Introduction
Parasitism plays an important role in population dynamics of small mammals (Krebs, Reference Krebs2011). This kind of interaction can affect host population sizes by potentially reducing survival, reproduction and individual movements, and can even generate population fluctuations (Tompkins and Begon, Reference Tompkins and Begon1999). This is specifically important for small populations as observed in patched or fragmented habitats, which are potentially more prone to local extinction (e.g. Macdonald, Reference Macdonald1996; Allan et al., Reference Allan, Keesing and Ostfeld2003). On wild populations, the role of parasites as significant drivers of population-level effects on hosts and which factors influence the capacity of a parasite to cause damage to their hosts is still unclear (Watson, Reference Watson2013). Moreover, parasitism is usually a neglected interaction in small mammal studies because of the difficulty in evaluating the multitude of disease effects on a population, since small mammals are generally hosts for many pathogens or parasites (e.g. Püttker et al., Reference Püttker, Meyer-Lucht and Sommer2008; Linardi, Reference Linardi and Cáceres2012).
Cuterebrid botflies are a common group of mammal parasites in the New World (Slansky, Reference Slansky2007). They cause myiasis, which is characterized by the formation of a large skin furuncle or warble containing a larva inside (Catts, Reference Catts1982; Colwell, Reference Colwell, Samuel, Pybus and Kocan2001; Slansky, Reference Slansky2007). Their larvae feed on sera and white blood cells at subdermal sites of the host, obtaining sufficient nutrients for a fast growth and development to the adult phase (Catts, Reference Catts1982; Colwell, Reference Colwell, Samuel, Pybus and Kocan2001). The adults, in their turn, do not interact with their hosts, since they do not oviposit on the hosts, but on substrate at sites frequented by the potential hosts (Catts, Reference Catts1982). Cuterebrid botflies tend to be highly host-specific and possibly because of the parasite–host coevolution, they are considered to have little or no deleterious effect on fitness of their coevolved hosts (Catts, Reference Catts1982; Slansky, Reference Slansky2007). However, their oviposition behaviour makes parasitism on non-coevolved hosts possible, leading to potential negative effects on these hosts, such as infection, prolonged healing of post-exit warbles, reduced survival and reproduction, and even death (Catts, Reference Catts1982; Slansky, Reference Slansky2007).
Some studies demonstrated that cuterebrid infestation depressed survival and reproduction (e.g. Sealander, Reference Sealander1961; Boonstra et al., Reference Boonstra, Krebs and Beacham1980; Nichols, Reference Nichols1994), others found no effect (e.g. Bergallo et al., Reference Bergallo, Martins-Hatano, Juca and Gettinger2000; Spessot et al., Reference Spessot, Gomez and Priotto2013) and still others found beneficial effects on hosts (Goertz, Reference Goertz1966; Hunter et al., Reference Hunter, Sadleir and Webster1972; Clark and Kaufman, Reference Clark and Kaufman1990; Munger and Karasov, Reference Munger and Karasov1991; Jaffe et al., Reference Jaffe, Zegers, Steele and Merritt2005; Cramer and Cameron, Reference Cramer and Cameron2006). Studies that found these positive effects suggest that they may be an artefact as parasitized individuals could have reduced movements and then reduced chances of emigration (Wecker, Reference Wecker1962), or just that individuals that live longer also have a longer exposure to the botfly and consequently a greater probability of being infested (Hunter et al., Reference Hunter, Sadleir and Webster1972). Moreover, there is evidence for opposite effects between survival and reproduction in some cases: even when parasitized individuals have higher survival rates, reproduction can be negatively affected through decrease in activity or success, contributing to the decline in population growth rates in years of high prevalence (Wecker, Reference Wecker1962; Burns et al., Reference Burns, Goodwin and Ostfeld2005).
We investigated the effects of botfly parasitism on a neotropical marsupial – the gracile mouse opossum Gracilinanus agilis – in a highly seasonal environment (Brazilian Cerrado), where marked dry and wet seasons occur every year. We examined the potential cost of these parasites on host condition in a general effect, i.e. body condition, and in a specific effect through haemoglobin concentration. We predicted that both body condition and haemoglobin concentration would be lower in parasitized animals. Furthermore, to better understand the underlying factors influencing health condition, we evaluated potential effects of several factors that could interact with our main variables of interest, namely sex, food supplementation, season, daily climatic variables and time in livetraps. We predicted: (1) that females would have lower body condition and haemoglobin concentration than males, (2) that the difference in the botfly effect on individuals’ health would be less pronounced in the food-supplemented areas, (3) that animals would have lower body condition and higher haemoglobin concentration in the dry season than in the wet season. We expected that females would have lower values of body condition than males due to intrinsic differences in the body mass to head–body length relationship between sexes, and lower values of haemoglobin concentration than males as observed in other small didelphid sexually dimorphic in size, the gray short-tailed opossum Monodelphis domestica (Evans et al., Reference Evans, Hewett, Clayton, Krubitzer and Griffey2010). We expected the difference in the botfly effect on individuals’ health would be less pronounced in the manipulated areas because of the increase in resource availability comparing to the control areas. Also because of differences in resource availability, we expected that the body condition would be lower in the dry season than in the wet season. Moreover, haemoglobin concentration would be higher during the former mainly due to dehydration. We also tested for differences between the seasons directly using daily climatic variables.
