Introduction
Historically, ecological studies have been conducted at different hierarchical scales, such as ecosystems, communities, guilds and populations (Rynkiewicz et al., Reference Rynkiewicz, Pedersen and Fenton2015); this result in the reduction of complexity facilitates comparing these components in different habitats and localities (Thomas et al., Reference Thomas, Guegan, Renaud and Guegan2005). Studies of species abundance, occurrence and fluctuation are considered central themes in Ecology (Holt et al., Reference Holt, Dobson, Begon, Bowers and Schauber2003). Parasites are considered good model organisms for testing ecological hypotheses since they are abundant in natural environments. In addition, their habitat (hosts) have well-defined limits and, depending on the group, can be effectively replicated (Pietrock & Marcogliese, Reference Pietrock and Marcogliese2003).
The study of parasites does have unique characteristics when compared to that of free-living organisms (Holmes & Price, Reference Holmes and Price1980). Characteristics such as dispersion and recruitment are closely related to the ecology and phylogeny of parasite hosts (Poulin, Reference Poulin2007). Infrapopulations (sensu Bush et al., Reference Bush, Lafferty, Lotz and Shostak1997) describe all individuals of a given parasitic species present in a single host specimen at the same time. Host characteristics including size and sex, seasonality, diet and habitat influence the composition and distribution of species within infrapopulations (Esch et al., Reference Esch, Bush and Aho1990; Holt et al., Reference Holt, Dobson, Begon, Bowers and Schauber2003; Poulin, Reference Poulin2007).
The component community (all infrapopulations living within a particular host species) is frequently an aggregate of host populations (Shaw & Dobson, Reference Shaw and Dobson1995). Usually, hosts offer heterogeneous environments for infection by parasites, and individual variations in behaviour, genetics and immune responses may contribute to the aggregation of parasites (Poulin, Reference Poulin2013). The aggregation of parasites is so prevalent with regard to host associations that it has been proposed as a premise to define the ecological relationship between parasite and host (Crofton, Reference Crofton1971; Pacala & Dobson, Reference Pacala and Dobson1988). An aggregate distribution may provide important information regarding the relationship between parasites and their hosts, and some models have been proposed to identify factors that determine such distribution patterns, such as host density, mortality rate, size, age and sex (May & Anderson, Reference May and Anderson1979; Gordon & Rau, Reference Gordon and Rau1982; Adjei et al., Reference Adjei, Barnes and Lester1986; Rousset et al., Reference Rousset, Thomas, Meeus and Renaud1996; Combes, Reference Combes2001).
Some patterns describing models of occurrence and distribution of parasites in wild hosts have been proposed. They include the competitive exclusion (King, Reference King1964), increased prevalence (Janovy et al., Reference Janovy, Clopton, Clopton, Snyder, Efting and Krebs1995) and resource partitioning (Mouillot et al., Reference Mouillot, George-Nascimento and Poulin2003) models. Additionally, null models (Harvey et al., Reference Harvey, Colwell, Silvertown and May1983; Krasnov et al., Reference Krasnov, Stanko and Morand2006) can be used to evaluate whether the occurrence of parasites is random in different host species or if it is the result of interspecific interactions (Ulrich & Gotelli, Reference Ulrich and Gotelli2010, Reference Ulrich and Gotelli2013). The organization of communities may present somewhere along a continuum that extends between randomness and structure (Price, Reference Price1987; Poulin, Reference Poulin1996; Leung, Reference Leung1998; Rolff, Reference Rolff2000; Lacerda et al., Reference Lacerda, Bellay, Takemoto and Pavanelli2013), where communities composed of small populations, or an organism with low vagility, are typically randomly organized (Gotelli & Rohde, Reference Gotelli and Rohde2002).
An alternative explanation for the aggregate distribution of parasites regards the relationship between the variance and the mean parasite abundance per host, which indicates how aggregation may be regulated by mean infection value (Shaw & Dobson, Reference Shaw and Dobson1995). This assumption is based on the aggregate distribution of organisms, and follows Taylor's law of species distribution (Taylor, Reference Taylor1961), in which the density of organisms varies proportionally in relation to the mean of the population density. Cooccurrence models can also be used to characterize parasite communities, where pairs of parasites may have positive or negative associations with levels of host infection (Price, Reference Price1987). These results may be difficult to interpret, since they reflect competition and/or specialization in the exploitation of a particular host (historical or current). Therefore, it is necessary to test more hypotheses concerning the mechanisms that shape parasite communities, especially those living within terrestrial hosts (Poulin, Reference Poulin1996; Krasnov et al., Reference Krasnov, Stanko and Morand2006; Budischak et al., Reference Budischak, Jolles and Ezenwa2012).
