Introduction
Recent and persistent challenges caused by infectious diseases like Ebola (Bonwitt et al., Reference Bonwitt, Dawson, Kandeh, Ansumana, Sahr, Brown and Kelly2018), Zika (Lambrechts et al., Reference Lambrechts, Scott and Gubler2010), malaria (Tang et al., Reference Tang JA, Templeton TJ and Culleton R2020), Chagas (Milei et al., Reference Milei, Guerri-Guttenberg, Grana and Storino2009) and coronavirus disease-2019 (COVID-19) (WHO, 2020) emphasize the importance of understanding transmission dynamics from evolutionary, ecological and epidemiological perspectives. Transmission to a new host is a fundamental step in the life cycle of every parasite (Lipsitch and Moxon, Reference Lipsitch and Moxon1997), and has crucial effects on the population dynamics and the fitness of both parasites and hosts (McCallum et al., Reference McCallum, Fenton, Hudson, Lee, Levick, Norman, Perkins, Viney, Wilson and Lello2017). Mathematically, transmission is the product of three components: (1) dissemination, which is the capacity to successfully leave an infected host to be translocated to another; (2) translocation or the movement of a pathogen from an infected host to an uninfected one and (3) infectivity, which is the ability to invade new hosts after contact with infected hosts, vectors or environmental reservoirs. The product of these components is not necessarily linearly related (Antolin, Reference Antolin2008). Therefore, transmission is one of the most challenging processes to model and to quantify (McCallum et al., Reference McCallum, Fenton, Hudson, Lee, Levick, Norman, Perkins, Viney, Wilson and Lello2017).
Here, we review an emerging model system for transmission experiments, Gyrodactylus species, which are contagious and ubiquitous ectoparasites of teleost fishes (Bakke et al., Reference Bakke, Cable and Harris2007). These monogeneans are especially suitable for revealing novel insights into the host–parasite ecology and evolution, including population dynamics and epizootiology for several reasons. First, they reproduce in situ on the host and are transmitted during host contact (Bakke et al., Reference Bakke, Cable and Harris2007). Second, all life stages can transmit between hosts and therefore transmission and infection are continuous (Bakke et al., Reference Bakke, Cable and Harris2007). Finally, gyrodactylids have a relatively high level of host preference (Bakke et al., Reference Bakke, Harris, Jansen and Hansen1992; Harris et al., Reference Harris, Shinn, Cable and Bakke2004). Indeed, this genus is one of the most species-rich taxa of Monogenea, with more than 400 described species (Harris et al., Reference Harris, Shinn, Cable and Bakke2004) where more than 73% of 319 species recorded have single hosts, while 4.1% infect more than four taxa with a wide range of up to 15 different hosts (Bakke et al., Reference Bakke, Harris, Jansen and Hansen1992; Harris et al., Reference Harris, Shinn, Cable and Bakke2004).
A notable feature of gyrodactylids is their reproduction: it is characterized by extreme progenesis and a combination of different reproductive models (Bakke et al., Reference Bakke, Cable and Harris2007). The genus mainly comprises viviparous gyrodactylids that give birth to fully grown young and, a few, oviparous species that lay eggs within the environment (Bakke et al., Reference Bakke, Cable and Harris2007). In viviparous species, worms may contain a fully grown daughter in utero, which in turn encloses a developing embryo, boxed inside one another (Cable and Harris, Reference Cable and Harris2002). Contrary to other helminths, these ectoparasites possess features of microparasites (e.g., direct reproduction on their host) with dynamics of macroparasites where adults are limited to only a few, asexual, parthenogenetic or sexually derived offspring (Cable and Harris, Reference Cable and Harris2002).
Viviparous gyrodactylids can transmit horizontally between adult hosts and move between fish during brief contacts through four main routes: (1) direct transfer during contacts between fishes; (2) contact between fishes and detached parasites on the substrate; (3) contact between fishes and detached parasites in the water column and (4) contact between living fishes and infected dead fishes (Bikhovski, Reference Bikhovski1961; Bakke et al., Reference Bakke, Harris, Jansen and Hansen1992). However, their transmission is risky: for example, only 35–39% worms of G. turnbulli (originally described as G. bullatarudis, but redescribed as G. turnbulli by Harris, 1986) that attempt to transfer are successful (Scott and Anderson, Reference Scott and Anderson1984). And in guppies, when the host is dead, only 50% of worms leave the host and spread to a new host (Harris, Reference Harris1980).
Despite the great gyrodactylid diversity, only a few species have been the subject of scientific research. For instance, initial studies of taxonomy and evolution of G. salaris and G. thymalli took place because these parasites devastated salmonid and grayling populations, respectively, in the mid-1970s (Bakke et al., Reference Bakke, Cable and Harris2007). Also, important works include those about infection dynamics of G. turnbulli and G. bullatarudis that parasite guppies (Poecilia reticulata) (see Scott, Reference Scott1982; Scott and Anderson, Reference Scott and Anderson1984; Scott and Nokes, Reference Scott and Nokes1984; Harris, Reference Harris1989; Harris and Lyles, Reference Harris and Lyles1992; Richards and Chubb, Reference Richards and Chubb1996, Reference Richards and Chubb1998). More recently, guppies and their gyrodactylids have been also used in studies of parasite dynamics (see Cable et al., Reference Cable, Harris and Bakke2000), pathology and host–parasite coevolution. Experimental infections have shown that these parasites can change the behaviour (Grether et al., Reference Grether GF, Kasahara S and Cooper EL2004; Kolluru et al., Reference Kolluru GR, Grether GF, Dunlop E and Liu L2006; Croft et al., Reference Croft, Edenbrow, Darden, Ramnarine, Van Oosterhout and Cable2011; Reynolds et al., Reference Reynolds, Arapi and Cable2018; Stephenson, Reference Stephenson2019), mate choice (Kennedy et al., Reference Kennedy, Endler, Poynton and McMinn1987; Houde and Torio, Reference Houde and Torio1992; López, Reference López1999) and immune responses of their hosts (Buchmann and Uldal, Reference Buchmann and Uldal1997; Buchmann and Lindenstrøm Reference Buchmann and Lindenstrøm2002 Grether et al., Reference Grether GF, Kasahara S and Cooper EL2004; Kolluru et al., Reference Kolluru GR, Grether GF, Dunlop E and Liu L2006; van Oosterhout et al., Reference van Oosterhout C and Harris PD2003; Cable and van Oosterhout Reference Cable and Van Oosterhout2007a, Reference Cable and van Oosterhout2007b; Konczal et al., Reference Konczal, Ellison, Phillips, Radwan, Mohammed, Cable and Chadzinska2020a). Recently, the transmission dynamics of gyrodactylids have been given more attention (Stephenson et al., Reference Stephenson, Young, Fox, Jokela, Cable and Perkins2017; Tadiri et al., Reference Tadiri, Scott and Fussmann2016, Reference Tadiri, Scott and Fussmann2018, Reference Tadiri, Kong, Fussmann, Scott and Wang2019).
We synthesize this recent research to highlight the main factors driving the spread of gyrodactylids, and to suggest further likely but untested drivers of transmission. In addition, we present the advances regarding mathematical models that measure the transmission dynamics in the genus. Particularly, this integrative review emphasizes the most studied host–parasite system within the genus Gyrodactylus: Poecilia reticulata (the host) and its two most abundant parasite species, G. turnbulli and G. bullatarudis (see Mohammed et al., Reference Mohammed, King, Bentzen, Marcogliese, van Oosterhout and Lighten2020). The significance of this review arises from the necessity to understand parasite and host features resulting from the interactions and feedbacks that make transmission possible for gyrodactylids, and at the same time, we bring an overall perspective about transmission dynamics that can be applied in other host–parasite systems.