Materials and methods
Natural history
Gracilinanus agilis (Burmeister 1854) is a nocturnal, solitary and scansorial mouse opossum of the family Didelphidae. It inhabits mainly forested areas in central Brazil, eastern Peru, eastern Bolivia, Paraguay, Uruguay and northern Argentina (Creighton and Gardner, Reference Creighton, Gardner and Gardner2007). Its diet is composed of fruits, invertebrates and small vertebrates (Bocchiglieri et al., Reference Bocchiglieri, Mendonça and Campos2010; Camargo et al., Reference Camargo, Ribeiro, Camargo and Vieira2014). It is sexually dimorphic in size (females = 13–25 g, males = 15–40 g; Costa et al., Reference Costa, Leite and Patton2003) and has a synchronized reproduction from the end of the dry season (August–September) until the adult population reduction (December–January) (Aragona and Marinho-Filho, Reference Aragona and Marinho-Filho2009; Andreazzi et al., Reference Andreazzi, Rademaker, Gentile, Herrera, Jansen and D'Andrea2011), as observed in the study area (Mendonça et al., Reference Mendonça, Armond, Camargo, Camargo, Ribeiro, Zangrandi and Vieira2015). Populations of this species may have semelparous (Lopes and Leiner, Reference Lopes and Leiner2015; Puida and Paglia, Reference Puida and Paglia2015) or partially semelparous (Martins et al., Reference Martins, Bonato, Da-Silva and Reis2006) reproductive strategies.
The botfly Cuterebra apicalis occurs from Mexico to Argentina (Twigg, Reference Twigg1965; Papavero and Guimarães, Reference Papavero and Guimarães2009), and parasitizes the gracile mouse opossum (Pujol-Luz et al., Reference Pujol-Luz, Mendonça and Henriques2004; Cansi, Reference Cansi2011a) and at least 20 other mammal species, including 17 native and three introduced ones (the Norway rat Rattus norvegicus, the black rat R. rattus and the domestic dog Canis lupus familiaris) (Forattini and Lenko, Reference Forattini and Lenko1959; Twigg, Reference Twigg1965; Everard and Aitken, Reference Everard and Aitken1972; Led et al., Reference Led, Colacelli, Boero and Colombo1976; Mello, Reference Mello1978; Leite and Williams, Reference Leite and Williams1988; Bossi and Bergallo, Reference Bossi and Bergallo1992; Vieira, Reference Vieira1993; Guimarães and Papavero, Reference Guimarães and Papavero1999; Pinto and Claps, Reference Pinto and Claps2005; Cansi, Reference Cansi2011b; a complete list of C. apicalis hosts is in Supplementary Material Table S1). The period of development within a typical host (Cerradomys subflavus) is 21–26 days (Leite and Williams, Reference Leite and Williams1988).
Study area
We collected field data in four patches of cerradão (savannah woodland) in central Brazil. The cerradão is a xeromorphic forest-like physiognomy of the Cerrado, with a canopy height varying from 8 to 12 m, and a canopy cover ranging from 50 to 90% (Oliveira-Filho et al., Reference Oliveira-Filho, Ratter, Flora, Oliveira and Marquis2002). The climate is tropical savannah (Aw: Köppen-Geiger classification; Kottek et al., Reference Kottek, Grieser, Beck, Rudolf, Rubel, Centre and Wetterdienst2006), with the dry season between May and September and the wet season between October and April (Eiten, Reference Eiten1972). Three sites (JB1 – 23.83R ha, JB2 – 27.33 ha, JB4 – 3.32 ha) were located at the Botanical Garden of Brasília (15°52′S, 47°50′W) and one site (FAL – 7.53 ha) at the Ecological and Agricultural Field Station of the University of Brasília (15°58′S, 47°59′W), located approximately 25 km SW of Brasília, Federal District, Brazil. A food supplementation experiment was carried out in two (JB2 and JB4) of our four grids. In each of these grids we provided milled cat food every 2–3 weeks through feeders evenly distributed in the understory as well as in an additional buffer (see Mendonça et al., Reference Mendonça, Armond, Camargo, Zangrandi and Vieira2017 for details).
Data collection
We set four 1.44 ha trapping grids (120 m × 120 m), each comprising 81 capture stations 15 m apart. Each capture station had one Sherman live trap on the ground and one on a tree branch (1.5–2.0 m), but only the trap placed in the understory had a timer. Traps were baited with a mixture of banana, peanut butter, maize flour, cod liver oil and vanilla essence. We trapped each grid for eight six-night capture sessions from April 2015 to December 2016. Opossums were marked with tags in both ears to avoid losing identification (model 1005-1; National Band and Tag, Newport, Kentucky). We recorded the individual number, species, sex and botfly occurrence for each capture, but body mass (to the nearest 0.1 g) and head–body length (to the nearest mm) for only the first capture of each session.
We considered the individual parasitized if the presence of a botfly larvae was confirmed or if it had a characteristic scar tissue indicating recent larvae emergence. Most cuterebrids occurred singly in the abdomen or on the back. We usually removed and weighed (to the nearest 0.1 g) the mature larvae. These were maintained in vials covered with gauze and containing moist sawdust until the flies emerged. All individuals that emerged were identified as C. apicalis, and this validated previous research (Pujol-Luz et al., Reference Pujol-Luz, Mendonça and Henriques2004; Cansi, Reference Cansi2011a).
