Introduction
Chronic hypoxia is a common etiological factor for pulmonary hypertension in fetuses, newborns, and adults.Reference Abman1–Reference Gao and Raj4 The fetal and neonatal lung is more vulnerable to chronic hypoxia than the adult lung.Reference Morrison, Berry and Botting5 The pathophysiological changes that occur during exposure to chronic hypoxia result in failure of the pulmonary vasculature to dilate adequately and decrease the arterial wall thickness after birth, particularly the small-resistance pulmonary arteries (SPA), leading to persistent pulmonary hypertension and hypoxemia.Reference Abman1,Reference Gao and Raj4 The prevalence of pulmonary arterial hypertension in neonates (PAHNs) is 1.9–7.0 per 1000 live births in lowlands,Reference Walsh-Sukys, Tyson and Wright6 with few data available at high altitude. For instance, a study conducted in La Paz, Bolivia (3600 m, 0.64 atm) showed that respiratory complications (respiratory distress syndrome) can increase by about 10% in comparison to lowlands.Reference Keyes, Armaza and Niermeyer7 A study performed at high altitude in China concluded that environmental hypoxia can aggravate the development of pulmonary hypertension, estimated at 8% of live births.Reference Li, Liu and Xie8 Therefore, this is a relevant health issue, considering that there are an estimated 170 million permanent residents at altitudes >2500 m, mainly on the Tibetan, Andean, and East African plateaus.Reference Moore, Shriver and Bemis9
The concept of “Developmental (or Fetal) Origins of Adult Health and Disease” has been receiving much attention for over two decades and has been supported by numerous studies.Reference Ducsay, Goyal and Pearce10 Among other environmental factors that affect fetal development, hypoxia is an important cause of fetal stress, impacting developmental plasticityReference Ducsay, Goyal and Pearce10–Reference Alexander, Dasinger and Intapad12 with phenotypic changes that predispose offspring to various dysfunctions and diseases.Reference Kubota, Miyake, Hariya and Mochizuki13 Antenatal hypoxia is an essential condition concerning maternal/fetal stresses and developmental programming of chronic diseases later in life.Reference Barker and Osmond14 The last decade has brought an explosion of interest in the epigenome, generally defined as changes to chromatin structure and function that do not involve altering the DNA sequence. Epigenetic control is critical to both normal homeostasis and disease.Reference Keating and El-Osta15 The main epigenetic mechanisms that mediate modulation in gene expression are DNA methylation, histone modifications, and micro RNAs. These mechanisms regulate the expression and function of several genes, including ion channels, membrane receptors, key enzymes, and signaling proteins. These actions have been shown in maternal and fetal organs and tissues.Reference Goyal, Limesand and Goyal16 Most of the epigenetic responses and mechanisms involved have been determined in animal experimental models.
Some of the most commonly used mammalian models of antenatal and perinatal hypoxia include sheep,Reference Gao and Raj4,Reference Papamatheakis, Blood, Kim and Wilson17–Reference Gonzaléz-Candia, Candia and Figueroa23 rats,Reference Ziino, Ivanovska and Belcastro24 mice,Reference Bierer, Nitta and Friedman25 piglets,Reference Hirenallur, Haworth and Leming26 guinea pigs,Reference Morrison, Berry and Botting5,Reference Murphy, Aronovitz and Reid27,Reference Herrera, Alegría and Farias28 and llamas.Reference Giussani, Riquelme, Sanhueza, Hanson, Blanco and Llanos29–Reference Reyes, Díaz and Ebensperger32 Sheep have been used as a model for lung development and pulmonary function for several reasons. First, stages of lung development in sheep are like those in humans and occur with similar timing in relation to birth.Reference Morrison, Berry and Botting5 Second, several studies on the pulmonary fetal to neonatal transition support the lamb as a good paradigm for perinatal cardiorespiratory transition in newborn humans.Reference Hillman, Kallapur and Jobe33 Third, the available data on fetal lung fluid volume, secretion, composition, and flow dynamics have been obtained from sheep and seem to represent the human characteristics.Reference Hillman, Kallapur and Jobe33 The similarities between these species in the establishment of pulmonary function by the newborn over several hours after birth allow the study of particular neonatal pulmonary disorders.Reference Papamatheakis, Blood, Kim and Wilson17,Reference Llanos, Ebensperger and Herrera19 Finally, the sheep has proven to be an excellent model for chronic preparations for maternal, placental, fetal, and neonatal vascular function studies. This allows the implantation of vascular catheters and flow probes to determine blood pressure and flow in different vascular beds under diverse conditions such as hypoxia and oxidative stress (OS).Reference Llanos, Ebensperger and Herrera19,Reference Herrera, Krause and Ebensperger34,Reference Torres, González-Candia and Montt35 The little information obtained from humans and other species is generally consistent with data from sheep.Reference Morrison, Berry and Botting5
Animal models offer a means of gaining better understanding of the fundamental basis of the disease. A number of experimental animal models are being used to generate pulmonary arterial hypertension in the fetus and newborn. The aim of this review is to highlight the advances in fetal and neonatal programming of pulmonary vascular function, using the sheep as a translational model of chronic hypoxia-induced pulmonary hypertension (Tables 1 and 2).