This review is divided into five sections. First, we describe our approach to searching and evaluating the literature (Fig. 1). We then discuss the abiotic and biotic factors important in driving gyrodactylid transmission in the second and third sections, respectively. We then expose the advances in measures and mathematical models of transmission in gyrodactylids. In the final section, we offer conclusions from the existing literature, new perspectives in the study of transmission dynamics of the genus Gyrodactylus and highlight potentially fruitful future research directions.
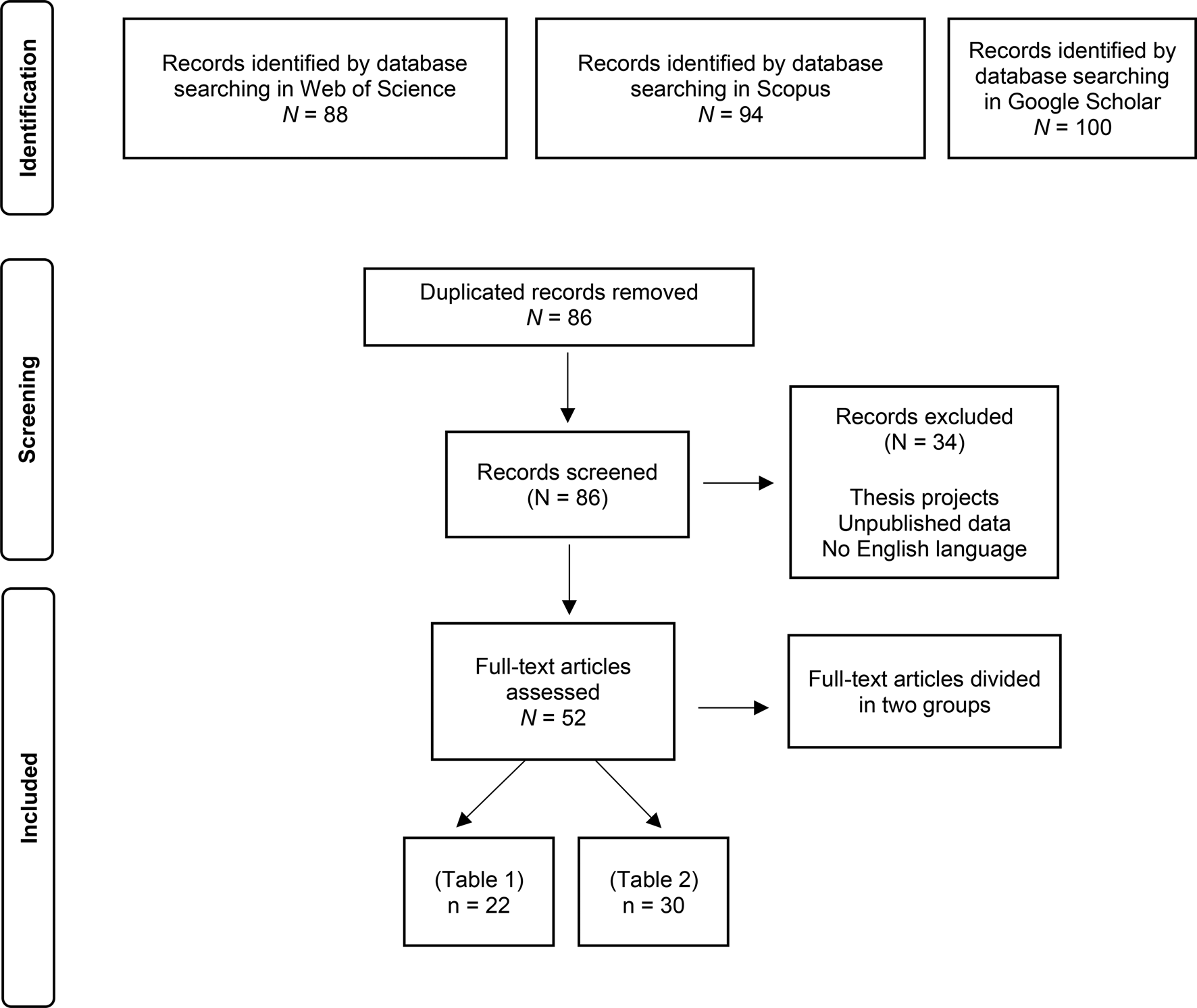
Fig. 1. Integrative review on main variables affecting Gyrodactylus spread on teleost fish, and the potential factors that enhance transmission. We used PRISMA guidelines (see Moher et al., Reference Moher, Liberati, Tetzlaff and Altman2015).
Literature search and selection
To identify the main transmission promoters of gyrodactylids, we searched on two databases: ISI Web of Science and Scopus. In addition, we searched on the web search engine Google Scholar to identify possible highly cited and lesser-known articles (Beel and Gipp, Reference Beel and Gipp2009; Martin-Martin et al., Reference Martin-Martin, Orduna-Malea, Harzing and López-Cózar2017). The searches were conducted in Puebla, Mexico using the browser Google Chrome at 10:00 h Mexican Central Standard Time on 30 June 2021. On Web of Science, we searched for articles using the following search string: TOPIC: (Gyrodactylus OR gyrodactylids OR monogenea AND TOPIC: transmission OR spread OR disease OR infection). Refined by CATEGORIES: parasitology OR ecology OR evolutionary biology OR behavioural sciences, OR fisheries, OR marine freshwater biology. On Scopus, the following syntax was used: TITLE-ABS-KEY (‘Gyrodactylus’ OR ‘gyrodactylids’ OR ‘monogenea’ AND TITLE (‘transmission’, ‘spread’ OR ‘disease’ OR ‘infection’. Finally, the search strings used for Google Scholar were: ‘Gyrodactylus’ AND ‘transmission’; ‘Gyrodactylids AND ‘transmission’; ‘Monogenea AND transmission’; ‘Gyrodactylus AND ‘spread’; Gyrodactylids AND ‘spread’; ‘Monogenea AND ‘spread’. For Google Scholar our criteria for search saturation were met when 10 consecutive pages of results (100 results in total) issued no new articles that met our inclusion criteria (articles that tested o proposed results about gyrodactylid transmission).
To refine the results of the searches, three eligibility criteria were used: obtained items had to be peer-reviewed papers, in English, published from 1 January 1980 to 30 June 2021. This timeframe was set because according to a primary search, the first articles that tested Gyrodactylus transmission were published in the 1980s (see Tables 1 and 2), just after early studies of population dynamics in the genus (see Scott and Nokes, Reference Scott and Nokes1984).