We collected the blood sample from the individuals only once in each capture session by submandibular bleeding using an insulin (8 mm × 0.3 mm; 30 G) needle, dispensing the need for anaesthesia (Hoff, Reference Hoff2000; Golde et al., Reference Golde, Gollobin and Rodriguez2005). Haemoglobin concentration (g dL−1) was determined immediately with a portable haemoglobin analyser (Hemo Vet; EKF Diagnostics) with 5 µL of blood. Although haematocrit is the primary value for interpretation in the field of veterinary medicine, haemoglobin concentration is more accurate than haematocrit when both blood parameters are calculated by an automated counter (Thrall, Reference Thrall, Thrall, Weiser, Allison and Campbell2012).
We obtained daily climatic data (maximum temperature, minimum temperature, precipitation and relative humidity) from the meteorological station at the Roncador Ecological Reserve (RECOR/IBGE). The four grids were in a sufficiently short distance from one another (800 m–15.5 km) that we assumed the same climate affected all grids.
Data analysis
We considered only one record of each individual during the entire study period for the analysis, not necessarily the first record of each animal. Since we had more records of non-parasitized than parasitized individuals, and more parasitized in the wet season than in the dry season, we tried to keep groups more balanced giving preference to records of parasitized individuals in the dry season.
We evaluated body condition using the scaled mass index (SMI) method (Peig and Green, Reference Peig and Green2009, Reference Peig and Green2010). SMI is the body mass of an individual standardized to the mean body size of all individuals from the same body mass to head–body length relationship (Peig and Green, Reference Peig and Green2009, Reference Peig and Green2010). We calculated the final body mass subtracting the mass of the ear-tags (0.5 g) when the animals were already marked, and the weight of botfly larvae in cases when the animals were infected. We used an average larva weight (1.675 g) based on field data when we did not have the exact information. We were not concerned about number of larvae occurring in the same host for calculating the average weight since we observed a lower mass of each larva in cases of multiple infections, so that the total mass was similar to cases of infection by a single bot. We tested for between-sex differences in the linear regressions of log body mass against log head–body length, as G. agilis is sexually dimorphic in size, before deciding for the calculation of SMI based on all individuals together or separated by sex.
First, we used random forest analyses to evaluate the relative importance of different variables, choosing a subset based on the per cent increase in mean square error (MSE) to reduce the number of models for further selection. Random forest is an ensemble learning method that combines many decision trees by repeatedly resampling data with replacement, helping to avoid overfitting and accounting for collinearity among variables (Cutler et al., Reference Cutler, Edwards, Beard, Cutler, Hess, Gibson and Lawler2007). The random forests algorithm performs a random selection of features to split each node and differs from bootstrap aggregating, or bagging, which uses an ordinary bootstrap sample of the entire feature set (Breiman, Reference Breiman2001). The percentage increase in MSE reflects the importance of a variable because it is the error that would result for the out-of-bag data from the removal of the given variable (De'ath and Fabricius, Reference De'ath and Fabricius2000; Calle and Urrea, Reference Calle and Urrea2010).
We checked the response variables haemoglobin concentration (Hb) and body condition (SMI) for normality and homoscedasticity. The tested explanatory variables were parasitism by C. apicalis (Botfly), supplementation experiment (Suppl – control/manipulated areas), climatic season (Season – dry/wet), area (four grids), sex (females and males), time inside trap (Time – in minutes) and the climatic variables: maximum temperature (Max.Temp), minimum temperature (Min.Temp), precipitation (Precip) and relative humidity (Humidity). For the response variable body condition (SMI), the explanatory variable sex was not included in the random forest (but was in all models from the model selection) since the index was previously calculated separately for females and males. Spearman correlations were performed between the four daily climatic variables before model analyses. We considered only the pairs including daily maximum temperature together in the same model because all other pairs had a moderate-to-high correlation (>0.40).
We evaluated the plausibility of the candidate models based on the Akaike's information criterion corrected for small samples (AICc; Burnham and Anderson, Reference Burnham and Anderson2002). We used for comparisons the AICc difference between models and the one with the lowest value (ΔAICc), and Akaike weight (w), which reflects the relative evidence of fit of a model to the data, proportional to the candidate set of models (Burnham and Anderson, Reference Burnham and Anderson2002). We used model averaged estimates to calculate predicted values of the response variables and plot them against the variables from the best models. Analyses were run using ‘randomForest’ (Liaw and Wiener, Reference Liaw and Wiener2002), lme4 (Bates et al., Reference Bates, Maechler, Bolker and Walker2015) and MuMIn (Bartoń, Reference Bartoń2018) packages in R, version 3.4.2 (R Core Team, 2017).
Results
From April 2015 to December 2016, we obtained 2073 captures of 555 G. agilis individuals, and detected botfly larva on 169 individuals (30.5%). The highest prevalence rates (number of infected individuals/total number of individuals) were in April 2015 (31.5%) and December 2016 (30.3%), and the lowest in September of both years (0% in 2015 and 1.4% in 2016; Fig. 1). Considering all captures, in 87.6% of the cases the parasitized opossums had one larva, 8.6% had two, 3.2% three and 0.5% four. Only 16 individuals (9.5%) were parasitized more than once during this period.