Table 1. Studies on lambs with neonatal pulmonary hypertension of the neonate
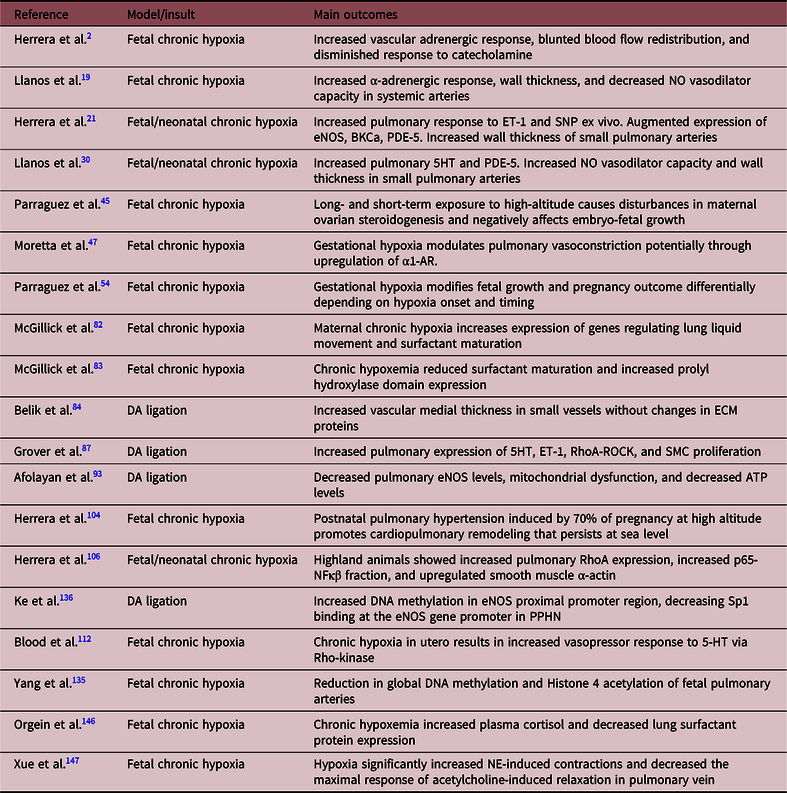
NO, nitric oxide; BKCa, large conductance calcium-activated potassium channels; PDE-5, phosphodiesterase-5; α1-AR, α1-adrenergic receptor; ATP, adenosine triphosphate; RhoA, Ras homolog family member A; PPHN, persistent pulmonary hypertension of the neonate.
Table 2. Studies with pharmacological interventions on lambs with neonatal pulmonary hypertension of the neonate
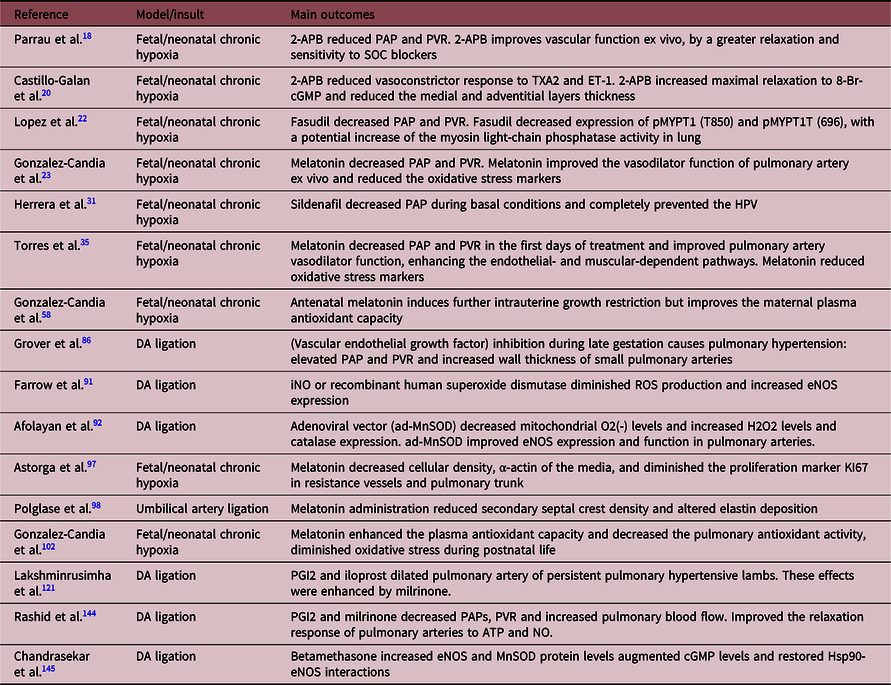
SOC, store-operated channels; iNO, inhaled NO; ATP, adenosine triphosphate; MnSOD, manganese superoxide dismutase; Hsp90, heat shock protein 90.
Fetal and neonatal cardiovascular response to chronic hypoxia
The fetal cardiovascular response to acute hypoxia has been well described in large animal models such as the sheep in the last 3–4 decades, using the fetal instrumentation preparation.Reference Cohn, Sacks, Heymann and Rudolph36–Reference Rudolph38 Fetal acute hypoxia induces an increase in pulmonary vascular resistance (PVR) and therefore reduces lung blood flow, as in other peripheral areas, prioritizing O2 flow to “vital” organs such as the brain, heart, and adrenals.Reference Cohn, Sacks, Heymann and Rudolph36 In marked contrast, the fetal response under chronic hypoxia is less studied, but a few studies suggest that this O2 distribution response is blunted.Reference Herrera, Rojas and Krause21,Reference Llanos, Green, Creasy and Rudolph39–Reference Shaw, Allison and Itani41 One of the most appropriate conditions to obtain fetal chronic hypoxia is exposure to high altitude during pregnancy.Reference Herrera, Pulgar and Riquelme2,Reference Papamatheakis, Blood, Kim and Wilson17,Reference Herrera, Rojas and Krause21,Reference Herrera, Ebensperger and Krause31,Reference Herrera, Krause and Ebensperger34 The sheep has been used as a model that represents an animal not adapted to high altitude, such as the human species.Reference Herrera, Rojas and Krause21,Reference Llanos, Riquelme and Sanhueza30,Reference Edea, Dadi, Dessie and Kim42–Reference Myers and Ducsay49
Pregnant women and their unborn babies from several regions of the world are chronically exposed to the low-oxygen milieu of high-altitude mountains or plateaus.Reference Herrera, Rojas and Krause21,Reference Wang, Zhang and Zhang50,Reference Browne, Julian and Toledo-Jaldin51 Pregnancy at high altitude is clearly a potential burden for both mother and fetus. The incidence of pregnancy complications and neonatal morbidity such as intrauterine growth restriction, fetal hypoxia, and respiratory distress in neonates is significantly increased in high-altitude populations.Reference Keyes, Armaza and Niermeyer7,Reference Giussani, Phillips, Anstee and Barker52,Reference Giussani and Davidge53 Pregnancy at high altitude induces impaired fetal sheep growth, leading to reduced fetal weight, brain, liver, and uteroplacental efficiency.Reference Herrera, Pulgar and Riquelme2,Reference Herrera, Rojas and Krause21,Reference Llanos, Riquelme and Sanhueza30,Reference Parraguez, Atlagich and Díaz54–Reference Gonzalez-Candia, Veliz and Araya58 Pregnancy induces a significant decrease in mean systemic arterial pressure in low-altitude sheep, while this adaptation is absent in animals acclimatized to high-altitude hypoxia, leading to increased systemic arterial pressure in high-altitude pregnant sheep relative to low-altitude animals.Reference Ducsay, Goyal and Pearce10
Chronic materno-fetal hypoxia occurs in human populations that live in hypobaric hypoxia at altitudes above 2500 m.Reference Moore, Shriver and Bemis9,Reference Herrera, Rojas and Krause21,Reference Moore, Niermeyer and Zamudio59,Reference Longo and Pearce60 Several researchers reported a decrease in birth weight related to an increase in altitude.Reference Keyes, Armaza and Niermeyer7,Reference Lichty61–Reference Mortola, Frappell, Aguero and Armstrong65 Chronic hypoxia, as observed in exposure to high altitude, is associated with pulmonary hypertension.Reference Penaloza and Arias-Stella66,Reference Niermeyer, Andrade Mollinedo and Huicho67