In the present study, we evaluate the influence of average abundance on the variance of endoparasites observed within lizards living in semiarid areas of the Caatinga in north-eastern Brazil. Studies on lizard endoparasites from Caatinga have become more frequently produced in recent years (Ávila et al., Reference Ávila, Anjos, Ribeiro, Morais, Silva and Almeida2012; Brito et al., Reference Brito, Corso, Almeida, Ferreira, Almeida, Anjos, Mesquita and Vasconcellos2014a; Araujo Filho et al., Reference Araujo Filho, Brito, Lima, Pereira, Mesquita, Albuquerque and Almeida2017; Teixeira et al., Reference Teixeira, Brito, Teles, Ribeiro, Araujo Filho, Lima, Pereira and Almeida2017; Teles et al., Reference Teles, Brito, Teixeira, Ribeiro, Araujo Filho, Lima, Pereira and Almeida2017), but our understanding of endoparasite community structures with regard to both the abundance and aggregation patterns of parasites is lacking (Ávila et al., Reference Ávila, Anjos, Ribeiro, Morais, Silva and Almeida2012; Araujo Filho et al., Reference Araujo Filho, Ribeiro, Brito, Teles, Sousa, Avila and Almeida2014). The endoparasitic fauna of Caatinga lizards is composed mainly of generalists, where parasite abundances are positively related to the size, sex and reproductive period of the hosts, as well as with the rainy season, which indicate that multiple factors determine the patterns of resource use by parasitic species (Anjos et al., Reference Anjos, Almeida, Vasconcellos, Freire and Rocha2007; Almeida et al., Reference Almeida, Freire and Lopes2008; Ávila et al., Reference Ávila, Anjos, Ribeiro, Morais, Silva and Almeida2012; Lima et al., Reference Lima, Brito, Araujo Filho, Teles, Ribeiro, Teixeira, Pereira and Almeida2017; Oliveira et al., Reference Oliveira, Teixeira, Queiroz, Araujo Filho, Teles, Brito and Mesquita2017; Teixeira et al., Reference Teixeira, Brito, Teles, Ribeiro, Araujo Filho, Lima, Pereira and Almeida2017; Teles et al., Reference Teles, Brito, Teixeira, Ribeiro, Araujo Filho, Lima, Pereira and Almeida2017).
Thus, our goals were to determine the community composition of lizard endoparasites and test the following hypothesis: (H0) due to interspecific competition, the occurrence of endoparasites within hosts is structured, and is homogeneously distributed; and (H1) due to the abundance of generalist endoparasitic species, they may occur randomly within host populations in an aggregate pattern. In addition, we determined the degree to which sample variance is influenced by the average abundance of endoparasitic species, patterns of cooccurrence and dominance among endoparasitic species and similarities between the abundance and richness of infracommunities of endoparasites in several host species.
Materials and methods
Study areas
Samples were collected from three areas of Alto Sertão, Sergipe, Northeast Brazil. Each area was composed of two sampling sites, which resulted in a total of six sample units. Area I was located in Porto da Folha (Quilombola settlement of Mocambo) and Poço Redondo (private farm) municipalities; area II was located in the Poço Redondo municipality (Grota do Angico Natural Monument and the Angico Farm); and area III was located in Canindé do São Francisco (Jerimum Farm) and Porto da Folha (Quilombola settlement of Mocambo) municipalities. The most distant areas were approximately 40 km apart.
All sampling sites were located in the Caatinga domain, a semiarid region of north-eastern Brazil with a ‘BSh’ climate, according to Köppe, which belongs to the Southern Sertaneja Depression (Ab’Saber, Reference Ab'Saber1974; Velloso et al., Reference Velloso, Sampaio and Pareyn2002), in the Alto Sertão region of Sergipe state. The rainfall levels vary in accordance with a four-month rainy season and eight-month dry season. The average annual precipitation level is approximately 500 mm, and the average annual temperature varies between 26 and 28°C. Vegetation within the area is composed of typical plants from the arboreal shrubby of Caatinga, in which cacti and bromeliads are present (Andrade-Lima, Reference Andrade-Lima1981; Sá et al., Reference Sá, Riché, Fotius, Silva, Tabarelli, Fonseca and Lins2004).
Data collection
Sites were visited for 30 days, within dry (April and August 2016) and rainy (September 2015 and February 2016) seasons. Specimens were collected using pitfall traps and by actively searching for animals. After collection, the specimens were euthanized and deposited in the Herpetological Collection of the Federal University of Paraíba (Universidade Federal da Paraíba – CHUFPB).
Lizards were dissected under a stereomicroscope, and lung, stomach, liver, gallbladder and small and large intestine tissues were analysed. Further cavities were searched for endoparasites. Endoparasites were counted and preserved in 70% ethanol for subsequent analyses. Specimens were identified to the lowest possible taxonomic level with the aid of a microscope equipped with an image analyser (Carl Zeiss Microimaging GmbH, Gottingen, Germany) and mounted on temporary slides with the use of either a lactic acid medium (nematodes), Hoyer's medium (pentastomids) or stained with acetic carmine and mounted in Eugenol (acanthocephalans, trematodes and cestodes).
Statistical analysis
Ecological terminology used was in accordance with definitions provided in Bush et al. (Reference Bush, Lafferty, Lotz and Shostak1997). To determine the distribution pattern of the infrapopulations (all endoparasites of a particular species present in a population of hosts), the dispersion index (DI) was calculated, which ranged from zero to one using Quantitative Parasitology 3.0 software (Rózsa et al., Reference Rózsa, Reiczigel and Majoros2000). A DI of zero indicated that all species were uniformly distributed and a DI of one indicated that all endoparasites were found in only one host (Poulin, Reference Poulin1993). In addition, the Morisita index of aggregation was calculated by computing the abundance of species, which had an associated P-value (chi-square), using XLstat software (Addinsoft, 2004).
To test whether the endoparasitic community was randomly distributed across host species, a pseudo community analysis was performed with 10,000 randomizations of a null model. The presence–absence matrix was built with species of endoparasites (rows) and hosts species (columns). We used the algorithm ‘R3’ because it maintained the specialization of each endoparasitic species, but considered the possibility of using newly available resources (Winemiller & Pianka, Reference Winemiller and Pianka1990).
Linear regression analysis was performed between variance of mean intensity (log10) and the average abundance of endoparasites, to identify a possible degree of restriction in aggregation, although this parameter is influenced by aggregate populations, reflecting possible competition for space (host/site of infection) and/or the ‘limit abundance’ that a host specimen can support (Shaw & Dobson, Reference Shaw and Dobson1995).
