Introduction
The Asian fish tapeworm (Schyzocotyle (formerly Bothriocephalus) acheilognathi) is an intestinal cestode parasite of fishes native to East Asia, and was originally described in 1934 as Bothriocephalus acheilognathi from a single small cyprinid fish (Acheilognathus rhombeus) in Japan (Yamaguti, Reference Yamaguti1934; Kuchta et al., Reference Kuchta, Choudhury and Scholz2018). Unusually for a tapeworm, S. acheilognathi has a very broad host range and has been found in at least 312 freshwater fish species, belonging to 38 families and 14 orders, as a result of worldwide introductions (Kuchta et al., Reference Kuchta, Choudhury and Scholz2018). The species has also been identified in non-piscine vertebrate hosts including a human, amphibians, reptiles and birds (Prigli, Reference Prigli1975; García-Prieto & Osorio-Sarabia, Reference García-Prieto and Osorio-Sarabia1991; Scholz, Reference Scholz1999; Yera et al., Reference Yera, Kuchta, Brabec, Peyron and Dupouy-Camet2013; de León et al., Reference de León, Lagunas-Calvo, García-Prieto, Briosio-Aguilar and Aguilar-Aguilar2018; Kuchta et al., Reference Kuchta, Choudhury and Scholz2018), although these are considered accidental hosts (Kuchta et al., Reference Kuchta, Choudhury and Scholz2018). Infection with S. acheilognathi can cause bothriocephalosis in its host, with clinical signs including blockage of the gastrointestinal tract, destruction of intestinal mucosa, intestinal rupturing, distended abdomen, weight loss, protein depletion, intestinal inflammation, anaemia, diminished swimming ability and eventual mortality (Davydov, Reference Davydov1978; Scott & Grizzle, Reference Scott and Grizzle1979; Brouder, Reference Brouder1999; Hansen et al., Reference Hansen, Choudhury, Heisey, Ahumada, Hoffnagle and Cole2006; Matey et al., Reference Matey, Ervin and Hovey2015; Cole & Choudhury, Reference Cole and Choudhury2016). The clinical signs can also be subtle, leading to reduced growth in the host, which can result in higher predation rates (Hansen et al., Reference Hansen, Choudhury, Heisey, Ahumada, Hoffnagle and Cole2006; Choudhury et al., Reference Choudhury, Zheng, de León, Martínez-Aquino, Brosseau and Gale2013). The global spread of invasive fish species and their parasites, such as S. acheilognathi, is likely attributable to the exotic fish trade (Košuthová et al., Reference Košuthová, Šmiga, Oros, Barčák and Košuth2015; Kuchta et al., Reference Kuchta, Choudhury and Scholz2018).
During the 1960s and 1970s, S. acheilognathi was imported from East Asia to Europe and North America in grass carp, which was introduced to reduce the growth of vegetation in freshwater ecosystems (Choudhury et al., Reference Choudhury, Charipar, Nelson, Hodgson, Bonar and Cole2006, Reference Choudhury, Zheng, de León, Martínez-Aquino, Brosseau and Gale2013; Matey et al., Reference Matey, Ervin and Hovey2015; de León et al., Reference de León, Lagunas-Calvo, García-Prieto, Briosio-Aguilar and Aguilar-Aguilar2018). The parasite has since spread globally (Cole & Choudhury, Reference Cole and Choudhury2016; Kuchta et al., Reference Kuchta, Choudhury and Scholz2018) and has been the cause of numerous devastating infections and high mortalities of naïve hosts in fish hatcheries, with serious economic consequences for the aquaculture industry (Han et al., Reference Han, Shin, Kim, Choresca, Jun and Gomez2010; Kilian et al., Reference Kilian, Klauda, Widman, Kashiwagi, Bourquin and Weglein2012; Oros et al., Reference Oros, Barčák, Bazsalovicsová and Hanzelová2015; Xi et al., Reference Xi, Li, Wang, Nie and Xie2016; Boonthai et al., Reference Boonthai, Herbst, Whelan, Van Deuren, Loch and Faisal2017). Fish are a major source of global income (Shelton & Rothbard, Reference Shelton and Rothbard2006; Olsen & Hasan, Reference