Introduction
Necrotic enteritis (NE) is an important intestinal disease of chickens which was previously known to be caused by Clostridium perfringens (CP) type A and to a lesser extent by type C strains (Songer, Reference Songer1996). It is of note, however, that with identification of B-like necrotic enteritis toxin (NetB) as the major CP toxin, the NE-producing CP strains are currently classified as type G (Rood et al., Reference Rood, Adams, Lacey, Lyras, McClane, Melville, Moore, Popoff, Sarker and Songer2018). As an opportunistic pathogen, CP colonizes the intestinal tract of healthy chickens at a density of 102 colony forming units (CFU) per gram of intestinal content. The proliferation of CP in the gut is associated with production of various toxins, including α-toxin, NetB, TpeL, and perhaps other undefined toxins, which may in turn cause NE. There are several predisposing factors that enhance CP proliferation and toxin production, including unbalanced composition of the diet, immunosuppression, and intestinal damage caused by other diseases such as coccidiosis. NE is commonly seen in 2- to 5-week-old broiler chickens and the clinical form of the disease is characterized by a high mortality rate of up to 50%, with consequent economic losses to the poultry industry. The subclinical form of NE does not result in significant mortality and is only associated with mild damage to the intestine. However, this form may cause significant financial losses through impairing the ability of the intestine to absorb nutrients, ultimately resulting in a significant reduction in performance parameters (Gholamiandehkordi et al., Reference Gholamiandehkordi, Timbermont, Lanckriet, Van Den Broeck, Pedersen, Dewulf, Pasmans, Haesebrouck, Ducatelle and Van Immerseel2007; Shojadoost et al., Reference Shojadoost, Vince and Prescott2012).
Over the past several decades, following the discovery of NE by Parish in 1961 (Parish, Reference Parish1961), several preventive measures have been attempted for disease prevention and control, with dietary inclusion of antibiotic growth promoters (AGPs) in poultry feed being the most widely used approach worldwide (Elwinger et al., Reference Elwinger, Berndtson, Engström, Fossum and Waldenstedt1998). However, the ban on preventive use of in-feed antibiotics in the European Union countries (Levy, Reference Levy2014), USA and Canada has the potential to impose substantial economic losses to the poultry industry due to the re-emergence of once well-controlled diseases, including NE (M'Sadeq et al., Reference M'Sadeq, Wu, Swick and Choct2015). As a result, a wide range of studies have been dedicated to understanding the pathogenesis of CP and development of novel methods for prevention of disease (Caly et al., Reference Caly, D'Inca, Auclair and Drider2015; Prescott et al., Reference Prescott, Parreira, Mehdizadeh Gohari, Lepp and Gong2016; Smyth, Reference Smyth2016). Indeed, research in this area has led to the discovery of a novel pore-forming toxin called NetB (Keyburn et al., Reference Keyburn, Boyce, Vaz, Bannam, Ford, Parker, Di Rubbo, Rood and Moore2008), as well as detection of specific genomic structures that play a role in the virulence potential of CP, indicating that NE is not merely a toxin-mediated disease (Lepp et al., Reference Lepp, Roxas, Parreira, Marri, Rosey, Gong, Songer, Vedantam and Prescott2010). These breakthroughs have paved the path toward a better understanding of CP pathogenesis and developing effective control strategies against NE.
Many studies have been conducted to investigate the effect of antimicrobial alternatives such as probiotics, prebiotics, enzymes, essential oils, and organic acids, in addition to vaccination for prevention of NE in broiler chickens (Adhikari et al., Reference Adhikari, Kiess, Adhikari and Jha2020). Yet, despite a tremendous amount of research on this topic, there is currently no vaccine or feed additive available that offers complete protection against NE; as a result, the annual global economic losses incurred by this disease are still estimated to be approximately $6 billion USD (Wade et al., Reference Wade, Keyburn, Seemann, Rood and Moore2015). In this article, we review different aspects of NE with a focus on the pathogenesis and associated immune responses, in addition to highlighting the current strategies of NE prevention in chickens, including vaccines and antimicrobial alternatives.
Pathogenic mechanisms of C. perfringens in chickens
Pathogenesis of CP is very complex and depends on host, microbe, and management (including diet) factors (Fig. 1). Pathogenesis of NE is complicated due to the involvement of many factors, including colonization by CP via intestinal mucus degradation and subsequent penetration of mucosal surfaces; quorum sensing; and production of tissue-degrading toxins, such as NetB (Keyburn et al., Reference Keyburn, Boyce, Vaz, Bannam, Ford, Parker, Di Rubbo, Rood and Moore2008), α-toxin and toxin C. perfringens large cytotoxin (TpeL) (Prescott et al., Reference Prescott, Parreira, Mehdizadeh Gohari, Lepp and Gong2016). Among the toxins produced by CP, the NetB toxin has long been thought to be the major virulence factor of CP. Following the initial identification of NetB, research efforts have provided a detailed understanding of the extra-chromosomal location of the gene encoding this toxin, uncovered its role in the pathogenicity of CP and explored the possibility of using it as a target antigen in vaccine strategies (Rood et al., Reference Rood, Keyburn and Moore2016). Genomic and transcriptomic analyses have provided new insights into pathogenicity. Novel findings surrounding pathogenic mechanisms have included toxin production, quorum sensing, attachment to host tissues, and production of other pathogenicity-related enzymes, in addition to new data related to subclinical CP infection.
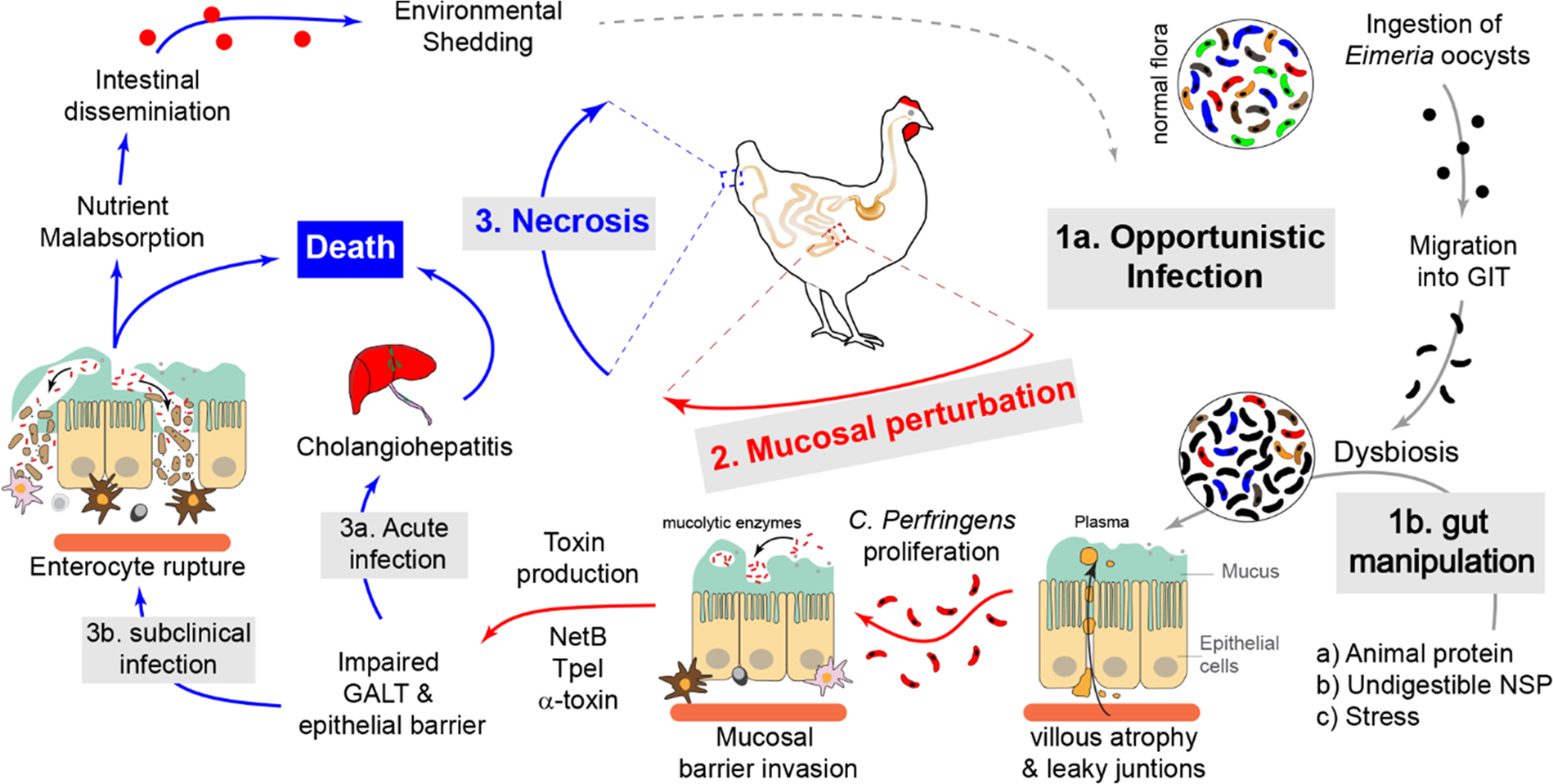
Fig. 1. Pathogenesis of C. perfringens infection in broiler chickens leads to NE. C. perfringens is an opportunistic pathogen that is normally found in a healthy gut flora of chickens. In a healthy gut, C. perfringens secretes low levels of enzymes and toxins that are subsequently neutralized by secretory antibodies (IgY and IgA). Mucosal and gut microbiota perturbation, caused by either feeding high animal protein or indigestible NSP diet (1b) and or ingestion of Eimeria oocysts which is associated with plasma leakage into the gut lumen, lead to C. perfringens growth and proliferation. The uncontrolled growth of C. perfringens is accompanied by secretion of a variety of mucolytic enzymes (sialidases, galactosidases, hexosaminidases and fucosidases) and pore-forming toxins such as NetB, allowing its penetration of the mucosa. Following penetration of the intestinal mucosa, C. perfringens secrete tissue-degrading toxins such as α-toxin and TpeL, which can lead to mild mucosal damage (subclinical NE) that is associated with nutrient malabsorption and dissemination of C. perfringens into the environment. In severe cases, the excessive secretion of these toxins may lead to extensive necrosis (clinical NE) and cholangiohepatitis that can eventually lead to death of chickens.