Table 1. Studies that measured variables related to parasite transmission in the genus Gyrodactylus
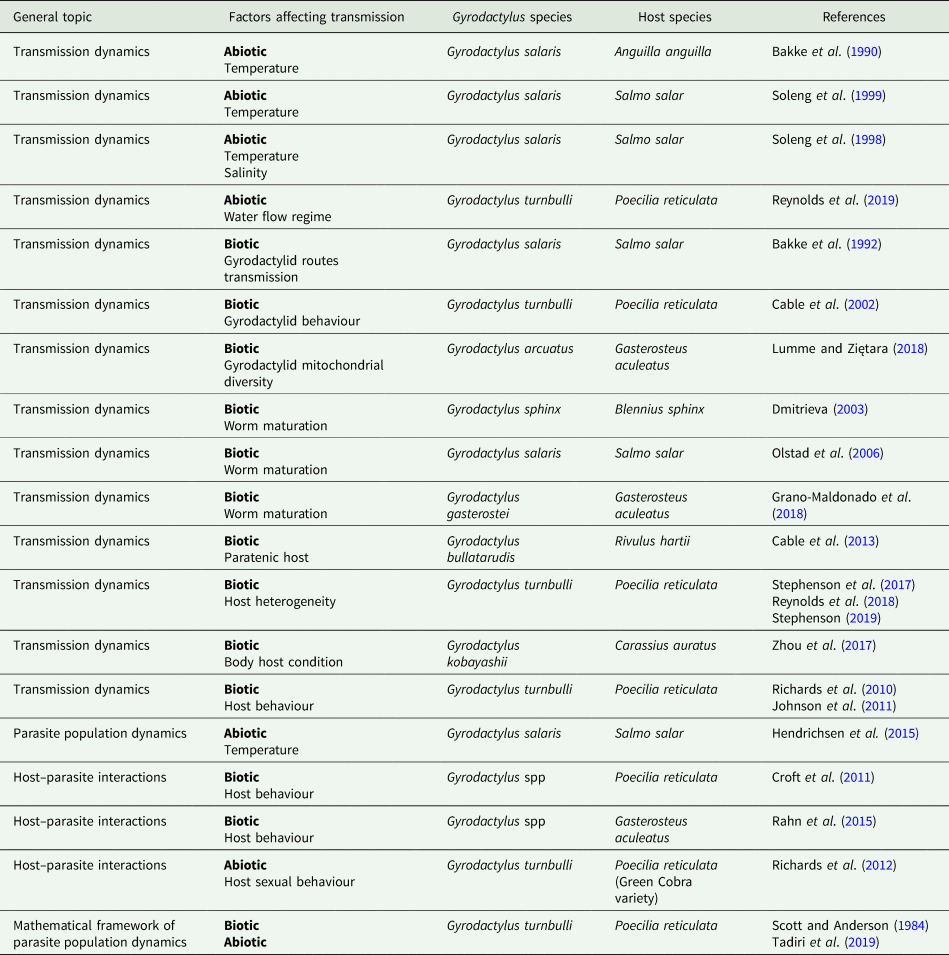
After the removal of duplicates, we obtained a total of 86 records; removal of those referring to secondary bibliographical sources as books, conference records and notes reduced this to a final pool of 52 articles (see PRISMA diagram in Fig. 1). A total of 35 articles were obtained from Web of Science and 12 from Scopus; the use of Google Scholar for systematic reviews and meta-analyses has been debated (Callicott and Vaughn, Reference Callicott and Vaughn2005), however, we decided to also include 5 articles from Google Scholar (Bakke et al., Reference Bakke, Harris, Jansen and Hansen1992; Soleng et al., Reference Soleng, Bakke and Hansen1998; Dmitrieva, Reference Dmitrieva2003; Olstad et al., Reference Olstad, Cable, Robertsen and Bakke2006 and Winger et al., Reference Winger, Kanck, Kristoffersen R and Knudsen R2007) because they fulfilled the requirements of our search and contributed to the topic of interest. In total, we included 52 articles in our review (Fig. 1).
We inspected the abstracts and titles of this final pool and classified them depending on their main research question. The first group consisted of studies that measured variables directly related to parasite transmission (Table 1). In the second group, we included all the studies where the authors did not measure parasite transmission per se, but based on their results, they suggested variables that could affect transmission (Table 2).
Table 2. Studies that suggest variables that could affect Gyrodactylus transmission based on their results or their conclusions
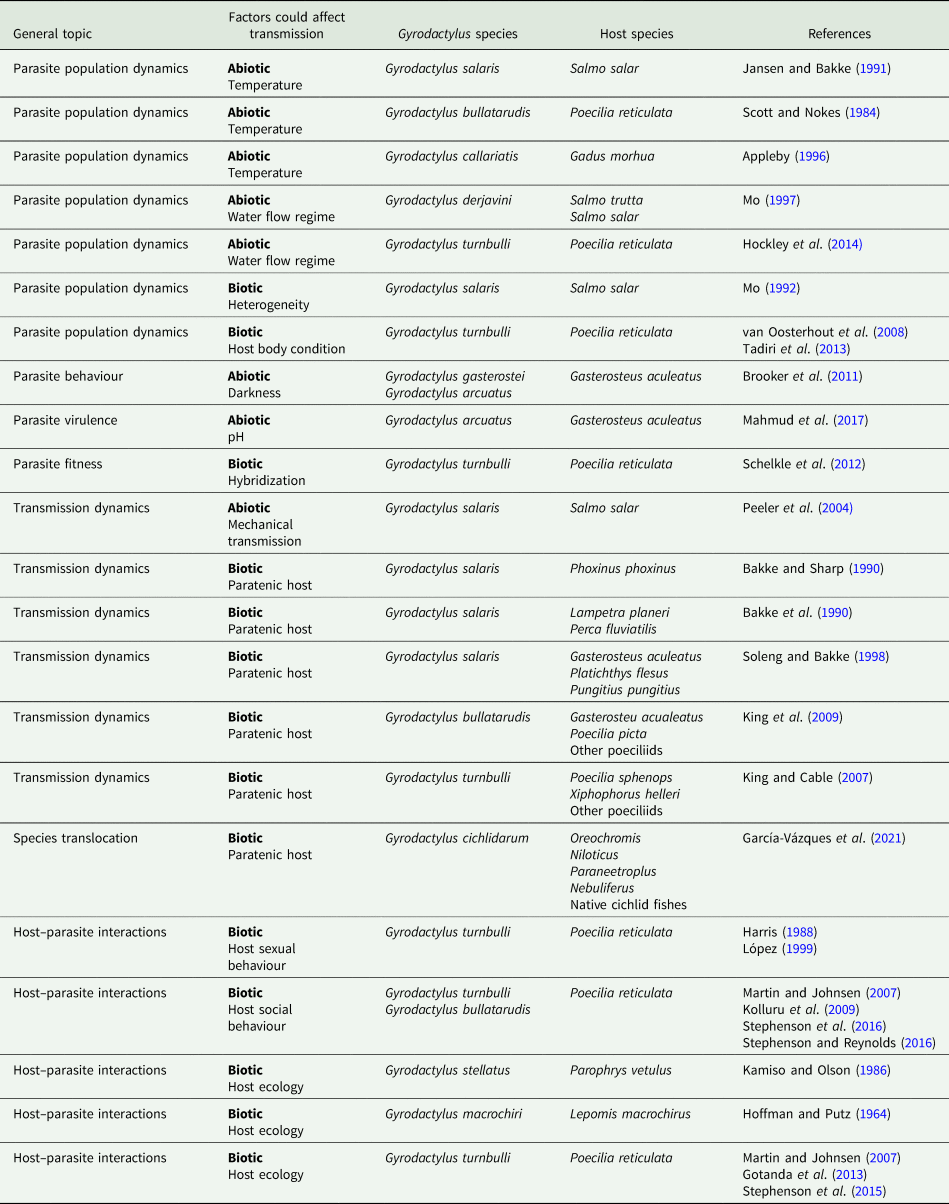
Abiotic factors affecting gyrodactylid transmission
The presence or absence of parasites in host populations is the result of biotic, abiotic factors and their interaction (Anderson and Sukhdeo, Reference Anderson and Sukhdeo2010). Although there is no consensus about which of these factors are dominant, abiotic factors have been suggested as key drivers of host physiology, parasite multiplication and transmission (Poulin, Reference Poulin2020). Here, we summarize our current understanding of the abiotic factors affecting when gyrodactylids leave their host, and thus promote transmission in the genus. For gyrodactylids, abiotic factors that modify their populations dynamics are broadly water temperature and chemistry, so it is not surprising that these factors are related to their successful transmission; additionally, water flow and darkness seem to affect Gyrodactylus ability to spread (Soleng and Bakke, Reference Soleng and Bakke1998; Soleng et al., Reference Soleng, Jansen and Bakke1999, Reference Soleng, Poleo Antonio and Bakke2005; Poleo et al., Reference Poleo, Schjolden, Hansen, Bakke, Mo, Rosseland and Lydersen2004).