Olsen and Hasan2012; Tacon & Metian, Reference Tacon and Metian2013), particularly for developing countries (Mulokozi et al., Reference Mulokozi, Mmanda, Onyango, Lundh, Tamatamah and Berg2020), where koi carp (Cyprinus carpio haematopterus) are often bred for consumption or aesthetic purposes (Rahaman et al., Reference Rahaman, Sabbir, Sarder, Biswas and Ghosh2012). In addition, the invasion of waterways outside of its natural range by S. acheilognathi may threaten native fishes (Choudhury et al., Reference Choudhury, Charipar, Nelson, Hodgson, Bonar and Cole2006; de León et al., Reference de León, Lagunas-Calvo, García-Prieto, Briosio-Aguilar and Aguilar-Aguilar2018). Brouder & Hoffnagle (Reference Brouder and Hoffnagle1997) studied fishes from the Colorado River, USA, and found that three of the rivers’ four endangered fish species host S. acheilognathi (Brouder & Hoffnagle, Reference Brouder and Hoffnagle1997). A subsequent study found S. acheilognathi parasitizing all fish species in the Little Colorado River (Choudhury et al., Reference Choudhury, Hoffnagle and Cole2004). Schyzocotyle acheilognathi has contributed to the decline of 40–60% of the humpback chub (Gila cypha) in the Grand Canyon National Park, Arizona, USA, since the 1990s and is being monitored in the desert of the south-west USA (Choudhury & Cole, Reference Choudhury, Cole and Francis2012).
In Australia, S. acheilognathi has been previously reported from the eastern coastal states including New South Wales (NSW), Queensland and Victoria (Dove & Fletcher, Reference Dove and Fletcher2000). Only three studies have molecularly confirmed the species identity of the parasite in Australia, and all are from NSW (Luo et al., Reference Luo, Nie, Zhang, Wang and Yao2002; Xi et al., Reference Xi, Li, Wang, Nie and Xie2016; Kuchta et al., Reference Kuchta, Choudhury and Scholz2018; Rochat et al., Reference Rochat, Blasco-Costa, Scholz and Unmack2020). With the advent of molecular technology, there has been an increasing need for genus-specific primers that amplify hypervariable regions to enable species identification. Hadziavdic et al. (Reference Hadziavdic, Lekang, Lanzen, Jonassen, Thompson and Troedsson2014) compared all of the regions within the 18S ribosomal RNA (rRNA) gene of S. acheilognathi and found that the hypervariable region V4 had the longest variable region with the greatest length of polymorphisms, and also included a 70 bp conserved region for primer annealing. Primers targeting the 18S rRNA V4 region in S. acheilognathi have been successful in identifying the species (Nickrent & Sargent, Reference Nickrent and Sargent1991; Bean et al., Reference Bean, Škeříková, Bonner, Scholz and Huffman2007; Hadziavdic et al., Reference Hadziavdic, Lekang, Lanzen, Jonassen, Thompson and Troedsson2014). In the present study, we report the first evidence of S. acheilognathi from Western Australia (WA), within the South-Western Ichthyological Province, a region of extreme endemism, using morphological and molecular identification at the 18S rRNA V4 locus.
Materials and methods
Study site and sample collection
Between February and May 2018, 134 introduced fish from three species were collected from Blue Lake, a modified wetland in the City of Joondalup, WA (S 31o 44.700′, E 115o 45.9668′) as part of an approved feral fish control program. The fish consisted of 91 goldfish (Carassius auratus), 26 koi carp (C. carpio haematopterus) and introduced eastern gambusia or mosquitofish (Gambusia holbrooki).
Freshly euthanized fishes were dissected at Murdoch University's Fish Health Unit, Perth, WA, and dead tapeworms were removed from the intestine. Half of the tapeworms from each sample were placed in 10% formalin for microscopy and the remainder placed in 100% ethanol for molecular analysis.