Comparative genomic and transcriptomic analyses of pathogenicity-related genes of C. perfringens
Transcriptomic analysis of virulence genes in CP
Analyses of genome content and gene expression allow for mechanisms of CP pathogenicity to be assessed from a broader viewpoint. For instance, transcriptomic analysis of a NetB positive CP strain has been used to assess relative differences in gene expression under in vitro and in vivo conditions (Parreira et al., Reference Parreira, Russell, Athanasiadou and Prescott2016). In a study by Parreira et al. (Reference Parreira, Russell, Athanasiadou and Prescott2016), significant differences in global gene expression were observed in CP subjected to different environmental conditions. When considering virulence genes specifically, it was found that the expression of these genes was the lowest in bacteria grown in a nutrient-poor medium, similar to those observed in CP recovered from chickens. More importantly, the notable decrease in the expression of virulence genes was found to coincide with decreased expression of regulatory VirR/VirS genes; however, expression of VirT, an alternate regulator of VirR/VirS regulated genes, was upregulated. This research highlighted the potential regulatory mechanisms employed by CP in environments of different nutrient availability.
Genomic analysis of virulence genes in CP
Genomic analysis of CP isolates allows for the identification of pathogenicity-related genes in CP and enables comparison between pathogenic and non-pathogenic strains. For instance, whole genome sequence analysis of CP isolated from a NE outbreak in chickens revealed the presence of genes related to toxin production, virulence elements, antibiotic resistance, and inserts of bacteriophage origin (Li et al., Reference Li, Yan and Lillehoj2017a). Further, comparative analyses of the genomes of different strains of CP have identified differences among them (Ronco et al., Reference Ronco, Stegger, Ng, Lilje, Lyhs, Andersen and Pedersen2017; Lacey et al., Reference Lacey, Allnutt, Vezina, Van, Stent, Han, Rood, Wade, Keyburn and Seemann2018a; Kiu et al., Reference Kiu, Brown, Bedwell, Leclaire, Caim, Pickard, Dougan, Dixon and Hall2019). In this regard, sequenced genomes from 30 chicken and turkey CP isolates revealed key differences in the presence of pathogenicity genes; NE-causing bacteria in chickens were found to contain NE pathogenicity loci (NELoc) 1, 2 and 3, netB and a collagen adhesin gene (cnaA), whereas in turkeys, only NELoc-2 was consistently observed along with limited representation of netB and cnaA (Ronco et al., Reference Ronco, Stegger, Ng, Lilje, Lyhs, Andersen and Pedersen2017). Despite identifying NetB in all NE-causing isolates, the results of the study by Ronco et al. (Reference Ronco, Stegger, Ng, Lilje, Lyhs, Andersen and Pedersen2017) suggest significant differences in pathogenic mechanisms between turkeys and chickens. This was further supported by identification of cnaD, a proposed collagen adhesin gene found in all isolates from diseased turkeys but only in a 39% of isolates from diseased chickens. Among strains of CP, some differences exist at the chromosomal level, although large-scale sequencing of isolates of CP from multiple host species demonstrated that pathogenicity was more closely linked to extra-chromosomal genes located on plasmids (Lacey et al., Reference Lacey, Allnutt, Vezina, Van, Stent, Han, Rood, Wade, Keyburn and Seemann2018a). In a similar study, comparing healthy and NE-affected-broiler CP isolates (Kiu et al., Reference Kiu, Brown, Bedwell, Leclaire, Caim, Pickard, Dougan, Dixon and Hall2019), netB was consistently observed in NE isolates, along with collagen adhesin genes and tpeL, the latter of which codes for a separate toxin, resulting in proposal of the existence of an extra-virulent lineage of bacteria. Overall, recent genomic and transcriptomic analyses of CP have provided further insight into the genes that in addition to netB, contribute to pathogenicity in chickens.
Toxin-mediated pathogenic mechanisms
The pathogenic mechanisms employed by CP toxins were recently summarized (Navarro et al., Reference Navarro, McClane and Uzal2018). In chickens, primary pathogenic mechanisms are attributed to the presence of NetB toxin, yet research has suggested roles for other toxins. Rehman et al. have reported that α-toxin dysregulates the function of gastrointestinal epithelial cell membranes (Rehman et al., Reference Rehman, Awad, Lindner, Hess and Zentek2006, Reference Rehman, Ijaz, Specht, Dill, Hellweg, Männer and Zentek2009). More recently, CP α-toxin has been shown to induce inflammatory responses in chicken intestinal epithelial cells (Guo et al., Reference Guo, Li, Liu and Guo2015). Nonetheless, in chickens, the association of NE and the presence of the NetB toxin in CP bacteria is well characterized. There has been considerable controversy whether other toxins that are produced by CP could also be mechanistically responsible for producing or enhancing NE disease in chickens; for example, production of the TpeL toxin has been suggested to enhance the virulence of NetB positive strains (Prescott et al., Reference Prescott, Parreira, Mehdizadeh Gohari, Lepp and Gong2016). However, no such effect was observed in a subsequent study (Yang et al., Reference Yang, Chou and Wang2018). To study this relationship further, recent work assessed 22 CP strains that all lacked the netB gene, while some harbored tpeL (Llanco et al., Reference Llanco, Nakano, de Moraes, Piazza and Avila-Campos2017). Specific in vitro pathogenicity analyses were performed, which showed that the presence of TpeL is associated with cellular adherence, but not necessarily with invasive processes. In a separate surveillance study, 19 netB-positive strains of CP were isolated from NE-afflicted broilers and TpeL carriage was observed in five of the strains which were also shown to produce the toxin protein (Gu et al., Reference Gu, Lillehoj, Sun, Lee, Zhao, Xianyu, Yan, Wang, Lin, Liu and Li2019). These strains were shown to be virulent in broilers, including one specific strain that decreased bird growth rate significantly. The study by Gu et al. (Reference Gu, Lillehoj, Sun, Lee, Zhao, Xianyu, Yan, Wang, Lin, Liu and Li2019) highlighted the importance of these toxins in the occurrence of NE, and suggested the use of TpeL- and NetB-positive strains of CP for challenge studies. However, despite the apparent importance of NetB and TpeL toxins with NE, a recent qPCR analysis of CP isolates from NE-afflicted and healthy chickens identified no significant differences in the presence or copy number of the netB gene (Yang et al., Reference Yang, Chou and Wang2018). Additionally, TpeL was only found in NetB-positive strains and was not associated with other NE-causing strains. These recent data cast doubt on the nature of the involvement of TpeL and NetB toxins in the pathogenesis of NE caused by CP, suggesting a multifactorial model of disease, although they do not negate NetB as a virulence factor. These results coincide with recent research examining the effects of NELoc-1-encoding genes, including netB, on the pathogenicity of CP in chickens (Zhou et al., Reference Zhou, Lepp, Pei, Liu, Yin, Ma, Prescott and Gong2017). Spontaneous loss of the plasmid that contains NELoc-1 after serial in vitro subcultures allowed Zhou et al. (Reference Zhou, Lepp, Pei, Liu, Yin, Ma, Prescott and Gong2017) to restore only NetB in these bacteria, to explore mechanisms of pathogenicity. Restoration of NetB alone recovered in vitro cytotoxicity during infection of chicken hepatoma cells; however, lesion scores after in vivo chicken infection were not completely restored compared to the wild-type NELoc-1-containing bacteria. Finally, recent research has shown that transfer of netB-containing plasmids, from one strain of CP bacteria to another strain that does not contain netB, can occur in the chicken gastrointestinal tract, leading to enhanced virulence in the new strain (Lacey et al., Reference Lacey, Keyburn, Ford, Portela, Johanesen, Lyras and Moore2017).
Non-toxin-mediated pathogenic mechanisms
Beyond toxin-mediated mechanisms, other proteins produced by CP have been shown to impact its virulence and pathogenicity. Previous work of Kulkarni et al. (Reference Kulkarni, Parreira, Sharif and Prescott2007) identified certain immunogenic CP proteins that were shown to induce robust antibody responses and immunity to NE in chickens. These proteins also included certain housekeeping enzymes such as glyceraldehyde-3-phosphate dehydrogenase (GPD), pyruvate: ferredoxin oxidoreductase (PFOR) and fructose 1,6-bisphosphate aldolase (FBA). The fact that these proteins are involved in NE protection (Kulkarni et al., Reference Kulkarni, Parreira, Sharif and Prescott2008, Reference Kulkarni, Parreira, Jiang and Prescott2010) highlighted the likely involvement of such housekeeping or energy metabolism proteins in the pathogenesis of NE. Along similar lines, one factor that is important in NE pathogenicity is the ability of CP to adhere to the intestinal mucosal surface. Binding capability to collagen and gelatin has been explored in disease- and non-disease-causing strains of CP, demonstrating that the former showed significantly greater binding capacity (Wade et al., Reference Wade, Keyburn, Seemann, Rood and Moore2015). This was attributed to the presence of a putative collagen adhesion gene, cnaA, in disease-causing strains, which was thought to be responsible for binding of CP to certain types of collagens in addition to gelatin. These observations were supported by further studies demonstrating that targeted mutation of cnaA, in two separate strains of CP, significantly decreases binding to collagens and gelatin, abolishing the ability for one strain to colonize while inhibiting colonization for the other (Wade et al., Reference Wade, Keyburn, Haring, Ford, Rood and Moore2016). In addition to adherence, degradation of mucus in the chicken gastrointestinal tract has also been proposed as a mechanism of colonization and pathogenicity employed by CP. Mucus degradation was explored by MacMillan et al. (Reference MacMillan, Vicaretti, Noyovitz, Xing, Low, Inglis, Zaytsoff, Boraston, Smith and Uwiera2019), where they showed that CP metabolizes specific monosaccharides that are present in mucin glycans present in the gastrointestinal tract of poultry, providing the bacteria a nutrient source and the ability to penetrate initial physical innate defenses. Similar work has been done, examining two zinc metalloproteases, ZmpA and ZmpB, which share some nucleic acid sequence homology but currently lack known substrates (Wade et al., Reference Wade, Keyburn, Haring, Ford, Rood and Moore2020). ZmpB is chromosomally located, while zmpA is located on a plasmid within NELoc-1; in 83 isolates of CP, zmpB was identified in every isolate, while zmpA was identified in 34 of 36 isolates from NE-afflicted chickens. Despite the apparent connection between ZmpA and pathogenesis, targeted mutation of both genes resulted in mutant bacteria that were significantly less able to cause intestinal disease, and the effects of mutating both were not significantly different from the effects of single mutations. This accumulation of recent research is showing that pathogenesis of CP-induced NE is a complex process that likely extends beyond production of the NetB toxin.
