1. Introduction
Stroke is one of the leading causes of death and the most significant cause of persistent and acquired disability in adults worldwide, with over 13 million new cases annually [Reference Feigin, Nichols, Alam, Bannick, Beghi, Blake, Culpepper, Dorsey, Elbaz, Ellenbogen, Fisher, Fitzmaurice, Giussani, Glennie, James, Johnson, Kassebaum, Logroscino, Marin, Mountjoy-Venning, Nguyen, Ofori-Asenso, Patel, Piccininni, Roth, Steiner, Stovner, Szoeke, Theadom, Vollset, Wallin, Wright, Zunt, Abbasi, Abd-Allah, Abdelalim, Abdollahpour, Aboyans, Abraha, Acharya, Adamu, Adebayo, Adeoye, Adsuar, Afarideh, Agrawal, Ahmadi, Ahmed, Aichour, Aichour, Aichour, Akinyemi, Akseer, Al-Eyadhy, Salman, Alahdab, Alene, Aljunid, Altirkawi, Alvis-Guzman, Anber, Antonio, Arabloo, Aremu, Ärnlöv, Asayesh, Asghar, Atalay, Awasthi, Quintanilla, Ayuk, Badawi, Banach, Belachew, Bensenor, Berhane, Beuran, Bhattacharyya, Bhutta, Biadgo, Bijani, Bililign and Sayeed1, Reference Katan and Luft2]. According to the location, amplitude, and severity of the injury, the stroke can cause a variety of impairment in motor, sensory, cognitive, perceptual, and language functions, with a consequent impact on daily life [Reference d. O. Ferro, d. S. Lins and Filho3, Reference Langhorne, Bernhardt and Kwakkel4]. The upper limb involvement is the most common disabling deficit being that approximately 70% of individuals present paresis in the upper limb with a consequent reduction in functional abilities [Reference Nakayama, Jørgensen, Raaschou and Olsen5, Reference o. Physicians6, Reference Sveen, Bautz-Holter, Sodring, Wyller and Laake7]. Reportedly, 50% of stroke survivors continue to experience upper arm disabilities after being discharged from the hospital [Reference Khor, Chin, Yeong, Su, Narayanan, Rahman and Khan8, Reference Loureiro, Harwin, Nagai and Johnson9].
Recent studies revealed a small and heterogeneous but significant effect of robot-assisted rehabilitation interventions on motor functions, upper extremity recovery, and activities of daily living, with an unclear clinical impact due to the relatively small effect size [Reference Ferreira, Chaves, Oliveira, Van Petten and Vimieiro10, Reference Mehrholz, Pohl, Platz, Kugler and Elsner11, Reference Veerbeek, Langbroek-Amersfoort, van Wegen, Meskers and Kwakkel12]. In this context, the use of robot-assisted therapy (RT) can offer an innovative approach to upper limb rehabilitation after stroke, involving intensive, repetitive, interactive, and individualized practice [Reference Brewer, McDowell and Worthen-Chaudhari13, Reference Duret, Grosmaire and Krebs14, Reference Sivan, O’Connor, Makower, Levesley and Bhakta15]. Furthermore, the great advantage of using this technology is the ability to control the dosage and intensity of treatment, offering high-intensity motor practices for long periods, consistently and accurately [Reference Mehrholz, Pohl, Platz, Kugler and Elsner11, Reference Yozbatiran and Francisco16].
Despite all the benefits of robotic therapy, most of the robotic orthoses for upper limb rehabilitation currently exhibit numerous disadvantages, limiting their application and their acceptance and restricting their use on a large scale [Reference Dunkelberger, Schearer and O’Malley17]. For example, they have unfavorable mechanical structures and esthetics because most devices are large, bulky, and heavy. These features make portability impossible or difficult, which is infeasible to in-home use [Reference Duret, Grosmaire and Krebs14, Reference Chang, Kim, Huh, Lee and Kim18, Reference Rahman, Rahman, Cristobal, Saad, Kenné and Archambault19, Reference Stein20, Reference Washabaugh, Guo, Chang, Remy and Krishnan21]. A portable device is defined as equipment that enables use in different environments, including the home, and allows the user to move during therapy [Reference Washabaugh, Guo, Chang, Remy and Krishnan21, Reference Manna and Dubey22]. Therefore, increasing accessibility, or extending robots to home settings, would benefit more stroke survivors and have a greater impact on rehabilitation.