Temperature might influence gyrodactylid spread because threshold temperatures increase components of their fitness. For instance, G. bullatarudis has the longest lifespan at 21°C, the highest average fecundity, and an intrinsic maximum rate of parasite population at 25.5°C, but they are not able to survive at 30°C. Within the temperature range of 6–13°C, G. salaris produces the maximal and higher number of offspring than other Gyrodactylus species (Jansen and Bakke, Reference Jansen and Bakke1991), but at 40°C the parasite dies (Koski et al., Reference Koski, Anttila and Kuusela2015). The effect of temperature on transmission has only been tested in G. salaris, where low temperatures reduce the rate of transmission (Bakke et al., Reference Bakke, Jansen and Brabrand1990), whereas high temperatures lead to a higher degree of accidental dislodgement (Harris, Reference Harris1980); G. salaris tend to be transmissible from salmon, S. salar to the eel, Anguilla Anguilla, and vice versa at 4 and 13°C, and between host to host, S. salar at 1.2 to 12.2°C (Soleng et al., Reference Soleng, Bakke and Hansen1998).
Higher temperatures also tend to increase host activity levels, potentially contact between hosts, and thus perhaps the likelihood of transmission (Scott and Nokes, Reference Scott and Nokes1984). So far, there are only indirect reports in G. salaris which indicate that during spring and summer in Norway, infections by these worms on S. salar increase, but in winter, when water temperature in Norwegian rivers are close to 0°C and fish activity is reduced to a minimum, parasite populations decline (Jansen and Bakke, Reference Jansen and Bakke1991). Also, early studies suggested that high activity of guppies promotes the transmission of G. bullatarudis (Scott and Nokes, Reference Scott and Nokes1984), but there are no empirical studies.
Specific levels of salinity also improve gyrodactylid spread in G. salaris. These worms successfully transmit from salmon smolt to parr at 0.0, 7.5, 10.0 and 20.0 ‰ salinity (Soleng et al., Reference Soleng, Bakke and Hansen1998) and most worms are transmitted at 7.5‰ salinity from infected salmon smolts to uninfected salmon parr (Soleng et al., Reference Soleng, Bakke and Hansen1998). Although these worms are not a euryhaline species (i.e., organisms that can adapt to a wide range of salinities), G. salaris are also able to reproduce in fresh water after direct transfer from high salinities, which indicates that G. salaris can be dispersed through estuaries, survive in saline waters, and reproduce in fresh water (Soleng et al., Reference Soleng, Bakke and Hansen1998). The effect of salinity on parasite transmission has only been studied in G. salaris, so it is imperative to broaden the species tested to fully understand the effect of this and other abiotic factors on the transmission dynamics of gyrodactylids.
Water flow regime is further likely important. Shoals of P. reticulata exposed to interrupted flow exhibited greater mean transmission rates of G. turnbulli compared to continuous and non-flow conditions (Reynolds et al., Reference Reynolds, Hockley, Wilson and Cable2019). In this experiment, a 12 h flow: 12 h no flow comprised the interrupted flow regime, and during flow, guppies aggregated in a refuge wherein flow was minimal (Reynolds et al., Reference Reynolds, Hockley, Wilson and Cable2019). Therefore, in interrupted flow conditions there was a higher likelihood of more direct contacts between hosts, and perhaps because guppies appear unable to discriminate olfactory cues between infected and uninfected conspecifics in these flow regimes, they were unable to avoid the elevated transmission risk (Reynolds et al., Reference Reynolds, Hockley, Wilson and Cable2019). This aggregation behaviour occurs in natural habitats with high predation regimes during the night (Seghers, Reference Seghers1974; Croft et al., Reference Croft, Arrowsmith, Bielby, Skinnern, White, Couzin, Magurran and Krause2003).
Despite the lack of empirical studies, darkness itself might enhance worm activity level, resulting in transmission (Brooker et al., Reference Brooker, Grano-Maldonado, Irving, Bron, Longshaw and Shinn2011). Transmission during darkness may also minimize the chances of gyrodactylids being eaten by hosts that forage during the day (Brooker et al., Reference Brooker, Grano-Maldonado, Irving, Bron, Longshaw and Shinn2011). Further, in systems like guppies, transmission may occur when infected fish are moving between resting conspecifics attempting to offload their parasite burdens (Reynolds et al., Reference Reynolds, Hockley, Wilson and Cable2019). Still, so far, the effect of light conditions on worm activity has been tested only in G. gasterostei and G. arcuatus (Brooker et al., Reference Brooker, Grano-Maldonado, Irving, Bron, Longshaw and Shinn2011).
As our review illustrates, so far, the effect of only a handful of abiotic factors such as temperature, salinity, and water flow has been studied on Gyrodactylus transmission. Among these factors, temperature appears to be of particular importance, and more research on this factor could be particularly useful. For instance, it is known that different temperature regimes change the size of gyrodactylid haptoral hooks and bars (Bakke et al., Reference Bakke, Cable and Harris2007) which are the organs that allow the attachment to the hosts. Temperature may have negative effects on gyrodactylids because host immunity is enhanced at higher temperatures, as reaction rates of complex proteins such as the complement cascade increase (Bakke et al., Reference Bakke, Cable and Harris2007). In addition, we propose studies in water pH. Studies indicated that parasites G. arcuatus are locally adapted to the water in their own lake (North Uist in the Scottish Western Isles) and interestingly, virulence is related to lake pH which suggests that the evolution of virulence can be substantially affected by the abiotic environment (Mahmud et al., Reference Mahmud, Bradley and MacColl2017).
Biotic factors affecting gyrodactylid transmission
Gyrodactylid features
All gyrodactylid life stages can be transmitted, but there seems to be a higher probability of transmission when worms are mature. This occurs in two situations: the worm has given birth at least once, as in G. salaris (Olstad et al., Reference Olstad, Cable, Robertsen and Bakke2006), or when the male reproductive organ is developed, as in G. sphinx (Dmitrieva, Reference Dmitrieva2003) and G. gasterostei (Grano-Maldonado et al., Reference Grano-Maldonado, Moreno-Navas and Rodriguez-Santiago2018). The male reproductive system in gyrodactylids consists of a posterior testis, an anterior seminal vesicle, and a penis which becomes functional once the second embryo has commenced development (Cable and Harris, Reference Cable and Harris2002). It is therefore likely that this apparent migration of mature parasites between hosts promotes the sexual reproduction of gyrodactylids, which might maintain the genetic diversity in the population (Janecka et al., Reference Janecka, Rovenolt and Stephenson2021).