Other potential determinants of pathogenesis include gene expression regulatory mechanisms such as the VirR/VirS two-component system and quorum sensing systems, including LuxS and Agr-like systems. Experimental mutation of these regulatory elements revealed that the loss of VirR/VirS-mediated regulation or Agr-like-mediated quorum sensing significantly decreases in vitro bacterial cytotoxicity, which is not affected by mutational loss of the LuxS system (Yu et al., Reference Yu, Lepp, Gohari, Wu, Zhou, Yin, Yu, Prescott, Nie and Xie2017). Expression of netB was also significantly decreased in a similar pattern following experimental mutations. Finally, Yu et al. (Reference Yu, Lepp, Gohari, Wu, Zhou, Yin, Yu, Prescott, Nie and Xie2017) showed that in vivo pathogenicity is also significantly reduced in broilers administered bacteria harboring mutations in the Agr-like system, but pathogenicity was restored in bacteria containing a plasmid with Agr-like gene sequences.
There is currently evidence demonstrating that pathogenesis of CP extends beyond the presence of NetB and includes other pathogenic proteins and enzymes which are under genetic regulation. Further understanding of these pathogenic mechanisms will allow for the design of novel prophylactic and therapeutic approaches for chickens.
Immunity against C. perfringens
Immunity against CP is mediated by a concerted interaction between innate and adaptive host mechanisms. The following summarizes our current understanding of these mechanisms.
Host innate responses
The intestinal epithelial cell barrier is well equipped for microbial sensing by means of pattern recognition receptors (PRRs), including Toll-like receptors (TLR) (Keestra et al., Reference Keestra, de Zoete, Bouwman, Vaezirad and van Putten2013). Most of the immunological processes that take place in the intestinal mucosa against CP are initiated by secreted products of cells of the epithelium and underlying lamina propria (Guo et al., Reference Guo, Li, Liu and Guo2015). Structural components of CP or its secreted toxins interact with epithelial cells leading to pro-inflammatory responses which contribute to disease progression. Lu et al. (Reference Lu, Sarson, Gong, Zhou, Zhu, Kang, Yu, Sharif and Han2009), have demonstrated significant increases in ileal expression of immune response genes in broiler chickens in response to CP infection, including expression of TLR1, myeloid differentiation primary response 88 (MyD88), tumor necrosis factor receptor-associated factor 6 (TRAF6), TIR-domain-containing adapter-inducing interferon-β (TRIF), interleukin (IL)-8 and interferon regulatory factor 3 (IRF3). Expression of TLR2, TLR4, TLR7 and TLR15 was also increased in the spleen. Thus, it is clear CP induces both mucosal and systemic responses to infection (Lu et al., Reference Lu, Sarson, Gong, Zhou, Zhu, Kang, Yu, Sharif and Han2009). Intracellular signaling mediated by TLR1, TLR2 and TLR4 can trigger expression of antimicrobial peptides (cathelicidins and β-defensins) (Cuperus et al., Reference Cuperus, Coorens, van Dijk and Haagsman2013; Wang et al., Reference Wang, Hussain, Zhou, Zeng, Wang, Zou, Gong, Zhao and Li2020). Defensins are host defense peptides (HDPs) that are involved in host innate immunity (Hancock et al., Reference Hancock, Haney and Gill2016). Avian β-defensins (avBD) are produced by epithelial cells of the gastrointestinal tract and play a critical role in gut defense mechanisms against a broad spectrum of enteric pathogens by disrupting microbial cell membranes, inducing cell death (van Dijk et al., Reference van Dijk, Veldhuizen and Haagsman2008; Sugimura et al., Reference Sugimura, Jounai, Ohshio, Tanaka, Suwa and Fujiwara2013). Hong et al. have examined expression of avBD 1−14 in intestines and spleens of broiler chicks infected experimentally with CP. CP induced distinct patterns of expression of β-defensins, with different avBD being expressed in spleen and intestinal tissues (Hong et al., Reference Hong, Song, Lee and Lillehoj2012). Significant differences in patterns of expression were noted between chicks from two commercial broiler lines, suggesting that genetic differences may contribute to variation in β-defensin responses. It has been demonstrated in vitro that a recombinant avBD6 is highly effective, in a time- and concentration-dependent manner, against CP by limiting its growth (van Dijk et al., Reference van Dijk, Veldhuizen, Kalkhove, Tjeerdsma-van Bokhoven, Romijn and Haagsman2007). Importantly, there is no evidence to date demonstrating that expression of intestinal β-defensins provides an effective anti-CP activity in vivo. Indeed, necrosis of intestinal tissue progresses despite expression of avBD.
Modulation of TLR signaling pathways has been investigated as a means of reducing morbidity due to CP. Diets formulated with yeast extract containing mannan-oligosaccharides (TLR2 ligands), however, have had limited impact on bird body weight gains, performance, and gut morphology of CP-infected chickens (Yitbarek et al., Reference Yitbarek, Echeverry, Brady, Hernandez-Doria, Camelo-Jaimes, Sharif, Guenter, House and Rodriguez-Lecompte2012; Alizadeh et al., Reference Alizadeh, Rogiewicz, McMillan, Rodriguez-Lecompte, Patterson and Slominski2016). An in vitro experiment has demonstrated that the pro-inflammatory effects of CP mediated through the TLR4 signaling pathway can be blocked by treatment with Saccharomyces boulardi, a non-pathogenic probiotic yeast (Wang et al., Reference Wang, Hussain, Zhou, Zeng, Wang, Zou, Gong, Zhao and Li2020). Confirmation from in vivo studies is needed.
Chicken professional antigen presenting cells (APCs), such as macrophages and dendritic cells, play an important role in the development of adaptive immunity (De Geus and Vervelde, Reference De Geus and Vervelde2013). There is very little information on the type and function of APCs involved in the initiation of immune responses against CP. While direct functions are not clear, detection of interleukin (IL)-1β, IL-6, IL-8 and IL-12 transcripts indicate a likely rapid host response and a role for APCs in supporting activation of B and T cells (Yitbarek et al., Reference Yitbarek, Echeverry, Brady, Hernandez-Doria, Camelo-Jaimes, Sharif, Guenter, House and Rodriguez-Lecompte2012; Fasina and Lillehoj, Reference Fasina and Lillehoj2019). In addition to pro-inflammatory properties of the chemokine, IL-8, it plays a role in the recruitment and activation of granulocytes and macrophages, with subsequent nitric oxide (NO) production (Guo et al., Reference Guo, Li, Liu and Guo2015) and translocation of major histocompatibility complex (MHC) class II receptors to the cell surface (Li et al., Reference Li, Lillehoj, Lee, Lee, Park, Jang, Bauchan, Gay, Ritter and Bautista2010). It has been reported that in vitro stimulation of chicken embryonic fibroblast cells (CEFs) with CP can lead to NO production in a TLR4-dependent manner (Zhang et al., Reference Zhang, Lv, Li, Guo, Liu and Guo2017a). Limiting TLR4 induction in macrophage cell lines can reduce CP-mediated inducible nitric oxide synthase expression (Guo et al., Reference Guo, Li, Liu and Guo2015) and corresponding NO production (Wang et al., Reference Wang, Hussain, Zhou, Zeng, Wang, Zou, Gong, Zhao and Li2020). Localized intestinal macrophage activation can increase the permeability of the endothelium leading to serum loss into the intestinal lumen. Thus, innate responses that are essential for defense against many pathogens may, in the case of CP, exacerbate the disease process.
Adaptive immune responses
The mechanism for B-cell activation is not well defined in chickens. B-cell activation and antibody production is mediated by a combination of cytokines and cognate antigen (Davani et al., Reference Davani, Pancer and Ratcliffe2014). Infection with CP leads to a significant increase in intestinal IL-4 and IL-10 transcripts (Collier et al., Reference Collier, Hofacre, Payne, Anderson, Kaiser, Mackie and Gaskins2008), key cytokines involved in B-cell activation. In addition, CP infection leads to induction of transforming growth factor (TGF)-β (Fasina and Lillehoj, Reference Fasina and Lillehoj2019) and IL-10 (Yitbarek et al., Reference Yitbarek, Echeverry, Brady, Hernandez-Doria, Camelo-Jaimes, Sharif, Guenter, House and Rodriguez-Lecompte2012) cytokine transcripts in the small intestine, traditionally associated with inducing an immunosuppressive milieu. In combination with specific cognate antigens, these cytokines (IL-10, TGF-β, IL-4) can induce antigen-specific B-cell differentiation (Davani et al., Reference Davani, Pancer and Ratcliffe2014). While these mechanisms have not been fully explored in CP-infected chickens, detection of antigen-specific IgY antibodies in vaccinated breeder hens demonstrates class switching (IgM to IgY antibody isotype) as well as the presence of plasma B cells (Keyburn et al., Reference Keyburn, Portela, Ford, Bannam, Yan, Rood and Moore2013a). Therefore, passive immunity in progeny chicks from vaccinated hens is mediated by maternal IgY antibodies that can effectively neutralize toxins secreted by pathogenic CP. In addition, IL-4, IL-10 and IL-23 initiate cellular repair processes, limit inflammation and promote B-cell activation (Degen et al., Reference Degen, Van Zuilekom, Scholtes, Van Daal and Schijns2005; Fasina and Lillehoj, Reference Fasina and Lillehoj2019). These results provide a framework whereby host response against infection with CP elicits a regulatory environment to limit tissue damage and increase antibody production while attempting to decrease bacteria tissue penetration.