Fig. 1. Prevalence of Cuterebra apicalis botflies in the marsupial Gracilinanus agilis during the haemoglobin study in four patches of cerradão from April 2015 to December 2016. Gray bars represent dry seasons.
Mean haemoglobin concentration (Hb) for the species was 14.0 ± 2.1 g dL−1, from a total of 405 records, 306 records of non-parasitized (mean Hb = 14.5 ± 1.7 g dL−1) and 99 records of parasitized individuals (mean Hb = 12.3 ± 2.1 g dL−1; Supplementary Material Table S2). Mean body condition (SMI) was 18.8 ± 3.5 g from 400 records, 304 records of non-parasitized (mean SMI = 18.7 ± 3.5 g) and 96 records of parasitized individuals (mean SMI = 19.1 ± 3.8 g). We removed two animals that had no value of head–body length, and three outliers were considered as wrong measures. We calculated SMI for females and males separately since model selection for body mass to head–body length relationship resulted in the model including interaction with sex accounting for an Akaike weight of 0.96 and the model without sex, only 0.04 (Table 1).
Table 1. Model selection of the linear models of natural log of body mass (Mi) against natural log of head–body length (Li) with and without the effect of sex

K is the number of parameters, AICc is the Akaike's information criterion corrected for small samples, ΔAICc is the difference of AICc value to the best model, w is the Akaike weight, LL is the log likelihood of the model.
The most important variable for determining Hb was botfly occurrence, followed by daily relative humidity and daily maximum temperature (Fig. 2). In contrast, parasitism was the least important variable for SMI, with a negative percentage increase in MSE, meaning error actually decreased when this variable was permuted. All four daily climatic variables were selected as the most important variables for SMI. Time inside trap was the last one in the Hb ranking and the second-last in SMI ranking. The supplementation of food was also of minor importance for both response variables.
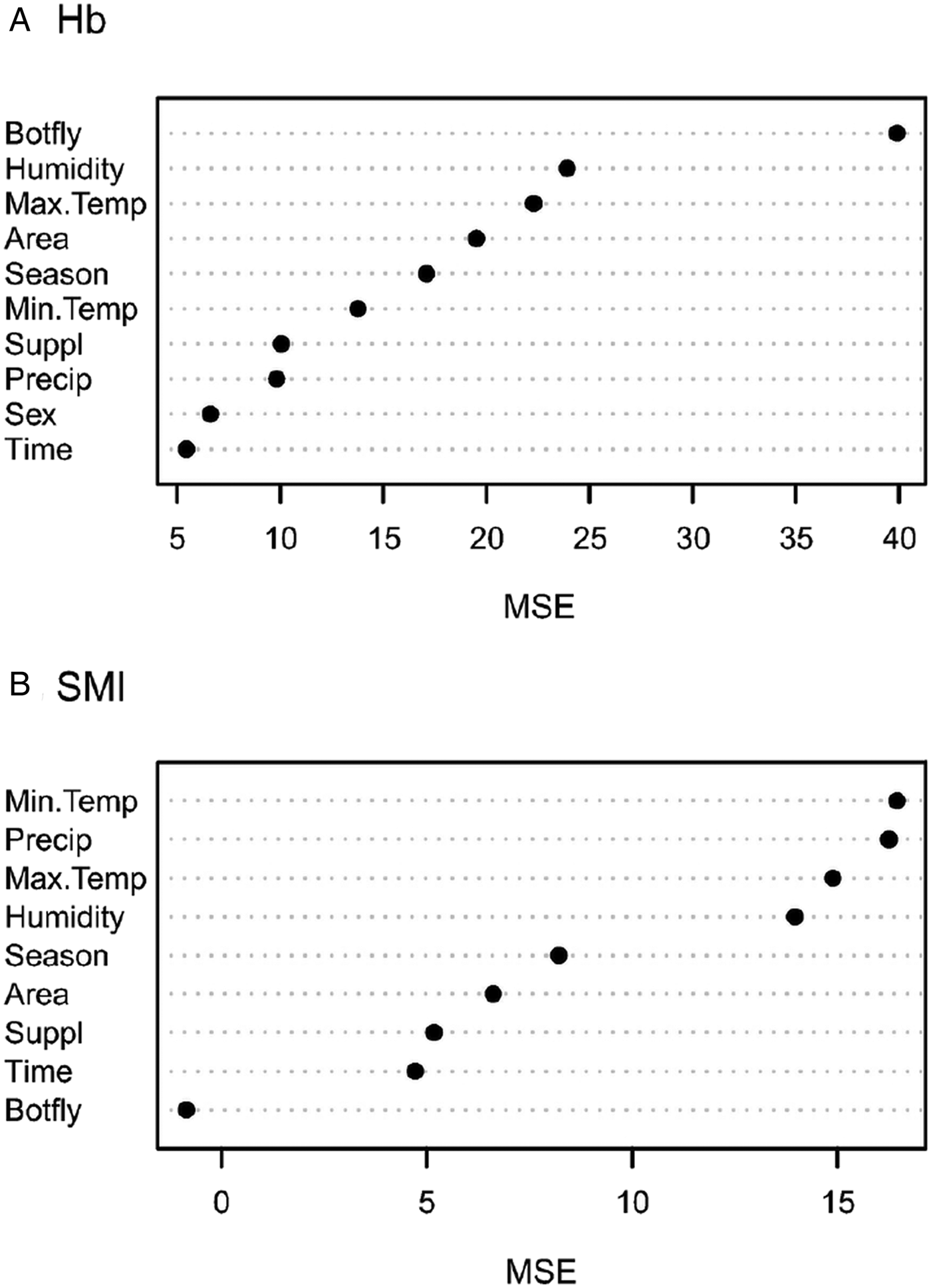
Fig. 2. Variable importance plot resulting from the random forest analyses of the effects on haemoglobin concentration (Hb) and body condition (SMI). Variables were ranked with regard to importance on the y-axis. MSE is the percentage increase in mean square error. The explanatory variables were parasitism by botflies (C. apicalis), food supplementation (Suppl – control/experiment), climatic season (Season – dry/wet), area (four grids), sex (females and males), time inside trap (Time – in minutes) and the daily climatic variables: maximum temperature (Max.Temp), minimum temperature (Min.Temp), precipitation (Precip) and relative humidity (Humidity).