Sexual reproduction also enables hybridization, which may enhance transmission at micro and macroevolutionary scales. In terms of microevolution, there is only one experimental work that demonstrates that outcrossing between monogeneans results in a higher parasite burden over time, and an increased maximum parasite burden (Schelkle et al., Reference Schelkle, Faria, Johnson, Van Oosterhout and Cable2012). Hybrid genotypes appear more tolerant and resistant to the fish immune response, allowing them to maintain a reproducing population on the host for longer than parental parasites (Schelkle et al., Reference Schelkle, Faria, Johnson, Van Oosterhout and Cable2012). At macroevolutionary scales, new genome analysis demonstrates the role of hybridization in the evolutionary success of G. bullatarudis in Tobago (Konczal et al., Reference Konczal, Ellison, Phillips, Radwan, Mohammed, Cable and Chadzinska2020a, Reference Konczal, Przesmycka, Mohammed, Phillips, Camara, Chmielewski, Hahn, Guigo, Cable and Radwan2020b). Indeed, there is phylogenetic evidence that co-infecting Gyrodactylus species may hybridize before and after host switches (Ziętara and Lumme, Reference Ziętara and Lumme2002; Kuusela et al., Reference Kuusela, Zietara and Lumme2007). In other parasites, hybridization may increase pathogen fecundity, infectivity, virulence, and transmission rates (Arnold, Reference Arnold2004; Detwiler and Criscione, Reference Detwiler and Criscione2010). However, the effect of hybridization on transmission abilities of gyrodactylids has not yet been tested. Directly comparing the transmission success of inbred and outbred strains within species, and hybrids between different Gyrodactylus species, represents an exciting and experimentally tractable research direction.
Despite their direct life cycle, some gyrodactylid species appear to use paratenic hosts to promote their transmission and survival under certain conditions. Paratenic hosts are optional hosts involved in the life cycle of parasites, but they are not required for its completion (Marcogliese, Reference Marcogliese1995). For instance, when experimental infections took place, G. bullatarudis transmit from guppies (its host) to a guppy predator killifish Rivulus hartii, and vice versa (Cable et al., Reference Cable, Archard, Mohammed, McMullan, Stephenson, Hansen and Van Oosterhout2013). Interestingly, G. bullatarudis survived longer than G. turnbulli on R. hartii out of water, which suggests that the parasite is more likely to survive in the wild when R. hartii migrate overland between isolated guppy populations (Reznick, Reference Reznick1995).
Intimately related to paratenic hosts is host switching, which is the ability of parasites to use new host species (Huyse et al., Reference Huyse, Audenaert and Volckaert2003; Araujo et al., Reference Araujo, Braga, Brooks, Agosta, Hoberg, Von-Hartenthal and Boeger2015). This appears to have been predominant mode of radiation within Gyrodactylus (Ziętara and Lumme, Reference Ziętara and Lumme2002; Meinilä et al., Reference Meinilä, Kuusela, Ziętara and Lumme2004). This is clearly demonstrated by molecular evidence and phylogenetic analysis (Cable et al., Reference Cable, Harris, Tinsley and Lazarus1999) in groups like ‘wageneri’ which, probably evolved in two ways: (1) dissemination of euryhaline species via anadromous fishes in periglacial environments of Northern Europe, and (2) dissemination of stenohaline cyprinids through Europe during the last glaciation (Bakke et al., Reference Bakke, Harris and Cable2002). In the first case, gyrodactylids may have switched from basal host Phoxinus Phoxinus (ciprinidae family) to salmonids and gasterosteids, whereas in the second scenario, other gyrodactylids may have switched from the same basal host to cottids (Cottidae family) (Ziętara and Lumme, Reference Ziętara and Lumme2002).
Host switching may occur in G. salaris, G. bullatarudis and G. turnbulli Boeger et al., Reference Boeger, Kritsky, Pie and Engers2005. The first gyrodactylid species, described originally from Baltic Salmon, has become widespread around the world infecting new fishes in the wild and in fish farms (Johnsen and Jensen, Reference Johnsen and Jensen1986, Reference Johnsen and Jensen1992). Furthermore, during experimental infections G. salaris can transmit to co-occurring non-salmonid hosts, like lampreys (Lampetra planeri), perch (Perca fluviatilis) (see Bakke et al., Reference Bakke, Jansen and Brabrand1990), minnows (P. phoxinus) (see Bakke and Sharp, Reference Bakke and Sharp1990), roach (Rutilus rutilus), and flounder (Platichthys flesus) (see Soleng and Bakke, Reference Soleng and Bakke1998) with infections that lasted a few days but without worm reproduction (Bakke and Sharp, Reference Bakke and Sharp1990; Bakke et al., Reference Bakke, Jansen and Hansen1991). Gyrodactylids that parasitize guppies can infect a range of different poeciliids and gasteroids under laboratory conditions. For example, G. turnbulli appeared to prefer Poecilia sphenops and Xiphophorus helleri in terms of attachment time showing longer duration of infection and higher parasite loads in comparison to other poeciliids species (King and Cable, Reference King and Cable2007), while G. bullatarudis is able to transmit and reproduce on G. acualeatus and P. picta (a sister species of P. reticulata; see King et al., Reference King, van Oosterhout and Cable2009). It is unclear to what extent these host ranges take place in the wild. In fact, studies found that mixed groups of P. reticulata and P. picta from Trinidad had a lower abundance of Gyrodactylus and were less likely to be infected than conspecifics in single-species groups (Darget et al., Reference Dargent F, Torres-Dowdall J and Ramnarine I2013).
New evidence indicates that translocation of fishes due to aquaculture, like carp, tilapia and trout, has resulted in the co-introduction of their gyrodactylid species (García-Vásquez et al., Reference García-Vásquez, Pinacho-Pinacho, Guzmán-Valdivieso, Calixto-Rojas and Rubio-Godoy2021) which may promote switching to native fishes. This is the case of various cichlids from three genera; Coptodon, Sarotherodon and Oreochromis (referred to generically as ‘tilapia’) and their Gyrodactylus pathogens like G. cichlidarum which was initially described from Sarotherodon galilaeus, but later was recorded in several farmed and wild cichlids on all continents except Antarctica (García-Vásquez et al., Reference García-Vásquez, Hansen, Christison, Rubio-Godoy, Bron and Shinn2010; Soler-Jiménez et al., Reference Soler-Jiménez, Paredes-Trujillo and Vidal-Martínez2017; Zhang et al., Reference Zhang, Zhi, Xu, Zheng, Bilong Bilong, Pariselle and Yang2019). For example, at least three African gyrodactylids translocate into Mexico with their ‘tilapia’. Today, these worms are widely distributed throughout the country infecting farmed and feral ‘tilapias’ but also native poeciliids fishes (García-Vásquez et al., Reference García-Vásquez, Pinacho-Pinacho, Guzmán-Valdivieso, Calixto-Rojas and Rubio-Godoy2021).
In summary, the gyrodactylid features that seem to promote transmission are worm maturation, hybridization, paratenic host usage and host switching. These could consist of opportunistic strategies that some Gyrodactylus spp. employ when environmental conditions are unsuitable for the transmission to their main hosts. Particularly, hybridization and host switching have provided positive effects at macroevolutionary scales enabling them to successfully colonize their main host but also distantly related fishes.