Intrauterine chronic hypoxia favors fetal pulmonary vascular remodeling, excessive proliferation and impaired permeability, and metabolism of endothelial and smooth muscle tissue, as well as intimal fragmentation.Reference Gao and Raj4,Reference Jeffery and Morrell68 There is also proliferation and trans-differentiation of fibroblasts with collagen deposits in the interstitium.Reference Gao and Raj4,Reference Jeffery and Morrell68,Reference Durmowicz and Stenmark69 This produces small pulmonary vessels with a thickened middle layer and marked fibroproliferative changes in the adventitia.Reference Gao and Raj4,Reference Stenmark, Fagan and Frid70 In addition to the effects on the pulmonary vessels, pulmonary hypertension from chronic exposure to hypobaric hypoxia is accompanied by right ventricular hypertrophy, polycythemia, and in some cases right heart failure.Reference Li, Liu and Xie8,Reference Penaloza and Arias-Stella66
In Chinese Han children, an ethnic group exposed to the Himalayan altitudes for less than 100 years (Lhasa, 3658 m), chronic pulmonary hypertension and decreased oxygen saturation due to environmental hypoxia play a central role in the pathogenesis of subacute high-altitude disease.Reference Niermeyer, Yang, Zhuang and Moore64 Surprisingly, congenital heart disease is 20 times higher relative to the lowland population.Reference Li, Liu and Xie8 In addition, the prevalence of PAHN rises to 8% in the Chinese population living above 2500 m.
In contrast, children of the Tibetan population, who have inhabited the same altitudes for 25,000 years, do not present this clinical picture. It is thus presumed that genetic adaptations have conferred resistance to pulmonary hypertension caused by hypobaric hypoxia.Reference Li, Liu and Xie8,Reference Niermeyer, Yang, Zhuang and Moore64 Tibetan populations have elevated pulmonary nitric oxide (NO) production through endothelial NO synthase (eNOS) upregulation, a response that may be part of the acclimatization process.Reference Beall, Laskowski and Strohl71 Secondarily, decreased NO levels are associated with high-altitude pulmonary edema susceptibility in humans, which further indicates the importance of NO signaling and acclimatization to high altitude.Reference Duplain, Sartori and Lepori72,Reference Sartori, Rimoldi and Duplain73 However, in the Andean area, newborn children in Morococha, Peru (4540 meters above sea level) have marked pulmonary hypertension and persistent ductus arteriosus (DA), even at 72 h of postnatal life.Reference Gamboa and Marticorena74 This pulmonary hypertension is also present in children aged 5–14 years.
Fetal pulmonary vascular response to chronic hypoxia in sheep
Studies on the pulmonary vasoactive response in sheep fetuses gestated in chronic hypoxia were carried out in studies reviewed by Llanos et al, in which they compared the vasoactive response of llama fetuses (an animal acclimated to chronic hypoxia) and sheep fetuses.Reference Llanos, Ebensperger and Herrera19 The llama fetus has several physiological adaptations, among them a marked alpha-adrenergic functionReference Herrera, Alegría and Farias28,Reference Llanos, Riquelme, Moraga, Cabello and Parer75 responsible for the high peripheral vascular resistanceReference Llanos, Ebensperger and Herrera19 and higher plasma catecholamine concentrations compared to those found in the fetal sheep. The authors highlighted the crucial role that the sympathetic system plays in the regulation of the fetal llama systemic circulation.Reference Llanos, Ebensperger and Herrera19,Reference Giussani, Riquelme, Sanhueza, Hanson, Blanco and Llanos29 Since the alpha-adrenergic mechanisms are upregulated in fetal llama systemic circulation, the contractile responses of SPA to norepinephrine (NE) are decreased in the llama fetus compared to the sheep fetus, with an increase in the maximal response at very high NE concentration. In addition, the sensitivity to the NO-dependent relaxation is also greater in fetal llama than in fetal sheep SPA.Reference Llanos, Ebensperger and Herrera19 Together, these data show that the fetal llama has higher sensitivity to a vasodilator (NO) and lower sensitivity to a vasoconstrictor (NE).
