Introduction
The Plotopteridae were penguin-like, flightless seabirds that inhabited the North Pacific Basin in the late Eocene and Oligocene and possibly survived into the middle Miocene. The fossil record of these birds has significantly increased in the past years and now includes four genera (Copepteryx, Hokkaidornis, Empeirodytes, and Stenornis) with five named species from Japan and six genera (Phocavis, Tonsala, Olympidytes, Klallamornis, Plotopterum, and Stemec) with eight named species from North America (Howard, Reference Howard1969; Olson, Reference Olson1980; Goedert, Reference Goedert1988; Olson and Hasegawa, Reference Olson and Hasegawa1996; Sakurai et al., Reference Sakurai, Kimura and Katoh2008; Kaiser et al., Reference Kaiser, Watanabe and Johns2015; Mayr and Goedert, Reference Mayr and Goedert2016; Ohashi and Hasegawa, Reference Ohashi and Hasegawa2020). Plotopterum and Olympidytes have also been tentatively recognized from rocks in Japan (Hasegawa et al., Reference Hasegawa, Okumura and Okazaki1977; Olson and Hasegawa, Reference Olson and Hasegawa1985; Mori and Miyata, Reference Mori and Miyata2021). The osteology and taxonomic diversity, stratigraphic occurrence, and interrelationships of plotopterids remain, however, insufficiently known mainly because many taxa are represented by only a few bones.
Plotopterids were initially considered to be most closely related to the suliform Phalacrocoracidae (cormorants) and Anhingidae (anhingas; Howard, Reference Howard1969; Olson, Reference Olson1980; Olson and Hasegawa, Reference Olson and Hasegawa1996). By contrast, Mayr (Reference Mayr2005) hypothesized that these birds are the sister taxon of the Sphenisciformes (penguins), but subsequent analyses recovered plotopterids as the sister taxon of either the Phalacrocoracidae and Anhingidae (Smith, Reference Smith2010) or a more inclusive clade including the Sulidae (gannets and boobies), Phalacrocoracidae, and Anhingidae (Mayr et al., Reference Mayr, Goedert and Vogel2015; see Mayr et al., Reference Mayr, Goedert, De Pietri and Scofield2021 for a more detailed discussion of the affinities of plotopterids).
In North America, plotopterid fossils were found in strata dating to the earliest Miocene in California (USA) and late Oligocene in British Columbia (Canada), which yielded the holotypes of Plotopterum joaquinensis Howard, Reference Howard1969 and Stemec suntokum Kaiser, Watanabe, and Johns, Reference Kaiser, Watanabe and Johns2015, respectively (Howard, Reference Howard1969; Kaiser et al., Reference Kaiser, Watanabe and Johns2015). The type locality for P. joaquinensis is in the Pyramid Hill Sand Member of the Jewett Sand and is considered to be earliest Aquitanian or earliest Miocene in age (Shimada et al., Reference Shimada, Welton and Long2014; contra Kaiser et al., Reference Kaiser, Watanabe and Johns2015, who assumed a late Oligocene age). One of the geochronologically earliest North American plotopterids, Phocavis maritimus Goedert, Reference Goedert1988, is from the late Eocene Keasey Formation in Oregon (Goedert, Reference Goedert1988). The only bones known of P. joaquinensis and S. suntokum are coracoids, whereas P. maritimus is represented by a tarsometatarsus.
Most North American plotopterids, however, come from deposits on the Olympic Peninsula in Washington State, USA. Intense collecting efforts by one of the authors (J.L.G.) during the past four decades have yielded comprehensive fossil material from various sites of the late Eocene or early Oligocene Lincoln Creek and Makah formations and the Oligocene Pysht Formation (see Goedert and Cornish, Reference Goedert, Cornish, Zhou and Zhang2002 for a detailed review of the geology and stratigraphy of these formations).
Although one of the species from the Olympic Peninsula, Tonsala hildegardae Olson, Reference Olson1980, is among the first plotopterids to be named (Olson, Reference Olson1980), the diversity of plotopterids from the Lincoln Creek, Makah, and Pysht formations is still poorly understood. Goedert and Cornish (Reference Goedert, Cornish, Zhou and Zhang2002) reviewed the then-known fossil material and noted the presence of at least five different species. However, Dyke et al. (Reference Dyke, Wang and Habib2011) studied the same material and recognized only two species, T. hildegardae and one they described as Tonsala buchanani Dyke, Wang, and Habib, Reference Dyke, Wang and Habib2011. The study by Dyke et al. (Reference Dyke, Wang and Habib2011) suffered from various shortcomings that have confused and impeded a well-resolved taxonomy of plotopterids from western Washington. Not only did Dyke et al. (Reference Dyke, Wang and Habib2011) choose one of the least substantial specimens available to them as the holotype of T. buchanani, but their diagnosis of the species did not include any characters preserved in the holotype, the dimensions of some of the fossils were incorrectly given, and most of the referred specimens clearly belong to species other than T. buchanani. As already detailed by Mayr et al. (Reference Mayr, Goedert and Vogel2015), some of the skeletal elements were misidentified or attributed to the wrong specimen number. Other errors, such as measurements attributed to the wrong specimen in table 1 of Dyke et al. (Reference Dyke, Wang and Habib2011) were repeated later (Mori and Miyata, Reference Mori and Miyata2021, p. 152, table 1). One of the aims of this paper is to clarify this confusion by formally revising the status of T. buchanani and various specimens assigned to that species by Dyke et al. (Reference Dyke, Wang and Habib2011).
Table 1. Overview of the stratigraphic provenance of the published plotopterid material from western Washington State (Olson, Reference Olson1980; Goedert and Cornish, Reference Goedert, Cornish, Zhou and Zhang2002; Dyke et al., Reference Dyke, Wang and Habib2011; Mayr et al., Reference Mayr, Goedert and Vogel2015; Mayr and Goedert, Reference Mayr and Goedert2016, Reference Mayr and Goedert2018; this study).

Increased preparation efforts of undescribed material yielded new insights into the diversity of plotopterids from southwest Washington State and the Olympic Peninsula and led to the description of three further species (Mayr et al., Reference Mayr, Goedert and Vogel2015; Mayr and Goedert, Reference Mayr and Goedert2016, Reference Mayr and Goedert2018). One of these, Olympidytes thieli Mayr and Goedert, Reference Mayr and Goedert2016, is a medium-sized species with stouter limb bones than Tonsala hildegardae and is so far the only named plotopterid species from the Lincoln Creek Formation. Two larger species were assigned to the taxon Klallamornis. The smaller of these, K. abyssa Mayr and Goedert, Reference Mayr and Goedert2016, is represented by partial skeletons and isolated bones of a few individuals. The larger species was tentatively referred to Klallamornis and described as ?K. clarki Mayr and Goedert, Reference Mayr and Goedert2016.
Herein, we report new specimens that inform the osteology and stratigraphic occurrence of some poorly known species. In addition to revising material assigned to Tonsala buchanani, we furthermore address the diversity of plotopterids from Washington State and present a new hypothesis concerning the evolutionary origin of these birds.
Materials and methods
Although plotopterid fossils are not uncommon in some localities on the Olympic Peninsula, they are typically preserved in very hard concretions, the preparation of which is difficult and very time consuming. In some cases, after excess rock has been mechanically removed, acid etching yields good results, but this depends on the chemistry of the concretion. Some matrices (and these can be from the same locality and rock unit) respond to dilute acetic acid; others respond only to formic acid. Other concretions are insoluble, and mechanical preparation is the only way to expose the bones, in which case extreme care must be taken.