Since parasitism was the most important variable for determining Hb and directly related to our main prediction, in the model selection we only built simple, additive and multiplicative models including this factor, totaling nine models (Table 2). The two first-ranked models had Hb varying with maximum temperature and relative humidity, both with interaction of parasitism and humidity, and either by additive or multiplicative combination of parasitism and maximum temperature. We selected only these two for model averaging because they summed a cumulative weight of 0.99.
Table 2. Model selection of the haemoglobin concentration of Gracilinanus agilis as a function of parasitism (Botfly), daily maximum temperature (Max.Temp) and daily relative humidity (Humid)
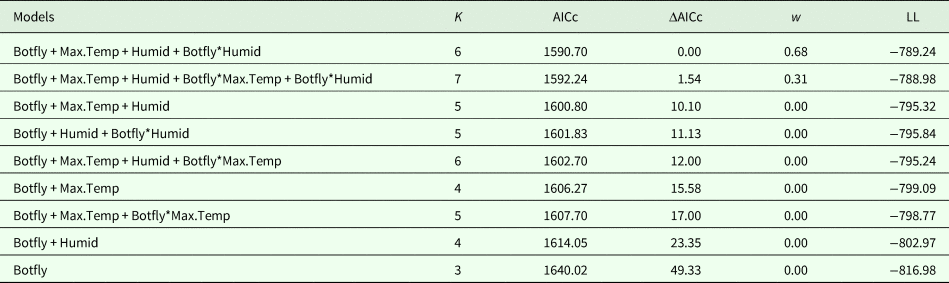
K is the number of parameters, AICc is the Akaike's information criterion corrected for small samples, ΔAICc is the difference of AICc value to the best model, w is the Akaike weight, LL is the loglikelihood of the model. The signals + and * indicate the additive and the multiplicative effects between variables.
Estimates of Hb were lower for parasitized than non-parasitized individuals trapped under same conditions (Fig. 3, Supplementary Material Table S3). Both groups had an increase in Hb with increasing maximum temperature. Haemoglobin decreased with increasing humidity among non-parasitized individuals, but increased with increasing humidity among parasitized individuals. Thus, Hb of parasitized individuals was closer to that of non-parasitized ones when humidity was high and differed from them when humidity was low.

Fig. 3. Estimates of haemoglobin concentration of Gracilinanus agilis (Hb; solid lines) and 95% confidence limits (dashed lines) obtained from model averaging the two first models of the candidate set and varying with daily maximum temperature (A) and relative humidity (B) for the non-parasitized (blue) and parasitized (orange) individuals. Daily relative humidity was maintained constant at its mean (69.8%) in (A) and daily maximum temperature was maintained constant at its mean (28.5°C) in (B).
We had a set of 11 candidate models for SMI model selection (Table 3). Since SMI was calculated separatedly for each sex, we had to account for this variable in all models of the model selection to treat the groups independently. We only joined maximum temperature with other climatic variables together in the same models. The two best models had SMI varying with maximum and minimum temperatures, and together had a cumulative weight of 0.80. Model averaged estimates from selected models showed a slight positive slope for the relationship between SMI and minimum temperature and negative relationship between SMI and maximum temperature (Fig. 4, Supplementary Material Table S4).