Low PO2 is a main determinant of the increase in PVR through acute inhibition of potassium currents and downregulation of K+ channel expression under long-term exposure.Reference Weir and Archer76 In subacute or chronic hypoxia, the hypoxia inducible factor (HIF) induces transcription of vasoconstrictor factors such as endothelinReference Franco-Cereceda and Holm77–Reference Simonneau, Galiè and Rubin80 and α1B receptors,Reference Herrera, Alegría and Farias28,Reference Ruijtenbeek, le Noble and Janssen81 favoring the vasoconstrictor effects. Although eNOS expression may be induced, its activity depends on oxygen availability and an adequate oxidative tone, decreasing NO synthesis and function. Chronic hypoxia also impairs lung maturation, affecting hypoxic signaling pathways, surfactant maturation, lung liquid movement, and oxidative tone determinants.Reference McGillick, Orgeig and Allison82 Each of the effects on vasoconstrictor or vasodilator signaling, lung maturation and oxidative balance are dependent on the timing of the hypoxic onset and its extension.Reference McGillick, Orgeig, Giussani and Morrison83 The airway maturational processes will also affect the postnatal vascular response as these are modulated by oxygen. At high altitude, the above mechanisms that restrict pulmonary vasodilation at birth likely prevail, and the resultant elevated PVR causes the fetal circulation to persist, inducing a right to left shunt, slowing the DA closure and leading to hypoxemia and pulmonary hypertension of the newborn.Reference Herrera, Rojas and Krause21
Pulmonary vascular remodeling during the antenatal period
The pulmonary remodeling induced by chronic hypoxia has been observed in sheep models by DA ligation in uteroReference Belik, Keeley, Baldwin and Rabinovitch84 and gestation under chronic hypoxia, both in sheep.Reference Herrera, Rojas and Krause21 Fetal pulmonary vasculature apparently undergoes less remodeling following hypertension by DA ligation relative to chronic hypoxia by altitude. Fetal sheep pulmonary hypertension induced by DA ligation results in increased medial thickness only in small acinar vessels, while the elastin and collagen contents of larger vessels expressed either per tissue weight or per DNA were not different from their controls.Reference Belik, Keeley, Baldwin and Rabinovitch84 These researchers found no evidence of altered connective tissue deposition in smaller resistance arteries. Dodson et al., in a model of fetal pulmonary hypertension by DA occlusion, found that the proximal main pulmonary artery (MPA) of fetal sheep has increased stiffness and altered extracellular matrix (ECM) remodeling compared to fetal normoxic controls.Reference Dodson, Morgan, Galambos, Hunter and Abman85 MPA thickening occurs with decreased elastin in the media and increased collagen deposition in the adventitia, suggesting that the ECM in the MPA is more fibrotic in this experimental model of pulmonary hypertension. In summary, the MPA of fetal sheep with pulmonary hypertension has increased stiffness and extensive ECM remodeling. This is characterized by a thin media but thicker adventitia, depending on collagen content and fiber orientation relative to controls.Reference Dodson, Morgan, Galambos, Hunter and Abman85–Reference Grover, Parker, Balasubramaniam, Markham and Abman87 These findings suggest that in addition to changes in the distal pulmonary arteries, proximal remodeling and stiffness of the pulmonary circulation may further contribute to impaired hemodynamics of pulmonary hypertension in the newborn (Fig. 1).

Fig. 1. Schematic outlining factors in the molecular pathogenesis of neonatal pulmonary arterial hypertension. The scheme summarizes the main results obtained from chronic hypoxic fetal and neonatal sheep as models of neonatal pulmonary hypertension. The mechanisms shown are responsible for EC dysfunction, proliferation of SMCs, and oxidative stress, associated with sustained vasoconstriction of the pulmonary arteries. Abbreviations: PDE-5, phosphodiesterase-5.
Pulmonary hypoxia and OS during antenatal hypoxia
Another mechanism by which chronic hypoxia induces damage is as a result of the increased generation of reactive oxygen species (ROS) by incomplete reduction of oxygen. ROS are free radicals produced as byproducts of oxidation–reduction reactions. There are various intracellular enzymatic pathways that produce ROS in mammals, such as mitochondrial electron transport, NADPH (nicotinamide adenine dinucleotide phosphate) oxidase, the cytochrome P450 monooxygenase system, xanthine oxidase, NO synthases, cyclooxygenase, and lipoxygenase.Reference Simon88
Fetal OS represents a strong candidate mechanism for insults associated with a persistent increase of ROS in the offspring in humans and animals.Reference Herrera, Krause and Ebensperger34,Reference Franco Mdo, Dantas and Akamine89 ROS cause endothelial dysfunction and facilitate hypoxic pulmonary vasoconstriction in experimental animal models (Fig. 1).Reference Hoshikawa, Ono and Suzuki90 In fact, several studies have linked increased ROS with neonatal pulmonary hypertension and have proposed antioxidant treatments.Reference Gonzaléz-Candia, Candia and Figueroa23,Reference Torres, González-Candia and Montt35,Reference Farrow, Lakshminrusimha and Reda91–Reference Astorga, González-Candia and Candia97 For instance, melatonin treatment as an antioxidant, either antenatalReference Polglase, Barbuto and Allison98–Reference Gonzalez-Candia, Veliz and Carrasco-Pozo102 or postnatal,Reference Gonzaléz-Candia, Candia and Figueroa23,Reference Torres, González-Candia and Montt35,Reference Astorga, González-Candia and Candia97 has been successfully studied using sheep models (Table 2).
Exaggerated ROS during the fetal period may induce endothelial dysfunction in the offspring by causing epigenetically induced alterations of the expression of genes involved in the regulation of endothelial function (Fig. 2).Reference Sartori, Rimoldi and Duplain73

Fig. 2. Potential epigenetic mechanisms in the pathogenesis of neonatal pulmonary arterial hypertension. These epigenetic changes may result in excessive vasoconstriction and pulmonary vascular remodeling, which are responsible for the initiation and progression of pulmonary hypertension. The three major epigenetic hallmarks are DNA methylation, histone modifications, and regulation by miRNAs, leading to altered gene expression in pulmonary EC and SMC. Abbreviations: AcH4, acetylated histone H4; H4K12ac, acetylation of lysine 12 on histone H4; H3K9me, methylation of histone H3K9.