Recent studies of immunity to CP have led to the discovery and characterization of antigens within its bacterial immunome (Kulkarni et al., Reference Kulkarni, Parreira, Sharif and Prescott2008; Keyburn et al., Reference Keyburn, Portela, Ford, Bannam, Yan, Rood and Moore2013a). Vaccine candidate antigens were identified by screening IgY and IgA antibodies from immunized and challenged broiler chickens against CP cellular and secretory antigens. PFOR, α-toxin, FBA and hypothetical protein (HP) were selected for further investigation (Kulkarni et al., Reference Kulkarni, Parreira, Jiang and Prescott2010). Epitope mapping studies demonstrated a broad range of B-cell epitopes in PFOR, α-toxin, FBA and HP (Kulkarni et al., Reference Kulkarni, Parreira, Sharif and Prescott2008, Reference Kulkarni, Parreira, Jiang and Prescott2010). Two major segments of α-toxin (amino acid positions 96−122 and 183−212) were highly antigenic (Kulkarni et al., Reference Kulkarni, Parreira, Jiang and Prescott2010) whereas the length of HP was demonstrated to be highly antigenic (Kulkarni et al., Reference Kulkarni, Parreira, Sharif and Prescott2008). In addition, B-cell epitope mapping demonstrated a total of 94 peptides in PFOR showing potential for broad antigen responses (Kulkarni et al., Reference Kulkarni, Parreira, Sharif and Prescott2008). Recombinant α-toxin, HP and PFOR administered intramuscularly induced significant protection in broiler chickens against challenge with CP (Kulkarni et al., Reference Kulkarni, Parreira, Sharif and Prescott2007). In subsequent work, B-cell epitopes of HP and α-toxin were cloned separately into a Salmonella enterica vector, and chickens were vaccinated orally with the live vaccines. Both antigens induced significant protection against experimental challenge (Kulkarni et al., Reference Kulkarni, Parreira, Jiang and Prescott2010).
Several groups have examined maternal vaccination to provide passive (maternal) antibodies against CP to their chicks (Kulkarni et al., Reference Kulkarni, Parreira, Jiang and Prescott2010; Keyburn et al., Reference Keyburn, Portela, Sproat, Ford, Bannam, Yan, Rood and Moore2013b). Keyburn et al. have investigated vaccines containing recombinant NetB protein and/or a CP toxoid preparation. Hens received the vaccine subcutaneously, and their progeny were challenged at 2 weeks post-hatch. Maternal antibodies from the hens mediated significant protection (Keyburn et al., Reference Keyburn, Portela, Ford, Bannam, Yan, Rood and Moore2013a). These studies highlight important practical considerations. Because NE is a major concern in rapidly growing young birds, vaccination schemes must provide protection at a young age and must be convenient for mass administration.
T helper (Th) cells can be viewed as an essential component in the early phase of CP pathogenesis (Collier et al., Reference Collier, Hofacre, Payne, Anderson, Kaiser, Mackie and Gaskins2008). Most experimental models of NE induce a T-cell-mediated inflammatory response, leading to enhanced intestinal mucogenesis (Collier et al., Reference Collier, Hofacre, Payne, Anderson, Kaiser, Mackie and Gaskins2008). Increased expression of pro-inflammatory IL-1β and decreased expression of anti-inflammatory TGF-β have been documented in the jejunum of broiler chicks 7 days post-challenge with CP (Fasina and Lillehoj, Reference Fasina and Lillehoj2019). Expression of IL-13 by intestinal T cells enhances mucin production, providing a growth advantage to CP (Collier et al., Reference Collier, Hofacre, Payne, Anderson, Kaiser, Mackie and Gaskins2008; Fasina and Lillehoj, Reference Fasina and Lillehoj2019). In contrast, mucosal effector T cells are characterized by expression of cytokines such as IL-2, IL-17 and interferon (IFN)-γ that activate innate immune system cells and enhance antigen presentation by APCs (Brisbin et al., Reference Brisbin, Parvizi and Sharif2012; Taha-Abdelaziz et al., Reference Taha-abdelaziz, Alkie, Hodgins, Shojadoost and Sharif2016). However, broiler chicks infected with CP are reported to have reduced IFN-γ and IL-2 transcripts and increased IL-10 and IL-17 transcripts in jejunal tissue by 7 days post-challenge (Fasina and Lillehoj, Reference Fasina and Lillehoj2019). Therefore, microbiota-driven IL-17 expression could indicate the involvement of Th17 which play a critical role in mucosal inflammation, induction of antimicrobial peptides and enhancing mucosal repair (Walliser and Göbel, Reference Walliser and Göbel2018). Along with these effects, it has also been observed that CP infections induce a reduction in IL-22, expressed by Th17 cells, which is critical for maintaining gut epithelial cell survival, proliferation and induction of antimicrobial peptides (Collier et al., Reference Collier, Hofacre, Payne, Anderson, Kaiser, Mackie and Gaskins2008). These results demonstrate a potential role for γδ T cells as an essential primary mucosal barrier defense against CP infection and progression to NE. Decreased intestinal T-cell function in CP-infected chickens compared to non-infected chickens has been demonstrated ex vivo, based on a lack of response to mitogen stimulation (Li et al., Reference Li, Lillehoj, Lee, Lee, Park, Jang, Bauchan, Gay, Ritter and Bautista2010). Studies of T-cell responses to CP have been relatively few in number compared to studies of B cells, and more extensive investigations are needed.
Farm management practices and nutritional strategies
Farm management is considered one of the key factors that contribute to the incidence of CP in poultry flocks (Tsiouris, Reference Tsiouris2016). Accumulating evidence indicates that environmental stressors, such as heat and cold stress, vaccination, processing in the hatchery, transportation to the farm, wet litter, poor ventilation, and high stocking density, can disturb the homeostasis of the intestine and negatively impact the immune systems of chicks, thereby increasing the incidence and severity of NE in chickens (Hangalapura et al., Reference Hangalapura, Nieuwland, Buyse, Kemp and Parmentier2004; Hirakawa et al., Reference Hirakawa, Nurjanah, Furukawa, Murai, Kikusato, Nochi and Toyomizu2020).
High stocking density increases the risk of horizontal transmission of CP between chickens by spreading spores through air or direct contact, and it is often associated with a substantial accumulation of litter, which provides a supportive niche for CP sporulation (McDevitt et al., Reference McDevitt, Brooker, Acamovic and Sparks2006; Guardia et al., Reference Guardia, Konsak, Combes, Levenez, Cauquil, Guillot, Moreau-Vauzelle, Lessire, Juin and Gabriel2011). Furthermore, it has been reported that high stocking density increases the CP-associated gut lesion scores and pH in the intestine as well as the CP counts in the caeca of chickens (Tsiouris et al., Reference Tsiouris, Georgopoulou, Batzios, Pappaioannou, Ducatelle and Fortomaris2015). In addition to increasing the susceptibility of birds to pathogens, cold stress may also contribute to the pathogenesis of NE in chickens (Regnier and Kelley, Reference Regnier and Kelley1981). Tsiouris et al. (Reference Tsiouris, Georgopoulou, Batzios, Pappaioannou, Ducatelle and Fortomaris2015) investigated the role of cold stress in the pathogenesis of NE in broiler chickens and found that exposure to cold stress increases the incidence of NE as well as the severity of lesions in chickens experimentally challenged with CP. Likewise, exposure of chickens to heat stress could also impair their growth, disrupt intestinal integrity, and suppress immune responses, thereby increasing the susceptibility of chickens to infections (Calefi et al., Reference Calefi, Honda, Costola-de-Souza, de Siqueira, Namazu, Quinteiro-Filho, da Silva Fonseca, Aloia, Piantino-Ferreira and Palermo-Neto2014). Chickens subjected to heat stress have been shown to have high intestinal lesion scores associated with CP infection in addition to the enhanced pH and viscosity of intestinal digesta (Tsiouris et al., Reference Tsiouris, Georgopoulou, Batzios, Pappaioannou, Ducatelle and Fortomaris2018). The mechanisms underlying these effects have not been fully established. However, existing evidence indicates that exposure of birds to low or high temperature causes immunosuppression, making them more vulnerable to intestinal infections (Tsiouris et al., Reference Tsiouris, Georgopoulou, Batzios, Pappaioannou, Ducatelle and Fortomaris2015, Reference Tsiouris, Georgopoulou, Batzios, Pappaioannou, Ducatelle and Fortomaris2018). Other environmental factors, such as reduced ventilation, high humidity, and poor litter condition, can also significantly influence the immune systems of birds and predispose them to NE (Dunlop et al., Reference Dunlop, Moss, Groves, Wilkinson, Stuetz and Selle2016; Hofacre et al., Reference Hofacre, Smith and Mathis2018).
In addition to the role of environmental stressors in increasing susceptibility to NE, gut damage caused by parasitic diseases such as coccidiosis is one of the major risk factors for NE (Williams, Reference Williams2005). Coccidiosis is a common parasitic intestinal disease caused in poultry by protozoan parasites of the genus Eimeria (Lillehoj and Lillehoj, Reference Lillehoj and Lillehoj2000). Infection with Eimeria usually occurs when birds ingest viable oocysts from contaminated litter. Following ingestion of sporulated oocysts, sporozoites penetrate the epithelial lining of the intestine and undergo extensive asexual reproduction, causing disruption of the gut integrity, followed by hemorrhage, inflammation, and excessive mucus production; all of these manifestations provide conditions favorable for CP colonization in the gut (Allen and Fetterer, Reference Allen and Fetterer2002; Williams, Reference Williams2005). Therefore, controlling coccidiosis through vaccination programs and litter management would reduce the incidence of NE in poultry flocks (Bangoura et al., Reference Bangoura, Alnassan, Lendner, Shehata, Krüger and Daugschies2014).
Biosecurity practices at the farm level are also very important for prevention of potential horizontal transmission of infections, including CP, within the flock or to other flocks (Tsiouris, Reference Tsiouris2016). Generally, farm biosecurity measures include disinfecting poultry houses, equipment, vehicles, fly screens, boot dips, and restricted entry to the barn with shower-in and shower-out facilities (Tsiouris, Reference Tsiouris2016). Dietary composition is also considered one of the critical factors that may contribute to the pathogenesis of NE in chickens (McDevitt et al., Reference McDevitt, Brooker, Acamovic and Sparks2006). High levels of non-starch polysaccharides (NSPs) in the diet have been shown to increase gastrointestinal viscosity and alter the gut microbiota composition, consequently leading to overgrowth of CP in the intestine (Jia et al., Reference Jia, Slominski, Bruce, Blank, Crow and Jones2009; Palliyeguru et al., Reference Palliyeguru, Rose and Mackenzie2010).