The normality of abundance and richness was assessed using the Shapiro–Wilk test. The data were not normally distributed (Wald = 0.410, P < 0.0001; W = 0.803, P < 0.0001, respectively). Therefore, the Spearman and Pearson correlation test was used to evaluate whether pairs of endoparasitic species were correlated. The Bray–Curtis similarity index was performed to evaluate the relationship between the richness and abundance of an endoparasitic infracommunity within each group of hosts analysed. Species assessed included Gymnodactylus geckoides Spix, 1825; Phyllopezus pollicaris Spix, 1825 (Phyllodactylidae); Ameivula ocellifera Spix, 1825 (Teiidae); Vanzosaura multiscutata Amaral, 1993 (Gymnophthalmidae); and Tropidurus hispidus Spix, 1825 and T. semitaeniatus Spix, 1825 (Tropiduridae). At least ten specimens from each of these species were collected in each area studied. A similarity matrix was constructed using the Bray–Curtis method. Analyses were performed using the R program (Core Team, 2019).
Dominance (d) of endoparasitic species was calculated according to the Berger–Parker index (Magurran, Reference Magurran2013). Later, the Kruskal–Wallis test was performed to determine whether differences in the dominance of the component community among host species and within each host species were significant (only endoparasitic species with prevalence greater than 5% were considered).
Results
A total of 2141 lizards were dissected, which belonged to 16 different species. Species identified included Acratosaura mentalis (number of specimens (n) = 62; mean snout-vent length ± standard deviation (SVL) = 48.1 ± 9.5); Ameiva (n = 12; SVL = 77.6 ± 38.5); Ameivula ocellifera (n = 541; SVL = 65.7 ± 10.1); Coleodactylus meridionalis (n = 1; SVL = 17.1); G. geckoides (n = 477; SVL = 38.7 ± 2.4); Hemidactylus brasilianus (n = 1; SVL = 39.1); Hemidactylus mabouia (n = 1; SVL = 59.7); Iguana (n = 2; SVL = 124.3); Lygodactylus klugei (n = 65; SVL = 27.5 ± 2.1); Brasiliscincus heathi (n = 25; SVL = 63 ± 5.7); Phyllopezus pollicaris (n = 173; SVL = 60.6 ± 9.3); Polychrus acutirostris (n = 1; SVL = 126.8); Tropidurus hispidus (n = 354; SVL = 71.1 ± 16.2); T. semitaeniatus (n = 307; SVL = 67.6 ± 10.2); Tupinambis merianae (n = 7; SVL = 90 ± 5.1); and V. multiscutata (n = 112; SVL = 32.4 ± 2.1). A total of 25,687 endoparasites were collected and identified, which included nematodes, pentastomids, acanthocephalans, trematodes and cestodes, from a total of 13 taxa (table 1) from 1233 host organisms.
Table 1. Endoparasites associated with the lizards collected in the Caatinga environment, a semiarid region of the Alto Sertão of Sergipe, Northeast Brazil.
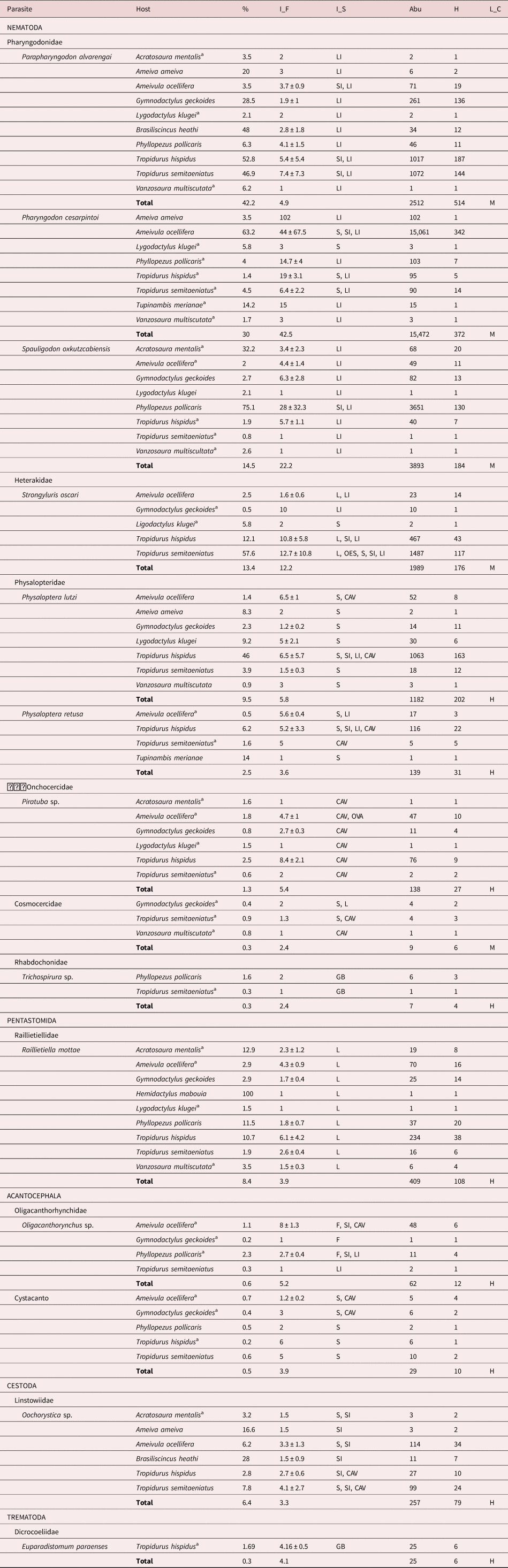
a New records of hosts. Prevalence (%). I_F, mean intensity of infection; I_S, infection site; Abu, abundance; H, number of hosts; L_C, life cycle; M, monoxenic; He, heteroxenic; L, L; S, stomach; SI, small intestine; LI, large intestine; GB, gallbladder; CAV, cavity; OES, oesophagus; OVA, ovary.