This study aims to improve a portable robotic orthosis called Pinotti Portable Robotic Exoskeleton (PPRE) and validate its biomechanical functioning in individuals with upper limb motor impairment due to stroke. This apparatus is an evolution of the robotic orthosis developed at the Bioengineering Laboratory (LABBIO) of the Federal University of Minas Gerais, described in our previous study [Reference Ferreira, d. P. Rúbio, d. L. Brandão, d. Mata, d. Avellar, Bonfim, Tonelli, Silva, Dutra, Petten and Vimieiro23]. The position of instruments and the mechanical system were changed to increase the portable potential of the device. It is expected that the rehabilitation robot should be comfortable, esthetically pleasing, and able to provide multiple training movements while enlarging its portability, usability, and ensuring patient safety [Reference Khor, Chin, Yeong, Su, Narayanan, Rahman and Khan8, Reference Washabaugh, Guo, Chang, Remy and Krishnan21].
2. Methods
2.1. Device
The PPRE (Fig. 1) is an innovative proposal for upper limb rehabilitation, with advances about the apparatus commercially available. Competitive advantages relate to esthetics, structure, control system, portability, and concurrency of articulation. The orthosis is compact, made of light and resistant material, with an adequate weight/power ratio. It has a modular and adjustable structure (hand module and elbow module – work together or separately; all parts are pluggable and removable and enable size adjustments for shoulder, arm, and forearm). In addition, with its optimized design, the entire drive system is embedded in the orthosis, enabling portability and allowing the reproduction of daily functional tasks in different contexts and environments, including the home environment. This characteristic extends the use of the device to individuals who have difficulty in locomotion and are unable to go to the predefined locations for rehabilitation. Authors [Reference Ferreira, Chaves, Oliveira, Martins, Vimieiro and Van Petten24, Reference Kehayia, Swaine, Longo, Ahmed, Archambault, Fung, Kairy, Lamontagne, Dorze, Lefebvre, Overbury and Poldma25] state the importance of embedding the drive system and enabling portability. When rehabilitation occurs in favorable physical and social environments, there is an optimization of participation and social inclusion of individuals with physical disabilities.
Furthermore, the orthosis will be detailed and is divided into two parts: static orthosis and mechanical structure, the first one consisting of the parts that attach to the user’s limb and position it and the last one the other device components. Figure 2 shows the organizational form of the structure. Subsequently, the control system and the actuator’s motors will also be detailed.
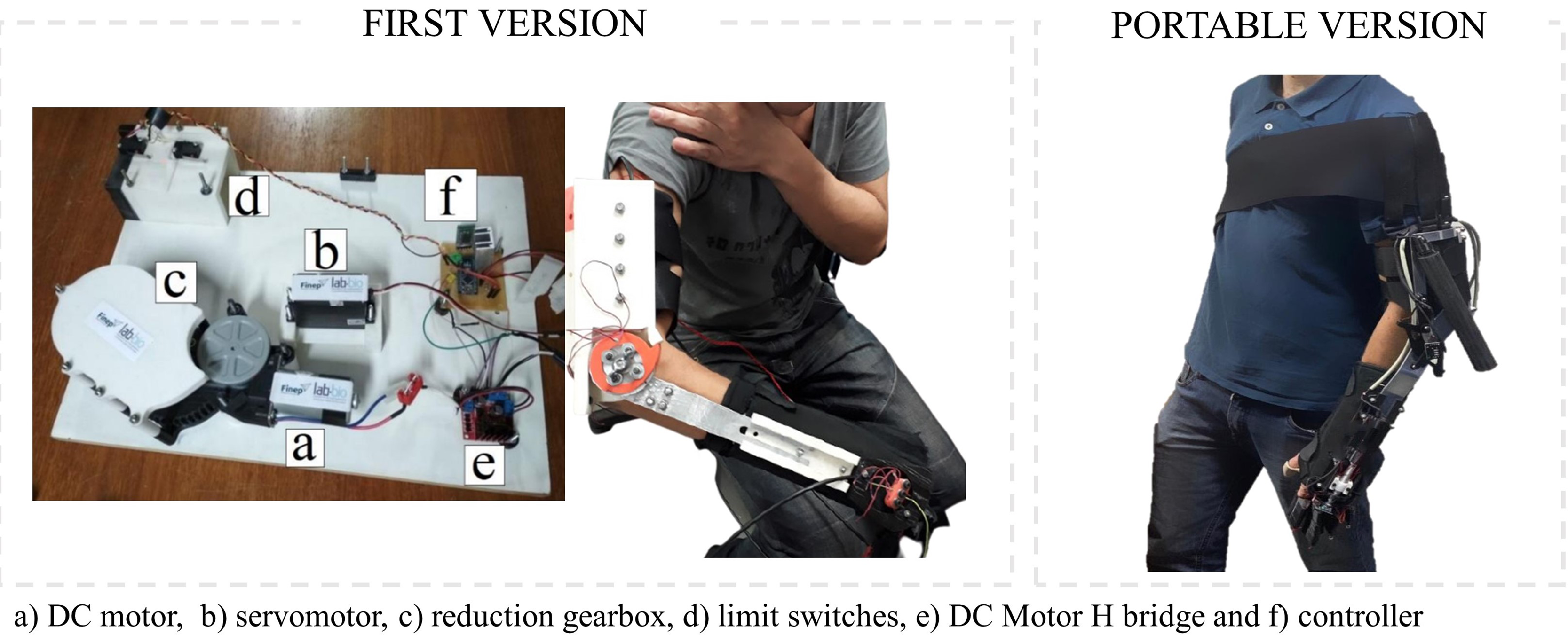