Repositories and institutional abbreviations
The fossils are deposited in the Natural History Museum of Los Angeles County, Los Angeles, CA, USA (LACM), the Senckenberg Research Institute, Frankfurt, Germany (SMF), the National Museum of Natural History, Smithsonian Institution, Washington, D.C., USA (USNM), and the Burke Museum of Natural History and Culture, University of Washington, Seattle, WA, USA (UWBM).
Systematic paleontology
Aves Linnaeus, Reference Linnaeus1758
Plotopteridae Howard, Reference Howard1969
Tonsalinae Mayr and Goedert, Reference Mayr and Goedert2018
Tonsala Olson, Reference Olson1980
Type species
Tonsala hildegardae Olson, Reference Olson1980, by original designation.
cf. Tonsala hildegardae Olson, Reference Olson1980
Figure 1.1–1.3
Holotype
USNM 256518: partial associated skeleton. Beach west of Murdock Creek, Clallam County, Washington; Oligocene, lower part of the Pysht Formation.
Description and comparisons
SMF Av 662 is the most complete scapula of a North American plotopterid described so far. The bone is, however, split in a transverse plane so that only internal structures are exposed. The tip of the acromion still appears to be hidden in the matrix. In its size and shape, SMF Av 662 corresponds well with the scapula of the T. hildegardae holotype (Fig. 1.4) and shows that the irregular margins of the scapular blade of the latter are due to postmortem damage, as already assumed in the original description (Olson, Reference Olson1980).

Figure 1. (1–3) cf. Tonsala hildegardae (SMF Av 662) left scapula compared with (4) a cast of the right scapula of the holotype (LACM 123791) and (5) the left scapula of gen. indet. sp. indet. A (UWBM 86871); in (3), the surrounding matrix was digitally removed. acr = acromion. Scale bars = 20 mm.
Material
SMF Av 662: left scapula, which is still preserved in a block of matrix (Fig. 1.1–1.3); from the Jansen Creek Member of the Makah Formation; collected by J.L.G. on 11 June 1999.
Measurement
Length as preserved, 123.8 mm.
Remarks
The tentative assignment of this fossil to T. hildegardae is based mainly on its small size. The specimen comes from the Makah Formation and is therefore somewhat older than the holotype of T. hildegardae from the Pysht Formation. The only other record of T. hildegardae from the Makah Formation is a coracoid, which was tentatively referred to the species by Mayr and Goedert (Reference Mayr and Goedert2016).
Klallamornis Mayr and Goedert, Reference Mayr and Goedert2016
Type species
Klallamornis abyssa Mayr and Goedert, Reference Mayr and Goedert2016, by original designation.
Klallamornis buchanani (Dyke, Wang, and Habib, Reference Dyke, Wang and Habib2011), n. comb.
Figures 2.1, 2.2, 2.8–2.12, 2.17, 2.18, 4.9, 4.13
- Reference Dyke, Wang and Habib2011
Tonsala buchanani Dyke, Wang, and Habib, p. 2, fig. 2A–V.
- Reference Mayr, Goedert and Vogel2015
Tonsala buchanani; Mayr, Goedert, and Vogel, restricted to holotype UWBM 86875 only.
- Reference Mayr and Goedert2016
“Tonsala” buchanani; Mayr and Goedert, fig. 1D.
- Reference Mayr and Goedert2018
“Tonsala” buchanani; Mayr and Goedert, p. 52, fig. 2D.

Figure 2. (1, 2) Left femur of the holotype of Klallamornis buchanani (UWBM 86875) in caudal view; in (2), the bone was coated with ammonium chloride and mirrored; the missing portion of the condylus medialis is indicated by the gray area. (3–5) Right femur of the holotype of Klallamornis abyssa in caudal view (SMF Av 610); in (4), the surrounding matrix was digitally removed, and the missing proximal end of the bone is indicated by the gray area; in (5), the bone was coated with ammonium chloride. (6, 7) Left femora (cranial view) of the extant Phalacrocorax carbo (Phalacrocoracidae) to illustrate the pronounced sexual size dimorphism in the length of the bone: (6) a female individual; (7) a male individual. (8, 9) Fragment of the proximal end of a right femur referred to K. buchanani (SMF Av 663): (8) caudal view; (9) medial view. (10, 11) Thoracic vertebra associated with SMF Av 663: (10) dorsal view; (11) cranial view. (12) Partial ungual phalanx associated with SMF Av 663 in lateral and plantar views; the arrow denotes an enlarged view of the bone. (13–16) Ungual phalanges of (13) Fregata magnificens (Fregatidae), (14) Anhinga anhinga (Anhingidae), (15) Morus bassanus (Sulidae), and (16) Phalacrocorax carbo (Phalacrocoracidae). (17, 18) Three thoracic vertebrae associated with the holotype of Klallamornis buchanani (UWBM 86875): (17) right lateral view; (18) cranial view. cdl = condylus lateralis; cdm = condylus medialis; fac = facies articularis acetabularis; fat = facies articularis antitrochanterica; flx = tuberculum flexorium; fvc = fovea costalis; psp = processus spinosus; ptv = processus transversus; pvt = processus ventralis; zcr = zygapophysis cranialis. Scale bars = 20 mm (the same scale applies for [13–16]).
Holotype
Partial skeleton: UWBM 98975; both femora, proximal end of a tibiotarsus, three vertebrae, ribs, and other bone fragments. Beach west of Murdock Creek, Clallam County, Washington; Oligocene, lower part of the Pysht Formation.
Description and comparisons
The fragmentary proximal end of the femur of the newly referred specimen SMF Av 663 consists of the caudal half of the proximal end of the bone (Fig. 2.8, 2.9). The fossil closely corresponds to the femur of the holotype of Klallamornis buchanani in size and morphology. It is smaller than the femur of the K. abyssa holotype (the width of which is estimated at 34–35 mm) and larger than that of the Olympidytes thieli holotype (which measures 28.6 mm). A referral to K. buchanani is also supported by the fact that the fossil is from the type locality and horizon of the species. As in K. buchanani, Tonsala hildegardae, and O. thieli, the distal margins of the facies articularis antitrochanterica and the facies articularis acetabularis form a distinct rim, whereas the distal portion of the facies articularis acetabularis smoothly merges into the femur shaft in Hokkaidornis abashiriensis Sakurai, Kimura, and Katoh, Reference Sakurai, Kimura and Katoh2008 and the species of Copepteryx (see also Mori and Miyata, Reference Mori and Miyata2021). As in other plotopterids, the fovea ligamenti capitis is very pronounced.
SMF Av 663 also includes an ungual phalanx, which lacks a part of its dorsal section and the proximal tip (Fig. 2.12). Ungual phalanges of plotopterids have not been described previously. The bone has an ovate cross section, lacks a laterally open sulcus neurovascularis, and exhibits numerous neurovascular openings on its surface. It is markedly curved and very robust, and in its overall shape is more like the ungual phalanges of the Fregatidae (Fig. 2.13) and Anhingidae (Fig. 2.14) than those of the Sulidae (Fig. 2.15) and Phalacrocoracidae (Fig. 2.16). However, unlike in the Fregatidae and Anhingidae but as in the Phalacrocoracidae and Sulidae, the tuberculum flexorium is only weakly prominent and forms a lip-like transverse ridge rather than a true tubercle.