Of the total number of hosts analysed, 57.58% were parasitized by at least one species, and an average of 20.8 ± 15.5 parasites were found in each infected host. A total of 39 new host records were identified (table 1). Within the host population, 23.76% were infected by two species of endoparasites; 6.40% were infected by three species; 1.54% were infected by four species; 0.24% were infected by five species; and only two specimens of T. hispidus (0.16%) were each infected by six endoparasitic species.
Nematodes were the most highly prevalent type of endoparasitic organism identified. Of the seven species that were found at a prevalence greater than 5%, five were nematodes, and one species of Pentastomida and Cestoda were also found (table 1). All endoparasitic species exhibited a typical aggregate distribution patterns (table 2). Although subtle variations were observed between monoxenic and heteroxenic species, the latter type produced slightly higher discrepancy values.
Table 2. Morisita aggregation index.

Imor, index value; Mclu, upper values; Muni, lower values; Imst, standardized index value; P, chi-square test value for each distribution; D, Poulin's discrepancy index. When Imor is >Mclu, the distribution is considered aggregate. Values of Imst >0.5 indicate aggregated distribution.
The occurrence of endoparasites in the host community did not differ significantly from that which would be expected to occur at random, and the observed index value of 0.617 was not smaller than the simulated index of 0.497, which indicated the absence of community structure (P > 0.9). Variance observed was strongly explained by mean value observed (F 942; R² = 0.98; P < 0.001) (fig. 1). In other words, there was a regulatory effect that affected the average number of parasites per host, and this restriction generated the aggregation limit observed.

Fig. 1. Relationship between variance and mean abundance (log10; F942; R² = 0.98; P < 0.001) of endoparasites associated with lizards, collected in a semiarid Caatinga environment, Northeast Brazil.
The species of endoparasites possessing the greatest correlation values were congeners Physaloptera lutzi and P. retusa (table 3). Similarly, monoxenic species were positively correlated, but the only species that was associated with others was Parapharyngodon alvarengai. Similarity analysis revealed that phylogenetically similar hosts, or those with similar foraging habits, possessed similar endoparasitic fauna (table 4). Sympatric lizards T. hispidus and T. semitaeniatus had endoparisitic fauna that were the most similar.
Table 3. Spearman correlation between pairs of endoparasite species (prevalence >5%) and Pearson correlation (P-values) associated with lizards from an environment in Caatinga, north-eastern Brazil. Statistically significant p-value (bold).

Table 4. Similarity between the lizard species grouped according to the composition of the endoparasites fauna, using the Bray–Curtis index.

Endoparasitic species exhibited different levels of dominance within each host species (table 5). Similarly, dominance was significantly variable among host species (Shannon index H' = 19.67; Degrees of freedon GL = 11; P < 0.001). For the teiid species A. ocellifera, the dominant species was the monoxenic nematode Pharyngodon cesarpintoi. For the lizards, G. geckoides, Brasiliscincus heathi and T. semitaeniatus, the dominant species was the monoxenic nematode Parapharyngodon alvarengai. Phlopezpezus pollicaris exhibited a high degree of dominance, and the monoxenic species, Spauligodon oxkutzcabiensis, was the most important. For the T. hispidus host, the heteroxenic species, P. lutzi, and the monoxenic species, P. alvarengai, had the highest dominance values.
Table 5. Berger–Parker dominance index and Kruskal–Wallis test (H = 19.67; GL = 11) corresponding to variation in dominance within each host species. Statistically significant p-value (bold).

Lizards: Am. oc, Ameivula ocellifera; Ac. me, Acratosaura mentalis; Aa. am, Ameiva; G. ge, Gymnodactylus geckkoides; L. kl, Ligodactylus klugei; B. he, Brasiliscincus heathi; P. po, Phyllopezus pollicaris; T. hi, Tropidurus hispidus; T. se, T. semitaeniatus; T. me, Tupinambis merianae; V. mu, Vanzosaura multiscutata. Endoparasites: P. lu, Physaloptera lutzi; P. re, P. retusa; R. mo, Raillietiella mottae; St. os, Strongyluris oscari; Ph. ce, Pharyngodon cesarpintoi; Sp. ox, Spauligodon oxkutzcabiensis; Pir. sp, Piratuba sp.; Pa. al, Parapharyngodon alvarengai; Ooc. sp, Oochorystica sp.; E. pa, Euparadistomum paraenses; Oli. sp, Olygachantharynchus sp.; Tri. sp, Trichospirura sp.
Discussion
Thirteen endoparasitic species were determined to be associated with lizards. Monoxenic nematodes were the most prevalent, which included P. alverengai, P. cesarpintoi and S. oxkutzcabiensis (Pharyngodonidae) species. These species were most prevalent within T. hispidus, T. semitaeniatus, A. ocellifera and P. pollicaris hosts. The absence of intermediate hosts, coupled with the habit of some lizards of ‘tasting’ the environment with their tongue (personal observation), may contribute to high infection rates of endoparasites with direct cycles (Anderson, Reference Anderson2000). However, foraging mode, contact between susceptible hosts and population density may also contribute to increases observed within this category. Pharyngodon were prevalent within lizards (Gupta et al., Reference Gupta, Bhaskar and Guptar2009; Anjos et al., Reference Anjos, Avila, Ribeiro, Almeida and Silva2012; Brito et al., Reference Brito, Corso, Almeida, Ferreira, Almeida, Anjos, Mesquita and Vasconcellos2014a; Teles et al., Reference Teles, Brito, Teixeira, Ribeiro, Araujo Filho, Lima, Pereira and Almeida2017). The genus is composed of monoxenic species, which are parasites of vertebrates, with a wide global distribution pattern (Ávila & Silva, Reference Ávila and Silva2010; Campião et al., Reference Campião, Morais, Dias, Aguiar, Toledo, Tavares and Silva2014; Goldberg et al., Reference Goldberg, Bursey and Kraus2016).