Neonatal pulmonary vascular response to chronic hypoxia in sheep
Pulmonary circulation normally undergoes important structural and functional changes during the perinatal period that allow the sudden transition from placental to pulmonary gas exchange. At birth, there is normally a significant increase in PaO2 when the newborn starts breathing. The brisk increase in alveoli PO2 increases NO synthesis, decreases endothelin type-A receptor (ETA) expression, and removes the hypoxic stimulus for hypoxic pulmonary vasoconstriction (HPV), which together reduce PVR.Reference Papamatheakis, Blood, Kim and Wilson17 Increases in arterial PO2 also facilitate DA closure, increasing cardiac output and pulmonary blood flow.Reference Rudolph103 Perinatal hypoxia impairs these processes, as illustrated in a hypoxic newborn sheep model where the PVR falling at birth is blunted, maintaining high pulmonary arterial pressure (PAP) and permeable DA.Reference Llanos, Ebensperger and Herrera19,Reference Herrera, Ebensperger and Krause31,Reference Herrera, Riquelme and Ebensperger104 This is associated with an enhanced HPV response in antenatal hypoxia-exposed sheep born at 3600 m in the INCAS Research Station (Putre, Chile).Reference Herrera, Pulgar and Riquelme2,Reference Llanos, Riquelme and Sanhueza30 In this model, the newborn lamb has stronger pulmonary vascular responses to acute hypoxia associated with higher arterial contractile status and an increased wall thickness in the small pulmonary arteries compared to low-altitude controls.Reference Herrera, Ebensperger and Krause31
Mechanisms of perinatal pulmonary vasoconstriction
HPV is an intrinsic property of the pulmonary vasculature that allows adequate ventilation to perfusion matching in the lung. During the prenatal period, the HPV response helps to maintain high PVR in the lung that diverts blood to a low-resistance vascular territory through the fetal vascular intercommunications (DA and foramen ovale). However, chronic perinatal hypoxia can exacerbate the hypoxia-induced pulmonary vasocontractile response.Reference Herrera, Pulgar and Riquelme2,Reference Papamatheakis, Blood, Kim and Wilson17,Reference Herrera, Riquelme and Ebensperger104
Multiple mechanisms contribute to the enhanced vasoconstrictor tone of SPA of the chronic hypoxic lamb. For instance, SPA from lambs gestated and born at high altitude show an increased response to potassium (KCl).Reference Herrera, Pulgar and Riquelme2,Reference Herrera, Ebensperger and Krause31 This may be the result of the increased contractile capacity, due to either increased smooth muscle components, increased activity of voltage-dependent Ca2+ channels and/or increased Ca2+ sensitivity in smooth muscle cells (SMC). Pulmonary vascular remodeling has been observed in hypoxic newborn sheep and is discussed later in this review.
Regarding the Ca2+ sensitivity of vascular smooth muscle contraction, this response is negatively regulated through NO-cyclic guanosine monophosphate-PKG (cGMP-dependent protein kinase) signaling, while it is positively regulated by RhoA–Rho kinase, among other factors.Reference Jernigan and Resta105 In high-altitude sheep with pulmonary hypertension, the enhancement of nitrergic vasodilator tone does not avoid pulmonary hypertension,Reference Herrera, Ebensperger and Krause31,Reference Herrera, Riquelme and Ebensperger104 probably because there is also increased expression and activation of RhoA that allows the maintenance of a strong Ca2+ sensitivity.Reference Lopez, Ebensperger and Herrera22,Reference Herrera, Ebensperger, Hernández, Sanhueza, Llanos and Reyes106 This response contrasts with the response observed in newborn of species resistant to hypoxic pulmonary hypertension like the llama, where the increased nitrergic together with decreased Ca2+ sensitization allows effective vasodilation.Reference Reyes, Díaz and Ebensperger32 In addition to Ca2+ sensitivity, intracellular Ca2+ concentration is an important mediator of the pulmonary arterial response to hypoxia, and Ca2+ entry through voltage-dependent or voltage-independent plasma membrane Ca2+ channels are major regulators. Voltage-dependent Ca2+ channels are important mediators of pulmonary vascular contraction in healthy vessels of adult animals, while voltage-independent Ca2+ channels of the family of receptor- or store-operated Ca2+ channels are involved in the pathological response of pulmonary vessels to hypoxia in both adult and newborn animals and humans.Reference Reyes, Castillo-Galán, Hernandez, Herrera, Ebensperger and Llanos107 Store-operated Ca2+ channels are involved in the HPV response of both low- and high-altitude lambs; their function and expression are enhanced in high-altitude lambs.Reference Parrau, Ebensperger and Herrera18 The treatment of pulmonary hypertensive lambs with the non-specific store-operated channel blocker 2-aminoethoxydiphenyl borate (APB) also significantly decreases PAP and resistance, suggesting that these channels are important in the maladaptive response of the pulmonary circulation to chronic hypoxia.Reference Castillo-Galán, Quezada and Moraga20 The voltage-dependent potassium channels (Kv) are an important component of the membrane depolarization process.Reference Shimoda, Sylvester and Booth108 This Kv channel activity tends to maintain a negative resting membrane potential and is important in the vasocontractile response to acute hypoxia.Reference Shimoda, Sylvester and Booth108,Reference Sylvester, Shimoda, Aaronson and Ward109 Suppressing Kv channel activity depolarizes the membrane, which elicits Ca2+ signals that cause SMC contraction.Reference Sylvester, Shimoda, Aaronson and Ward109
Serotonin (5-HT) is another mediator involved in hypoxic vasoconstriction. Several studies have shown a role for 5-HT in the regulation of acute and chronic hypoxic responses, particularly as they relate to contraction and growth of SMC.Reference MacLean, Deuchar and Hicks110,Reference Long, MacLean and Jeffery111 5-HT is a vasoactive, and in some SMCs, a mitogenic molecule released from platelets, pulmonary neuroendocrine cells, and endothelial cells (ECs). Plasma 5-HT levels increase under hypoxic conditions in a sheep model of persistent pulmonary hypertension of the newborn (PPHN).Reference Papamatheakis, Blood, Kim and Wilson17,Reference Blood, Terry and Merritt112 5-HT synthesis in pulmonary ECs is increased in experimental PPHN, and both tryptophan hydroxylase 1 (Tph1) and the 5-HT2A receptor expressions are increased in this model.Reference Delaney, Gien, Roe, Isenberg, Kailey and Abman113 These findings provide evidence that 5-HT contributes to an elevated pulmonary vascular tone (Fig. 1).