Figure 1. The first version and the current portable version of the Pinotti Portable Robotic Exoskeleton (PPRE).

Figure 2. Pinotti Portable Robotic Exoskeleton (PPRE) hand module and elbow module.
2.1.1. Elbow module: static structure
The elbow module static structure was composed of an arm splint and a shoulder pad. The position of the arm splint is on the triceps, and it has the function of supporting the mechanical parts of the actuators. It is formed by a custom-made thermoplastic splint molded on the participant’s arm and inserted in a Neoprene® wrapper. In order to allow a better fixation of the user’s arm to apparatus and prevent twisting of the elbow support structure [Reference Ferreira, d. P. Rúbio, d. L. Brandão, d. Mata, d. Avellar, Bonfim, Tonelli, Silva, Dutra, Petten and Vimieiro23], this structure has been extended in length, promoting an increase in the contact area with the upper limb.
The shoulder pad is a strip of Neoprene® that diagonally wrapped the user’s trunk. Its function is to prevent the slipping of the arm splint during the movements. In addition, it allows the weight distribution of the apparatus across the trunk. Due to the elastic and flexible characteristic of Neoprene®, the shoulder pad does not limit the range of shoulder movement.
2.1.2. Elbow module: mechanical design
The mechanical structure of the elbow module is composed of two parallel rods and support, providing structural reinforcement and attachment of motor in the body of the orthosis. The actuator mechanism of the elbow module is four-bar type as previously described [Reference d. P. Rúbio, Ferreira, d. L. Brandão, Machado, Tonelli, Kozan and Santos26]. This structure allows a maximum elbow amplitude (flexion) of 120
$^\circ$
and a minimum amplitude (extension) of 10
$^\circ$
. These values include most of the functional activities [Reference Neumann27]. Extreme amplitudes were avoided to not overload and stress the articular and ligament structures.
The transmission system of the elbow module also consists of a power screw [Reference Rúbio, Ferreira, Brandão, Machado, Tonelli, Martins, Kozan and Vimieiro28]. It acts as a physical limiter that prevents elbow movement from reaching undesirable angles. Besides that, it was necessary to develop a support for a two-stop sensor and an encoder to measure the elbow angles.
2.1.3. Hand module: static structure
The hand module static orthosis is composed of rigid splints made of thermoplastic in the ventral and dorsal forearm and pieces made of Neoprene® which casing the splints of the arm, forearm, and fingers. The rigid splints have the function of stabilizing and correctly positioning the forearm, the wrist, and the thumb in functional position, containing hypertonia and allowing the user to hold objects and perform successful daily activities [Reference Neumann27].