Material
SMF Av 663: fragment of proximal end of right femur, fragmentary thoracic vertebra, and ungual phalanx (Fig. 2.8–2.12); from the lower part of the Pysht Formation west of Murdock Creek, regarded as late early Oligocene or early late Oligocene (Barnes and Goedert, Reference Barnes and Goedert2001), or late but not latest Oligocene (Nesbitt et al., Reference Nesbitt, Martin, Carroll and Grieff2010); collected by J.L.G. on 26 June 1983.
Measurement
SMF Av 663: proximal width of femur, 32.0 mm.
Remarks
The holotype of Tonsala buchanani (UWBM 86875) is a partial skeleton consisting of both femora, the proximal end of a tibiotarsus (incorrectly labeled as UWBM 86871 by Dyke et al., Reference Dyke, Wang and Habib2011, fig. 2T), three vertebra, ribs, and other bone fragments. Contrary to the statement of Dyke et al. (Reference Dyke, Wang and Habib2011, p. 2), the specimen does not include the proximal end of a tarsometatarsus or a fibula; Dyke et al. (Reference Dyke, Wang and Habib2011, fig. 2R) furthermore illustrated the right femur of T. buchanani without the associated head.
Tonsala buchanani was not properly diagnosed because all the characters listed by Dyke et al. (Reference Dyke, Wang and Habib2011) pertain to the humerus, coracoid, and carpometacarpus and can therefore not be assessed in the holotype, which does not include these bones. Although the “Whiskey Creek specimen” (UWBM 86869) was only tentatively referred to T. buchanani by Dyke et al. (Reference Dyke, Wang and Habib2011, p. 6), the associated carpometacarpus was considered to have characters diagnostic for T. buchanani. The alleged differences of this carpometacarpus and that of T. hildegardae furthermore appear to be based on comparisons with the os carpi ulnare of the latter species (Mayr and Goedert, Reference Mayr and Goedert2016). The humerus and coracoid referred to and used to diagnose T. buchanani belong to a specimen (UWBM 86871; Fig. 3) that is discussed in the following and likely represents a different species. Whether the tarsometatarsus UWBM 86870, which was referred to T. buchanani by Dyke et al. (Reference Dyke, Wang and Habib2011), belongs to this species cannot be determined for sure; Goedert and Cornish (Reference Goedert, Cornish, Zhou and Zhang2002) thought it was referrable to the species represented by UWBM 86871 because of its size and because it was found in the same rock unit. All of this leaves only the femora (Fig. 2.1, 2.2), proximal tibiotarsus, and thoracic vertebrae (Fig. 2.17, 2.18) of the holotype as unambiguously identified material of T. buchanani.

Figure 3. (1–9) Major bones of the plotopterid specimen UWBM 86871 from the Makah Formation (gen. indet. sp. indet. A), which was previously assigned to, and in part used to diagnose (incorrectly), Tonsala buchanani by Dyke et al. (Reference Dyke, Wang and Habib2011). (1, 2) Left coracoid: (1) dorsal view; (2) ventral view. (3) Cranial portion of the left scapula in lateral view. (4–6) Left humerus: (4) cranial view; (5) caudal view; (6) caudoventral view. (7) Proximal and distal portions of left ulna. (8, 9) Left femur: (8) caudal view; (9) cranial view. (10) Right humerus, which is here assigned to the same indeterminate species (SMF Av 650). acr = acromion; fac = facies articularis clavicularis; ppc = processus procoracoideus; scp = portion of scapular blade; tbd = tuberculum dorsale. Scale bars = 20 mm.
Dyke et al. (Reference Dyke, Wang and Habib2011, table 1) gave the distal width of the holotype femur of T. buchanani as 25.2 mm, but this value is too small and may pertain to the width of the bone as it is preserved. The actual distal width of the complete bone is here estimated at approximately 30.5 mm. With a length of 134.2 mm (Dyke et al., Reference Dyke, Wang and Habib2011, table 1), the femur of T. buchanani is shorter than that of Klallamornis abyssa (Fig. 2.3–2.5), which measures about 143 mm and has a distal width of about 34 mm (Mayr and Goedert, Reference Mayr and Goedert2016). Although the holotype of K. abyssa is therefore distinctly larger than that of T. buchanani, the magnitude of this size difference falls within the intraspecific sexual size dimorphism of extant Phalacrocoracidae, in which the males are significantly larger than the females, with their limb bones measuring up to 120% the length of those of females (Fig. 2.6, 2.7).
The holotypes of both T. buchanani and K. abyssa are from the lower part of the Pysht Formation near Murdock Creek, and at present it is not possible to reliably differentiate both species on the basis of morphological features other than size. The femur of T. buchanani appears to have a straighter shaft than that of Klallamornis abyssa, and the caudal portion of the condylus lateralis seems to have a wider medial rim. However, these differences are not reliably diagnostic, and the possibility remains that Klallamornis abyssa is a junior synonym of T. buchanani. Currently, however, we refrain from a formal synonymization and note that the preparation of two partial skeletons of similar-sized species from the Olympic Peninsula is pending, which may inform the osteology of these species. In any case, the femur of T. hildegardae is not as stout as that of T. buchanani, and we here transfer the latter species to the genus Klallamornis.
Gen. indet. sp. indet. A (cf. Klallamornis or Olympidytes)
Figures 1.5, 3.1–3.9, 4.1, 4.3, 4.4, 4.7, 4.8, 4.12, 5.8
- Reference Goedert, Cornish, Zhou and Zhang2002
Plotopteridae, new species?; Goedert and Cornish, p. 67, figs. 3b, 4b, 5d.
- Reference Dyke, Wang and Habib2011
Tonsala buchanani (in part); Dyke, Wang, and Habib, p. 2, figs. 2G–J, 2M, 2P–Q, 2U–V.
- Reference Mayr and Goedert2018
“Tonsala” buchanani; Mayr and Goedert, fig. 2D.
- Reference Mayr, Goedert, De Pietri and Scofield2021
Plotopteridae, unidentified species; Mayr et al., p. 2, fig. 3a.