Host features
Heterogeneity, the individual differences between hosts in their physiology and behaviour (VanderWaal and Ezenwa, Reference VanderWaal and Ezenwa2016; White et al., Reference White, Forester and Craft2018), means that outbreaks can be explosive if key individuals become infected (Lloyd-Smith et al., Reference Lloyd-Smith, Schreiber, Kopp and Getz2005b; White et al., Reference White, Forester and Craft2018). Physiological and behavioural features are incorporated in epidemiological models where the transmission rate β is the product of two component rates: βc, behavioural component, is the effective contact rate between infected and uninfected individuals, and βp, physiological component, is the infected host's probability of transmitting an infection given that contact (Hawley et al., Reference Hawley, Etienne, Ezenwa and Jolles2011). As with other parasites, βp and βc, may covary in gyrodactylid transmission, having important implications for disease dynamics (Stephenson et al., Reference Stephenson JF and Perkins SE2018). Recently, donor heterogeneity was evaluated in guppies parasitized by G. turnbulli. The results revealed that three features from donors affected transmission speed, transmission load, and the fitness of transmitted parasites (Stephenson et al., Reference Stephenson, Young, Fox, Jokela, Cable and Perkins2017). These are: (1) Infection load (calculated as the number of donor worms on the day of transmission) that affected transmission speed, where heavily infected donors transmitted infection more quickly, but without a linear relationship; (2) More resistant individuals (quantified using the integral of infection load over the course of infection) transmitted more parasites in comparison to those less resistant; (3) Donors exposed to naïve recipients twice during infection – experienced donors – transmitted 3.1 more parasites than inexperienced donors exposed to naïve recipients just once during infection.
Hosts can be heterogeneous in their physiological defences against parasites, which comprise two broad mechanisms: they can directly attack parasites to reduce parasite loads (resistance), or they can limit the harm caused by these loads (tolerance) (Råberg et al., Reference Råberg, Graham and Read2009). Both can be either innate or acquired (Janeway et al., Reference Janeway, Travers and Walport2001). For instance, guppies present different innate and acquired immunity against G. turnbulli between and within populations (Scott and Anderson, Reference Scott and Anderson1984; Madhavi and Anderson, Reference Madhavi and Anderson1985; Cable and van Oosterhout, Reference Cable and Van Oosterhout2007a; Stephenson et al., Reference Stephenson, Van Oosterhout, Mohammed and Cable2015); in Trinidad, guppies from Lower Aripo show a superior innate response and greater resistance than fish from Upper Aripo (Cable and van Oosterhout, Reference Cable and van Oosterhout2007b). Similar variation is reported in other fishes parasitized by gyrodactylids, such as the Atlantic salmon, S. salar (Bakke et al., Reference Bakke, Jansen and Harris1996; Buchmann et al., Reference Buchmann, Lindenstrøm, Sigh, Dalgaard and Larsen2005; Gilbey et al., Reference Gilbey, Verspoor, Mo, Sterud, Olstad, Hytterod, Jones and Noble2006; Matějusová et al., Reference Matejusova, Felix, Sorsa-Leslie, Gilbey, Noble, Jones and Cunningham2006), three-spined stickleback, G. aculeatus (de Roij et al., Reference de Roij, Harris and MacColl2010; Robertson et al., Reference Robertson, Bradley and MacColl2017), goldfish, C. auratus (Zhou et al., Reference Zhou, Li, Zou, Zhang, Wu, Li and Wang2018, Reference Zhou, Liu, Dong, Yang, Xu, Yang, Gu and Ai2021) and rainbow trout, O. mykiss (Lindenstrøm and Buchmann, Reference Lindenstrøm and Buchmann2000; Lindenstrøm et al., Reference Lindenstrøm, Secombes and Buchmann2004).
Tolerance and resistance are responses modulated by the host's immune system and ultimately could depend on host condition (Beldomenico and Begon, Reference Beldomenico and Begon2010): host condition could therefore affect host infection intensities and therefore transmission by either improving host defence, or by providing more resources for the parasite (Cornet et al., Reference Cornet, Bichet, Larcombe, Faivre and Sorci2014). Two gyrodactylid hosts show both responses results; in guppies, P. reticulata there is a positive relation between condition of an initial ‘source’ fish (a fish with high relative condition index, Kn) and major epidemic variables (i.e., parasite incidence, peak parasite load and the degree of parasite aggregation), resulting in parasites either aggregated on ‘source’ hosts of high condition or transferred to hosts of high condition (Tadiri et al., Reference Tadiri, Dargent and Scott2013). In goldfish, C. auratus, there is a negative influence of initial body condition of uninfected fish on total abundance of parasites (Zhou et al., Reference Zhou, Zou, Wu, Wang, Marcogliese and Li2017). Computer models propose that larger fish are individuals with higher relative condition that support heavier parasite loads because larger fish can offer more living space for parasites (van Oosterhout et al., Reference van Oosterhout, Potter, Wright and Cable2008), however, further research into host body condition and fish size on mapping parasite transmission is needed.
Host behaviour drives parasite transmission dynamics across host–parasite systems. Mating behaviour, for instance, is critical for sexually transmitted bacteria, protozoa and other pathogens (Thrall et al., Reference Thrall, Antonovics and Dobson2000; Moore, Reference Moore2002; Knell and Webberley, Reference Knell and Webberley2004); foraging behaviour is one of the most important routes of infection for bacteria and helminths (Moore, Reference Moore2002); and social behaviour affects in general the dissemination of parasites (Altizer et al., Reference Altizer, Nunn, Thrall, Gittleman, Antonovics, Cunningham, Dobson, Ezenwa, Jones, Pedersen, Mary and Pulliam2003; Briard and Ezenwa, Reference Briard and Ezenwa2021). In social animals like guppies, even at lower densities, the contact rate among hosts is sufficiently high to allow transmission (Johnson et al., Reference Johnson, Lafferty, Van Oosterhout and Cable2011). Sex-specific differences in social behaviours also drive different transmission dynamics; female guppies tend to shoal more than males (Magurran and Seghers, Reference Magurran and Seghers1994) which results in females becoming infected earlier in the epidemic with fourfold higher likelihood of becoming infected than males (Richards et al., Reference Richards, Van Oosterhout and Cable2010; Johnson et al., Reference Johnson, Lafferty, Van Oosterhout and Cable2011). Conversely, male guppies are more likely to be key in intershoal parasite transmission (Stephenson et al., Reference Stephenson, Kinsella, Cable and Van Oosterhout2016) due to their lower propensity to shoal, as they prefer to move between shoals of females searching for mating opportunities (Croft et al., Reference Croft, Edenbrow, Darden, Ramnarine, Van Oosterhout and Cable2011).
While host social behaviour affects parasite spread, gyrodactylid infection affects the social behaviour of the host. As infection becomes more prevalent within a population, individuals modify their social preferences, and thus their social network position (Funk et al., Reference Funk, Bansal, Bauch, Eames, Edmunds, Galvani and Klepac2015). For example, guppies avoid gyrodactylid-infected conspecifics, despite the negative effects on their social associations, through initiating more shoal fission events and spending less time associated with the shoal (Croft et al., Reference Croft, Edenbrow, Darden, Ramnarine, Van Oosterhout and Cable2011). Similarly, three-spined sticklebacks prefer to spend more time near a group of uninfected conspecifics than near a group of infected conspecifics (Rahn et al., Reference Rahn, Hammer and Bakker2015). Surprisingly, young guppies imprinted with chemical cues of infected fish prefer to associate with, rather than avoid, parasitized individuals (Stephenson and Reynolds, Reference Stephenson and Reynolds2016).