Statistical analysis
Prevalence [percentage (%) of hosts infected with S. acheilognathi, with 95% confidence intervals (CIs)] and intensity of infection (number of S. acheilognathi found in each host) (Bush et al., Reference Bush, Lafferty, Lotz and Shostak1997) were calculated using the Program QPweb 3.0 (Reiczigel et al., Reference Reiczigel, Marozzi, Fábián and Rózsa2019).
Morphological analysis
For morphological analysis on samples from goldfish and koi carp, tapeworms were first relaxed in sterile water overnight and then stained using a dilute Semichon's acetocarmine stain, dehydrated using a graded alcohol series, cleared in methyl salicylate, mounted whole in Canada balsam and viewed in brightfield on an Olympus BX50 compound microscope [10×–40× (Olympus Corporation, Shinjuku, Tokyo, Japan)] with an Olympus DP71 universal camera (Olympus Corporation, Shinjuku, Tokyo, Japan), using cellSens software (Olympus Corporation, Shinjuku, Tokyo, Japan). The eggs were viewed on the same microscope with a DP1 universal camera but using a Nomarski filter. Infection intensities were viewed on an Olympus stereo microscope SZX7 with an Olympus DP27 camera, using cellSens software.
Molecular analysis
Total genomic DNA (gDNA) was extracted from ~25 mg of tapeworm tissue (one tapeworm per extraction) and fish intestinal tissue. The tapeworms and intestinal tissue were dissected in sterile petri dishes and extractions were conducted using a Qiagen DNeasy Blood and Tissue kit (Qiagen, St Louis, Missouri, USA). The 18S rRNA V4 region was amplified using the forward primer Ces1 (5′-CCAGCAGCCGCGGTAACTCCA-3′) and reverse primer Ces2 (5′-CCCCCGCCTGTCTCTTTTGAT-3′) producing a ~420 bp product as previously described (Scholz et al., Reference Scholz, Škeříková, Hanzelová, Koubková and Baruš2003; Bean et al., Reference Bean, Škeříková, Bonner, Scholz and Huffman2007), except that the annealing temperature was adjusted to 64°C after optimization. Polymerase chain reaction (PCR) was conducted using 25 μl reaction volumes, which consisted of 0.02 U/μl Taq DNA polymerase, 1× reaction buffer, 1 mM MgCl2, 200 μM of each dNTP, 1 μM of each primer, 2 μl template gDNA and PCR-grade water to the final volume. PCR cycling conditions for the Ces1/Ces2 primer set consisted of an initial denaturation at 95°C for 15 min, followed by 45 cycles of 94°C for 1 min, 64°C for 1 min and 72°C for 2 min, with a final elongation step at 68°C for 10 min. PCR products were separated by gel electrophoresis using a 1.0% (w/v) agarose gel (Fisher Biotec, Wembley, WA, Australia) in Tris-acetate buffer (consisting of 40 mm Tris-hydrochloride, 20 mM ethylenediamine tetra-acetic acid at pH 7.0). PCR products of the expected size were excised from the agarose gel, purified using an in-house filter-tip-based method (Yang et al., Reference Yang, Murphy, Yong, Ng-Hublin, Estscourt and Hijjawi2013) and sequenced using a Big Dye version 3.1 Terminator Cycle Sequencing Kit (Applied Biosystems, Massachusetts, USA) on a 96-capillary 3730xl DNA Analyzer (Thermo Fisher Scientific, Waltham, Massachusetts, USA) at Murdoch University and at the Australian Genome Research Facility (AGRF), Perth, WA. Sequences were assembled in the forward and reverse directions to produce a consensus sequence, assessed for quality and trimmed of primers using Geneious v10.2.2 (Kearse et al., Reference Kearse, Moir, Wilson, Stones-Havas, Cheung and Sturrock2012). Consensus sequences were checked against nucleotide sequences in the GenBank database using the Basic Local Alignment Search Tool (BLAST) and aligned with related sequences using the MUSCLE alignment tool (Edgar, Reference Edgar2004) with Geneious software. The 364 bp alignment (including gaps) was imported into the PhyML program (Guindon et al., Reference Guindon, Dufayard, Lefort, Anisimova, Hordijk and Gascuel2010) to assess nucleotide substitution models based on Bayesian Information Criterion. The model general time reversible + gamma distribution (GTR + G) was used to construct a Bayesian phylogenetic tree using Geneious and the MrBayes plugin v3.2.6 (Huelsenbeck & Ronquist, Reference Huelsenbeck and Ronquist2001). The tree was built using the following parameters: Hasegawa-Kishino-Yano (HKY85) + GTR + G model; 1,100,000 Markov chain Monte Carlo (MCMC) length; ‘burn-in’ length of 10,000; subsampling frequency of 200. The tree was rooted with the outgroup sequence Parabothriocephalus gracilis (KR780945) (not shown).