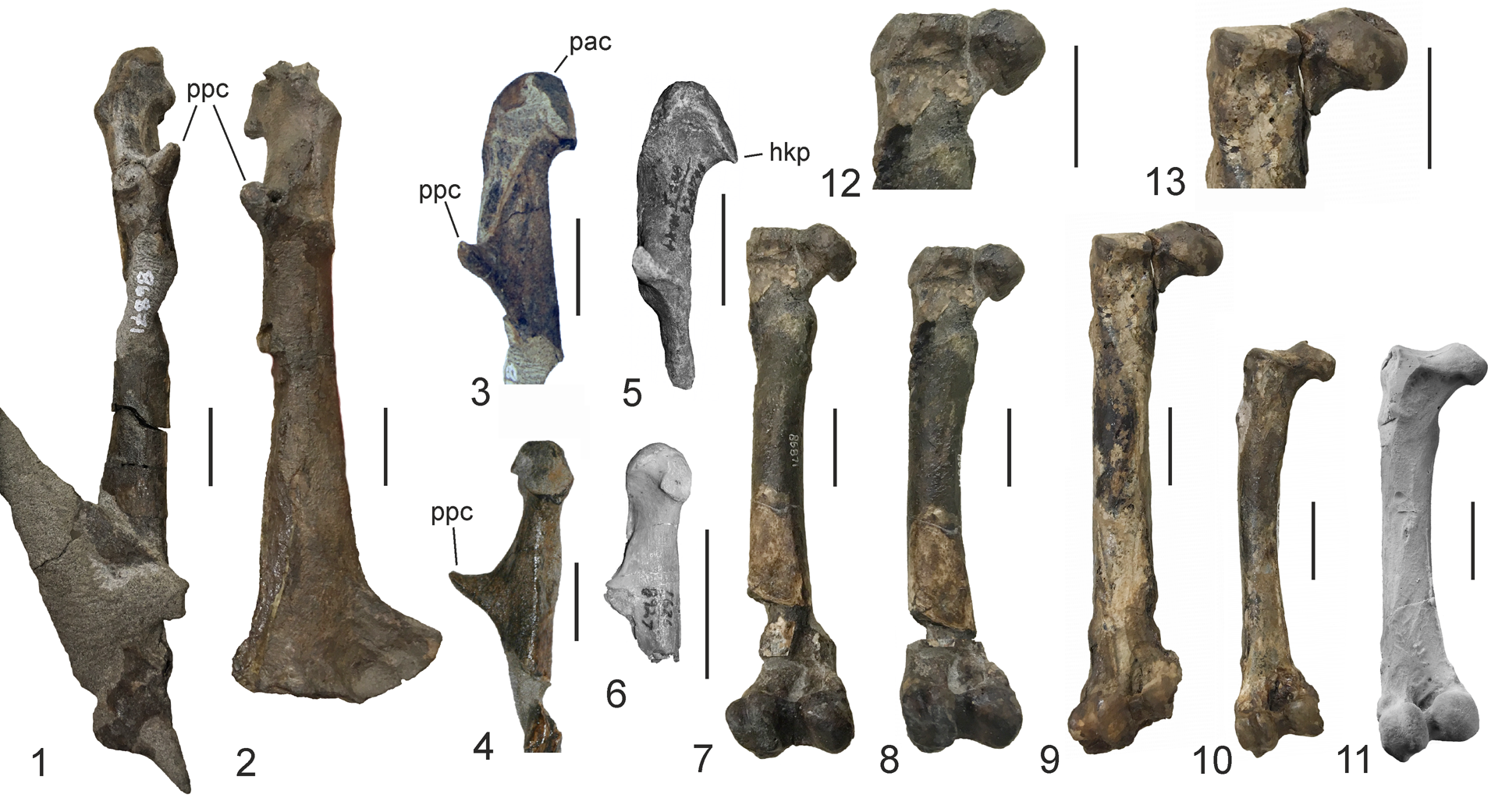
Figure 4. Selected bones of specimen UWBM 86871 (gen. idet. sp. indet. A) compared with other plotopterids. (1) Left coracoid of UWBM 86871 in dorsal view. (2) Right coracoid (UWBM 108400; dorsal view), which was assigned to Klallamornis abyssa by Mayr and Goedert (Reference Mayr and Goedert2016). (3, 4) Omal extremity of the left coracoid of UWBM 86871: (3) dorsomedial view; (4) medial view. (5) Omal extremity of the coracoid of Tonsala hildegardae in dorsomedial view (cast of the holotype, LACM 123791). (6) Omal extremity of the coracoid of Plotopterum joaquinensis, earliest Miocene, California, in medial view (holotype, LACM 8927). (7, 8) Left femur of UWBM 86871; in (8) the bone was digitally brought to its presumed original length. (9) Left femur of Klallamornis buchanani (holotype, UWBM 86875). (10) Left femur of Tonsala hildegardae (UWBM 86873). (11) Left femur of Olympidytes thieli (cast of holotype, SMF Av 608; coated with ammonium chloride). (12, 13) Details of the proximal ends (caudal view) of the femora of (12) UWBM 86871 and (13) K. buchanani (holotype, UWBM 86875). hkp = hook-like projection formed by processus acrocoracoideus; pac = processus acrocoracoideus; ppc = processus procoracoideus. Scale bars = 20 mm.
Description and comparisons
UWBM 86871 is the only plotopterid in which the humerus, coracoid, and femur of a single individual are preserved in their entire length. As such, the specimen is of significance for an assessment of the limb proportions of plotopterids, even though all three bones are broken in two or more parts that were shifted apart owing to tectonic movements during the fossilization process.
UWBM 86871 includes the cranial extremity of the left scapula, which has been freed of matrix, with a portion of the scapular blade still being appressed to the sternal end of the coracoid. The scapula was mentioned by Goedert and Cornish (Reference Goedert, Cornish, Zhou and Zhang2002) but not by Dyke et al. (Reference Dyke, Wang and Habib2011), who stated that the bone is known only from the T. hildegardae holotype. As in other plotopterids, the scapula of UWBM 86871 has a very long acromion, which forms a sharper angle with the cranial margin of the bone than in T. hildegardae, in which the base of the acromion smoothly merges into the cranial margin of the scapula (Fig. 1.4, 1.5). The acromion of UWBM 86871 is less ventrally deflected than in a plotopterid from Japan (Ando and Fukata, Reference Ando and Fukata2018). The bone appears to be slightly larger than the scapula we refer to T. hildegardae in the present study (SMF Av 662; see the preceding notes).
Dyke et al. (Reference Dyke, Wang and Habib2011, p. 2) stated that the coracoid of UWBM 86871 consists of the “cranial [sic] end […] broken at the level of the sternocoracoid impression,” but the bone is completely preserved. The UWBM 86871 coracoid (Fig. 4.1) is distinctly smaller than the coracoid that was referred to Klallamornis abyssa (Fig. 4.2) by Mayr and Goedert (Reference Mayr and Goedert2016). The fossil differs from the coracoid of the Tonsala hildegardae holotype in that the facies articularis clavicularis does not form a pronounced hook. It is distinguished from the coracoid SMF Av 614, which was referred to Tonsala sp. by Mayr and Goedert (Reference Mayr and Goedert2016), in that the processus procoracoideus more strongly points in the omal direction. The sternal extremity of UWBM 86871 is too poorly preserved for close comparison with other plotopterids. Apart from being smaller, the coracoid of UWBM 86871 resembles that of the recently described Stenornis kanmonensis Ohashi and Hasegawa, Reference Ohashi and Hasegawa2020 from the early Oligocene of Japan (Ohashi and Hasegawa, Reference Ohashi and Hasegawa2020). However, since the coracoids of other similar-sized taxa, such as Olympidytes thieli, are unknown, it is not possible to assess the phylogenetic significance of this resemblance. Ohashi and Hasegawa (Reference Ohashi and Hasegawa2020, p. 288) did not list measurements for the length of the Stenornis kanmonensis coracoid but noted that “the size is almost the same as Copepteryx hexeris,” for which the length of the coracoid was given as 180 mm by Olson and Hasegawa (Reference Olson and Hasegawa1996). With an estimated length of ~150 mm, the coracoid of UWBM 86871 is distinctly shorter.