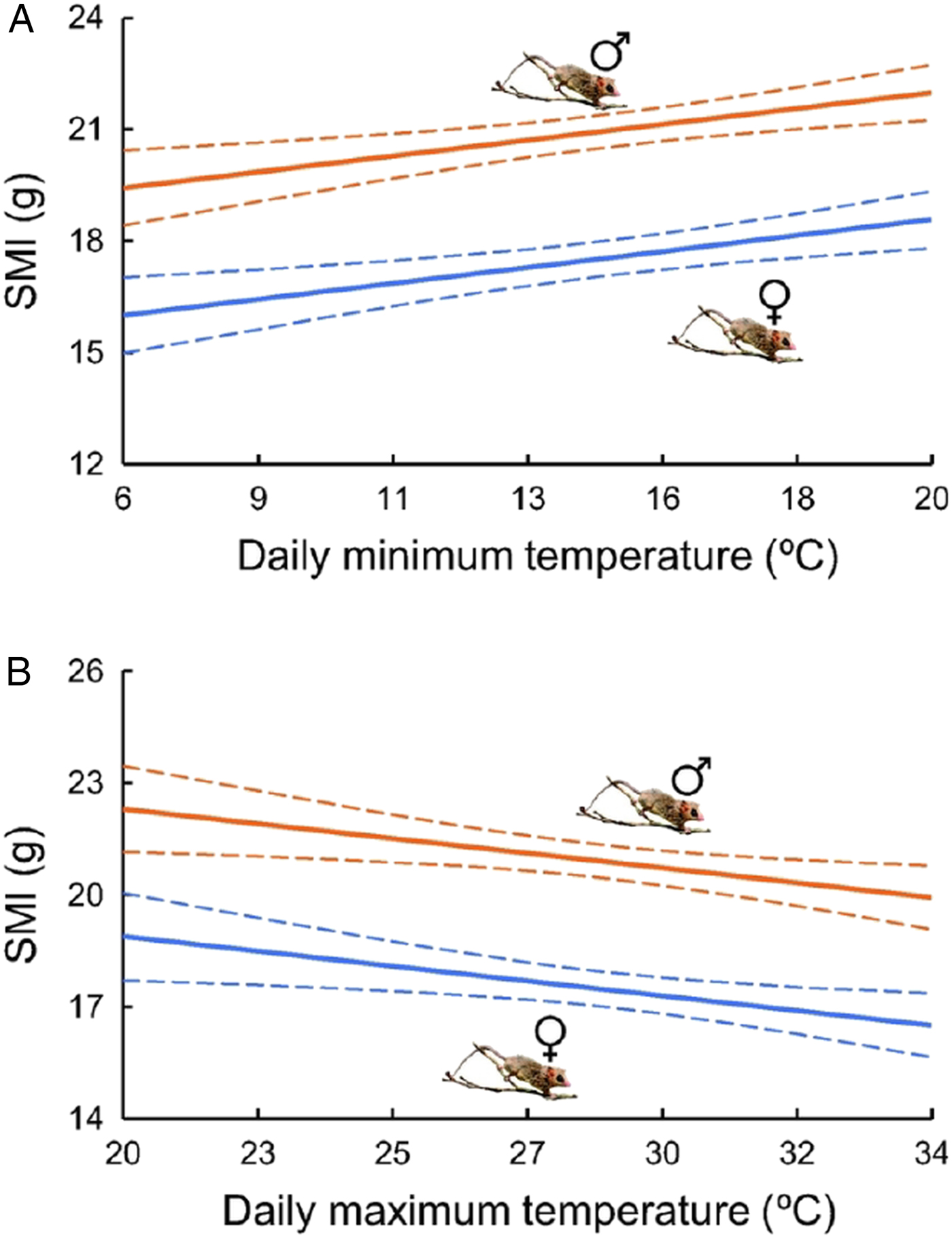
Fig. 4. Estimates of body condition indexes of Gracilinanus agilis (SMI; solid lines) and 95% confidence limits (dashed lines) obtained from model averaging the two first models of the candidate set and varying with daily minimum (A) and maximum temperatures (B) for males and females. Daily maximum temperature was maintained constant at its mean (28.5 °C) in (A) and daily minimum temperature was maintained constant at its mean (14.4 °C) in (B).
Table 3. Model selection of the body condition index of Gracilinanus agilis as a function of sex, daily maximum temperature (Max.Temp), daily minimum temperature (Min.Temp), daily relative humidity (Humid) and daily precipitation (Precip)

K is the number of parameters, AICc is the Akaike's information criterion corrected for small samples, ΔAICc is the difference of AICc value to the best model, w is the Akaike weight, LL is the log likelihood of the model. The signals + and * indicate the additive and the multiplicative effects between variables.
Discussion
Our study was the first one to investigate the effects of botfly parasitism on the health of a neotropical marsupial. Our main hypotheses and predictions were partially supported, that the haemoglobin concentrations were lower when G. agilis individuals were parasitized by the botfly C. apicalis. However, body condition did not differ between parasitized and non-parasitized animals.
Our results suggest that the presence of botfly larvae leads to a certain degree of anaemia and this has also been seen in previous studies with rodents (Sealander, Reference Sealander1961; Dunaway et al., Reference Dunaway, Payne, Lewis and Story1967; Bennett, Reference Bennett1973; Hunter and Webster, Reference Hunter and Webster1974). Anaemia is a considerable reduction in the red blood cells, caused by either abnormal loss or decreased production, and leads to a deficiency in oxygen transport (Campbell, Reference Campbell2015). Even though cuterebrid botflies feed on the host's interstitial fluid instead of its blood (Hunter and Webster, Reference Hunter and Webster1974), anaemia is probably a consequence of meaningful physiological stress (Nichols, Reference Nichols1994).
