zircons in sample R2; (d) CL image of the zircons in sample R4b; (e) density distribution diagram of the zircons in sample R4b; (f) concordia diagram for the four youngest zircons in sample R4b.
At Petrovo, a 10 m thick layer of Fe-rich calc-schist is underlain by a 10 m thick layer of biotite schist. Both lithologies are interbedded within marble. The schist succession is overlain by white marble, which contains fine-grained shale laminae. A medium-grained white massive marble underlies the schist succession. In thin-section, the Fe-rich calc-schist consists of modally decreasing quartz, chlorite, hematite, calcite and feldspar. Quartz forms mono- and polycrystalline grains, while red-brown chlorite defines discontinuous aggregates and nests together with hematite. Rare magnetite and quartz–feldspar lithic fragments also occur. Accessory minerals include zircon, apatite and rare white mica flakes. The foliation of calc-schist is parallel to the primary lamination of the host marble. We sampled Fe-rich calc-schist (sample R4b) for U–Pb geochronology of detrital zircons, and the white overlying marble containing fine shale laminae (sample R4a) for Sr isotope geochemistry. The whole-rock chemical composition classifies sample R4b as calcareous Fe-rich shale (e.g. Herron, Reference Herron1988), while sample R4a is a calcitic marble (Supplementary Table S1, available online at https://doi.org/10.1017/S0016756819001183). In addition, sample R6, consisting of gray calcitic marble, was used for Sr isotope geochemistry (Fig. 1b for location). The studied marble samples are characterized by low contents of trace and rare-earth elements (Supplementary Table S1, available online at https://doi.org/10.1017/S0016756819001183).
4. Results
4.a. U–Pb zircon geochronology
In order to date the marble deposition in the Pirin unit, we have analysed detrital zircons of the two schist samples R2 and R4b for U–Pb geochronology (see Fig. 4). The locations of samples are given in Figures 1 and 3 and Supplementary Table S1 (available online at https://doi.org/10.1017/S0016756819001183). The sample preparation and analytical procedures are the same as described by Bonev et al. (Reference Bonev, Filipov, Raicheva and Moritz2019 a). The analytical data are presented in Supplementary Table S2 (available online at https://doi.org/10.1017/S0016756819001183). U–Pb in situ LA-ICP-MS zircon dating was performed at the laboratory of the Geological Institute of the Bulgarian Academy of Sciences using a New Wave UP193FX LA coupled to a Perkin Elmer ELAN DRC-e quadrupole ICP-MS.
The dated zircons in sample R2 vary in size from 50 µm to 300 µm, with an average aspect ratio of 1.86. They display irregular or semi-rounded shapes and rarely preserved oscillatory zoning patterns disturbed by resorption and corrosion, which are characteristic for a magmatic origin (Fig. 4a). Thirty-six concordant zircon analyses out of 92 performed in total yielded Neoproterozoic to Permian ages (Fig. 4b). Four-grains concordant ages range from 607.7 Ma to 563.5 Ma, corresponding to the Neoproterozoic (Supplementary Figure S1, available online at https://doi.org/10.1017/S0016756819001183). The main age cluster of 25 zircons between 545.6 and 491.2 Ma encompasses the Cambrian, with a peak around 520 Ma. A further minor age cluster includes five Ordovician zircons dated between 468.8 Ma and 429 Ma. One zircon yielded a Carboniferous age of 372.1 ± 7.2 Ma. The youngest zircon yielded an age of 266.4 ± 4.8 Ma, and hence defines the maximum depositional age in the latest Middle Permian (Fig. 4c). The Th/U ratios of the dated zircons vary from 0.01 to 0.79, with the majority of zircon grains having a high ratio of 0.12–0.79 (Supplementary Table S2, available online at https://doi.org/10.1017/S0016756819001183), which is characteristic for magmatic zircons (Rubatto, Reference Rubatto2002; Tiepel et al. Reference Tiepel, Eichhorn, Loth, Rohrmülle, Höll and Kennedy2004).