Results
Morphological identification
The tapeworms from the koi carp and goldfish were identified as S. acheilognathi based on the following morphological characters: fleshy heart-shaped scolex with unarmed bothria that were short and deep; proglottids had rounded edges with an absent neck; the first proglottids were immediately posterior to the scolex and much narrower than the scolex; and in mature proglottids, genital pores and vitelline follicles were clearly visible (figs 1 and 2a, b). All tapeworms examined using microscopy had gravid proglottids (fig. 2c), with eggs being both operculated and unembryonated (fig. 2d).

Fig. 1. Semichon's acetocarmine-stained heart-shaped scolex of Schyzocotyle acheilognathi, from the intestines of koi carp (Cyprinus carpio haematopterus), showing unarmed bothria and proglottids much narrower than the scolex with rounded edges (photo: Aileen Elliot).

Fig. 2. Semichon's acetocarmine-stained mature and gravid proglottids of Schyzocotyle acheilognathi. (a) Arrows indicate genital pores and (b) vitelline follicles. (c) Gravid proglottids ready for dispersal and (d) operculated and unembryonated eggs, with arrow indicating operculum (photos: Aileen Elliot).
Prevalence and intensity of infection
The length and weight range of the koi carp, goldfish and eastern gambusia as well as molecular confirmation of S. acheilognathi in each of the hosts was recorded with an overall tapeworm prevalence of 8.5% (95% CI 7.5–9.5) (table 1).
Table 1. Length, weight and prevalence of fishes infected with Schyzocotyle acheilognathi from Blue Lake, Joondalup.

The intensity of infection measured in goldfish and koi carp was high, ranging from 203 to 607 parasites per infected fish (mean = 386, 95% CI 296–486) (table 2 and fig. 3), with evidence of intestinal perforation, blockage and ischemia. Several tapeworms exceeded 400 mm in length, which was almost equal to the length of the intestines in some fish. Eastern gambusia also had a high occurrence of parasite burdens, although intensity of infection was not measured.

Fig. 3. Intensity of Schyzocotyle acheilognathi infection in one koi carp (photo: Aileen Elliot).
Table 2. Number of tapeworm scolices found in the goldfish and koi carp from Blue Lake, Joondalup in Western Australia.

a Sample identification code.
b Each scolex was counted as one cestode.
Molecular characterization
The 18S rRNA V4 region was amplified from two koi carp (CP1, CP4), two goldfish (CPAFT3, CPAFT4) and four eastern gambusia (CPAFT1, CPAFT2, CPAFT5 and CPAFT7) (table 3). Sequencing confirmed that two of the koi carp isolates (CP1 and CP4, GenBank accession numbers MT898660 and MT898661) were identical to each other (supplementary appendix) and to S. acheilognathi (KX060604) isolated from red shiner (Cyprinella lutrensis) in the Czech Republic, and from a human sample (HM367066) (Yera et al., Reference Yera, Kuchta, Brabec, Peyron and Dupouy-Camet2013; Brabec et al., Reference Brabec, Kuchta, Scholz and Littlewood2016). Furthermore, isolates CP1 and CP4 were 99.0% similar to 18S rRNA V4 sequences from 12 Schyzocotyle isolates from other fishes, including threadfin shad (Dorosoma pretensense) and C. lutrensis (DQ86690 and AY340106, respectively) (Škerıková et al., Reference Škerıková, Hypša and Scholz2004; Bean et al., Reference Bean, Škeříková, Bonner, Scholz and Huffman2007). Two of the eastern gambusia isolates (CPAFT1 and CPAFT2) (GenBank accession numbers MT898662 and MT898663) were identical and were 99.0% similar to nine BLAST hits, including S. acheilognathi from red shiner (C. lutrensis) (KX060604) and largemouth yellowfish (Labeobarbus kimberleyensis) (KX060602) (Brabec et al., Reference Brabec, Kuchta, Scholz and Littlewood2016). Four of the isolates obtained in this study from two goldfish (C. auratus) (CPAFT3 and CPAFT4) and two eastern gambusia (CPAFT5 and CPAFT7) were identical to each other (GenBank accession numbers (MT898664–MT898667), but only 98.7% similar to a single sequence named S. acheilognathi (AY340104) (Škerıková et al., Reference Škerıková, Hypša and Scholz2004). These Schyzocotyle sp. Blue Lake genotype isolates were then most similar to 12 S. acheilognathi isolated from Nazas chub (Gila conspersa) and a common carp (KX060601 and KX060600, respectively) (Brabec et al., Reference Brabec, Kuchta, Scholz and Littlewood2016). However, sequence similarity with these 12 isolates was only 91.0%, with 99.0% query cover (table 3) (see supplementary appendix table S1).