The model selection indicated daily climatic variables as important for explaining body condition and haemoglobin concentration. Among the non-parasitized individuals, predicted haemoglobin increased with the increase of the maximum temperature and the decrease of the relative humidity, a pattern found at the peak of the dry season. As expected, it appears that animals were dehydrated on the hottest and driest days. The evaporative water loss rate of G. agilis, rate at which the individual loses water through respiration, increases linearly with environmental temperature (Cooper et al., Reference Cooper, Withers and Cruz-Neto2009). During the dry seasons of 2015 and 2016, we commonly observed individuals with sunken eyes, and even with a gap between the eyeball and the surrounding tissue, which is a symptom of 8–12% of dehydration in mammals (Silverstein and Campbell, Reference Silverstein, Campbell, Tobias and Johnston2012; Donohoe, Reference Donohoe, Battaglia and Steele2016).
The responses of the parasitized individuals to the environmental variables differed from those of the non-parasitized ones. The relationship between Hb and daily maximum temperature was similar but with lower values for the parasitized animals. For daily relative humidity, however, we detected an opposite pattern: haemoglobin increased with increasing humidity. This pattern is counterintuitive because anaemia and dehydration alone show opposite effects, and we would expect anaemia being masked by dehydration, as observed in dogs and cats clinical cases (Lynch et al., Reference Lynch, Respess, Boll, Bozych, Mcmichael, Fletcher, De Laforcade and Rozanski2016). It seems there was a synergistic effect, in which the effect of botfly parasitism was much more pronounced in face of an additional stressor, dehydration. Botflies are estimated to be approximately 80% water (Smith, Reference Smith1977), so this uptake during larvae fast growth could be challenging for the hosts in times of water limitation. Interactions between the effect of botfly and an environmental stress were also observed regarding higher trap mortality of Peromyscus eremicus during cold nights in a desert climate (Nichols, Reference Nichols1994), and lower winter survival for a thermoregulation-deficient rodent (Akodon azarae; Zuleta and Vignau, Reference Zuleta and Vignau1990). Although temperatures have an effect on G. agilis activity (Vieira et al., Reference Vieira, Camargo, Colas, Ribeiro and Cruz-Neto2017), low nocturnal temperature was not a key environmental stressor in our study, probably because this marsupial faces less harsh minimum temperatures than those that occur in desert during night and also because it can enter in torpor.
Nevertheless, this synergistic effect between anaemia and dehydration would probably cause a minor effect on the host populations because the presence of botflies seems to injure more the hosts with the extra physiological stress in September, during the peak of the dry season, when prevalence reaches its lowest values. However, botfly parasitism has the potential to have a major effect on small mammal populations, especially the ones isolated in patches, in face of climate change towards more occurrence of days under drought conditions in central Brazil (Dai, Reference Dai2011; Bustamante et al., Reference Bustamante, Nardoto, Pinto, Resende, Takahashi and Vieira2012; Prudhomme et al., Reference Prudhomme, Giuntoli, Robinson, Clark, Arnell, Dankers, Fekete, Franssen, Gerten, Gosling, Hagemann, Hannah, Kim, Masaki, Satoh, Stacke, Wada and Wisser2014).
We did not find between-sex differences in haemoglobin concentration of G. agilis. For other small didelphid (the short-tailed opossum M. domestica), however, an opposite pattern was reported, in which haemoglobin concentration of males was 8% greater than females (Evans et al., Reference Evans, Hewett, Clayton, Krubitzer and Griffey2010). Mean Hb of G. agilis was close to the overall values reported for M. domestica (13.0 g dL−1, range 12.2–13.8 g dL−1), but lower than those reported for dasyurid marsupials (Australian mouse-like marsupials) of similar size (Clark, Reference Clark2004).
Our results indicated that potential deleterious effects of botfly parasitism on marsupial host are not apparent through evaluation of body condition. This parameter is assumed to be an indicator of health since it would reflect body fat reserves or lean muscle mass of the individuals. There is still much controversy regarding the validity of those assumptions (Schulte-Hostedde et al., Reference Schulte-Hostedde, Millar and Hickling2001; Wilder et al., Reference Wilder, Raubenheimer and Simpson2016) and which of the many available indices must be used (Jakob et al., Reference Jakob, Marshall and Uetz1996; Peig and Green, Reference Peig and Green2009, Reference Peig and Green2010; Labocha et al., Reference Labocha, Schutz and Hayes2014). Body condition, however, is still widely used in ecological studies for its practicality and for being a non-invasive approach.
Although some studies investigating the effects of cuterebrid botflies recorded body mass loss (Dunaway et al., Reference Dunaway, Payne, Lewis and Story1967; Smith, Reference Smith1978), others found that individuals had the same or a better condition when parasitized (Bergallo et al., Reference Bergallo, Martins-Hatano, Juca and Gettinger2000; Cramer and Cameron, Reference Cramer and Cameron2006) or a faster growth in smaller animals (Boonstra et al., Reference Boonstra, Krebs and Beacham1980). In laboratory experiments, the host changes from losing weight to increasing food intake during the period of infestation to compensate for the food reserves drainage (Hunter and Webster, Reference Hunter and Webster1974). This shift occurs at the time the botfly larva needs to grow rapidly to a large size (many thousand-fold), and it continues to cause protein deficiency and to alter the host blood pattern (Hunter and Webster, Reference Hunter and Webster1974). Therefore, unless there is no food limitation in the field, wild individuals would show the same pattern of change in weight of their counterparts in the laboratory.