Zircons from the Fe-rich calc-schist sample R4b show semi-rounded to rounded shapes, as well as prismatic and pyramidal crystals varying in size from 100 µm to 400 µm (average aspect ratio 1.4), which have a homogeneous and a strongly obliterated magmatic oscillatory zoning pattern (Fig. 4d). The 206Pb/238U ages obtained from 105 analyses range from 359.5 Ma to 290.2 Ma. Sixty-two concordant zircon grain analyses yielded Carboniferous–Permian ages from 334.5 Ma to 296.9 Ma. Zircon ages span across the Carboniferous, with concordant clusters at 333.9 ± 1.5 Ma, 323.98 ± 0.92 Ma, 317.54 ± 0.68 Ma, 311.6 ± 1.1 Ma and 305.4 ± 1.1 Ma (Supplementary Figure S2, available online at https://doi.org/10.1017/S0016756819001183). The youngest zircon out of 105 grains provided an age of 290.2 ± 3.7 Ma, and hence defines a maximum Early Permian depositional age. This age is consistent with four more grains, which define a concordia age of 299.5 ± 1.3 Ma. The Th/U ratios of the zircons in this sample range from 0.05 to 1.29 (Supplementary Table S2, available online at https://doi.org/10.1017/S0016756819001183), but the majority of these ratios are in the 0.36–1.29 range, which is typical for magmatic zircons.
4.b. Sr isotopes
For strontium chemical separation, whole rock samples were prepared in the clean laboratory at the University of Geneva, where Sr isotopes were also measured on a Thermo Scientific TRITON Thermal Ionization Mass Spectrometer. 87Sr/86Sr ratios were corrected for internal fractionation using a 88Sr/86Sr ratio of 8.357209 and for external fractionation using a nominal value of SRM 987, with a 87Sr/86Sr ratio of 0.710248 (McArthur et al. Reference McArthur, Howarth and Bailey2001). Analytical details and procedures can be found in Chiaradia et al. (Reference Chiaradia, Müntener and Beate2011). The whole-rock Sr isotopic compositions are given in Supplementary Table S3 (available online at https://doi.org/10.1017/S0016756819001183).
Strontium isotopic ratios of the three marble samples (R1, R4a and R6) range from 0.707420 to 0.707653 (see Supplementary Table S3, available online at https://doi.org/10.1017/S0016756819001183). These values are close to the previously published Sr isotopic compositions of the Pirin unit marble, with a 87Sr/86Sr ratio between 0.70766 and 0.70776 (Georgiev et al. Reference Georgiev, Peytcheva, Von Quadt, Marchev, Grozdev and Balkanska2012). In Figure 5, Sr isotopic compositions of the Pirin unit marble samples are plotted against the Sr isotopic compositions of Phanerozoic sea-water (McArthur et al. Reference McArthur, Howarth and Bailey2001). The range of the Sr isotopic compositions of the marble under study are consistent with a Middle Permian depositional age (Fig. 5).

Fig. 5. Diagram of Sr isotopic compositions of the Pirin unit marble samples vs age-constrained Sr isotopic compositions of Phanerozoic sea-water (after McArthur et al. Reference McArthur, Howarth and Bailey2001).
5. Discussion
The U–Pb detrital zircon geochronological data obtained for the Pirin unit indicate a latest Middle Permian maximum depositional age as young as 262.4 Ma (within error) for the biotite schist layer within marble. The Fe-rich calc-schist layer within the marble has a Lower Permian maximum depositional age of 287.5 Ma (within error) (Fig. 4). The number of dated zircons from the R2 biotite schist nearly meets the required amount for statistical criteria, which is met for the Fe-rich calc-schist (Vermeesch, Reference Vermeesch2004). Taken collectively, the number of dated zircons from both schist samples satisfies and exceeds the statistical confidence at the 95 % level. Thus, the Lower–Middle Permian detrital zircon record obtained in this study provides an unequivocal depositional age constraint for the Pirin–Pangeon marble hosting the schist layers.