The humerus of UWBM 86871 and the referred humerus SMF Av 650 are the only completely preserved humeri of plotopterids from the Olympic Peninsula discovered so far. The shaft of the bone shows a marked sigmoid curvature, which is characteristic of the plotopterid humerus. It is more slender than the humerus shaft of Copepteryx hexeris Olson and Hasegawa, Reference Olson and Hasegawa1996, the only other plotopterid species for which a complete humerus is known. The shaft is not as strongly bulging dorsally as it is in the T. hildegardae holotype, a condition that was considered of pathologic origin by Olson (Reference Olson1980). The distal end of the bone resembles the humerus of T. hildegardae in the dorsoventrally narrow process flexorius. As detailed by Goedert and Cornish (Reference Goedert, Cornish, Zhou and Zhang2002), however, the trochlear ridges are better developed than in the T. hildegardae holotype.
The ulna (Fig. 3.7) is broken in two portions and lacks the intermediate section. The proximal end of this bone was previously illustrated by Dyke et al. (Reference Dyke, Wang and Habib2011, fig. 2M) but misidentified as the proximal radius. As far as comparisons are possible, the preserved portions of the bone resemble the ulna of T. hildegardae (Olson, Reference Olson1980; Mayr et al., Reference Mayr, Goedert and Vogel2015).
Dyke et al. (Reference Dyke, Wang and Habib2011, p. 2) stated that the femur of UWBM 86871 was “glued together in the UWBM collection back-to-front.” However, and as noted by Mayr and Goedert (Reference Mayr and Goedert2016), the bone has merely been freed from surrounding rock and is in the same state it was in the original matrix.
Material
UWBM 86871: partial skeleton (Fig. 3.1–3.9), including the left coracoid, left scapula, left humerus (erroneously stated to be from the right side by Dyke et al., Reference Dyke, Wang and Habib2011, p. 2 but correctly labeled as a left humerus by Dyke et al., Reference Dyke, Wang and Habib2011, fig. 2I–J; Dyke et al., Reference Dyke, Wang and Habib2011 also listed the distal end of a left humerus, but UWBM 86871 includes only the left humerus, which is complete), partial left ulna (erroneously identified as the radius by Dyke et al., Reference Dyke, Wang and Habib2011), and left femur; from the Jansen Creek Member of the Makah Formation, southeast of Bullman Creek. SMF Av 650: right humerus (Fig. 3.10); from the Jansen Creek Member of the Makah Formation, approximately 200 meters west of the mouth of Rasmussen Creek; collected by JLG on 27 December 1986. The Jansen Creek Member is an olistostrome and latest Eocene to earliest Oligocene in age.
Measurements
UWBM 86871: humerus, length as preserved, 143.8 mm (Dyke et al., Reference Dyke, Wang and Habib2011, table 1), distal depth, 16.1 mm; coracoid, length as preserved, ~150 mm; femur, length as preserved, 133.0 mm (Dyke et al., Reference Dyke, Wang and Habib2011, table 1 erroneously listed the femur measurements for UWBM 86870, which is an isolated tarsometatarsus). SMF Av 650: humerus, maximum length along longitudinal axis, 136.0 mm; width of shaft in midsection, 20.0 mm; maximum proximal width, 33.0 mm; maximum distal width, 28.6 mm.
Remarks
UWBM 86871 was referred to Klallamornis buchanani by Dyke et al. (Reference Dyke, Wang and Habib2011) and is much more complete than the holotype of this species. As preserved, the length of the femur of UWBM 86871 is subequal to that of the K. buchanani holotype (135.1 versus 133.0 mm; Fig. 4.7, 4.9), but the bone is broken in the distal section of the shaft, and the two portions are shifted apart by some distance. It was, therefore, originally shorter than the femur of the Klallamornis buchanani holotype (Fig. 4.8), from which it also differs in having a narrower proximal end (28.3 versus 33 mm; Fig. 4.12, 4.13). The condylus lateralis of UWBM 86871 is furthermore narrower than that of the K. buchanani holotype.
Apart from being larger, UWBM 86871 differs from Tonsala hildegardae in the shape of the processus acrocoracoideus of the coracoid, which has a bulkier tip and forms a less-pronounced hook (Fig. 4.3, 4.5); the acromion of the scapula forms a sharper angle with the cranial margin of the bone (Fig. 1.4, 1.5); the shaft of the femur is stouter than in T. hildegardae.
UWBM 86871 is distinctly smaller than specimens assigned to Klallamornis abyssa, and the femur is longer than that of the Olympidytes thieli holotype (Fig. 4.11). A well-founded assignment to Klallamornis or Olympidytes, or a delimitation from either taxon is, however, not possible currently.
?Klallamornis clarki Mayr and Goedert, Reference Mayr and Goedert2016
Figures 5.1–5.6, 5.9, 5.10, 6.1, 6.2, 6.8–6.11
- Reference Goedert, Cornish, Zhou and Zhang2002
New genus and species?; Goedert and Cornish, p. 67, fig. 3c.
- Reference Mayr2009
“unnamed species”; Mayr, p. 68, fig. 7.4a.
- Reference Mayr and Goedert2016
?Klallamornis clarki Mayr and Goedert, p. 9, figs. 7A–F, 8A–E.
- Reference Mayr and Goedert2018
?Klallamornis clarki; Mayr and Goedert, p. 54, fig. 2E.

Figure 5. New material referred to ?Klallamornis clarki. (1–5) Proximal ends of the left (1–3) and right (4, 5) humerus (SMF Av 664; ?K. clarki): (1, 4) cranial views; (2, 5) caudal views; (3) ventral view. (6) Shaft fragment of a right humerus (SMF Av 665; ?K. clarki). (7) Distal portion of left humerus (caudal view) of Hokkaidornis abashiriensis, late Oligocene, Japan (SMF, cast of holotype). (8) Left humerus (caudal view) of UWBM 86871 (gen. indet. sp. indet. A). (9, 10) Fragmentary thoracic vertebra associated with specimen SMF Av 664 (?K. clarki): (9) caudal view; (10) left lateral view. fac = facies articularis caudalis; fvt = foramen vertebrale; ldg = cranially protruding ledge formed by distal margin of sulcus transversus; lip = lip-like process formed by ventral rim of facies articularis caudalis; mld = tubercle for attachment of musculus latissimus dorsi, pars cranialis; stv = sulcus transversus; tbd = tuberculum dorsale; zcd = articular surface of zygapophysis caudalis. Scale bars = 20 mm.

Figure 6. Comparison of selected elements of ?Klallamornis clarki and Hokkaidornis abashiriensis. (1, 2) Partial pelvis of ?Klallamornis clarki (SMF Av 612) from the Jansen Creek Member of the Makah Formation in right and left lateral views; the fossa that is situated caudal of the foramen acetabuli distinguishes the specimen from the pelvis of Hokkaidornis abashiriensis. (3) Pelvis of H. abashiriensis in right lateral view (SMF, cast of holotype); the frame indicates the position of the portion preserved in SMF Av 612. (4–7) Right tarsometatarsus of H. abashiriensis (SMF, cast of holotype): (4) plantar view; (5) dorsal view; (6) distal view; (7) proximal view. (8–11) Left tarsometatarsus (holotype, LACM 129405) of ?K. clarki from the Jansen Creek Member of the Makah Formation: (8) plantar view; (9) dorsal view; (10) distal view; (11) proximal view. (12, 13) Partial right tarsometatarsus from the Jansen Creek Member of the Makah Formation (SMF Av 609), which was tentatively referred to Olympidytes thieli by Mayr and Goedert (Reference Mayr and Goedert2016): (12) plantar view; (13) dorsal view; coated with ammonium chloride. In (4, 6, 8, 10, 12), the tarsometatarsal trochleae are numbered. cht = crista hypotarsi; fdl = sulcus for tendon of musculus flexor digitorum longus; fos = fossa caudal of foramen acetabuli; inc = incisura intertrochlearis lateralis; sul = sulcus between trochlea metatarsi III and IV. Scale bars = 20 mm.