A higher incidence of NE has been observed in chickens fed wheat- or barley-based diets (contain large amount of NSPs; i.e. arabinoxylans and ß-glucans) than those fed corn-based diets (Annett-Christianson, Reference Annett-Christianson2012). This is thought to be due to the role of NSPs in increasing the water-holding capacity and the viscosity of the digesta, resulting in a prolonged passage rate of gut contents and excessive mucus production that serve as a nutrient source for CP (Jia et al., Reference Jia, Slominski, Bruce, Blank, Crow and Jones2009; Annett-Christianson, Reference Annett-Christianson2012).
There is some evidence that the level and source of dietary protein can significantly impact severity of NE in chickens. High indigestible protein in the digestive tract serves as a protein source for CP growth and proliferation, thus subsequently for NE. For example, high levels of amino acids such as methionine and glycine in the diet have been shown to accelerate CP growth in the small intestine of chickens (Drew et al., Reference Drew, Syed, Goldade, Laarveld and Van Kessel2004; Xue et al., Reference Xue, Wu, Choct and Swick2017). Drew et al. (Reference Drew, Syed, Goldade, Laarveld and Van Kessel2004) compared the effects of animal-based protein (fishmeal) and plant-based protein (soybean meal) on intestinal populations of CP in broilers and found that birds fed fishmeal-based diets had higher numbers of CP in ileum and caeca compared to those fed soybean-based diets. These data provide an explanation for the common use of animal proteins, especially fishmeal, to experimentally produce NE in chickens.
Considering the contributing roles of various environmental stressors, poor biosecurity practices and unbalanced diet composition in induction of NE, optimization of farm management practices together with implementing nutritional as well as disease control strategies could reduce the prevalence of NE in poultry farms. NE can be controlled with in-feed AGP; however, with concerns about bacterial resistance to antibiotics and antibiotic residues in poultry products, there is increased interest in the use of alternatives, such as probiotics, prebiotics, essential oils and organic acids for the control of NE (M'Sadeq et al., Reference M'Sadeq, Wu, Swick and Choct2015). Among all potential alternatives, probiotics have gained significant attention because of their broad immunomodulatory and antimicrobial activities (Koenen et al., Reference Koenen, Kramer, Van Der Hulst, Heres, Jeurissen and Boersma2004; Sornplang and Leelavatcharamas, Reference Sornplang and Leelavatcharamas2010). Thus, in this review we focus on the protective role of probiotic bacteria against NE in chickens.
Probiotics
Probiotics are defined as ‘live microorganisms which when administered in adequate amounts confer a health benefit to the host’. Probiotics exert their beneficial effects on chicken health though modulation of mucosal immune responses and intestinal microbiota, improvement of the integrity of the intestinal epithelial barrier, alteration of mucus secretion, competitive exclusion, and production of antimicrobial and immunomodulatory substances (Ng et al., Reference Ng, Hart, Kamm, Stagg and Knight2009; Bermudez-Brito et al., Reference Bermudez-Brito, Plaza-Díaz, Muñoz-Quezada, Gómez-Llorente and Gil2012). The probiotic mechanisms of action in prevention and control of CP-induced NE are summarized in Fig. 2.

Fig. 2. Modulation of the gut microbiota by probiotic lactobacilli mitigates against CP-induced NE. The roles of probiotic lactobacilli in prevention and treatment of CP-induced NE are depicted in four different mechanisms. (1) Modulation of gut microbiota composition by enhancing microbial diversity and richness with specific microbial groups, such as microbial members phyla Firmicutes and Bacteroidetes. Probiotics also compete with gut microbial pathogens including CP for intestinal niches and nutrients. (2) Enhancing the production of SCFAs by gut microbes which in turn enhance local and systemic immune responses. Locally, SCFA induces mucin production and enhances epithelial cell integrity and the expression of an immunoregulatory cytokine, IL-10. Systemically, SCFA enhances secretion of chemotactic factors leading to gut infiltration of both innate (macrophages and γδ T cells) and adaptive immune system cells (αβ T cells and B cells), and, more importantly, an increased responsiveness to stimulation. (3) Modulation of immune responses in intestinal mucosa and gut-associated lymphoid tissue (GALT) by stimulating the tissue-resident B cells to produce secretory antibodies (IgA and IgY) that are released via transcytosis, and by stimulating tissue-resident macrophages that directly activate various T-cell subsets, making them functionally more responsive to pathogenic challenge. Specific Lactobacillus species can have distinct immunomodulatory effects, mainly by limiting colonic inflammation (e.g. reducing Th17, increasing Treg expression and shifting macrophages to the M2 subtype) or by enhancing antibacterial immunity (e.g. enhancing Th17, reducing Treg expression and MHC-1 expression). (4) Secretion of antimicrobial substances either through direct or indirect competition. Direct competition is recognized as either competitive exclusion (aggregation and production of bacteria-derived antibiotics) or limiting/inhibiting colonization. These functions are mediated by lowering luminal pH and access to their respective binding sites on epithelial cells. Alternatively, Lactobacillus stimulate the intestinal epithelial barrier through TLR (TLR 4 and TLR21) as well as tissue-resident immune cells which actively produce antimicrobial peptides (β-defensins and cathelicidins) that possess direct bactericidal activity against CP.
Effects of probiotics on intestinal immune response of NE-infected chickens
Immunomodulatory activities of probiotics have been reported in several studies (Haghighi et al., Reference Haghighi, Gong, Gyles, Hayes, Sanei, Parvizi, Gisavi, Chambers and Sharif2005; Brisbin et al., Reference Brisbin, Zhou, Gong, Sabour, Akbari, Haghighi, Yu, Clarke, Sarson and Sharif2008, Reference Brisbin, Gong, Orouji, Esufali, Mallick, Parvizi, Shewen and Sharif2011; Bai et al., Reference Bai, Wu, Ding, Lei, Bai, Zhang and Chio2013; Alizadeh et al., Reference Alizadeh, Shojadoost, Astill, Taha-Abdelaziz, Karimi, Bavananthasivam, Kulkarni and Sharif2020). Probiotics can stimulate immune responses through interaction with PRRs expressed by various immune system cells and epithelial cells (Plantinga et al., Reference Plantinga, van Maren, van Bergenhenegouwen, Hameetman, Nierkens, Jacobs, de Jong, Joosten, van't Land and Garssen2011). This activation, however, does not lead to inflammation, but rather maintains intestinal homeostasis and keeps the immune system in a state of readiness to fight off opportunistic or invading pathogens (Yan and Polk, Reference Yan and Polk2011). Additionally, probiotics may reduce intestinal inflammation in response to enteric pathogens and inhibit apoptosis of intestinal epithelial cells (Plaza-Díaz et al., Reference Plaza-Díaz, Ruiz-Ojeda, Vilchez-Padial and Gil2017; Azad et al., Reference Azad, Kalam, Sarker and Wan2018). In the context of NE, CP induces intestinal inflammation, causing disruption of the structure of the gut barrier and enhancement of gut permeability (Prescott et al., Reference Prescott, Parreira, Mehdizadeh Gohari, Lepp and Gong2016). The potential role of probiotics in ameliorating CP-induced inflammation has been studied in several clinical trials. Cao et al. (Reference Cao, Yang, Li, Sun, Wu and Yao2012) demonstrated that oral administration of Lactobacillus fermentum in chickens challenged with CP significantly reduces the severity of gut inflammation caused by CP. This protective effect was associated with reduced expression of TLR2 and IFN-γ, and increased expression of IL-10 in the ileum of lactobacilli-treated birds compared to non-treated, CP-infected cohorts, indicating the role of probiotics in regulating intestinal mucosal immune response and in maintaining gut homeostasis during the course of NE (Cao et al., Reference Cao, Yang, Li, Sun, Wu and Yao2012).
In an in vitro study, pre-treatment of CP-infected intestinal cells with two Lactobacillus species (L. acidophilus and L. fermentum) was shown to reduce CP-induced expression of the transcription factor nuclear factor kappa B (NF-kB), peptidoglycan receptors, TLR2 and nucleotide-binding oligomerization domain-containing protein 1 (NOD1) receptors (Guo et al., Reference Guo, Liu, Zhang, Li, Li, Ding and Guo2017). In another study, Wang et al. (Reference Wang, Ni, Qing, Liu, Lai, Khalique, Li, Pan, Jing and Zeng2017), evaluated the effect of L. johnsonii on intestinal mucosal immunity of chickens challenged with CP. Supplementation with lactobacilli mitigated immune-related adverse events associated with NE, by enhancing the production of immunoglobulins (IgG and IgM) and the proliferation of IgA+ B cells and T-cell subsets (CD3+CD4+ and CD3+CD8+) in the ileum. In addition, L. johnsonii down-regulated the CP-induced mRNA expression of various cytokines, including IL-2, IL-8, IL-10, and IFN-γ, suggesting immunomodulatory activities of lactobacilli and their role in maintaining intestinal homeostasis following CP infection (Wang et al., Reference Wang, Ni, Qing, Liu, Lai, Khalique, Li, Pan, Jing and Zeng2017).
Effects of probiotics on intestinal barrier integrity
In chickens, intestinal barrier function is regulated by antimicrobial peptides and tight-junction proteins (TJPs) (Chelakkot et al., Reference Chelakkot, Ghim and Ryu2018). This section briefly reviews current knowledge on the role of probiotics in strengthening intestinal barrier integrity.