Transmission of ectoparasites, including gyrodactylids, also occurs during sexual interactions because they often involve males and females in physical contact with each other. For example, the gyrodactylid Isancistrum subulatae transmits to the pelagic squid Alloteuthis subulate during agonistic behaviour or copulation (Llewellyn, Reference Llewellyn1984). For promiscuous hosts like guppies, parasite transmission has been suggested to take place during mating or sexual harassment (Harris, Reference Harris1988; López, Reference López1999). Indeed, male ornamental guppies (Green Cobra variety) are more likely to become infected and transmit G. turnbulli to conspecifics during the performance of courtship behaviour that is generally directed towards females (Richards et al., Reference Richards, Van Oosterhout and Cable2012). However, nobody has evaluated how the transmission rates of gyrodactylids during host sexual interactions compares to their transmission during non-sexual social interactions.
Host behaviour and parasite infection interact bidirectionally (Ezenwa et al., Reference Ezenwa, Archie EA, Hawley DM, Martin, Moore and White2016; Hawley et al., Reference Hawley, Gibson, Townsend, Craft and Stephenson2021), and the interaction likely depends on infection characteristics. Negative covariation between βp and βc, such as the most infectious hosts being the most strongly avoided, can lead to parasite extinction in host populations, whereas a positive covariation, such as the most infectious hosts having the highest contact rates, can lead to rapid epidemic spread (Hawley et al., Reference Hawley, Etienne, Ezenwa and Jolles2011). Indeed, in the absence of infection, susceptible male guppies are less social than resistant ones, and during late infection (15–17 days post-infection), the most susceptible males spent more time shoaling (Stephenson, Reference Stephenson2019). Similarly, uninfected guppies only avoid infected conspecifics when they are at the most infectious stage of infection (Stephenson et al., Reference Stephenson, Young, Fox, Jokela, Cable and Perkins2017). This indicates a negative correlation between host infectiousness (βp) and transmission-relevant social behaviour (βc) in male guppies, but also that feedbacks between animal behaviour and parasite infection are dynamic, and depend on host sex and susceptibility (Stephenson, Reference Stephenson2019).
Previous research highlights two host ecology variables that may affect the transmission of gyrodactylids: habitat structure and predation pressure. Host habitat may dictate transmission route: host–host contact could be the most important for gyrodactylids of pelagic fish (Parker, Reference Parker1965; Malmberg, Reference Malmberg1970; Harris, Reference Harris1982; Kamiso and Olson, Reference Kamiso and Olson1986), but for benthic hosts, transmission by detached parasites is probably the most important route because hosts are continuously in contact with the substrate. For example, G. macrochiri can achieve higher infections when wire cages containing the hosts were placed in contact with the substrate rather than suspended in the water column (Hoffman and Putz, Reference Hoffman and Putz1964). Predators can also affect transmission. For example, because guppy shoaling behaviour increases in high-predation populations (Houde, Reference Houde1998), there is a higher probability of parasite transmission there, and correspondingly, observational studies report higher prevalence in these populations (Martin and Johnsen, Reference Martin and Johnsen2007; Gotanda et al., Reference Gotanda, Delaire, Raeymaekers, Perez-Jvostov, Dargent, Bentzen, Scott, Fussmann and Hendry2013; Stephenson et al., Reference Stephenson, Van Oosterhout, Mohammed and Cable2015).
In conclusion, host physiology, host behaviour, and their interaction (i.e., host heterogeneity) could be the host driver features of gyrodactylid transmission. Altogether, these shape individuals vulnerable to infection or particularly adept at transmitting the parasite. In addition, we propose that host ecology is an unexplored feature that probably also plays an important role in disease dynamics of gyrodactylids.
Advances in the measurement of transmission in the genus Gyrodactylus
Measuring parasite transmission is challenging. The most important route of transmission in gyrodactylids is direct contact between infected and uninfected fish, either between live hosts or from a dead to a live host (Scott and Anderson, Reference Scott and Anderson1984). Then, according to the simple transmission function dI/dt = βSI/(S + I), where β is the transmission coefficient (Getz and Pickering, Reference Getz and Pickering1983; Anderson and May, Reference Anderson and May1992; Lloyd-Smith et al., Reference Lloyd-Smith, Cross, Briggs, Daugherty, Getz, Latto, Sanchez, Smith and Swei2005a, Reference Lloyd-Smith, Schreiber, Kopp and Getz2005b), S, the number of susceptible hosts, and I as the number of infected hosts (Smith et al., Reference Smith, Acevedo-Whitehouse and Pedersen2009; McCallum et al., Reference McCallum, Fenton, Hudson, Lee, Levick, Norman, Perkins, Viney, Wilson and Lello2017), the probability of transmission in the genus Gyrodactylus could be at a rate βSI (frequency-dependent transmission) rather than βSI/N (density-dependent transmission) (Heggberget and Johnsen, Reference Heggberget and Johnsen1982; Johnsen and Jensen, Reference Johnsen and Jensen1986, Reference Johnsen and Jensen1992; Johnson et al., Reference Johnson, Lafferty, Van Oosterhout and Cable2011; Zhou et al., Reference Zhou, Zou, Wu, Wang, Marcogliese and Li2017). Still, because pathogen transmission often occurs through more than one route, each of which may have a different functional relationship with population density (Ryder et al., Reference Ryder, Miller, White, Knell and Boots2007), it is likely that Gyrodactylus transmission combines frequency- and density-dependent dynamics.
Transmission models using research in guppies show that the probability of an epidemic increases with the product of duration and mean intensity of infection in the primary infected fish, and that the total parasite population increases with the host population density, but density does not necessarily affect the probability of an epidemic (Johnson et al., Reference Johnson, Lafferty, Van Oosterhout and Cable2011). In species like G. kobayashii, faster spreading epidemics are not detected in larger populations of goldfish, Carassius auratus at constant density, and before day 20 of the infection, epidemics occurred faster in smaller host populations (Zhou et al., Reference Zhou, Zou, Wu, Wang, Marcogliese and Li2017). In addition, total mean prevalence and total mean abundance are not affected by host population size (Zhou et al., Reference Zhou, Zou, Wu, Wang, Marcogliese and Li2017). The same dynamic is reported in G. salaris, which has a prevalence of almost 100% without a density threshold of their host, Atlantic salmon (Heggberget and Johnsen, Reference Heggberget and Johnsen1982; Johnsen and Jensen, Reference Johnsen and Jensen1986, Reference Johnsen and Jensen1992). In other words, gyrodactylids could persist despite low host population density (Ryder et al., Reference Ryder, Miller, White, Knell and Boots2007).
In brief, taking into account the reported information about gyrodactylid transmission and basic concepts from epidemiologic theory, we propose that if there is an efficient contact rate among individuals (i.e., effective transmission rate), frequency-dependent transmission could be prominent throughout the entire infection (Ryder et al., Reference Ryder, Miller, White, Knell and Boots2007). However, since the contact rate among individuals is not constant through the infection and host population, density-dependent transmission might take place with the drop of infection, because parasites need the largest number of susceptible hosts for arising new effective contacts (Frank, Reference Frank1996; McCallum, Reference McCallum2001; Begon et al., Reference Begon, Bennett, Bowers, French, Hazel and Turner2002; Ryder et al., Reference Ryder, Miller, White, Knell and Boots2007).
Another important measurement of parasite transmission is transmission potential, R 0, which has been suggested as the best metric of parasite fitness (Antolin, 2008). R 0 is the number of secondary infections arising from an initial infection in a population of susceptible hosts (Heesterbeek, Reference Heesterbeek2002; Roberts, Reference Roberts2007) and theory predicts that a macroparasite can spread or invade when R 0 is greater than one (VanderWaal and Ezenwa, Reference VanderWaal and Ezenwa2016). We only detected two studies that modelled transmission dynamics: one early study on G. turnbulli that proposed a model to estimate the rate of transmission as the number of parasites directly transferred from the donor fish proportional to the density of parasites at a specific time (Scott and Anderson, Reference Scott and Anderson1984); and one more recent study that modelled guppy-Gyrodactylus dynamics in small populations with the estimation of R 0 (Tadiri et al., Reference Tadiri, Kong, Fussmann, Scott and Wang2019).