The most important hosts (prevalence > 45%) for P. alvarengai were T. hispidus (52.82%) and T. semitaeniatus (46.90%). Previous studies (Anjos et al., Reference Anjos, Avila, Ribeiro, Almeida and Silva2012; Brito et al., Reference Brito, Corso, Almeida, Ferreira, Almeida, Anjos, Mesquita and Vasconcellos2014a) reported high prevalence values for P. alverengai within the same host species in different regions of Caatinga. Concerning the host A. ocellifera, the prevalence of the nematode P. cesarpintoi was high (63.21%). In addition, the nematode, S. oxkutzcabiensis, was highly prevalent (75.14%) within the host P. pollicaris, which was a finding that was similar to that which was reported previously in Caatinga (Lima et al., Reference Lima, Brito, Araujo Filho, Teles, Ribeiro, Teixeira, Pereira and Almeida2017). Despite being considered generalists, these species were more prevalent in particular hosts than others. This variation may be associated with undescribed cospeciation mechanisms, as well as with the specific microhabitat and diet of the hosts (Esch et al., Reference Esch, Bush and Aho1990; Hamann et al., Reference Hamann, Kehr and Gonzalez2006; Brito et al., Reference Brito, Ferreira, Ribeiro, Anjos, Almeida, Mesquita and Vasconcellos2014b).
The most prevalent heteroxenic endoparasites were P. lutzi in T. hispidus (46.04%) and P. retusa in T. merianae (14.02%) (Physalopteridae). Physalopterids are endoparasites of several vertebrates (Goldberg et al., Reference Goldberg, Bursey and Cheam1998; Campião et al., Reference Campião, Morais, Dias, Aguiar, Toledo, Tavares and Silva2014) and can be found in 38 omnivorous lizards in Brazil (Ávila & Silva, Reference Ávila and Silva2010; Araujo Filho et al., Reference Araujo Filho, Ribeiro, Brito, Teles, Sousa, Avila and Almeida2014; Lima et al., Reference Lima, Brito, Araujo Filho, Teles, Ribeiro, Teixeira, Pereira and Almeida2017). The prevalence of these endoparasites varies according to the area considered (Brito et al., Reference Brito, Corso, Almeida, Ferreira, Almeida, Anjos, Mesquita and Vasconcellos2014a; Lima et al., Reference Lima, Brito, Araujo Filho, Teles, Ribeiro, Teixeira, Pereira and Almeida2017), and it may be related to changes in intermediate host communities and seasonality (Narayanan et al., Reference Narayanan, Rao and Thontadaraya1961; Vasconcellos et al., Reference Vasconcellos, Andreazze, Almeida, Araujo, Oliveira and Oliveira2010).
The pentastomid genus Raillietiella (Raillietiellidae) infects a wide variety of insectivorous lizards in Brazil (Vrcibradic et al., Reference Vrcibradic, Rocha, Bursey and Vicente2002; Almeida et al., Reference Almeida, Freire and Lopes2008; Ribeiro et al., Reference Ribeiro, Ferreira, Silva, Vieira and Moura2012; Brito et al., Reference Brito, Corso, Almeida, Ferreira, Almeida, Anjos, Mesquita and Vasconcellos2014a; Sousa et al., Reference Sousa, Brito, Avila, Teles, Araujo Filho, Teixeira, Anjos and Almeida2014; Lima et al., Reference Lima, Brito, Araujo Filho, Teles, Ribeiro, Teixeira, Pereira and Almeida2017). Herein, the genus was most highly prevalent in A. mentalis (12.9%), P. pollicaris (11.56%) and T. hispidus (10.73%) lizards. As demonstrated by Lima et al. (Reference Lima, Brito, Araujo Filho, Teles, Ribeiro, Teixeira, Pereira and Almeida2017), species of Raillietiella were prevalent in gecko hosts (Gekkonidae and Phyllodactylidae), and their high degree of prevalence within A. mentalis (Gymnophthalmidae) and their newly discovered association with Raillietiella mottae may be a result of the species’ flexibility, in the infection of new hosts, variations in host use and the availability intermediates. These variations have also been found in several other Raillietiella species (Kelehear et al., Reference Kelehear, Spratt, Dubey, Brown and Shine2011, Reference Kelehear, Brown and Shine2012).
Trichospirura nematodes occur in amphibians (Moravec & Kaiser, Reference Moravec and Kaiser1994), lizards (Goldberg et al., Reference Goldberg, Bursey and Cheam1998) and mammals (Bain & Junker, Reference Bain and Junker2013) and were first recorded to infect lizards in South America by Lima et al. (Reference Lima, Brito, Araujo Filho, Teles, Ribeiro, Teixeira, Pereira and Almeida2017) in Caatinga. We found few specimens of Trichospirura sp. that were associated with the gallbladder of P. pollicaris (1.69%) and T. semitaeniatus (0.32%), the latter of which is a new record. The trematode Euparadistomum paraenses was found in the gallbladder of the lizard T. hispidus, which was the first record of that genus of endoparasite infecting lizards globally. Typically, they parasitize marsupial mammals (Betterton, Reference Betterton1980), and the low prevalence determined (1.69%) may indicate an accidental infection by the parasitic species. Other new host records (table 1) indicate the potential for the exploration of host lizard assemblages, since endoparasitic species of intermediate prevalence values may not have been found to date due to either their absence or insufficient host population samples in certain areas.