Motors were attached directly to the body of the orthosis to enable the portability of the apparatus. Therefore, it was necessary to add a lattice-shaped polylactic acid (PLA) structure above the dorsal static structure of the hand module to accommodate the mechanical components.
The linear rail located on the patient’s forearm and made of PLA was used to house the elbow rod and let it slide freely. Besides that, the linear rail connected the hand module to the elbow module. Figure 2 shows all components of the PPRE.
2.1.4. Hand module: mechanical design
The mechanical design of the hand module aims to perform the finger’s opening and closing movement, in a way that was permitted to the user grip objects during the rehabilitation sessions. To make this, the module owns a power screw transmission and ropes, called artificial tendons (AT), which were coupled in a mechanism with the use of an artificial phalanx (AP), as indicated in Fig. 2. The authors [Reference Ferreira, d. P. Rúbio, d. L. Brandão, d. Mata, d. Avellar, Bonfim, Tonelli, Silva, Dutra, Petten and Vimieiro23, Reference d. P. Rúbio, Ferreira, d. L. Brandão, Machado, Tonelli, Kozan and Santos26, Reference Rúbio, Ferreira, Brandão, Machado, Tonelli, Martins, Kozan and Vimieiro28] demonstrated the specifications and validations of the system previously.
Since post-stroke patients present spasticity and, consequently, difficulty opening the fingers, it was necessary to analyze the biomechanics and adjust the AT mechanism. Thus, an AT and AP set was developed [Reference d. P. Rúbio, Ferreira, d. L. Brandão, Machado, Tonelli, Kozan and Santos26]. In this mechanism, during the opening of fingers movement, the AT is tensioned, pulling the distal AP and leading it to rotation in its joint axis up to its collision with the middle AP. Maintained the traction of the AT, middle AP also rotated in its joint axis until the collision with proximal AP. It leads the system to rotate relatively of the proximal joint axis until the path limiter sensor has reached the transmission system or the AP has collided with the artificial metacarpal (AM). The principal advantage of this system is its safety because the most opening position is limited by the collision of the APs, which does not allow the biological unfunctional positions and does not harm the user. The closing passively movement was performed by the relaxation of the AT.
The APs are triangular pieces made by PLA, manufactured using 3D printers, and have a relative length with the user’s phalanx. The AM is also made by PLA and covers the user’s metacarpal fixing the module transmission system and blocking the proximal AP rotating movement. Finally, the AT is Kevlar ropes, which were selected as previously shown [Reference Rúbio, Ferreira, Brandão, Machado, Tonelli, Martins, Kozan and Vimieiro28].
In order to pull and relax the AT, a power screw transmission was designed [Reference d. P. Rúbio, Ferreira, d. L. Brandão, Machado, Tonelli, Kozan and Santos26]. In the transmission, stop sensors were placed to stop the movement when reached. In addition, an encoder, coupled to the screw, measured the rotation relative to the opening angle to control the position of the fingers.
2.1.5. Motors and control
Figure 3 shows a schematic diagram of the orthosis control and drive system. The parameters for performing the activities, such as repetitions, duration, speed, and amplitude of movement, are defined using an Android® application. The commands were sent to the control system via Bluetooth®. When processing the signal, the control board sends a drive signal to the actuators of the portable orthosis, which reproduces the movement. Feedback with the information collected by the sensors is sent to the control board for monitoring during the activity. This control system provides the signals acquired for display on the cell phone or computer.

Figure 3. Schematic of the rehabilitation robot system.
Due to the motors being attached directly in the orthosis, the priority was using motors with a good weight and torque ratio. Therefore, motors with a reducer coupled reduction were selected, with an output torque of 0.797 nm and a nominal output speed of 180 rpm. In addition, it works with 12 V of voltage and consumes a nominal current of 1.71 A. This set has a weight of 61 g and 16 mm diameter with 78 mm length. The servo motor controller board manages the operation of the motor, such as speed and direction of rotation.