Conclusions and future directions
In summary, from our review of 52 articles published between 1980 and 2021, transmission dynamics in the genus Gyrodactylus are affected by both abiotic variables such as temperature, salinity and water flow, and biotic factors like gyrodactylid biology and host heterogeneity. Relationships between behavioural and physiological components may arise under an assortment of contexts, and the effects of these interactions can be intensified by host behaviour–parasite feedback loops.
Since these monogeneans are directly transmitted between hosts, the probability of transmission depends on the frequency of contact between hosts but may also be density-dependent at some point during the infection (Ryder et al., Reference Ryder, Miller, White, Knell and Boots2007). G. turnbulli and G. bullatarudis that infect guppies are a good system to illustrate the complexity of transmission rates. On the one hand, female guppies could maintain frequency-dependent rates when engaging more contacts during shoaling behaviour, but also density-dependent rates because they are highly social with other females. Male guppies, on the other, may only drive a frequency-dependent transmission during sexual interactions since they have different contacts when they move among shoals to obtain mating opportunities.
Although gyrodactylids have a direct life cycle, worms post-1st birth and those with a functional male reproductive system are more likely to transmit (Olstad et al., Reference Olstad, Cable, Robertsen and Bakke2006). Considering the high risk of failing to transmit to a new host, leaving at least one offspring on a host seems the optimal strategy for continuation of parasite population. Meanwhile, the sexual maturity of worms could promote a migratory behaviour for sexual reproduction, thereby increasing the genetic variability in the population. Experimental studies that test the relationship between worm maturity and transmission likelihood are needed.
Hybridization represents a tractable research direction in the study of gyrodactylid transmission. At a macroevolutionary scale, recent hybridization has played an important role in shaping genetic variation of G. turnbulli and G. bullatarudis, followed by clonal reproduction and recombination, respectively, in each species (Konczal et al., Reference Konczal, Przesmycka, Mohammed, Phillips, Camara, Chmielewski, Hahn, Guigo, Cable and Radwan2020b, Reference Konczal, Przesmycka, Mohammed, Hahn, Cable and Radwan2021). However, parasite strains of hybrid origin may additionally show enhanced phenotypic features such as higher infectivity, expanded host range and increased transmission potential as it is suggested in other pathogens (Ravel et al., Reference Ravel C, Cortes S and Dedet JP2006). We propose empirical studies which compare transmission rates between inbred and outbred gyrodactylid strains. Importantly, human activity, migrations and climate change may increase the hybridization and prompt adaptation of many species. This could be the case of gyrodactylids which are commonly kept in aquaria and farm populations around the world (Trujillo-González et al., Reference Trujillo-González, Becker, Vaughan and Hutson2018; Maceda-Veiga and Cable, Reference Maceda-Veiga and Cable2019; Paladini et al., Reference Paladini, Shinn, Taylor, Bron and Hansen2021).
Importantly, some studies indicate that gyrodactylid microhabitat use in terms of competition and parasite density may drive transmission events (see Rubio-Godoy et al., Reference Rubio-Godoy, Muñoz-Córdova, Garduño-Lugo, Salazar-Ulloa and Mercado-Vidal2012). Increases in parasite infrapopulations appear to result in gyrodactylids occupying sites that promote transmission (Mo, Reference Mo1997; Harris, Reference Harris1988), but more studies are needed.
Though the spread of worms is predicted to happen in a short time, parasites will not necessarily leave their host since there is a high risk of mortality during the translocation as well as low probability to attach to a suitable new host. Notably, some gyrodactylid species use host switching and paratenic hosts under specific conditions to complete transmission (Olstad et al., Reference Olstad, Cable, Robertsen and Bakke2006). Indeed, host switching appears to be the predominant mode of radiation within the genus that allowed their survival during glaciation events. Today, species like G. salaris, G. bullatarudis, and G. turnbulli could persist and reproduce in some cases by using host species that inhabit the same environments as their main hosts. We consider it pertinent to study these possible gyrodactylid features in the wild. Remarkably, aquaculture among others is accelerating the translocation of G. cichlidarum and possible host-switching into native Mexican poeciliids (see García-Vásquez et al., Reference García-Vásquez, Razo-Mendivil and Rubio-Godoy2017).
The rate of contact between hosts that allows gyrodactylid spread can co-vary with host immune responses, host behaviour and infection-induced behavioural changes. These changes are likely non-uniform, where some individuals can infect only a few others while a small subset of hosts is responsible for most new infections. Host ability to transmit more gyrodactylids is then probably a result of superior tolerance, high body condition, highly social behaviour, strong social networks and elevated promiscuity. Importantly, host ecology like predation regime and aquatic environments may change disease dynamics. For instance, we propose that in guppies, females have high rates of transmission to other females during social interactions whereas males are infected or infect females during sexual interactions or when they move among shoals. This pattern could be different between high and low predation sites and depend on fish community structure.
Despite abiotic factors being the first tested factors in gyrodactylid dynamics, today these receive less research attention than biotic factors. We stress the need to conduct more studies of abiotic factors such as: water pH, since studies point a strong relationship between gyrodactylid virulence and water pH (Mahmud et al., Reference Mahmud, Bradley and MacColl2017); salinity, taking into consideration that some species like G. salaris shows wide salinity tolerance, and dark light conditions, which could trigger host-seeking behaviour. Collectively, these abiotic factors can potentially alter dynamics of gyrodactylid–host interactions and may determine transmission rates.
Overall, only a few species, mainly G. salaris, G. gasterostei, G. kobayashii, G. turnbulli and G. bullatarudis, have been used in studies of transmission, which is a bias with possible important implications because not all Gyrodactylus species necessarily show the same dynamics. Particularly, guppies and G. turnbulli is the only host–parasite system widely studied in terms of biotic features and transmission dynamics. We need research on more aspects of host heterogeneity, incorporating the feedbacks between host behaviour and parasite transmission in other host–parasite systems. Possibly because of the small amount of data and few experiments testing transmission, there is a lack of mathematical models that quantify and explain transmission patterns, which is a general pattern for most parasites, not only gyrodactylids.
Parasite transmission is a multi-faced process (Antolin, 2008). Here, we have synthesized research on an important parasite genus to identify host, parasite and environmental factors that influence gyrodactylid spread. With aquaculture pressures, climate change and human-mediated translocation, this investigation contributes to the understanding of pathogen transmission dynamics in times of especial urgency to the public and wildlife health.
Acknowledgements
Tepox-Vivar Natalia is grateful to Consejo Nacional de Ciencia y Tecnología (CONACYT) for the research fellowship #1001626 that was received as a master's student in the program #005671, Maestría en Ciencias Biológicas. We thank Oscar Ríos and Miguel Rubio-Godoy for comments on the manuscript and Gerardo Islas for his observations. We are also grateful to an anonymous referee for his/her review.
Author contribution
Tepox-Vivar N selected and classified the studies and drafted the manuscript. Stephenson JF and Guevara-Fiore P edited the manuscript and contributed to the discussion and ideas for the review. All authors read and approved the final version of the manuscript.
Financial support
We were supported by CONACYT (research fellowship #1001626 to N. T.-V.) and the University of Pittsburgh (J. F. S.).
Conflicts of interest
None to declare.
Ethical standards
Not applicable.