Regarding distribution patterns, all component communities considered in this study exhibited aggregate distribution patterns and high discrepancy values. Endoparasite populations of lizards are aggregated even when the hosts lived in different microhabitats and have different diets and foraging activities (Anjos et al., Reference Anjos, Avila, Ribeiro, Almeida and Silva2012; Barreto-Lima et al., Reference Barreto-Lima, Toledo and Anjos2012; Macedo et al., Reference Macedo, Gardner, Melo, Giese and Santos2017; Cabral et al., Reference Cabral, Teles, Brito, Almeida, Anjos, Guarnieri and Ribeiro2018; Ribeiro et al., Reference Ribeiro, Ferreira, Brito, Teles, Ávila, Almeida, Anjos and Guarnieri2018). Individual susceptibility, food specificity and variability with regard to exposure to infective parasitic forms are responsible for the distribution of parasites among hosts (Anderson & May, Reference Anderson and May1979; Anderson & Gordon, Reference Anderson and Gordon1982). Heteroxenic endoparasites have higher discrepancy values than monoxenic species; and infective forms can occur in environmental ‘pockets’ and accumulate in individuals used as intermediate hosts, which may contribute to the aggregation of endoparasitic populations (Anderson & May, Reference Anderson and May1979; Anderson & Gordon, Reference Anderson and Gordon1982).
Anjos et al. (Reference Anjos, Avila, Ribeiro, Almeida and Silva2012) identified depauperate fauna dominated by generalist endoparasites in T. hispidus and determined intermediate values of discrepancy (D = 0.51) in Caatinga areas in Ceará (CE) state. Ribeiro et al. (Reference Ribeiro, Ferreira, Silva, Vieira and Moura2012) determined that discrepancy values were high for the nematode genus, Rhabdias sp. (D = 0.83), in a population of the Anolis brasiliensis lizard species in the same area. Cabral et al. (Reference Cabral, Teles, Brito, Almeida, Anjos, Guarnieri and Ribeiro2018) determined that values of discrepancy were high for P. alvarengai (0.68), Physalopteroides venacioi (0.97), Physaloptera sp. (0.98) and Strongyluris oscari (0.98) nematodes associated with the Mabuya arajara lizard species in the humid forest within the Araripe Plateau (as ‘brejo de altitude’), CE.
In the Atlantic Forest, the nematode species S. oscari and Oswaldocruzia burseyi exhibited intermediate discrepancy values (D = 0.57 and D = 0.62, respectively), which were associated with the population of the lizard, Enyalius perditus, in the state of Minas Gerais (Barreto-Lima et al., Reference Barreto-Lima, Toledo and Anjos2012). For teiid lizards in the Amazon region, Macedo et al. (Reference Macedo, Gardner, Melo, Giese and Santos2017) found determined intermediate (D = 0.52) to high (D = 0.92) discrepancy values for endoparasitic nematodes. In addition to aggregation, we determined that average abundance strongly influenced the number of endoparasites per host observed, and determined the variance of samples (fig. 1). Due to the nature of the parasite/host relationship, an interpretation of these values can clarify aspects of the acquisition and establishment of infrapopulations (Shaw & Dobson, Reference Shaw and Dobson1995).
Infrapopulations may be directly influenced by host species and, thus, be distributed according to their genetic predisposition (Schad & Anderson, Reference Schad and Anderson1985; Quinnell, Reference Quinnell2003), immune response (Galvani, Reference Galvani2003) and duration of exposure of the host to infective forms (Gordon & Rau, Reference Gordon and Rau1982). As a result, older hosts tend to be more highly infected than juvenile hosts, which may result in higher mortality rates within the age category (Rousset et al., Reference Rousset, Thomas, Meeus and Renaud1996).
Small differences in the niche occupied by individuals from the same population facilitate the occurrence of encounters different from the infective forms present in the environment (Janovy & Kutish, Reference Janovy and Kutish1988), thus increasing the aggregate pattern of infection with parasitic species. Lizard populations may exhibit individual variations with respect to niche use, especially in communities that have few species (Costa et al., Reference Costa, Mesquita, Colli and Vitt2008); unfortunately, there is a lack of knowledge regarding subpopulation distributions in lizard hosts, making it difficult to compare and understand the distribution patterns of parasites.
Regarding the pseudo community analysis, for parasite species, assemblages are likely structured as a result of nesting (González & Oliva, Reference González and Oliva2009), temporal variation (Krasnov et al., Reference Krasnov, Stanko and Morand2006) and aggregation (Leung, Reference Leung1998). However, there have also been reports of assemblages of various groups of hosts including fish, amphibians, birds and mammals with no apparent structure (Gotelli & Rohde, Reference Gotelli and Rohde2002; González & Oliva, Reference González and Oliva2009; Ulrich & Gotelli Reference Ulrich and Gotelli2010, Reference Ulrich and Gotelli2013; Delfino et al., Reference Delfino, Ribeiro, Furtado, Anjos and Almeida2011; Lacerda et al., Reference Lacerda, Bellay, Takemoto and Pavanelli2013).