Holotype
LACM 129405: tarsometatarsus; Jansen Creek Member of the Makah Formation, Clallam County, Washington; latest Eocene or earliest Oligocene.
Description and comparisons
Apart from being much larger, the humerus shows a close similarity to the proximal humerus of Tonsala hildegardae (Olson, Reference Olson1980; Goedert and Cornish, Reference Goedert, Cornish, Zhou and Zhang2002) and to that of UWBM 86871. It also resembles the proximal humerus of Copepteryx hexeris (only a small fragment of the proximal humerus of Hokkaidornis abashiriensis is known). The globose caput humeri protrudes slightly farther ventrally than the tuberculum ventrale (the latter is, however, damaged in both humeri of SMF Av 664). The tuberculum dorsale is proximodistally long, but its distal end does not reach farther distally than the crista bicipitalis. The distal margin of the sulcus transversus forms a cranially protruding ledge. On the caudal surface, distal of the fossa pneumotricipitalis, is a marked tubercle for the attachment of musculus latissimus dorsi, pars cranialis (Fig. 5.5).
The ventral rim of the facies articularis caudalis of the thoracic vertebra preserved in SMF Av 664 forms a distinct, caudally directed lip-like process (Fig. 5.10). We also refer to ?K. clarki a thoracic vertebra also from the lower part of the Lincoln Creek Formation along the middle fork of the Satsop River, which was figured by Goedert and Cornish (Reference Goedert, Cornish, Zhou and Zhang2002, fig. 5a, b).
Material
SMF Av 664: proximal ends of left and right humeri as well as a fragmentary thoracic vertebra (Fig. 5.1–5.5, 5.9, 5.10); float concretion from the lower part of the Lincoln Creek Formation (late Eocene or earliest Oligocene), gravel bar on west side of the middle fork of the Satsop River; collected by J.L.G. on 30 June 2017. SMF Av 665: shaft section of right humerus (Fig. 5.6); from the Jansen Creek Member of the Makah Formation, float from terrace approximately 1,100 m southeast of Bullman Creek; collected by J.L.G. on 11 May 2012. UWBM 86872: thoracic vertebra; from the Lincoln Creek Formation, collected by J.L.G.
Measurements
Humerus (SMF Av 664), maximum proximal width, 56.2 mm; estimated total length, ~210–220 mm.
Remarks
?Klallamornis clarki is the largest plotopterid species from the Olympic Peninsula and approaches the size of the very large Oligocene species Copepteryx hexeris and Hokkaidornis abashiriensis from Japan (Fig. 6). Its large size clearly distinguishes ?K. clarki from all other plotopterids from North America. Its genus-level assignment, however, is uncertain, because the known specimens have no skeletal elements in common with the fossils assigned to K. abyssa and K. buchanani.
The previously known material of ?K. clarki consisted of only the holotype, a tarsometatarsus (LACM 129405; Fig. 6.8–6.11), and a partial pelvis (Fig. 6.1, 6.2), which were described by Mayr and Goedert (Reference Mayr and Goedert2016).
cf. ?Klallamornis clarki
(“Whiskey Creek specimen”)
Figures 7.1–7.5, 7.8, 7.9, 7.13, 7.15, 7.16
- Reference Goedert, Cornish, Zhou and Zhang2002
New genus and species(?), the Whiskey Creek specimen; Goedert and Cornish, p. 68, figs. 4c, 5c.
- Reference Mayr2009
“Whiskey Creek specimen”; Mayr, p. 68, fig. 7.4b.
- Reference Dyke, Wang and Habib2011
Tonsala buchanani (in part); Dyke, Wang, and Habib, p. 2, figs. 2A, B, F, K, L, N, O.
- Reference Mayr, Goedert, De Pietri and Scofield2021
cf. Klallamornis; Mayr et al., p. 2, figs. 1a, 1h, 3m.

Figure 7. The Whiskey Creek specimen UWBM 86869 (cf. ?Klallamornis clarki). (1, 2) Caudal portion of left mandible of UWBM 86869: (1) dorsal view; (2) dorsomedial view. (3) Right pterygoid of UWBM 86869. (4) Right extremitas omalis of furcula of UWBM 86869. (5) Fragmentary portion of the omal extremity of the left coracoid of UWBM 86869 in dorsal view. (6) Omal extremity of left coracoid of UWBM 86871 (gen. indet. sp. indet. A) in dorsal view. (7) Omal extremity of a right coracoid referred to K. abyssa (UWBM 108400; dorsal view). (8, 9) Distal end of the left humerus of UWBM 86869: (8) cranial view; (9) caudal view. (10, 11) Distal end of the left humerus of Hokkaidornis abashiriensis (SMF cast of holotype): (10) cranial view; (11) caudal view. (12) Distal end of the left humerus of UWBM 86871 (gen. indet. sp. indet. A) in caudal view. (13, 14) Distal ends (distal view) of the left humeri: (13) UWBM 86869; (14) UWBM 86871 (gen. indet. sp. indet. A). (15, 16) Left carpometacarpus of UWBM 86869: (15) ventral view; (16) dorsal view. (17) Right carpometacarpus (ventral view) of H. abashiriensis (SMF cast of holotype). cdd = condylus dorsalis; cdv = condylus ventralis; csc = cotyla scapularis; dtr = dorsal trochlear ridge; faa = facies articularis acrocoracoidea; flx = processus flexorius; htp = sulcus humerotricipitalis; itr = intermediate trochlear ridge; oma = os metacarpale alulare; pac = processus acromialis; pis = processus pisiformis; pmd = processus medialis; ppc = processus procoracoideus; stp = sulcus scapulotricipitalis; vtr = ventral trochlear ridge. Scale bars = 20 mm.
Description and comparisons
Mandible (Fig. 7.1, 7.2) and pterygoid (Fig. 7.3) of UWBM 86869 were already described and compared with stem group Sphenisciformes and extant Suliformes by Mayr et al. (Reference Mayr, Goedert, De Pietri and Scofield2021). As detailed by Mayr et al. (Reference Mayr, Goedert and Vogel2015), an alleged pterygoid figured by Dyke et al. (Reference Dyke, Wang and Habib2011, fig. 2A) is a vertebra.
Of the coracoid, only the portion around the cotyla scapularis is visible, which is still unprepared and partly embedded in matrix (Fig. 7.5). The processus procoracoideus appears to be proportionally longer than in specimens referred to Klallamornis abyssa (Mayr and Goedert, Reference Mayr and Goedert2016).
Of the furcula, the right extremitas omalis is preserved and was listed by Goedert and Cornish (Reference Goedert, Cornish, Zhou and Zhang2002) but not mentioned by Dyke et al. (Reference Dyke, Wang and Habib2011). The bone exhibits a large articular facet for the coracoid and a long but narrow processus acromialis (Fig. 7.4).