Table 3. 18S rRNA V4 isolates from fishes with closest matches to sequences in GenBank.

Phylogenetic analysis
All isolates were distinct from Bothriocephalus and Senga species and grouped within Schyzocotyle clades (fig. 4). The koi carp sequences (CP4, MT898661; and CP1, MT898660) grouped with S. acheilognathi sequences (Schyzocotyle Group 2A), and two of the novel eastern gambusia genotypes (CPAFT1, MT898662; and CPAFT2, MT898663) formed a discrete S. acheilognathi subgroup (Schyzocotyle Group 2B) with strong support (posterior probability (PP) = 0.9). Four sequences (MT898664–MT898667) formed a discrete subgroup to S. acheilognathi (Group 3) with strong support (PP = 1.0), which included two goldfish (CPAFT3 and CPAFT4) and two eastern gambusia (CPAFT5 and CPAFT7) (fig. 4).
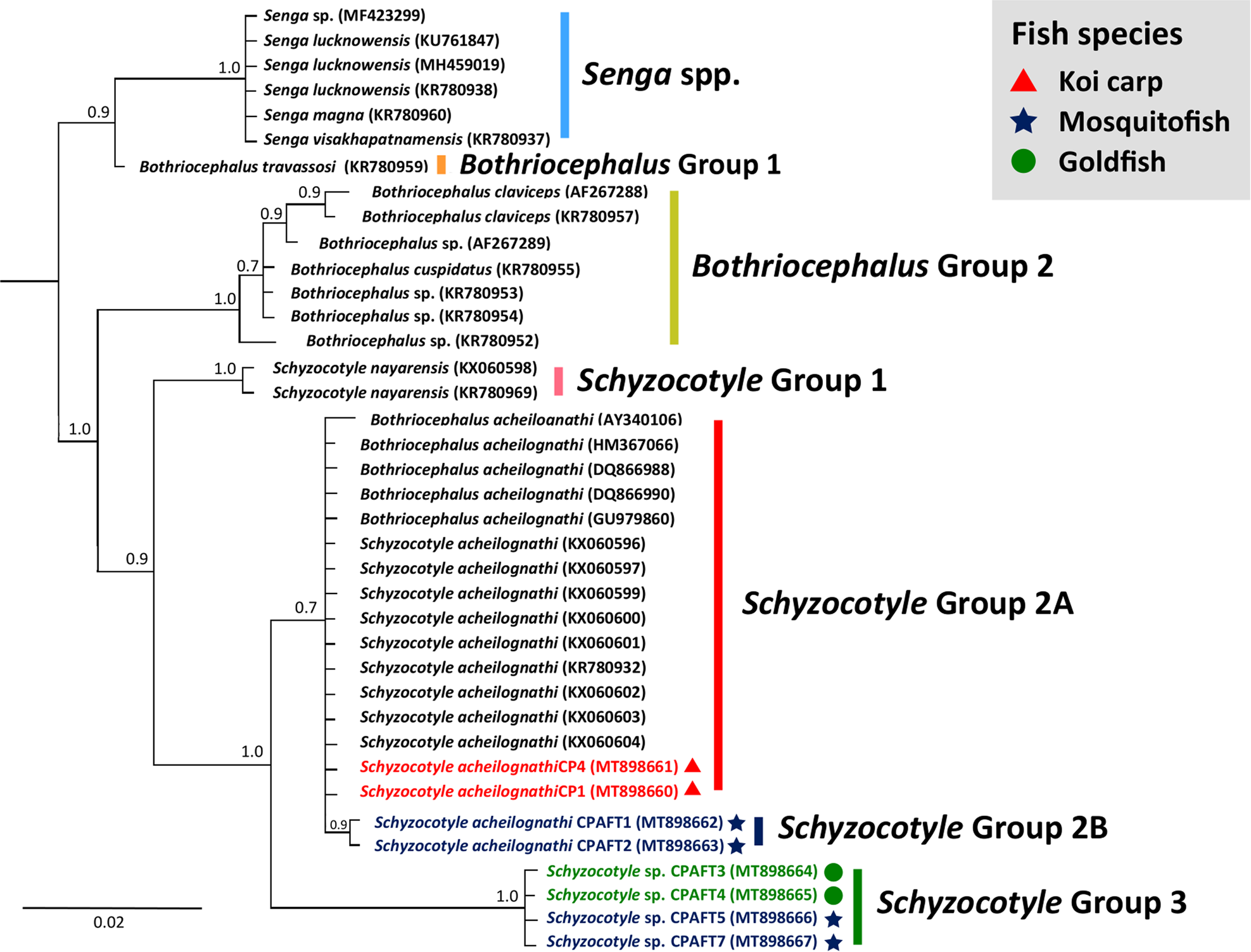
Fig. 4. Bayesian phylogenetic tree of a 354 bp alignment (including gaps) of 18S rRNA V4 sequences of known Schyzocotyle acheilognathi species and Schyzocotyle sequences derived from this study. Scale bar shows the number of nucleotide substitutions per site. Sequences from this study are indicated by colours and symbols in the figure's legend.
Discussion