For the control system, four snap action switches were selected as the stop sensor. In addition, two encoders had the function to measure the position of the system, and the microcontroller had the function to manage the system. Table I shows the instrument specifications. Additional technical details describing the device are similar to the previous version of the orthosis, and they are available in previous articles [Reference Ferreira, d. P. Rúbio, d. L. Brandão, d. Mata, d. Avellar, Bonfim, Tonelli, Silva, Dutra, Petten and Vimieiro23, Reference d. P. Rúbio, Ferreira, d. L. Brandão, Machado, Tonelli, Kozan and Santos26, Reference Rúbio, Ferreira, Brandão, Machado, Tonelli, Martins, Kozan and Vimieiro28].
Table I. Characteristics of the instruments.

The Android application, which controlled the device via Bluetooth, has a command menu where it is possible to select the module activated and perform manual or automatic tasks. Figure 4 shows a sequence of application screens for the activation of the hand module.
2.2. Device validation
Clinical tests verified the correct biomechanical functioning of the PPRE. These tests also evaluated the ability to perform flexion and extension movements of the elbow and fingers. Movement performance was assessed through the functionality and comfort of the orthosis, working at different angulations and speeds and in different activities. Grasping different objects, moving them on a table, and bringing them closer and away from the face are examples of developed activities. In addition, the ease of installation and operation of the apparatus was also verified.

Figure 4. Orthosis control application during a movement using only the hand module.
2.2.1. Participants
The recruitment of participants to validate the device was in stroke associations, rehabilitation centers, hospitals, and social media. Participants were selected according to the inclusion and exclusion criteria and informed about the study’s objectives. The inclusion criteria in this study were individuals aged 18 years or older with a diagnosis of stroke in the chronic phase (evolution time above 6 months) [Reference Wechsler, Bates, Stroemer, Andrews-Zwilling and Aizman29]; hemiparetic, characterized by increased tone of the elbow flexors (score other than zero on the Modified Ashworth Scale) or by handgrip muscle weakness (difference more significant than 10% between the measurement of the paretic and non-paretic limbs), measured by Hydraulic Hand Dynamometer® (Model SH5001, Saehan Corporation, Masan, Korea) [Reference Faria-Fortini, Basílio, Polese, Menezes, Faria, Scianni and Teixeira-Salmela30]; upper extremity Fugl-Meyer (FM) score
$\geq$
11 and
$\leq$
39 at the time of screening [Reference Fugl-Meyer, Jääskö, Leyman, Olsson and Steglind31]; movements of at least 45
$^\circ$
of flexion and abduction of the shoulder – minimum movement necessary to perform most activities of daily living [Reference Oosterwijk, Nieuwenhuis, van der Schans and Mouton32]; a complete passive range of motion of the elbow, hand, and fingers; without impairment of proprioceptive sensitivity assessed using the FM sensitivity domain [Reference Fugl-Meyer, Jääskö, Leyman, Olsson and Steglind31, Reference Fagundes, Binda, Faria, Peres and Michaelsen33] and without severe cognitive deficits, assessed using the Brazilian version of the Mini-Mental State Examination (the cutoff for illiterate individuals is 13, 18 for low and medium schooling, and 26 for high schooling) [Reference Bertolucci, Brucki, Campacci and Juliano34] demonstrating skills to follow instructions to perform motor tasks.
Individuals were excluded with a bilateral motor impairment; excessive spasticity of the affected limb (Modified Ashworth Scale > 3) [Reference Bohannon and Smith35]; severe additional orthopedic or rheumatological impairment, before stroke preventing or limiting the use of the orthosis; severe pain in the affected upper limb, measured using the Visual Analog Scale (VAS) (>8 on a scale of 0 to 10) [Reference Langley and Sheppeard36]; open lesions on the skin where the device will be attached; use of botulinum toxin in the last 3 months for spasticity or other drugs known to increase recovery motor, as well as participation in the last 3 months of another research study to improve upper limb function. After selection and the participants’ consent, they signed the Informed Consent Form (ICF). The Research Ethics Committee of the Federal University of Minas Gerais approved this study project (CAAE Registry: 22207213.5.0000.5149).
2.2.2. Procedures
The study was carried out in the Bioengineering Laboratory (LABBIO) premises at the Federal University of Minas (UFMG). The responsible researcher (occupational therapist), accompanied by mechanical engineers, performed the tests with the orthosis.