UWBM 86869 includes the distal end of the left humerus, which closely resembles the distal humerus of Copepteryx hexeris in its morphology. Unlike in T. hildegardae and UWBM 86871, the ventrodistal portion of the bone is broadly rounded (Fig. 7.8, 7.9). As already noted by Goedert and Cornish (Reference Goedert, Cornish, Zhou and Zhang2002), the trochlear ridges are furthermore better developed than in Tonsala hildegardae. The dorsal trochlear ridge, which borders the sulcus humerotricipitalis, forms a ventrally directed projection. In distal view, however (Fig. 7.13, 7.14), the intermediate trochlear ridge of UWBM 86869 is less prominent than in UWBM 86871 and is directed toward the ventral trochlear ridge, rather than being positioned parallel to it as in UWBM 86871, C. hexeris, and H. abashiriensis.
Although Dyke et al. (Reference Dyke, Wang and Habib2011, p. 6) stated the carpometacarpus of UWBM 86869 was an incomplete proximal portion, the bone is nearly complete (Goedert and Cornish, Reference Goedert, Cornish, Zhou and Zhang2002, fig. 5c; Dyke et al., Reference Dyke, Wang and Habib2011, fig. 2N, O; herein Fig. 7.15, 7.16). The specimen closely resembles the carpometacarpi of Hokkaidornis abashiriensis (Fig. 7.17) and Copepteryx hexeris in its proportions. However, it exhibits a better-developed processus pisiformis than the carpometacarpus of H. abashiriensis, and the os metacarpale alulare is proportionally shorter than in H. abashiriensis and C. hexeris, with its proximal margin being more clearly offset from the carpal trochlea.
Material
UWBM 86869: caudal portion of left mandibular ramus, right pterygoid, right extremitas omalis of furcula, distal end of left humerus, left carpometacarpus (Fig. 7), and a vertebra; from late Eocene (35.3–34 Ma) strata of the Makah Formation west of Whiskey Creek (see Goedert and Cornish, Reference Goedert, Cornish, Zhou and Zhang2002).
Measurements
Carpometacarpus, length, 67.0 mm (Dyke et al., Reference Dyke, Wang and Habib2011, table 1).
Remarks
As detailed by Goedert and Cornish (Reference Goedert, Cornish, Zhou and Zhang2002), this specimen is from rocks mapped as the Makah Formation west of Whiskey Creek (mapped in error as the Pysht Formation by Nesbitt et al., Reference Nesbitt, Martin, Carroll and Grieff2010; E.A. Nesbitt, personal communication, 2011) and is possibly the oldest North American plotopterid; Phocavis maritimus is from strata nearly the same age. The Whiskey Creek specimen was tentatively referred to Klallamornis (“Tonsala”) buchanani by Dyke et al. (Reference Dyke, Wang and Habib2011, p. 6), but it clearly represents a species that had a much larger size than K. buchanani.
As detailed by Goedert and Cornish (Reference Goedert, Cornish, Zhou and Zhang2002), UWBM 86869 distinctly differs in the shape of the distal end of the humerus from UWBM 86871, another specimen originally referred to and used in part to diagnose Klallamornis (“Tonsala”) buchanani by Dyke et al. (Reference Dyke, Wang and Habib2011). Apart from differences in the size and orientation of the trochlear ridges (see Goedert and Cornish, Reference Goedert, Cornish, Zhou and Zhang2002: fig. 4 [the specimen in fig. 4e is the holotype of H. abashiriensis]), UWBM 86869 has a less-protruding and more-rounded processus flexorius than UWBM 86871 (Fig. 7.8, 7.9, 7.12). UWBM 86869 is also clearly distinguished from Tonsala hildegardae and Hokkaidornis abashiriensis in the morphology of the distal end of the humerus. The carpometacarpus of UWBM 86869 furthermore differs from that of Copepteryx and Hokkaidornis in the better-developed processus pisiformis (Fig. 7.15, 7.17).
Our tentative assignment of UWBM 86869 to ?K. clarki is based on the very large size of the fossil. There are, however, no skeletal elements in common between UWBM 86869 and definitive specimens of ?K. clarki. Compared with the holotype of Hokkaidornis abashiriensis, definitive ?K. clarki specimens are only slightly smaller, and UWBM 86869 is also slightly smaller than what would be expected for the sizes of the carpometacarpus and distal humerus of ?K. clarki. Whether this size difference is due to sexual dimorphism or the older geological age of the fossil, or whether it is indicative of species-level differences, cannot be said.
Discussion
So far, five species of plotopterids from western Washington State, including the Olympic Peninsula, have been formally named: Tonsala hildegardae Olson, Reference Olson1980, Olympidytes thieli Mayr and Goedert, Reference Mayr and Goedert2016, Klallamornis buchanani (Dyke, Wang, and Habib, Reference Dyke, Wang and Habib2011), K. abyssa Mayr and Goedert, Reference Mayr and Goedert2016, and ?K. clarki Mayr and Goedert, Reference Mayr and Goedert2016. The smallest and the largest of these—T. hildegardae and ?K. clarki—are readily separated by their sizes and morphological characters (Mayr and Goedert, Reference Mayr and Goedert2016, Reference Mayr and Goedert2018). These two species are also represented by multiple specimens, which include several diagnostic limb elements. Klallamornis buchanani and K. abyssa, however, are less well known and more difficult to distinguish, and as detailed in the preceding sections, there remains a possibility that these two species represent different size classes of a species that was sexually dimorphic in size (if so, the Japanese species Copepteryx hexeris and the larger C. titan Olson and Hasegawa, Reference Olson and Hasegawa1996 may likewise represent males and females of the same species; compare Fig. 2.6, 2.7 with Olson and Hasegawa, Reference Olson and Hasegawa1996, fig. 8).
The tarsometatarsus of Phocavis maritimus—which is the holotype and only known skeletal element of the species—differs from that of other plotopterids in plesiomorphic features (Goedert, Reference Goedert1988; Goedert and Cornish, Reference Goedert, Cornish, Zhou and Zhang2002; Mayr and Goedert, Reference Mayr and Goedert2016, Reference Mayr and Goedert2018), and all plotopterids except Phocavis, Plotopterum, and Stemec were united in the new taxon Tonsalinae by Mayr and Goedert (Reference Mayr and Goedert2018). In particular, the Phocavis tarsometatarsus has a fully enclosed foramen vasculare distale, and the hypotarsus exhibits a distinct sulcus for the tendon of musculus flexor hallucis longus (Mayr et al., Reference Mayr, Goedert, De Pietri and Scofield2021, fig. 4g). The reduction of the foramen vasculare distale characterizes the Tonsalinae (Mayr and Goedert, Reference Mayr and Goedert2018), whereas a sulcus for the tendon of musculus flexor hallucis longus is also present in an undescribed smaller tonsaline plotopterid from early Oligocene rocks in Japan (Mayr et al., Reference Mayr, Goedert, De Pietri and Scofield2021, fig. 4i). The tarsometatarsi of the early Miocene Plotopterum and the late Oligocene Stemec are unknown, but these two taxa exhibit a more plesiomorphic coracoid morphology than tonsaline plotopterids (Mayr and Goedert, Reference Mayr and Goedert2016, Reference Mayr and Goedert2018; also compare Fig. 4.4 and 4.6).