Thromboxane (TXA2) and endothelin-1 (ET-1) are other important pathways that contribute to the pulmonary vasoconstrictor phenotype associated with chronic hypoxia. These vasoactive agents act through the transduction of signals dependent on protein G (metabotropic receptors), increase the bioavailability of Ca2+, and ultimately the signals come together and enhance the phosphorylation and activity of the myosin light chain.Reference Stenmark, Frid, Graham and Tuder114 Vasoconstriction is achieved via the phosphorylating effect of myosin light-chain kinase on myosin and its interaction with actin. In pulmonary arteries of newborn sheep, chronic hypoxia reduces the synthesis of prostacyclin (PGI2), a strong vasodilator prostanoid, but not that of TXA2, a vasocontractile prostanoid.Reference Stenmark, Frid, Graham and Tuder114 This leads to increased vasoconstriction due to a decreased ratio of PGI2 to TXA2.Reference Fike, Kaplowitz, Zhang and Pfister115 Chronic hypoxia has been found to increase the sensitivity and reactivity of neonatal pulmonary arterial myocytes of neonatal pigs to the TXA2 agonist.Reference Gao and Raj4
ET-1 causes pulmonary vasoconstriction primarily through activation of ETA; activation of endothelin receptor type B (ETB) can attenuate this effect because of its high expression in vascular endothelium.Reference Trow and Taichman116 ET-1 signaling has received significant attention in the setting of increasing levels in lung vasculature due to acute hypoxia and elevated PAP.Reference Papamatheakis, Blood, Kim and Wilson17 It is also thought to induce pulmonary artery SMC mitogenesis and is likely involved in hypoxia-induced pulmonary vascular remodeling. Using ovine pregnancy at high altitude as an experimental model and following return to sea level of the neonate, Herrera et al. showed that pregnancy in the highlands programs lung function of the neonates, increasing ET-1 sensitivity and vasoconstriction in SPA.Reference Herrera, Riquelme and Ebensperger104
The RhoA/Rho-kinase (ROCK) pathway also participates in pulmonary vasoconstriction in several species such as rat, mouse, and sheep, contributing to pulmonary vascular remodeling and vasoconstriction by Ca2+ sensitization.Reference Lopez, Ebensperger and Herrera22 Fasudil and Y-27632, ROCK-specific inhibitors, reduced adult and fetal hypoxic pulmonary contraction.Reference Fagan, Oka and Bauer117,Reference Broughton, Walker and Resta118 Treatment with Fasudil lessens the development of chronic hypoxic PAHN in newborn lambs.Reference Lopez, Ebensperger and Herrera22
Mechanisms of perinatal pulmonary vasodilatation
The pulmonary vasculature dilates when SMCs relax, which is primarily due to inhibition of vasoconstrictor pathways. The endothelium acts as a major vasodilator promoter.Reference Papamatheakis, Blood, Kim and Wilson17 For instance, agonist stimulation of ECs by acetylcholine or other factors causes increases in endothelial cytosolic Ca2+ that activates several pathways, leading to the generation of NO and PGI2.Reference Tirosh, Resnik, Herron, Sukovich, Hong and Weir119
The pathways associated with NO-dependent vasorelaxation have been intensively studied in the perinatal vasculature. NO is generated predominantly by eNOS, an enzyme expressed in vascular endothelium and stimulated by increases in endothelial cytosolic Ca2+. Diminished eNOS activity is thought to be an important determinant for development of persistent pulmonary hypertension in the newborn and pulmonary hypertension by chronic hypoxia.Reference Llanos, Ebensperger and Herrera19 In contrast, eNOS protein expression and activity are increased in sheep born and studied in highlands.Reference Herrera, Reyes and Giussani120 However, phosphodiesterase-5 expression is increased, generating a decreased NO-dependent vasodilation (Fig. 1).Reference Llanos, Ebensperger and Herrera19In vivo studies with endogenous NO blockade have shown that NO function is enhanced at high altitude, but not enough to prevent pulmonary hypertension.Reference Herrera, Ebensperger, Hernández, Sanhueza, Llanos and Reyes106 The latter was associated with reduced soluble guanylate cyclase (sGC) protein expression at high altitude in comparison to newborn lambs born in low altitude, suggesting a decrease in cGMP and therefore vasodilation.Reference Herrera, Reyes and Giussani120 Pulmonary vasodilator responses to exogenous NO from sodium nitroprusside (SNP) administration were diminished in SPA from neonatal lambs exposed to chronic hypoxia.Reference Herrera, Ebensperger and Krause31 In contrast, lambs born at high altitude that returned to sea level had accentuated vasodilation with SNP,Reference Herrera, Riquelme and Ebensperger104 showing that the NO response depends on the timing and extension of hypoxia exposure.
PGI2 is the predominant PGI2 released from the endothelium; along with some other products of arachidonic acid metabolism, it may provide protection from chronic hypoxic stress.Reference Gao and Raj4 These endothelial-derived substances dilate vessels and attenuate proliferation in SMC. PGI2 are released from the vascular endothelium and activate Gs-coupled GPCRs (G protein-coupled receptors) in SMCs.Reference Papamatheakis, Blood, Kim and Wilson17 Chronic hypoxia reduces the production of PGI2 in neonatal pulmonary vasculature. Lakshminrusimha et al. showed that lung tissue from ductal-ligation lambs has reduced PGI2-induced relaxation as a result of decreased PGI2 receptor levels.Reference Lakshminrusimha, Porta and Farrow121
Pulmonary Vascular Remodeling during the perinatal period
Pulmonary arterial wall thickening and muscularization are important features of hypoxic pulmonary hypertension in the newborn, since these increase PVR.Reference Gao and Raj4,Reference Pak, Aldashev, Welsh and Peacock122 Structural changes include the appearance of SM (smooth muscle)-like cells (as defined by the expression of α-SM-actin) in previously non-muscularized vessels of the alveolar wall.Reference Stenmark, Frid, Graham and Tuder114 The remodeling involves the intimal, medial, and adventitial compartments of the pulmonary arterial and venous vessel wall and often the perivascular space.Reference Abman1 The medial thickening is attributable to hypertrophy and increased accumulation of SMCs as well as increased deposition of ECM proteins, predominantly collagen and elastin. Herrera et al. demonstrated that exposure to chronic hypoxia results in a histological fetal pattern of thickened media and adventitia in newborn sheep (Fig. 1).Reference Herrera, Ebensperger and Krause31 The adventitial thickening is caused by accumulation of fibroblasts and myofibroblasts, and there is an increase in ECM accumulation (collagens, elastin, fibronectin, and tenascin).Reference Stenmark, Frid, Graham and Tuder114 These activated fibroblasts and myofibroblasts contribute to the structural remodeling of the vessel through increased production of collagen and other ECM proteins, including fibronectin and elastin.Reference Frid, Brunetti and Burke123 Myofibroblast accumulation can also contribute to the abnormalities of smooth muscle vascular tone observed in chronic hypoxic pulmonary hypertension. The contractile properties of myofibroblasts appear to be different from those of SMCs, with a slower onset of contraction in response to vasoactive stimuli and a failure to relax in response to vasodilating stimuli.Reference Desmouliere, Chaponnier and Gabbiani124 In newborn sheep with partial or total gestation at high altitude, blockade of the store-operated Ca2+ channel with 2-APB or blockade of Rho-kinase with Fasudil decreases both PVR and remodeling. Fasudil decreases the medial layer area, while 2-APB decreases both medial and adventitial layers of pulmonary arteries of hypertensive animals, suggesting that this remodeling is in part regulated by Rho-kinases and store-operated Ca2+ entry in the ovine model.Reference Castillo-Galán, Quezada and Moraga20,Reference Lopez, Ebensperger and Herrera22 Collectively, these observations suggest that chronic hypoxia induces changes in the pulmonary parenchyma that contribute to the elevated maintenance of RVP (right ventricular pressure) in models of neonatal pulmonary hypertension.