The main objective of this initial clinical trial was to validate the device in terms of its functioning. Participants sat on a chair in front of a height-adjustable table. The orthosis was fixed on his upper limb. In this fixation, those responsible for the test evaluated the anatomical adjustments, possible pressure points on the skin, postural adequacy, safety, and comfort of each participant. The VAS [Reference Langley and Sheppeard36] was used to investigate the occurrence of pain and adverse events.

Figure 5. The change in the center of gravity after the structure modification.
The correct biomechanical functioning with the ability to perform flexion and extension movements of the elbow and fingers efficiently and comfortably in a different range of motion angulation and speed was analyzed. In addition, the gripping of different objects moves them on the table and brings them closer and away from the face. The angle of opening of the elbow and finger was measured using a plastic PVC Carci goniometer to verify the system’s angles. Furthermore, consistency and accuracy in the opening angle were observed by verifying what was requested by the system and the angle measured by the goniometer. The time of opening of the fingers, the elbow, and the installation of the device on the participant’s upper limb was measured using a Digital chronometer model AK68. The weight of the equipment was verified using a Baxtran® precision balance. In addition, the ease of installation and operation of the equipment was also analyzed by measuring the time required to install the equipment in the participants’ upper limbs and to adjust the ATs, using a Digital chronometer model AK68.
The tests lasted 2 months, with 1 month of initial contact with the device and 1 month of testing with the apparatus for adjustments and functionality evaluation, and were performed weekly in 1-h sessions. In all, each participant performed eight sessions. Each session had approximately 20 repetitions of the flexion and extension movement of the elbow and fingers. The movements could involve the elbow, the fingers, or both. The tests were filmed for further analysis from researchers and the exact identification of the main problems to be modified. Despite the video analysis, the participants’ feedback, with questions during the use of the device, was essential in this stage.
3. Results
The previously developed orthosis [Reference Ferreira, d. P. Rúbio, d. L. Brandão, d. Mata, d. Avellar, Bonfim, Tonelli, Silva, Dutra, Petten and Vimieiro23] was modified to improve biomechanical function, portability, comfort, and versatility. Adaptations to the structure allowed a change in the center of gravity, as shown in Fig. 5, and a reduction in weight (172 g). In addition, the new structure allowed the coupling of motors to the orthosis. Consequently, it provided the device’s portability since the integration of the motors to the orthosis, together with other characteristics such as low weight and volume, favors its use in any environment. The apparatus was weighed with a precision scale and found 1.390 kg, with 0.820 kg in the elbow module and 0.570 kg in the hand module. These values refer to the structures under the individual’s arm. However, part of the orthosis is a battery and control board in an external compartment, inside a backpack, and weighing 0.217 kg.
Four subjects performed clinical trials to validate these modifications. The average age of the participants was 46
$\pm$
17.59 years, 50% were men, and the average post-stroke time was 19.83
$\pm$
7.17 months. It is noteworthy that the mean age is not a negative impact parameter as age does not negatively influence upper limb recovery after robotic therapy [Reference Cecchi, Germanotta, Macchi, Montesano, Galeri, Diverio, Falsini, Martini, Mosca, Langone, Papadopoulou, Carrozza and Aprile37]. Table II shows the sociodemographic and clinical characteristics.
Table II. Characteristics of the participants.

Tests showed no changes in the skin (pressure point on the skin, flushing) of the upper limb while using the device. In addition, the participants did not mention pain (mean score 0 points on the VAS), discomfort, tingling, and paresthesia. It was found that the static components of the hand module were able to position the limb properly. The static components also containing the hypertonia of the flexor musculature of the wrist and hand fulfill the purpose for which it was intended. The shoulder pad promoted an efficient fixation of the orthosis to the patient’s upper limb, preventing the device from slipping, in addition to allowing weight distribution throughout the trunk, avoiding imbalance.
The participants could perform flexion and extension movements of the elbow and fingers in a different range of motion, angulation, and speed. In addition, they were also apt to hold and support different objects (Fig. 6), as well as move them on a table and bring them closer to and away from the face. The hand module performed the total opening of the fingers (90
$^\circ$
in the metacarpophalangeal joints) in 2.32 s, with the maximum speed. The use of a goniometer (as shown in Fig. 2) confirmed the flexion range actively performed by the participants. The extension was performed by the device going from 90
$\mathrm{^\circ }$
to 0
$\mathrm{^\circ }$
, with a free range of motion of 90
$\mathrm{^\circ }$
. It also was observed that the fingers presented consistency and precision in the opening angle, making repeated partial openings of 80
$^\circ$
, 60
$^\circ$
, and 40
$^\circ$
.