CP infection of chickens induces the expression of β-defensin genes, which is indicative of the critical role of HDPs in controlling NE (Hong et al., Reference Hong, Song, Lee and Lillehoj2012). Lactobacilli can improve intestinal barrier function by up-regulating the expression of β-defensins without provoking inflammatory responses. Treatment of intestinal Caco-2 cells with L. acidophilus, L. plantarum, and L. fermentum has been shown to enhance the expression and secretion of human β-defensin 2 (Schlee et al., Reference Schlee, Harder, Köten, Stange, Wehkamp and Fellermann2008). Similar observations were made in chicken intestinal epithelial cells; treatment of these cells with L. plantarum SJ, L. fermentum F6, L. rhamnosus MLGA, and L. rhamnosus MB12 enhanced mRNA expression of AvBD9, with L rhamnosus MLGA exhibiting stronger effects (Li et al., Reference Li, Hong, Jia, You, Zhang and Liu2012). In contrast, Akbari et al. demonstrated that the expression of antimicrobial peptides in cecal tonsils of chickens infected with Salmonella enterica was not altered following treatment with probiotics (L. acidophilus, Bifidobacterium bifidum, and Enterococcus faecalis) (Akbari et al., Reference Akbari, Haghighi, Chambers, Brisbin, Read and Sharif2008). These conflicting results might be explained by differences in Lactobacillus strains used in these studies, as well as the dose and frequency of administration. Furthermore, it should be noted that the latter study used only one concentration of these probiotics; it is unclear whether higher concentrations would affect the outcome and whether these lactobacilli would exert the same activity in CP-infected chickens. Overall, only a limited number of studies have evaluated the effects of probiotics on HDPs in chickens, and further research is needed to provide solid evidence of probiotic effects on intestinal antimicrobial peptides in CP-infected chickens.
TJPs play a critical role in maintaining intestinal barrier functions in chickens by holding intestinal epithelial cells together, protecting the gut from pathogen invasion (Vermette et al., Reference Vermette, Hu, Canarie, Funaro, Glover and Pierce2018). Disruption of TJPs will, therefore, lead to increased epithelial permeability allowing translocation of luminal pathogens and their toxins to the submucosa and internal organs, resulting in endogenous infection, and eventually tissue damage (Chen et al., Reference Chen, Ge, Fox and Schauer2006). It has been reported that CP endotoxins interact with structural components of epithelial TJPs, such as claudin and occludin, leading to increased tight junction permeability and diarrhea (Emami et al., Reference Emami, Calik, White, Young and Dalloul2019). There is some evidence that probiotics can enhance tight junction stability and decrease membrane permeability to CP. Wu et al. (Reference Wu, Zhen, Geng, Wang and Guo2019), investigated the effects of oral administration of Enterococcus faecium on intestinal integrity of chickens infected with CP. The results of this study revealed that while CP infection significantly decreased mRNA expression of TJPs, including CLDN-3 and ZO-1, and protein levels of ZO-1, and increased expression and protein levels of MLCK (a protein that increases paracellular permeability) in the jejunum, administration of E. faecium counteracted the adverse immunological effects of CP by up-regulating CLDN-1 mRNA transcript and protein levels in the jejunum of infected birds.
Alteration of mucin production and competitive exclusion
Mucus overlies the gut epithelium and functions as the first line of defense against pathogenic microorganisms (Pelaseyed et al., Reference Pelaseyed, Bergström, Gustafsson, Ermund, Birchenough, Schütte, van der Post, Svensson, Rodríguez-Piñeiro and Nyström2014). Mucus mainly consists of mucin, a highly glycosylated and interlinked protein secreted by specialized epithelial goblet cells (Pelaseyed et al., Reference Pelaseyed, Bergström, Gustafsson, Ermund, Birchenough, Schütte, van der Post, Svensson, Rodríguez-Piñeiro and Nyström2014). The mucus layer is the primary site for adherence and colonization by both commensal and pathogenic bacteria, including CP (Martens et al., Reference Martens, Neumann and Desai2018). Thus, strategies that can enhance resistance to colonization by CP could potentially alleviate NE. CP secretes various toxins including mucin-degrading and pore-forming toxins that disrupt the intestinal mucosal barrier, ultimately leading to necrotic lesions in the gut (Prescott et al., Reference Prescott, Parreira, Mehdizadeh Gohari, Lepp and Gong2016). Lactobacilli and their metabolites may induce mucus production through regulation of intestinal mucin gene expression (Rosique et al., Reference Rosique, Chamignon, Mhedbi-Hajri, Chain, Derrien, Vazquez, Garault, Cotillard, Pham and Chervaux2019). Xu et al. (Reference Xu, Chen, Yu, Wang, Huang and Zhu2020) demonstrated that dietary supplementation with L. plantarum, in laying hens infected with CP, significantly increased MUC2 gene expression in the ileum. Another study used Bacillus subtilis as a dietary supplement for broiler chickens and demonstrated a significant increase in the expression of intestinal MUC-2 mRNA, whereas supplementation with a multi-strain probiotic resulted in a significant increase in the number of goblet cells, with no detectable alteration of MUC-2 expression (Aliakbarpour et al., Reference Aliakbarpour, Chamani, Rahimi, Sadeghi and Qujeq2012). Treatment of Caco-2 cells with L. casei has also been shown to significantly enhance mRNA expression and protein levels of MUC-2 (Mattar et al., Reference Mattar, Teiltelbaum and Drongowski2003). Taken together, these findings highlight the ability of probiotic bacteria in promoting mucus production, and suggest their potential use as prophylactic agents to potentiate mucosal resistance against CP or as therapeutic agents to restore mucosal barrier function following infection with CP.
In addition to their role in promoting mucin secretion, probiotic bacteria can adhere to and colonize the mucus layer of the small intestine, driven by non-specific physical binding or by specific surface adhesion proteins such as mucin binding proteins (produced mainly by LAB) (Boekhorst et al., Reference Boekhorst, Helmer, Kleerebezem and Siezen2006). The adhesion abilities of probiotic bacteria enable them to compete with opportunistic enteric pathogens for ecological niches and nutrients, through a process referred to as competitive exclusion (CE) (Woo and Ahn, Reference Woo and Ahn2013). Recently, the anti-CP activity of L. acidophilus and L. fermentum has been investigated in CP-infected chicken intestinal epithelial cells. The results revealed that probiotic lactobacilli possess strong antagonistic activity against CP, demonstrated by a significant reduction of CP growth and α-toxin production as well as suppression of CP adhesion to intestinal epithelial cells, with L. acidophilus showing greater inhibitory effects (Guo et al., Reference Guo, Liu, Zhang, Li, Li, Ding and Guo2017). In an in vivo study, La Ragione et al. (Reference La Ragione, Narbad, Gasson and Woodward2004) demonstrated that oral inoculation of 1 × 109 CFU of L. johnsonii FI9785 to chicks 24 h prior to challenge with CP, significantly reduces CP colonization and shedding. The authors suggested that the beneficial effects observed in the study were a result of CE. However, the inconclusive nature of these results warrants more in-depth investigation to determine whether the observed effects are attributable to CE or related to other immunomodulatory effects associated with probiotics.
Antimicrobial activity of probiotics
One of the important mechanisms of action of probiotics is their ability to produce antimicrobial substances such as hydrogen peroxide, NO, and bacteriocins (Cotter et al., Reference Cotter, Ross and Hill2013). Bacteriocins are a large family of ribosomally synthesized peptides that have antimicrobial activities against bacterial pathogens (Zacharof and Lovitt, Reference Zacharof and Lovitt2012). These molecules have broad-spectrum activity and can target specific pathogens without exerting negative effects on commensal bacteria (Dobson et al., Reference Dobson, Cotter, Ross and Hill2012). Bacteriocins may directly eliminate pathogens or may function as signaling peptides that facilitate coordination of multicellular processes and synchronize group behavior within bacterial populations or may serve as colonizing peptides giving probiotics a competitive advantage over resident pathogens (Cotter et al., Reference Cotter, Ross and Hill2013). Antagonistic activities of bacteriocin-producing probiotics against CP have been reported in different studies (Ben Lagha et al., Reference Ben Lagha, Haas, Gottschalk and Grenier2017). In an in vitro study, it was found that bacteriocin-producing B. subtilis PB6 exhibits significantly high inhibitory effects on the growth of various strains of CP (Teo and Tan, Reference Teo and Tan2005). In another study, Grilli et al. (Reference Grilli, Messina, Catelli, Morlacchini and Piva2009) demonstrated that bacteriocin produced by Pediococcus pentosaceus (pediocin A), exhibited potent antagonistic activities against CP and significantly improved growth performance of chickens infected with CP.
Production of short-chain fatty acids (SCFAs)
Another important mechanism by which probiotics contribute to pathogen clearance is through secretion of SCFAs, such as acetate, butyrate, and propionate (Sun and O'Riordan, Reference Sun and O'Riordan2013). In addition to their beneficial effects as a source of energy for intestinal epithelium, SCFAs lower the pH of the intestine, making the conditions unfavorable for growth and proliferation of entropathogens. There is also evidence that SCFAs influence bacterial populations in the ceca of broiler chickens (Van der Wielen et al., Reference van der Wielen, Biesterveld, Notermans, Hofstra, Urlings and van Knapen2000). Although fermentative bacteria, such as lactobacilli, are intrinsically resistance to low pH, a significant negative correlation has been observed between numbers of Enterobacteriaceae and the concentration of undissociated SCFAs in the cecum of chickens (Bearson et al., Reference Bearson, Bearson and Foster1997). It has been suggested that undissociated SCFAs may diffuse across bacterial membranes into bacterial cells and dissociate in the cytoplasm, leading to a decreased internal pH and finally cell death (Russell and Diez-Gonzalez, Reference Russell and Diez-Gonzalez1997). Recent studies have demonstrated that dietary inclusion of microencapsulated sodium butyrate significantly reduces the CP-induced intestinal lesions and improves body weight gain of chickens experimentally challenged with CP (Song et al., Reference Song, Li, Wu, Zhen, Wang, Xia and Guo2017; Liu et al., Reference Liu, Lumpkins, Mathis, Williams and Fowler2019).