According to our model, host infection occurs randomly, and endoparasite occurrence is randomly distributed across hosts. When intraspecific interactions between endoparasites does not significantly shape the component community, it does not differ significantly from the community predicted by the null model (Poulin, Reference Poulin1996). Communities composed of few species or those that are generalists are typically not structured (Gotelli & Rohde, Reference Gotelli and Rohde2002; González & Oliva, Reference González and Oliva2009). With regard to lizards, especially those living in Caatinga environments, few endoparasite species are specific to lizard hosts, such as the nematodes Ozolaimus cirratus and O. megatyphlon. Alaeuris caudatus and A. vogelsangi are, however, specific parasites of iguanas, and Gynaecometra bahiensis specifically parasitizes Polycrhus acutirostris. These specificities are probably related to arboreal habits and the herbivorous and omnivorous diets of hosts, respectively (Ávila & Silva, Reference Ávila and Silva2010; Araujo Filho et al., Reference Araujo Filho, Ribeiro, Brito, Teles, Sousa, Avila and Almeida2014; Teles et al., Reference Teles, Brito, Teixeira, Ribeiro, Araujo Filho, Lima, Pereira and Almeida2017).
The resources sought by parasites may occur in hosts of distinct taxa, which may contribute to the formation of random communities. This does not necessarily indicate a low degree of richness, competitive exclusion or lack of interaction among species (Brooks et al., Reference Brooks, León-Règagnon, McLennan and Zelmer2006). For the Caatinga domain, historical and ecological factors, and seasonality and geographic variations have a greater influence on the establishment of endoparasite communities (Brito et al., Reference Brito, Corso, Almeida, Ferreira, Almeida, Anjos, Mesquita and Vasconcellos2014a, Reference Brito, Ferreira, Ribeiro, Anjos, Almeida, Mesquita and Vasconcellosb; Araujo Filho et al., Reference Araujo Filho, Brito, Lima, Pereira, Mesquita, Albuquerque and Almeida2017; Lima et al., Reference Lima, Brito, Araujo Filho, Teles, Ribeiro, Teixeira, Pereira and Almeida2017; Teixeira et al., Reference Teixeira, Brito, Teles, Ribeiro, Araujo Filho, Lima, Pereira and Almeida2017; Teles et al., Reference Teles, Brito, Teixeira, Ribeiro, Araujo Filho, Lima, Pereira and Almeida2017).
According to Jaenike (Reference Jaenike1996), to understand the effects of variance on average abundance, the following premise must be considered: (1) the parasite has the ability to regulate host fitness; and (2) average abundance depends on parasite frequency within the host population. Thus, the greater the degree of parasite aggregation, the greater the fitness of the host population. If the degree of aggregation is low, the fitness of the host population is also low (Shostak & Dick, Reference Shostak and Dick1987; Jaenike, Reference Jaenike1996). Similarly, aggregation is associated with increased fitness of the parasite population.
In contrast, aggregation can facilitate meeting potential partners for reproduction. Heavily infected hosts may have high mortality rates (Adjei et al., Reference Adjei, Barnes and Lester1986), compromising the maintenance of infrapopulations (provided the life cycle of the species does not depend on the death of its host). The restriction of variance on the average abundance of parasitic species provides a balance between aggregation and infrapopulation viability, which can be evolutionarily established (Wilson et al., Reference Wilson, Bjørnstad, Dobson, Merler, Poglayen, Randolph, Read and Skorping2002). This mechanism may be especially prominent in parasitic species and may be one of the primary ecological forces shaping infrapopulation distribution (Shaw & Dobson, Reference Shaw and Dobson1995; Lagrue et al., Reference Lagrue, Poulin and Cohen2015). This association has been found in several studies of aggregated populations of parasites (Anderson & Gordon, Reference Anderson and Gordon1982; Shaw & Dobson, Reference Shaw and Dobson1995; Wilson et al., Reference Wilson, Bjørnstad, Dobson, Merler, Poglayen, Randolph, Read and Skorping2002; Poulin, Reference Poulin2013).
It is widely accepted that variance dominated by the mean indicates that there is a restriction in the level of aggregation within a population. This results in a trade-off between a high degree of aggregation of endoparasites, which can cause high mortality rates in their hosts (Rousset et al., Reference Rousset, Thomas, Meeus and Renaud1996), and low levels of aggregation, which may cause difficulties in finding partners for reproduction. As a result, populations of parasites are produced that have optimal levels of aggregation (Poulin, Reference Poulin2007). We found that 98% of the variance observed was explained by mean abundance, a value that was unexpectedly high. Shaw & Dobson (Reference Shaw and Dobson1995) reviewed the relationship between mean variance and abundance in 269 populations of hosts (amphibians, birds and mammals) and found that 87% of observed variance is related to average abundance. However, there was a lack of research on reptiles, especially in semiarid areas, so comparisons with this work are not straightforward.
In the endoparasitic species P. lutzi and P. retusa, a positive correlation between abundance and the use of intermediate and definitive hosts was observed (Anderson, Reference Anderson2000). Parapharyngodon alvarengai was positively correlated with P. lutzi, P. retusa and S. oscari species. Pharyngodon cesarpintoi and S. oxkutzcabiensis exhibited a negative (or low degree of) correlation with all other species. These species may be considered generalist when infecting lizards, but their abundance and prevalence were greater in teiid and gecko hosts, respectively (Ávila & Silva, Reference Ávila and Silva2010; Brito et al., Reference Brito, Corso, Almeida, Ferreira, Almeida, Anjos, Mesquita and Vasconcellos2014a; Lima et al., Reference Lima, Brito, Araujo Filho, Teles, Ribeiro, Teixeira, Pereira and Almeida2017). Taxonomic proximity and life cycle may contribute to associations found; however, competition, density-dependence and use of resources by endoparasites may also be important, but these features are difficult to measure in natural populations. Nevertheless, P. alvarengai was the only species that presented a statistically significant association with the other species. This relationship was likely enhanced by the generalist habit and monoxenic cycle of P. alvarengai. The species has been present in nearly all studies that have included lizards of South America (Ávila & Silva, Reference Ávila and Silva2010; Brito et al., Reference Brito, Corso, Almeida, Ferreira, Almeida, Anjos, Mesquita and Vasconcellos2014a; Galdino et al., Reference Galdino, Ávila, Bezerra, Passos, Melo and Zanchi2014).