AFT identification has historically been based on the unique morphology of the scolex, which is inadequate for detecting closely related subtypes (Brabec et al., Reference Brabec, Kuchta, Scholz and Littlewood2016; Xi et al., Reference Xi, Li, Wang, Nie and Xie2016). Indeed, in this study, the gross morphology of the AFT and Schyzocotyle sp. Blue Lake genotype (GenBank accession numbers MT898664–MT898667) was indistinguishable. Schyzocotyle sp. Blue Lake genotype was evident by the low sequence homology (91.9% sequence similarity) to AFT sequences in GenBank (table 3), and the phylogenetic grouping of this tapeworm was distinct from other Schyzocotyle species, with strong support (PP = 1.0) (fig. 4). This emphasizes the need for molecular methods for the subtype identification of Schyzocotyle sp. Blue Lake genotype and the AFT in future studies. As this study targeted a partial fragment of the 18S rRNA gene, characterization of the complete or near full-length of 18S rRNA is required to confirm that Schyzocotyle sp. Blue Lake genotype represents a novel species from an unknown origin. Future studies should also aim to morphologically and genetically characterize Schyzocotyle species identified by this study, which will also aid our understanding of the potential origins of the AFT in south-western WA (Luo et al., Reference Luo, Nie, Zhang, Wang and Yao2002; Xi et al., Reference Xi, Li, Wang, Nie and Xie2016).