Pulmonary OS during perinatal hypoxia
ROS such as superoxide anions and hydrogen peroxide have been implicated in cell proliferation of various cell types.Reference Gao and Raj4,Reference Bedard and Krause125 Production of ROS in the lung and pulmonary vasculature is stimulated not only by hypoxia but also by various vasoconstrictive and mitogenic agents that are elevated during hypoxia such as ET-1, HIF-1α, and TGF-β (Transforming growth factor beta).Reference Ismail, Sturrock and Wu126–Reference Wu, Li and Xu128 There are currently models that relate ROS generation and vasoconstriction in pulmonary hypoxia. In the first model, the so-called redox hypothesis, the mitochondrial electron transport chain (ETC) senses hypoxia and decreases ROS production (ROS being produced as an inevitable byproduct of oxidative phosphorylation), causing intracellular calcium influx secondary to redox-sensitive potassium channel inhibition.Reference Delaney, Gien, Roe, Isenberg, Kailey and Abman113,Reference Bonnet and Boucherat129 In support of this, Michelakis et al. demonstrated that hypoxia and ETC inhibitors suppress the production of activated O2 species and increase the vasoconstriction of ex vivo pulmonary arteries.Reference Michelakis, Hampl and Nsair130 The second model is challenged by the ROS hypothesis, which proposes that hypoxia increases mitochondrial ROS generation, mediating elevation of intracellular calcium concentration.Reference Sylvester, Shimoda, Aaronson and Ward109 This theory is supported by studies showing that antioxidants such as melatonin attenuated the contractile response in lungs from sheep neonates with pulmonary hypertension,Reference Torres, González-Candia and Montt35,Reference Gonzalez-Candia, Veliz and Araya58 and exogenous H2O2 induces contraction in cultured pulmonary artery SMCs in isolated lungs.Reference Waypa, Marks and Mack131 Gonzalez-Candia et al. showed that melatonin treatment as an antioxidant decreased the vasoconstriction associated with TXA2 in SPA in a model of pulmonary hypertension due to chronic hypoxia (Fig. 1).Reference Gonzalez-Candia, Veliz and Araya58 These authors also demonstrated that melatonin decreased the vasoconstriction associated with ET-1 and increased pulmonary tissue expression of the endothelial ETB receptor, decreasing the vasoconstrictive action of ET-1 (Fig. 1).Reference Gonzalez-Candia, Veliz and Araya58 Melatonin also decreased the mean PAP by an increased expression of the PGI2-dependent pathway, increasing the expression of PGI2 synthase and PGI2 receptor in neonatal pulmonary parenchyma.Reference Gonzalez-Candia, Veliz and Araya58,Reference Aguilar, Arias and Canquil132
Astorga et al. demonstrated that melatonin treatment also decreases media cell density and SMC proliferation (reduced percentage of Ki-67 positive cells), showing a potential antiproliferative effect. However, it decreases α-actin and smoothelin-β pulmonary expression, which are SMC markers in sheep neonates with HPN (pulmonary hypertension of the newborn).Reference Astorga, González-Candia and Candia97 These studies demonstrate that OS plays important roles in the mechanisms determining arterial reactivity and pulmonary remodeling induced by chronic hypoxia.
Epigenetic mechanisms associated with pulmonary vascular programming
Pulmonary arterial hypertension by chronic hypoxia is a progressive disease characterized by a hyperproliferative, antiapoptotic and inflammatory phenotype of pulmonary vascular cells, which leads to excessive vasoconstriction and abnormal vascular remodeling.Reference Humbert, Guignabert and Bonnet133 Adverse intrauterine conditions such as undernutrition and hypoxia can program cardiovascular cell and organ function by epigenetic mechanisms.Reference Hanson and Gluckman134 The three most relevant mechanisms known to regulate gene transcription epigenetically are DNA methylation, histone modifications, and regulation by noncoding RNA.
DNA Methylation
DNA methylation is the most studied epigenetic mechanism. It occurs principally in the promoter or enhancer region of genes, specifically in cytokines adjacent to guanosine, the so-called CpG island.Reference Goyal, Limesand and Goyal16 DNA hypomethylation is associated with overexpression of the gene, whereas hypermethylation is associated with reduced gene expression.Reference Goyal, Limesand and Goyal16 Yang et al. reported a reduction in global DNA methylation in pulmonary arteries from fetal lambs exposed to high altitude.Reference Yang, Lu, Ramchandran, Longo and Raj135 The authors described a gene inactivation by methylation in the promoter region of p21 protein, involved in cell arrest. Therefore, chronic hypoxia modulates the epigenome of pulmonary arteries, decreasing p53-p21 expression and increasing the proliferative phenotype of SMC in fetal lungs. Lambs with PPHN induced by prenatal DA constriction showed a decrease in mRNA expression of eNOS in pulmonary artery endothelial cells (PAECs). This was associated with an increased DNA methylation at 19 CpG sites in the eNOS proximal promoter region around estrogen receptor elements (EREs).Reference Ke, Johnson and Jing136 These contribute to a downregulation of eNOS expression, inhibition of eNOS activity, and hence reduced NO synthesis. All of the above tends to an imbalance in the vasoconstriction–vasodilation status toward an increased vascular tone in the pulmonary vascular bed.