The hosts of endoparasite species that were most similar were T. hispidus and T. semitaeniatus. In addition to their taxonomic proximity, these species shared similar microhabitats and diets (Rodrigues, Reference Rolff2003; Gomes et al., Reference Gomes, Caldas, Santos, Silva, Santana, Rocha, Ferreira and Faria2015). Gimnodactylus geckoides was similar to tropidurids, and is a species with general foraging habits, which may contribute to the similarities between the endoparasites observed (Rodrigues, Reference Rolff2003). These species are commonly associated with rocky areas, and have general diets (Rodrigues, Reference Rolff2003; Gomes et al., Reference Gomes, Caldas, Santos, Silva, Santana, Rocha, Ferreira and Faria2015). The use of similar habitats and consumption of similar diets may contribute to similarities observed in the composition of endoparasite species (Hamann et al., Reference Hamann, Kehr and Gonzalez2006; Brito et al., Reference Brito, Ferreira, Ribeiro, Anjos, Almeida, Mesquita and Vasconcellos2014b).
There was significant variation observed regarding the dominance of endoparasites among host species, as well as within each host species. For the component community present in A. ocellifera, dominance was determined by the pharyngodonid, P. cesarpintoi. This endoparasite genus is widely dispersed in South America, and infects several families of lizards, notably infecting Teiidae (Ávila & Silva, Reference Ávila and Silva2010). In G. geckoides and B. heathi, the nematode P. alvarengai was the dominant species. Along with other pharyngodonids, P. alvarengai has a monoxenic cycle and is commonly found in generalist lizards (Ávila et al., Reference Ávila, Anjos, Ribeiro, Morais, Silva and Almeida2012, Brito et al., Reference Brito, Corso, Almeida, Ferreira, Almeida, Anjos, Mesquita and Vasconcellos2014a).
Within the host species P. pollicaris, the nematode S. oxkutzcabiensis was dominant. The nematode has been frequently associated with lizards (Gekkota) in several environments of South America (Ávila & Silva, Reference Ávila and Silva2010), including semiarid regions (Brito et al., Reference Brito, Corso, Almeida, Ferreira, Almeida, Anjos, Mesquita and Vasconcellos2014a, Reference Brito, Ferreira, Ribeiro, Anjos, Almeida, Mesquita and Vasconcellosb; Lima et al., Reference Lima, Brito, Araujo Filho, Teles, Ribeiro, Teixeira, Pereira and Almeida2017). The generalist lizard T. hispidus had the greatest number of associated endoparasites in this study. The species is widely dispersed in Caatinga areas and its habitat use and diet is variable (Rodrigues, Reference Rolff2003). The heteroxenic nematode P. lutzi was the dominant species in the infracommunity of this host. Physaloptera sp. are often associated with carnivorous lizards (Bursey et al., Reference Bursey, Goldberg and Parmelee2005; Ávila & Silva, Reference Ávila and Silva2010; Brito et al., Reference Brito, Corso, Almeida, Ferreira, Almeida, Anjos, Mesquita and Vasconcellos2014a; Lima et al., Reference Lima, Brito, Araujo Filho, Teles, Ribeiro, Teixeira, Pereira and Almeida2017). The component community of the tropidurid, T. semitaeniatus, is primarily composed of the nematode S. oscari, which infects a wide range of hosts from different families in South America (Ávila & Silva, Reference Ávila and Silva2010).
To the best of our knowledge, this is the first study to evaluate the influence of average abundance on endoparasitic variance in semiarid areas, and our results indicate that endoparasite infrapopulation variance are highly restricted by average abundance per host values. Further, the structure of the component community regarding the use of host species is random.
Environments that are subjected to large seasonal variations have high parasite aggregation values, because species can synchronize the release of infective forms according to season, which may contribute to increased levels of infection and aggregation (Sherrard-Smith et al., Reference Sherrard-Smith, Perkins, Chadwick and Cable2015). In contrast, parasite populations that are highly interactive (e.g. occupy the same site of infection) tend to be more strongly regulated by average abundance (Bottomley et al., Reference Bottomley, Isham and Basanez2005). These assumptions may be especially true for lizard hosts in semiarid regions, and their effects in other groups of hosts should be assessed.
Acknowledgements
We are grateful to Érica G. Silva; Cicero L.M. Pinto and Lucas R. Chaves for their help in the laboratory. We also thank staff at the Universidade Federal da Paraíba (UFPB) for professional revision of the English version of the manuscript.
Financial support
This work was supported by research grants from the Conselho Nacional de Desenvolvimento Científico e Tecnológico (CNPq) (W.O.A., grant number 302429/2015-8), (D.O.M., grant number 306541/2017-3); and a scholarship awarded to D.A.T. and J.A.A.F. (142310/2016-6). We thank CAPES (Coordenação de Aperfeiçoamento de Pessoal de Nível Superior) for research fellowships awarded to A.A.M.T.
Conflicts of interest
None.
Ethical standards
All authors gave their consent to participation in the study. The study was approved by the Instituto Chico Mendes de Conservação da Biodiversidade (ICMBio) with permission to collect the animals.