In the present study, S. acheilognathi was identified by microscopy and molecular characterization for the first time in WA from introduced goldfish, koi carp and eastern gambusia in a modified urban wetland. If translocated from this locality (e.g. by humans, birds, floods, etc.) to adjacent lentic or lotic systems, this parasite would pose a serious threat to the unique freshwater (and possibly estuarine) fish fauna of south-western WA. Several studies have already shown that AFT can infect fish in environments with low to moderate salinity (Ozturk et al., Reference Ozturk, Aydogdu and Dogan2002; Bean, Reference Bean2008; Bean & Bonner, Reference Bean and Bonner2010; İnnal et al., Reference İnnal, Aydogdu and Guclu2016; Sara et al., Reference Sara, Barour, Sameh, Boualleg and Mourad2016; McAllister et al., Reference McAllister, Bursey, Robison, Connior and Trauth2017; Güven & Öztürk, Reference Güven and Öztürk2018; Zhokhov et al., Reference Zhokhov, Pugacheva, Molodozhnikova and Berechikidze2019), and has already threatened the populations of several endemic species in other countries (Salgado-Maldonado & Pineda-López, Reference Salgado-Maldonado and Pineda-López2003; Choudhury et al., Reference Choudhury, Charipar, Nelson, Hodgson, Bonar and Cole2006, Reference Choudhury, Zheng, de León, Martínez-Aquino, Brosseau and Gale2013; de León et al., Reference de León, Lagunas-Calvo, García-Prieto, Briosio-Aguilar and Aguilar-Aguilar2018).
The inland waters in the south-west of WA have the highest proportion of endemic freshwater fish on the continent and are considered one of the world's biodiversity hotspots (Myers et al., Reference Myers, Mittermeier, Mittermeier, Da Fonseca and Kent2000; Beatty & Morgan, Reference Beatty and Morgan2013; Morgan et al., Reference Morgan, Unmack, Beatty, Ebner, Allen and Keleher2014). Invasive fish species may predate or compete with native fishes, or alter the habitat to the detriment of native species (Olden et al., Reference Olden, Kennard and Pusey2008; Beatty & Morgan, Reference Beatty and Morgan2013; Morgan et al., Reference Morgan, Unmack, Beatty, Ebner, Allen and Keleher2014). An additional threat posed by alien fishes, and one that is often under-appreciated, is the introduction of new parasites and pathogens (co-invaders) (Lymbery et al., Reference Lymbery, Morine, Kanani, Beatty and Morgan2014). Co-invading parasites can be more pathogenic to native fishes than to their natural hosts, possibly due to the lack of coevolutionary existence (Lymbery et al., Reference Lymbery, Morine, Kanani, Beatty and Morgan2014) and, as such, can cause morbidity and mortality on already declining native populations. In south-western WA, many river systems have seasonally intermittent flow, and fish communities must survive the dry season in small, disconnected refuge pools, which could amplify the transmission and effects of co-invading parasites (Lymbery et al., Reference Lymbery, Lymbery and Beatty2020). At least two other species of co-invading parasites, including Ligula intestinalis (Morgan, Reference Morgan2003; Chapman et al., Reference Chapman, Hobbs, Morgan and Gill2006) and Lernaea cyprinacea, have recently been identified in native fishes in south-western WA and they also infect diadromous and estuarine fishes that venture into freshwaters (Hassan et al., Reference Hassan, Beatty, Morgan, Doupé and Lymbery2008). Lernaea cyprinacea was introduced to WA's native waterways as a co-invader with goldfish and now infects at least six native freshwater species (Hassan et al., Reference Hassan, Beatty, Morgan, Doupé and Lymbery2008). The original source of the S. acheilognathi in WA is unknown, but it appears that either goldish or koi carp are likely to be the source as these are the natural hosts for this particular parasite (Dove & Fletcher, Reference Dove and Fletcher2000; Košuthová et al., Reference Košuthová, Šmiga, Oros, Barčák and Košuth2015; Oros et al., Reference Oros, Barčák, Bazsalovicsová and Hanzelová2015; Salgado-Maldonado et al., Reference Salgado-Maldonado, Matamoros, Kreiser, Caspeta-Mandujano and Mendoza-Franco2015; Kuchta et al., Reference Kuchta, Choudhury and Scholz2018).