Figure 6. Grasping of different objects.
The mechanical structure of the elbow module, with double support, enabled movements of flexion and extension of the elbow correctly, respecting the physiological and biomechanical characteristics of the limb, without causing twisting of the elbow support structure, as seen in the previous study [Reference Ferreira, d. P. Rúbio, d. L. Brandão, d. Mata, d. Avellar, Bonfim, Tonelli, Silva, Dutra, Petten and Vimieiro23]. It was observed in this module that the equipment was able to perform movements from 10
$\mathrm{^\circ }$
to 120
$\mathrm{^\circ }$
of flexion, with an arc of free movement of 110
$\mathrm{^\circ }$
in 4.14 seconds with the maximum speed.
Figure 7 confirms the results. It is possible to observe the complete opening of the fingers of a study’s volunteers using the robotic orthosis, which is not possible without the device, through only voluntary control. This image shows the device’s functionality, demonstrating correct biomechanical functioning and validating the orthosis PPRE.

Figure 7. Participant opening fingers: (a) without therapy, (b) human therapy, and (c) robotic therapy using the PPRE.
The device proved to be adequate and functional at different levels of motor impairment assessed. The equipment was tested on four participants with different stages of motor recovery, measured by FM, and levels of spasticity, measured by the Modified Ashworth Scale (see Table 1). In all cases, the ability to perform movements was observed correctly. In addition, it verified the device’s ease of installation and operation to the user’s member (average time spent on the dressing process, installation, and regulation of ATs was 4 min 35 s). The versatility resulting from the presence of a modular structure contributed to this practicality. However, the participation of a professional (therapist) was needed to mold the rigid splints, wear the orthosis on the patient, and operate it with good graduation of the parameters (speed, angulation, time).
The PPRE orthosis’s advantages were confirmed by comparing results obtained with currently available devices. The summary of elbow-only, finger-only, and elbow-and-finger orthoses is presented in Tables III, IV and V, respectively.
Table III. Summary of the characteristics of orthotics with flexion and extension movements of the elbow.

Table IV. Summary of the characteristics of orthotics with flexion and extension movements of the fingers.

Table V. Summary of the characteristics of orthotics with flexion and extension movements of the elbow and fingers.

Although there is much robotic equipment designed and a considerable amount of research, few portable exoskeletons are available for users. This limited the comparison to the few devices presented in Tables III, IV and V. The main reason is due to the difficulty in choosing actuators and support mechanisms to develop lightweight exoskeletons that allow transport and use in different environments [Reference Manna and Dubey22].
The most widely used commercial portable robotic orthosis currently in use, the MyoPro (Myomo Inc., Cambridge, Massachusetts), performs elbow and finger flexion and extension movements, with a torque of 7 nm and 1.27 nm, respectively, weighs 1.81 kg [Reference Dunaway, Dezsi, Perkins, Tran and Naft64]. The portable robotic orthosis called hybrid elbow orthosis performs elbow movements only, with a torque of 6.5 nm, weighs 1.2 kg [Reference Pylatiuk, Kargov, Gaiser, Werner, Schulz and Bretthauer38]. Therefore, it can be observed that the PPRE (1,607 kg) has a similar weight to those currently available. Motors’ active drive did not impact the final weight as most devices use the passive, hydraulic, or pneumatic drive. Furthermore, the PPRE orthosis provides the highest torque at the elbow, equivalent to 12 nm, providing the highest torque density of all devices. These values are within expectations since, to perform activities of daily living, a torque of 5.8 nm is required at the elbow [Reference Murray and Johnson65]. Some devices, such as Cadel [Reference Zuccon, Bottin, Ceccarelli and Rosati39] and Portable Elbow Exoskeleton [Reference Manna and Dubey22], do not reach this minimum value. Although the PRRE orthosis elbow flexion and extension movement speed are lower than that of other devices, clinical tests indicated that the speed is sufficient to perform the tasks within the user’s expectations.