Most of the records of parasitism by C. apicalis in our study area occurred with gracile mouse opossum, which is an indication of high specificity of the botfly. The position of the larvae, however, varied in their body. For the 165 individuals whose information about the position of larvae was recorded, in 86.3% of cases the larvae were located in an abdominal position at the host body and only 1.4% of cases in an inguinal position. The remainder were lateral, dorsal, pectoral and even in the region of the throat. Warble site specificity is characteristic of botflies in their native hosts (but appears less defined in secondary hosts; Hunter and Webster, Reference Hunter and Webster1973; Boonstra et al., Reference Boonstra, Krebs and Beacham1980; Slansky, Reference Slansky2007). For G. agilis, even considering that in most cases the warbles were located on the abdominal position, it is not clear if the marsupial can be considered a typical host for C. apicalis.
The variables daily minimum and maximum temperatures, although selected for being related with body condition of G. agilis, are unlikely to represent a major effect for two reasons. Firstly, those climatic variables had low values of the percentage increase in MSE. Secondly, the predicted body condition indices from the model averaging had a small range of variation in relation to both temperature amplitudes. The possible explanation for the slight negative relationship between maximum temperature and body condition is the same as mentioned previously regarding Hb, related to the increase in water loss through respiration with increasing temperatures. Moreover, the positive relationship between minimum temperature and body condition could be explained by the increase in respiratory frequency and oxygen consumption with the decrease in temperature (Cooper et al., Reference Cooper, Withers and Cruz-Neto2009). These relationships, however, do not occur in torpid animals. During torpor, body temperature can drop to 14.6 °C (at 12 °C), which results in absolute energy and water savings (Cooper et al., Reference Cooper, Withers and Cruz-Neto2009).
Contrary to our predictions, the relevance of the variable food supplementation was very low, both for haemoglobin concentration and body condition. We expected that the areas with artificial increase in food resource would have animals with better body condition, as observed in other studies with small mammals (Boutin, Reference Boutin1990; Prevedello et al., Reference Prevedello, Dickman, Vieira and Vieira2013). We even predicted that the individuals from areas subject to food supplementation would show a less pronounced effect of the botfly parasitism than the ones from the non-manipulated areas, as more resources and consequent better nutrition might enhance host immune defence by increasing pathogen clearance or resistance to infection (Becker et al., Reference Becker, Streicker and Altizer2018). In our study, it is possible that an increase in host density in areas with artificial food addition would maintain the available energy per individual somewhat similar in comparison to control areas. Also, we expected that the degree of dehydration would increase with the time spent by the animals inside traps, reducing the plasma volume in the blood and consequently increasing the red blood cell concentration (Buffenstein et al., Reference Buffenstein, Maloney and Bronner1999; Fletcher and Boonstra, Reference Fletcher and Boonstra2006), which did not occur, either.
In conclusion, our results indicated that the mouse opossum G. agilis was affected by the botfly parasitism by C. apicalis, even though the effect is not apparent on the body condition. Erythrocyte metrics such as haemoglobin concentration can detect changes in population health when they are not evident in the demographic parameters (Johnstone et al., Reference Johnstone, Lill and Reina2015), and that extends to mass/length data. Parasitized animals seem to conceal the nutrient deficiency with the increase in food intake, maintaining or even gaining weight. We demonstrated that the effect of myiasis could be magnified by environmental stressors and such relationship may be critical for the maintenance of populations in small forest patches such as the woodland savannah (cerradão).
Supplementary material
The supplementary material for this article can be found at https://doi.org/10.1017/S003118201900026X.
Author ORCIDs
Priscilla Lóra Zangrandi, 0000-0003-1406-944X; André Faria Mendonça, 0000-0002-8248-0639; Emerson M. Vieira, 0000-0003-3488-621X
Acknowledgements
We are thankful to all undergraduate and graduate students who provided valuable help in our fieldwork. We thank the permission granted by the Botanic Garden of Brasília (JBB) and the Ecological and Agricultural Field Station of the University of Brasília (Fazenda Água Limpa – FAL/UnB) to our fieldwork. We also thank Nícholas F. de Camargo for the picture of the G. agilis for the figures and José Roberto Pujol-Luz for the species identification of the collected botfly specimens.
Financial support
This work was supported by grant from the Brazilian State Funding Agency of Federal District to EMV (FAPDF – 193.000.914/2015) and from State Funding Agency of São Paulo to APC-N (FAPESP – 2014/16320-7). While conducting this study PLZ received a scholarship from the National Council for Scientific and Technological Development (CNPq); EMV received a personal research grant from CNPq (No 308992/2013-0); and AFM received a post-doctoral fellowship from the Brazilian Federal Agency for Support and Evaluation of Graduate Education (PNPD/CAPES).
Ethical standards
All field methods were approved by the Institutional Animal Care and Use Committee of the University of Brasília (CEUA – UnB; No. 62274/2015) and complied with the requirements of The Brazilian Institute for the Environment and Natural Resources (IBAMA) (Permit No. 15424-1, IBAMA Registration No. 15778628). Permissions for field data collections were given by the director of the Ecological and Agricultural Field Station of the University of Brasília (FAL), José Mauro da Silva Diogo, and by the technical and scientific superintendent of the Botanical Garden of Brasília, Vânia de Araújo Soares. The field studies did not involve endangered or protected species.
Conflict of interest
None.