The predominantly high Th/U ratios of the detrital zircons reflect mostly a magmatic provenance and a subsidiary provenance from metamorphic basement rocks. In this sense, the Th/U ratios and the documented age clusters of detrital zircons correspond to the same age as the magmatic protoliths of the metamorphic basement of the Rhodope and Serbo-Macedonian massifs (Fig. 1b). The detrital zircons age clusters of the studied samples differ spatially in function of the immediate sedimentary source area, but the clusters precisely reflect the age of the basement rocks of each source area location. In particular, biotite schist sample R2 reveals a major cluster of Neoproterozoic–Cambrian zircons, which corresponds to the age of the abundant Neoproterozoic–Cambrian orthogneisses in the nearby Carpatho-Balkan units (Kounov et al. Reference Kounov, Graf, Von Quadt, Bernoulli, Burg, Seward, Ivanov and Fanning2012). The same applies to the minor cluster of Ordovician zircons, with ages overlapping with those of the exposed Ordovician meta-magmatic rocks in the Rhodope and Serbo-Macedonian units (Macheva et al. Reference Macheva, Peytcheva, Von Quadt, Zidarov and Tarassova2006; Peytcheva et al. Reference Peytcheva, Von Quadt, Sarov, Voinova and Kolcheva2009b; Kounov et al. Reference Kounov, Graf, Von Quadt, Bernoulli, Burg, Seward, Ivanov and Fanning2012; see Fig. 1) adjacent to sample R2, suggesting that the biotite schist contains sedimentary material coming from a nearby source area. The Fe-rich calc-schist sample R4b contains a major detrital component from Carboniferous–Permian magmatic rocks, which are exposed at the base of the Pirin–Pangeon–Thasos carbonate unit (Turpaud & Reischmann, Reference Turpaud and Reischmann2010; see Fig. 1). As the major detrital zircon age cluster of the Fe-rich calc-schist overlaps with the ages of the underlying Carboniferous–Permian meta-magmatic rocks, it suggests that the source area was not far from the depositional area.
Collectively, the detrital zircon record in both schist samples is evidence for a provenance of the sedimentary material from the continental Rhodope–Serbo-Macedonian high-grade basement. The studied schist samples are devoid of any zircon input from Triassic magmatic bodies (e.g. Bonev et al. Reference Bonev, Moritz, Borisova and Filipov2019c, and references therein). This supports a pre-Triassic deposition of the PPT carbonate platform. Our interpretation is consistent with the Sr isotopic compositions of the Pirin unit marble, pointing to carbonate platform sedimentation during the Middle Permian (Figs 4, 5).
There is evidence of Permian carbonate sedimentation (de Bono et al. Reference DeBono, Martini, Zaninetti, Hirsch and Stampfli2001; Ferriere et al. Reference Ferriere, Baumgartner and Chanier2016 and this study) on top of the Rhodope–Serbo-Macedonian and the Pelagonian Proterozoic and Palaeozoic igneous and metamorphic basements (e.g. Anders et al. Reference Anders, Reischmann, Kostopoulos and Poller2006, Reference Anders, Reischmann and Kostopoulos2007; Himmerkus et al. Reference Himmerkus, Reischmann, Kostopoulos, Robertson and Mountrakis2006, Reference Himmerkus, Reischmann and Kostopoulos2009a; Schenker et al. Reference Schenker, Burg, Kostopoulos, Moulas, Larionov and Von Quadt2014). Furthermore, Permian limestone clasts and blocks were documented in the Rhodope uppermost metamorphic unit (Bonev, Reference Bonev2005), and Permian sedimentation was also established in the Sakar–Strandzha Zone (Bonev et al. Reference Bonev, Filipov, Raicheva and Moritz2019b) c. 60 km to the NE of the Rhodope (see Fig. 1a). The Rhodope–Serbo-Macedonian and the Pelagonian massifs are interpreted as a part of the Eurasian continental margin, which is supported by biostratigraphic and geochronological evidence for the Permian. However, the Rhodope–Serbo-Macedonian massifs and the Pelagonian Massif were separated from each other during Triassic rifting in the Neotethys in the Aegean region (e.g. Ferriere et al. Reference Ferriere, Baumgartner and Chanier2016 and references therein). Thus, from a palaeogeographic viewpoint, there is more evidence for the deposition of the PPT carbonate platform at the Eurasian margin than for the deposition along the northern passive margin of Gondwana.
6. Conclusions
Our study shows that the Pirin–Pangeon–Thasos carbonate platform includes schist layers in which detrital zircon ages constrain sedimentation during the Permian. Because the sedimentation source areas were the Rhodope igneous and metamorphic basement and adjacent basement units, this implies platform deposition along the Rhodope continental margin of Eurasia, but an affinity with the northern passive Gondwana margin cannot be ruled out.
Supplementary material
To view supplementary material for this article, please visit https://doi.org/10.1017/S0016756819001183
Acknowledgements
The study was supported by the Bulgarian National Science Fund project no. DN 04/6. We thank Jean-Pierre Burg and Dimitrios Papanikolaou for their helpful comments on the manuscript.