The histopathological signs of S. acheilognathi in fish hosts are frequently extreme, with the parasite often killing its host (Liao & Shih, Reference Liao and Shih1956; Bauer et al., Reference Bauer, Musselius and Strelkov1969; Edwards & Hine, Reference Edwards and Hine1974; Scott & Grizzle, Reference Scott and Grizzle1979; Pool et al., Reference Pool, Ryder and Andrews1984; Schäperclaus et al., Reference Schäperclaus, Kulow and Schreckenbach1991; Brouder, Reference Brouder1999). In the present study, there was evidence of intestinal perforation and a large number (>600) of S. acheilognathi detected in each fish – far greater than previous studies, which have reported infection intensities of between two and 45 tapeworms (Scholz, Reference Scholz1997; Brouder, Reference Brouder1999; Košuthová et al., Reference Košuthová, Šmiga, Oros, Barčák and Košuth2015). In this study, the high intensity of tapeworms caused blockage and ischemia in parts of the intestines, resulting in intestinal perforations. The size of the tapeworms varied according to the size of the host and in one koi carp sample a single tapeworm measured over 400 mm in length. Over one quarter of the native fishes in south-western WA are listed as threatened with many very small in size (<100 mm total length) (Morgan et al., Reference Morgan, Unmack, Beatty, Ebner, Allen and Keleher2014). As with many parasitic interactions, the pathogenicity of S. acheilognathi increases in smaller hosts, with the potential to impact native fishes further. Gambusia holbrooki has been previously identified as a host for S. acheilognathi (Dove et al., Reference Dove, Cribb, Mockler and Lintermans1997; İnnal et al., Reference İnnal, Aydogdu and Guclu2016; McAllister et al., Reference McAllister, Bursey, Robison, Connior and Trauth2017). Gambusia holbrooki is already widespread throughout Australia and, because of its very large population sizes, represents a potential vector for further spread of the parasite (Morgan & Buttemer, Reference Morgan and Buttemer1996; Reynolds, Reference Reynolds2009). The discovery of S. acheilognathi in WA waters reinforces the importance of invasive fish control programs. Communication and education programs to the wider community are needed to help reduce the release of alien fishes into Australian waterways. It should also be noted that S. acheilognathi poses a potential health risk to humans. Although not generally considered zoonotic, there is one case study that identified a 32-year-old male who was initially diagnosed with Diphyllobothrium; however, molecular analysis of the DNA extracted from eggs in the patient's faeces identified them as S. acheilognathi, highlighting the importance of molecular identification techniques (Yera et al., Reference Yera, Kuchta, Brabec, Peyron and Dupouy-Camet2013). Future research investigations should also study other potential vectors, such as frogs, reptiles and birds (Kuchta et al., Reference Kuchta, Choudhury and Scholz2018), for the transmission of Schyzocotyle spp. in south-western WA. It is imperative that the potential spread and impacts of the invasion of S. acheilognathi be identified in future research to effectively control and manage the transmission of the parasite.
Supplementary material
To view supplementary material for this article, please visit https://doi.org/10.1017/S0022149X21000365.
Acknowledgements
We acknowledge the Whadjuk people of the Noongar nation, the Traditional Owners of the land on which this research was conducted. Our thanks to Prof Una Ryan, Prof Alan Lymbery and Dr Charlotte Oskam from Murdoch University, Perth, WA, for their assistance with initial edits on an early draft of the manuscript. We also thank Frances Brigg for technical support with Sanger sequencing at the Western Australian State Agricultural Biotechnology Centre (SABC), Perth WA, and David Chandler (AGRF) for providing valuable advice on PCR applications and technical support. We also thank the anonymous reviewers that edited the manuscript.
Financial support
This research has been co-funded by the City of Joondalup Shire (grant number 19619), the Australian Government's Department of Industry, Innovation and Science National Landcare Program (Environment Small Grants) and by Murdoch University. Cindy Palermo is supported by a Murdoch University Research Scholarship.
Conflicts of interest
None.
Ethical standards
The authors declare that the animals used in this research were euthanized in compliance with Animal Ethics Protocol ID. 401 (permit number R2949/17).
Author contributions
C.J.P., D.L.M. and S.J.B. contributed to the conception and design of the study, and acquisition of data. C.J.P., S.J.B. and A.E. analysed and interpreted the morphological data. C.J.P. and T.L.G. analysed and interpreted the molecular data. C.J.P. drafted the article, and D.L.M., S.J.B., A.E. and T.L.G. critically revised the manuscript. All authors approved the final version of the manuscript submitted for publication.