Antioxidant activity of probiotics
The ability of lactobacilli to stimulate the antioxidant system of the host has been investigated both in vivo and in vitro (Mishra et al., Reference Mishra, Shah, Mokashe, Chavan, Yadav and Prajapati2015). It is thought that probiotics exert their antioxidant activities through scavenging free radicals in the intestine (Kodali and Sen, Reference Kodali and Sen2008). In the context of NE, it has been reported that infection with CP significantly lowers the antioxidant capacity of birds by increasing the malondialdehyde (MDA) level (an indicator of lipid peroxidation) and decreasing the activity of superoxide dismutase (SOD), catalase (CAT), and glutathione peroxidase (GSH-Px) in serum (Zhou et al., Reference Zhou, Zeng, Ni, Tu, Yin, Pan and Jing2016; Wang et al., Reference Wang, Ni, Qing, Liu, Lai, Khalique, Li, Pan, Jing and Zeng2017). On the other hand, dietary supplementation of L. johnsonii to CP-challenged chickens enhances the antioxidant capacity of intestinal mucosa by reducing MDA levels and increasing the total antioxidation capacity (T-AOC), CAT, and SOD activities, all of which are vital for healthy intestinal function (Wang et al., Reference Wang, Ni, Qing, Liu, Lai, Khalique, Li, Pan, Jing and Zeng2017). Similar observations have been made in another study demonstrating that B. licheniformis supplementation to broiler chickens challenged with CP significantly increases CAT and GSH-Px activities and reduces MDA levels in serum (Zhou et al., Reference Zhou, Zeng, Ni, Tu, Yin, Pan and Jing2016). In addition, the expression levels of genes related to fatty acid synthesis (acetyl-CoA carboxylase) and oxidation (carnitine palmitoyltransferase-1 and proliferator-activated receptor-α) were upregulated. The authors added that the positive impact of probiotics on chicken growth performance is mostly attributable to their role in improving antioxidant activities and lipid metabolism.
Effects of probiotics on gut microbiome
It has been reported that gut dysbiosis caused by CP may enhance host susceptibility to other bacterial infections leading to exacerbation of an already existing mucosal inflammation (Lacey et al., Reference Lacey, Stanley, Keyburn, Ford, Chen, Johanesen, Lyras and Moore2018b). Probiotics play a critical role in maintaining intestinal homeostasis and restoring bacterial eubiosis in infected birds (Gagliardi et al., Reference Gagliardi, Totino, Cacciotti, Iebba, Neroni, Bonfiglio, Trancassini, Passariello, Pantanella and Schippa2018). During the course of NE, it has been shown that probiotics can be used to restore the composition of the gut microbiome and to ameliorate intestinal inflammation caused by CP (Lin et al., Reference Lin, Xu, Zeng, Ni, Zhou, Zeng, Wang, Zhou, Zhu and Pan2017; Qing et al., Reference Qing, Zeng, Wang, Ni, Liu, Lai, Khalique, Pan and Jing2017). Lin et al. (Reference Lin, Xu, Zeng, Ni, Zhou, Zeng, Wang, Zhou, Zhu and Pan2017) demonstrated that manipulation of the gut microbiota of chickens by probiotics such as B. licheniformis alleviates disturbances caused by CP on the cecal microbial community of chickens. In another study, CP infection significantly decreased diversity indices of ileal microbiota composition (ACE and Chao 1) by increasing the relative abundance of Gammaproteobacteria and decreasing the relative abundance of the phylum Firmicutes (Li et al., Reference Li, Wang, Liu and Guo2017b). On the other hand, L. acidophilus treatment increased the diversity index of the ileal microbiota by enriching the members of phylum Firmicutes and reducing the relative abundance of phylum Proteobacteria. The authors suggested that L. acidophilus treatment modulates the relative abundance of certain bacterial species and restores the ileal microbiome disrupted by CP infection.
NE vaccines
Efforts have been made to develop an efficacious vaccine that can provide effective protection against NE. Since NE outbreaks may start as early as 2–3 weeks of age, early vaccination is of utmost importance for prevention of the disease. NE vaccines can be developed as live-attenuated vaccines, whole inactivated vaccines, subunit vaccines, toxoids, or recombinant vectored vaccines (Thompson et al., Reference Thompson, Parreira, Kulkarni and Prescott2006; Zhang et al., Reference Zhang, Wang, Wang, Ma and Wang2017b). Toxoid vaccines containing inactivated CP toxins, such as α-toxin, NetB and TpeL could induce toxin-neutralizing antibodies, preventing damage to the gut mucosa (Keyburn et al., Reference Keyburn, Portela, Ford, Bannam, Yan, Rood and Moore2013a). While a subunit vaccine comprises a pathogen-specific immunogenic antigen and an appropriate adjuvant to enhance its immunogenicity, a recombinant vector vaccine is made of a microbial vector carrying the antigen-encoding DNA sequences (Kulkarni et al., Reference Kulkarni, Parreira, Sharif and Prescott2008; Hegazy and Hensel, Reference Hegazy and Hensel2012). However, when making a recombinant or subunit vaccine, extra care should be taken to select the most immunogenic antigen that can induce a robust immune response. Therefore, molecular pathogenesis studies are necessary to identify the role of each antigen/toxin in pathogenesis as well as host responses to the infection. Other than α-toxin, NetB or TpeL, studies have shown that pathogenic CP have other immunogenic, protective antigens (Jiang et al., Reference Jiang, Kulkarni, Parreira and Prescott2009), which once identified could be used for developing an effective NE vaccine. NE vaccine studies are summarized in Table 1.
Table 1. Overview of NE vaccines

a SC: Subcutaneous.
b GPD: Glyceraldehyde-3-phosphate dehydrogenase.
c PFOR: Pyruvate: ferrodoxin oxidoreductase.
d FBA: Fructose 1,6-bisphosphate aldolase.
e HP: Hypothetical protein.
f IM: Intramuscular.
g Endo-beta-N-acetylglucosaminidase.
h Phosphoglyceromutase.
i Metallopeptidase.
Live-attenuated vaccines
Thompson et al. (Reference Thompson, Parreira, Kulkarni and Prescott2006) have demonstrated differences in the ability of live-attenuated vaccines to protect against NE. Vaccinating chickens with a live virulent strain of CP protected against subsequent infection with a homologous strain, with a significant reduction in intestinal lesions, but vaccination with an avirulent CP strain did not protect against challenge with a virulent CP strain. In another study, two out of four attenuated α-toxin-negative mutants of a virulent CP strain conferred protection against experimental challenge, suggesting involvement of other immunogens (Thompson et al., Reference Thompson, Parreira, Kulkarni and Prescott2006). The variability in protection among different CP strains might suggest the existence of differences in antigenic composition and or genetic variation of the chromosome and plasmid content of CP strains (Lacey et al., Reference Lacey, Allnutt, Vezina, Van, Stent, Han, Rood, Wade, Keyburn and Seemann2018a). Therefore, caution should be exercised in selection of CP strains for developing a live-attenuated vaccine.
Toxoid vaccines
For production of protein-based vaccines, the immunogenic protein components of CP have been investigated for NE vaccine development using different approaches. For instance, some investigators have explored the potential of the CP-secreted toxins in culture supernatants against NE. Despite the simplicity of their production, care should be taken to ensure that toxoid vaccines are produced correctly, as inactivation by formalin may negatively affect their immunogenicity (Mot et al., Reference Mot, Timbermont, Delezie, Haesebrouck, Ducatelle and Van Immerseel2013). In an in vivo study, subcutaneous vaccination of broiler chickens with a formalin-inactivated NetB toxoid or NetB genetic toxoid (W262A; a domain of netB that plays a role in regulating the binding of NetB to the cell membrane) provides partial protection against experimental NE. The results showed that both vaccines resulted in a significant decrease in the intestinal lesion scores and increased antibody responses to NetB (da Costa et al., Reference da Costa, Mot, Bokori-Brown, Savva, Basak, Van Immerseel and Titball2013).
In fact, the efficacy of toxoid vaccines appears to be highly strain-dependent, as it has been demonstrated that only one out of eight toxoid vaccines provides full protection against NE in chickens (Lanckriet et al., Reference Lanckriet, Timbermont, Eeckhaut, Haesebrouck, Ducatelle and Van Immerseel2010). When evaluated against CP type A and type C in broiler chickens, toxoid vaccines resulted in a significant increase in CP type A- and C-specific antibodies associated with a significant decrease in intestinal lesions (Saleh et al., Reference Saleh, Nabil, Fathalla and Mosaad2010). However, despite their protective ability against NE, vaccinating the birds subcutaneously at 7 and 21 days of age, and challenging them at day 35 of age (which is 2–3 weeks after the onset of naturally occurring outbreaks), raises concerns about the feasibility of this vaccine in commercial poultry farms.
As NE mostly occurs in the early weeks of life, the role of passively transferred immunity against NE following vaccination of parent stocks has been investigated. In view of this, Lovland et al. demonstrated that vaccination of parent stock with a toxoid vaccine, prepared from CP types A and C, confers protection against both types of CP in their progeny chicks (Lovland et al., Reference Lovland, Kaldhusdal, Redhead, Skjerve and Lillehaug2004). Following vaccination of broiler breeder hens with CP type A and type C toxoids adjuvanted with aluminum hydroxide, CP α-toxin-specific IgY antibodies were detected in sera of breeder hens and their progeny chicks. These passively immunized progeny chicks showed a high-level resistance against subsequent natural infection with both types of CP. In a NE disease model, CP type C induced a better protection, demonstrated by reduced intestinal and hepatic lesions (Lovland et al., Reference Lovland, Kaldhusdal, Redhead, Skjerve and Lillehaug2004). Despite their promising role in control of subclinical NE, the protective effects of these vaccines against the clinical form of NE were not evaluated in this study.
Subunit vaccines (toxin/other protein-based)
In another approach, genes encoding immunogenic proteins, such as toxins, can be cloned into plasmids that are then expressed in mammalian, bacteria, or insect cells, followed by identification and purification of the expressed proteins to produce a subunit protein-based vaccines (Nascimento and Leite, Reference Nascimento and Leite2012). In the past, due to a lack of adequate understanding of the immunogenicity profiles of various protein targets in CP, attention was given to α-toxin as a potential vaccine candidate for NE (Kulkarni et al., Reference Kulkarni, Parreira, Sharif and Prescott2007). The immunogenicity and protective efficacy of recombinant α-toxin-based subunit vaccines have been extensively evaluated in NE challenge models in broiler chickens. Despite efforts to unveil the potential role of α-toxin as a vaccine antigen, there is still controversy regarding its effectiveness in inducing protection against NE in chickens. For instance, subcutaneous immunization of broiler chickens with a recombinant α-toxin-based subunit vaccine at 5 days of age, followed by a booster dose at 15 days of age, conferred a partial protection against experimental CP infection at 25 days of age, as assessed by α-toxin serum IgY levels and gut lesion scores (Cooper et al., Reference Cooper, Trinh and Songer2009). In another study, priming the birds with CP α-toxin toxoid at 7 and 14 days of age followed by vaccination with an active α-toxin at 21 days of age resulted in a higher level of protection than did either one alone (Kulkarni et al., Reference Kulkarni, Parreira, Sharif and Prescott2007). Attempts have been made to enhance the protective efficacy of α-toxin vaccines. For example, a previous study by Kulkarni et al. (Reference Kulkarni, Parreira, Sharif and Prescott2007) evaluated the effectiveness of a single or various combination of five different recombinant proteins including α-toxin, GPD, PFOR, FBA, and a HP, against oral virulent CP challenge in broiler chickens. While vaccination with each of these proteins resulted in a significant increase in serum IgY and protection against a mild challenge with CP, a combination of α-toxin, HP and PFOR offered better protection against more severe challenge as compared to other combinations.