Whereas an early divergence of Phocavis is well supported, the interrelationships of tonsaline plotopterids are poorly resolved. Of particular interest are the affinities between the North American and Japanese taxa (i.e., the questions as to whether the very large-sized species ?Klallamornis clarki, Hokkaidornis abashiriensis, Copepteryx hexeris, and C. titan form a clade and how often giant size evolved in the Plotopteridae).
If the largest plotopterid from the Olympic Peninsula, ?Klallamornis clarki, indeed belongs to the taxon Klallamornis, very large size would have evolved independently in plotopterids from North America and Japan because K. buchanani and K. abyssa are both distinctly smaller than ?K. clarki and the giant species from Japan. Evolution of large body size in plotopterids may have been triggered by oceanic cooling. The tarsometatarsus of ?K. clarki agrees with smaller tarsometatarsi from the Olympic Peninsula, which were assigned to Olympidytes thieli (Mayr and Goedert, Reference Mayr and Goedert2016; Fig. 6.12, 6.13) and K. buchanani (UWBM 86870; Dyke et al., Reference Dyke, Wang and Habib2011), in that the crista hypotarsi is mediolaterally narrow, the incisura lateralis very wide, and the trochlea metatarsi IV shorter than in the equally large-sized taxa Copepteryx and Hokkaidornis from Japan (Fig. 6.4–6.11). The tarsometatarsus of ?K. clarki is furthermore distinguished from that of Copepteryx and Hokkaidornis in that there is no dorsally open sulcus between the trochleae metatarsorum III and IV. However, these differences between the tarsometatarsus of ?K. clarki and the large taxa from Japan may be due to the retention of plesiomorphic features in the North American plotopterids, and at present no unambiguously derived characters are known that support a clade including K. buchanani, K. abyssa, and ?K. clarki.
The “Whiskey Creek specimen” UWBM 86869 is distinguished from the smaller plotopterids from the Olympic Peninsula for which the humerus is known (T. hildegardae and UWBM 86871) in that the ventrodistal portion of the bone is broadly rounded. This morphology is shared with Copepteryx (the corresponding portion of the humerus of Hokkaidornis is damaged). If UWBM 86869 indeed belongs to ?Klallamornis clarki, this species is clearly different from smaller plotopterids from the Olympic Peninsula. The carpometacarpus of the Whiskey Creek specimen also exhibits a more plesiomorphic morphology than that of the giant taxa from Japan, Copepteryx and Hokkaidornis, in that the processus pisiformis is better developed and the os metacarpale alulare proportionally shorter, with its proximal margin being more clearly offset from the carpal trochlea (Fig. 7.15–7.17).
Irrespective of its exact affinities, the Whiskey Creek specimen, as possibly the oldest known plotopterid (Goedert and Cornish, Reference Goedert, Cornish, Zhou and Zhang2002), shows that very large size was achieved early in the evolution of the Plotopteridae, as it also was in stem group Sphenisciformes (Mayr et al., Reference Mayr, Scofield, De Pietri and Tennyson2017). Very large-sized plotopterids, that is, specimens of ?Klallamornis clarki, are as yet unknown from the later Oligocene rocks of the Pysht Formation (Table 1). The absence of this very large species in the geochronologically youngest plotopterid-bearing strata of the Olympic Peninsula may be of evolutionary significance, but well-based conclusions can be drawn only when more-robust data on the stratigraphic distribution of the plotopterids from the Olympic Peninsula are available.
The femora of Klallamornis and Olympidytes have a similar shape, whereas the shaft of the femur of Tonsala hildegardae is more slender. Although these differences in the proportions of the bone may be functionally related to the different sizes of the involved species, a slender femur and small size are likely to represent the plesiomorphic conditions within tonsaline plotopterids (Mayr and Goedert, Reference Mayr and Goedert2018). With Phocavis being the sister taxon of tonsaline plotopterids (to which all currently known species from Japan belong) and Tonsala being the sister taxon of other tonsaline plotopterids of which the femur is known, the interrelationships of plotopterids suggest a North American origin of the clade.
The high diversity of plotopterids in the late Eocene and Oligocene of the North Pacific Basin is notable, and their geographic restriction to the North Pacific calls for an explanation. The fossil record indicates that plotopterids underwent a rapid radiation in the late Eocene. This coincides with profound climatic, environmental, and ecological changes in marine ecosystems in the North Pacific Ocean. Paleogeographic reconstructions of what is today western Oregon and Washington show, due to subduction-related volcanism, the emergence of a succession of mostly small to moderately large volcanic islands far off the coast starting possibly in the late Paleocene and continuing throughout most of the Eocene (e.g., Snavely and Wagner, Reference Snavely and Wagner1963; Wells and Coe, Reference Wells and Coe1985; Snavely and Wells, Reference Snavely and Wells1996). During the Oligocene, most of this offshore volcanism had ceased; islands were eroding, and relatively rapid subduction formed broad offshore basins; and the oceans were cooling. By the early Miocene, the islands were gone, as were most of the plotopterids. Offshore islands and rocks, free of potential terrestrial mammalian predators that were diverse on the North American continent, would have been essential for the evolution and continued survival of flightless birds such as plotopterids (Warheit and Lindberg, Reference Warheit, Lindberg and Burger1988). The situation was different on the western side of the Pacific Ocean, with the islands of Japan being separated from Asia comparatively late in the Cenozoic (e.g., Maruyama et al., Reference Maruyama, Isozaki, Kimura and Terabayashi1997). Despite this, there were still places where plotopterids were able to breed and nest because plotopterids are known from at least the latest Eocene in Japan.
The origin of highly productive marine biotas in the North Pacific Ocean has been attributed to cooling events during the late Eocene and Oligocene (Vermeij, Reference Vermeij2018; Vermeij et al., Reference Vermeij, Banker, Capece, Hernandez, Salley, Vriesman and Wortham2019), but recent molecular analyses also date the radiation of kelp forests in the North Pacific to the Eocene/Oligocene boundary (Starko et al., Reference Starko, Gomez, Darby, Demes, Kawai, Yotsukura, Lindstrom, Keeling, Graham and Martone2019; Vermeij et al., Reference Vermeij, Banker, Capece, Hernandez, Salley, Vriesman and Wortham2019). Here we hypothesize that the evolution and diversification of plotopterids may have been causally linked to these events. According to Starko et al. (Reference Starko, Gomez, Darby, Demes, Kawai, Yotsukura, Lindstrom, Keeling, Graham and Martone2019), complex kelps originated in the northeast Pacific Ocean, where the earliest and phylogenetically most basal plotopterids evolved. However, whether the diversification of both kelp and plotopterids was related to an increased productivity in marine biotas of this area or whether either was triggered by the other in a coevolutionary process needs to be addressed in future studies. An understanding of plotopterid evolution would be fostered by an improved knowledge of the temporal sequence of the evolution of these birds and by a better-resolved phylogeny, which we aim to analyze in more detail once critical new fossil material has been prepared.
Acknowledgments
We thank Green Diamond Resource Company for allowing access to collecting localities on the Satsop River, M. Golliet for facilitating permits for access, and C. Sidor and K. Anderson (UWBM) for enabling the study of fossil specimens. O. Vogel is thanked for the time-consuming preparation of the new fossils. M. Kimura provided a cast of the Hokkaidornis abashiriensis holotype. The photos were taken by S. Tränkner and G.M. Comments from two anonymous reviewers and the associate editor D. Ksepka improved the manuscript.