Histone modifications
DNA is packed in nucleosomes, where approximately 146 base pairs wrap a core of histone proteins. Post-translational modifications in these proteins such as acetylation, methylation, phosphorylation and ubiquitination can change the accessibility to DNA, increasing or reducing gene expression.Reference Kim, Ryan, Marsboom and Archer137 Histone modifications are produced by histone acetyltransferases, histone deacetylases, histone methyltransferases, and histone demethylases, among others.Reference Dodd, Micheelsen, Sneppen and Thon138
Yang et al. showed that chronic hypoxia reduced histone acetylation and DNA methylation. Chronic hypoxia induced histone deacetylase (HDAC) activity that covalently modifies the interaction between histones and gene promoter regions, increasing compaction and decreasing gene expression.Reference Yang, Lu, Ramchandran, Longo and Raj135 In particular, this study demonstrated the inverse relationship between HDAC activity and p21 transcript expression, increasing cell proliferation and SMC remodeling in pulmonary arteries.Reference Yang, Lu, Ramchandran, Longo and Raj135
Ke et al. reported a significant decrease in the activation of mark H4K12ac density in the proximal promoter of the eNOS gene in two distal EREs. They also found an increase in repressive mark H3K9me3 around the transcription factor Sp1 binding site in PAECs from PPHN lambs.Reference Ke, Johnson and Jing136 These changes, together with the increased DNA methylation in the eNOS promoter, contribute to a reduced eNOS mRNA expression (Fig. 2). It is important to mention that acetylation and methylation are not permanent changes and their pattern may change during the lifetime.Reference Kim, Ryan, Marsboom and Archer137
MicroRNAs
miRNAs are small, noncoding RNA molecules composed of 21–25 nucleotides, which are capable of inhibiting translation or decreasing RNA stability in a sequence-specific manner by pairing with RNA, usually in the 3′ untranslated region.Reference Goyal, Limesand and Goyal16,Reference Kim, Ryan, Marsboom and Archer137 miRNAs can cross the placental barrier, being part of the maternal–fetal communication.Reference Chang, Mouillet and Mishima139 Huo et al. showed an altered miRNA lung profile in newborn rats gestated in hypoxia. Of the analyzed miRNAs, these authors found 55 overexpressed and 14 downregulated, which was related to a decrease in lung weight and alveolar count. The two most upregulated miRNAs were miR-465 and miR-377, while the most downregulated were miR-327 and miR-338.Reference Huo, Luo and Wang140 Nothing is known about these miRNAs in lung development; however, miR-338 has been found to be downregulated in pulmonary fibrosis.Reference Zhuang, Dai and Wang141 Xu et al. reported a model of hypoxia-induced PPHN in rats with an increase in miR-126a-5p levels, which is involved in endothelial-to-mesenchymal transition and is likely acting through the p85-β/p-AKT (Protein kinase B) pathway. In this process, an EC undergoes a series of molecular events that lead to a change in phenotype toward a mesenchymal cell (e.g., myofibroblast or SMC), shifting the pulmonary vascular bed to a proliferative and vasocontractile phenotype. This result was also found in plasma from human neonates with PPHN and was correlated with a poor oxygenation index.Reference Xu, Wang and Liu142 Xu et al. reported an overexpression of miR-34c-5p which repressed sGCβ1 expression during hypoxia in mouse SMC primary cultures, decreasing both cGMP bioavailability and smooth muscle-dependent vasodilation.Reference Xu, Wang and Liu142 Until now, no miRNA studies have been performed on developmental chronic hypoxia in sheep. Few studies have been done in sheep and miRNA-target interactions in this species are still scarce.Reference Pasquini and Kunej143
In summary, it has been shown that epigenetic mechanisms are associated with fetal and neonatal pulmonary vascular dysfunction. However, although some of them have shown important associations with pulmonary arterial hypertension in neonatal lambs, we are still missing clues to understand the long-lasting programming of pulmonary vascular function by hypoxia (Fig. 2).
Perspectives
Chronic antenatal and neonatal hypoxia can cause pulmonary arterial hypertension. Most of our current knowledge comes from studies on a sheep model as a translational model (Table 1). Chronic hypoxia during pregnancy and the perinatal period may induce a maladaptive response that triggers sustained increases in PVR by prolonged vasoconstriction and vascular remodeling, leading to the establishment of PAHN. The endothelial dysfunction and the remodeling of SMCs are key factors in triggering vasoconstriction and subsequent vascular remodeling. The chronic and long-lasting effects include vascular dysfunction and eventual cardiac insufficiency. Finally, epigenetic regulation such as the reduction in global DNA methylation, histone modifications, and regulation by miRNAs seem to be key determinants of the pulmonary arterial dysfunction, but there is still much to discover about these mechanisms.
With the current knowledge and lack of efficient treatments, there is a need to evaluate potential therapeutic strategies such as vasoactive, antioxidant, and antiproliferative agents. This will be clearly facilitated by the use of animal models such as the perinatal sheep preparation. Researchers have had the privilege of using animal models and obtained the information to accomplish outstanding discoveries. All in all, sheep studies have improved our knowledge about the basic mechanisms underlying PAHN pathophysiology and treatment (Tables 1 and 2). The valuable data given by animal studies augur a complete understanding of PAHN determinants and future development of treatments.
Acknowledgments
This work was funded by FONDECYT grants 1140647, 1110595, 1151119, and 1201283; and Líneas de apoyo a la investigación financiadas por el ICBM (Puente ICBM 2018–2019).