Following the discovery of NetB as a major virulence factor in the pathogenesis of CP (Keyburn et al., Reference Keyburn, Boyce, Vaz, Bannam, Ford, Parker, Di Rubbo, Rood and Moore2008), a significant amount of research was directed toward the use of NetB as a vaccine. Savva et al. designed different recombinant netB mutants and evaluated their toxicity, as compared to the pure NetB toxin, on a chicken hepatocellular carcinoma epithelial cell line (LMH). As the mutated proteins did not exhibit toxic effects on the cells, the authors hypothesized that these mutants could be used for NE vaccine development (Savva et al., Reference Savva, da Costa, Bokori-Brown, Naylor, Cole, Moss, Titball and Basak2013).
Comparative evaluation of effectiveness of various clostridial immunogenic proteins, including α-toxin, NetB toxin, PFOR, and elongation factor-Tu (EF-Tu) adjuvanted with ISA 71 VG, against experimental NE challenge in broiler chickens, revealed that all of them afford comparable levels of protection against NE (Jang et al., Reference Jang, Lillehoj, Lee, Lee, Lillehoj, Hong, An, Jeong, Chun and Bertrand2012). However, among these vaccines, a better weight gain was observed in chickens vaccinated with NetB toxin and PFO recombinant proteins. In another study, Keyburn et al. (Reference Keyburn, Portela, Sproat, Ford, Bannam, Yan, Rood and Moore2013b) evaluated the efficacy of purified NetB recombinant toxin (rNetB) alone or in combination with a formalin inactivated bacterin or a cell free CP supernatant toxoid against different challenge levels of virulent CP. The results showed that rNetB alone protects the birds against a mild challenge, while significant protection against moderate or heavy CP challenge was observed when rNetB was combined with either cell-free CP toxoid or bacterin. Similar to what has been observed for α-toxin (Cooper et al., Reference Cooper, Trinh and Songer2009), these results indicate that, although known as the major CP toxin, NetB alone does not induce an efficient, protective immune response against NE and a combination with other immunogens is required to potentiate its effect (Keyburn et al., Reference Keyburn, Portela, Ford, Bannam, Yan, Rood and Moore2013a). Recently, Escherichia coli BL21 strain was used to express and purify a recombinant metallopeptidase (a CP virulence factor) to make an injectable vaccine against NE, which was shown to be protective in a CP challenge model (Katalani et al., Reference Katalani, Ahmadian, Nematzadeh, Amani, Ehsani, Razmyar and Kiani2020). Further, tobacco plants were used to make an edible NE vaccine through the expression of a fusion protein containing NetB, α-toxin and metallopeptidase (Katalani et al., Reference Katalani, Ahmadian, Nematzadeh, Amani, Ehsani, Razmyar and Kiani2020). Using this vaccine resulted in serum antibody response and partial protection in the CP-challenge model.
Taken together, these findings point to the variability in the immunogenicity and protective potential of CP antigens. Indeed, none of the studied CP antigens has shown ability to provide complete protection against severe CP challenge when administered alone. Nonetheless, a multivalent subunit CP vaccine consisting of hybrid antigens could conceivably result in a higher level of protection. Another point of consideration is that any parenteral route used for administration of inactivated, toxoid or recombinant vaccines will be less than ideal for mass administration on poultry farms. A more practical route and time of vaccination that results in an acceptable level of protection by 2–3 weeks of age is desirable.
Live vectored vaccines
Attenuated Salmonella enterica strains have been extensively utilized as vaccine vectors for genes encoding various CP toxins (Hegazy and Hensel, Reference Hegazy and Hensel2012). Although attenuation of Salmonella strains is critical to prevent the adverse effects of this bacterium on the host, care should be taken to avoid over attenuation as it may reduce the effectiveness of the vaccine. Moreover, the vector should be attenuated by two mutations to ensure it does not revert to virulence (Hegazy and Hensel, Reference Hegazy and Hensel2012). In a study by Zekarias et al., oral administration of a recombinant attenuated S. enterica serovar Typhimurium vaccine (RASV), expressing the c-terminal part of α-toxin, followed by a parenteral boost vaccination with a recombinant PlcC protein (rPlcC), induced significantly high levels of α-toxin-neutralizing serum antibodies and serum IgG and bile IgA titers associated with a reduction in CP colonization and enteric pathology in chickens (Zekarias et al., Reference Zekarias, Mo and Curtiss2008). In another study, oral immunization of broiler chickens with a recombinant Salmonella vaccine expressing either a gene encoding FBA or HP has been shown to provide protection against NE challenge, and both were associated with higher serum and mucosal antibody responses. However, no such effects were observed for a PFOR vaccine (Kulkarni et al., Reference Kulkarni, Parreira, Sharif and Prescott2008). Following the identification of the B-cell epitopes of HP and α-toxin, the efficacy of an attenuated recombinant Salmonella vaccine expressing truncated HP (tHP) as well as α-toxin toxoid on NE was investigated in broiler chickens (Kulkarni et al., Reference Kulkarni, Parreira, Jiang and Prescott2010). Chickens vaccinated with α-toxoid were significantly protected against moderate challenge, while vaccination with tHR provided protection against both moderate and severe challenges. Further studies were conducted to assess and compare the effects of oral vaccination of broiler chickens with a RASV expressing genes encoding α-toxin and NetB (Jiang et al., Reference Jiang, Kulkarni, Parreira and Prescott2009). While vaccination with constructs expressing either toxin alone did not confer protection, concurrent vaccination with the two vaccines or vaccination with a vector expressing the two toxins resulted in protection against moderate to heavy challenges with CP. Additionally, in a more recent study, vaccination with the RASV system expressing either FBA (an enzyme known to be important for CP virulence) alone or a mixture of FBA, α-toxin, and NetB toxin provided better protection against NE than using either α-toxin or NetB toxin alone (Wilde et al., Reference Wilde, Jiang, Tafoya, Horsman, Yousif, Vazquez and Roland2019). In addition to using Salmonella strains as vaccine vectors, Bacillus subtilis and Lactococcus lactis bacteria have also shown considerable promise as potential vaccine vectors when used in mice against clostridial infections (Robinson et al., Reference Robinson, Chamberlain, Schofield, Wells and Le Page1997; Hoang et al., Reference Hoang, Hong, Clark, Titball and Cutting2008). The advantage of using Bacillus and lactic acid bacteria over Salmonella is that, in addition to being non-pathogenic, they can confer other benefits such as immunomodulatory effects (Sugiarto and Yu, Reference Sugiarto and Yu2004; Rhayat et al., Reference Rhayat, Maresca, Nicoletti, Perrier, Brinch, Christian, Devillard and Eckhardt2019), which could add an additional layer of protection against NE. Thus, experimental studies are needed to explore the possibility of using these vectors as vaccine carriers for CP antigens in chickens.
Route and time of vaccination
One of the most important points that needs to be taken into consideration when developing poultry vaccines is their suitability for mass application. Despite their considerable success in limiting NE in chickens, one of the shortcomings of toxoid vaccines is that they must be parenterally administered (Kulkarni et al., Reference Kulkarni, Parreira, Sharif and Prescott2007; Cooper et al., Reference Cooper, Trinh and Songer2009). On the other hand, in addition to their role in inducing local mucosal immune responses following oral administration, attenuated vector vaccines are suitable for mass immunization (Kulkarni et al., Reference Kulkarni, Parreira, Sharif and Prescott2008). Another important point is that the immune responses to vaccination should reach a protective level before 2–3 weeks of age, the time at which chickens are more vulnerable to NE. All these vaccines have shown comparable levels of protection, however, the delay in vaccination (1- or 2-week-old birds) together with the need for booster doses raises concern about the feasibility of these vaccines. The poultry industry is, therefore, seeking a vaccine which could be effective with just a single vaccination at a very early age, but attempts to immunize day-old broiler chickens have not been successful (Mot et al., Reference Mot, Timbermont, Delezie, Haesebrouck, Ducatelle and Van Immerseel2013). Another promising strategy is vaccination of parent stock. The idea is that if the breeder flocks are vaccinated, the maternally derived antibodies will be vertically transferred to their progeny chicks, thereby providing protection against NE in their early life. Keyburn et al., demonstrated that vaccination of broiler breeder hens with purified recombinant NetB toxoid at 22, 24 and 26 weeks of age induced the production of significant levels of specific NetB-IgY antibodies in hens and egg yolks of the fertile eggs 4 weeks after the last vaccination and resulted in partial protection against NE in broilers at 14 and 21 days of age (Keyburn et al., Reference Keyburn, Portela, Ford, Bannam, Yan, Rood and Moore2013a). It is, however, important to note that one of the limitations of parent stock vaccination is the gradual reduction of antibody titers in eggs, which are laid later in their production period together with the gradual decrease of the passive immunity in chickens as they age, which may indeed influence chicken resistance to late NE outbreaks. Therefore, more work is required to ascertain the effectiveness of this passive immunity transfer through breeder vaccination.
Conclusions
NE is a complex disease that is caused by an imbalance in the intricate relationship between CP and its host. Further understanding of the pathogenicity of CP and its interaction with the host immune system will allow the development of effective prevention and control interventions for NE. Optimization of nutritional and farm management practices in addition to the application of probiotics and new generation vaccines should be pursued as preventive strategies to control NE in broiler farms.
Acknowledgements
The authors acknowledge the support provided by the Canadian Poultry Research Council, Agriculture and Agri-Food Canada, and Ontario Ministry of Agriculture, Food and Rural Affairs.