Regarding the torque of the fingers, the PPRE orthosis has a lower torque (0.797 nm) than the commercial ones. However, this is not a negative point since this torque is sufficient to carry out activities of daily living, as shown in clinical trials. Furthermore, the weight of the PPRE orthosis together with the Polygerinos [Reference Polygerinos, Wang, Galloway, Wood and Walsh52] is the only one close to the 0.5 kg limit, indicated as the maximum limit by ref. [Reference Aubin, Sallum, Walsh, Stirling and Correia66]. On the other hand, the PPRE torque is lower than the others, indicating that the lowest weight does not represent the highest torque density.
When comparing orthoses that feature both the flexion and extension movement of the fingers and the elbow (Table V), the PPRE orthosis provides the least weight, emphasizing potential portability. Therefore, the proposed device presents advantages compared to available devices, showing functionality, usability, biomechanical functioning, and versatility.
4. Discussion
Tests carried out with the PPRE device demonstrated good biomechanical functioning. The participants were able to efficiently perform flexion and extension movements of the elbow and fingers, together and separately, and grasp different objects. For example, the apparatus offered the amplitudes of 110
$^\circ$
in the elbow, being that to perform most of the delivered activities requires a functional arc of 100
$^\circ$
in the elbow [Reference Neumann67].
The mechanical system of the orthosis allowed controlled movements and forces to be supported in the active rehabilitation process under the command of an application. According to ref. [Reference Manna and Dubey22], a portable upper limb orthosis must be able to incorporate exercises for individuals with different levels of motor impairment and in different stages of recovery. The tests of the PPRE device were performed on stroke patients with moderate to severe impairment, and it is possible to reproduce them in patients with mild impairment of the upper limb. Then, the offer of an orthosis for RT, from the initial stages to the final stages of rehabilitation for a better recovery rate, has been achieved. It is noteworthy that some portable robots can act with passive rehabilitation in the final stages of recovery [Reference Washabaugh, Guo, Chang, Remy and Krishnan21]. Implementing sensors with closed-loop control of the PPRE orthosis torque will allow passive performance in the rehabilitation process. In addition, considering that stroke patients are concerned with their ability to perform daily activities [Reference Khor, Chin, Yeong, Su, Narayanan, Rahman and Khan8], tasks developed during clinical tests indicate the potential of the PPRE orthosis for day-to-day use in a home environment.
Nevertheless, the uni and bilateral tasks emphasized the active use of both upper extremities and the aid from one end to the other during the execution of the task, making the device more versatile [Reference Page, Hill and White58]. Moreover, it is shown that orthosis promoted finger movement, which is the most effective in the rehabilitation process [Reference Conti, Allotta, Meli and Ridolfi68, Reference Morone, Cocchi, Paolucci and Iosa69]. It confirms the potential of using the device as a portable orthosis [Reference Manna and Dubey22].
Regarding the changes made to previous work [Reference Ferreira, d. P. Rúbio, d. L. Brandão, d. Mata, d. Avellar, Bonfim, Tonelli, Silva, Dutra, Petten and Vimieiro23], the main differences were the implementation of a compacted set and the elbow structure to couple the system in the individual’s limb. Implementing a double-sided structure presented the user with a low perception of mass due to the proximity of the center of gravity (CG) of the device to the CG of the arm. This way, users did not complain about the excess weight of the apparatus, as observed in the previous study. Another point observed was the torsional movement in the structure due to the pronation of the volunteers’ arms. The load was distributed on both sides of the armrest through a double lateral structure, solving the problem. These adaptations added to portability and the satisfactory weight and volume reinforce the differential of the prototype since, despite the number of exoskeletons currently available to be high, most are not portable due to the type of actuators and mechanisms used [Reference Manna and Dubey22]. In addition, PREP makes it possible to maintain the alignment between the axis of the activated joint and the axis of functional rotation of the elbow, avoiding contact pressures, discomfort, or irritation [Reference Ercolini, Trigili, Baldoni, Crea and Vitiello70].
The electromechanical actuators that provide the necessary forces are generally heavy and require high power, limiting portability [Reference Stewart, Pretty and Chen71]. However, the actuators used in the orthosis meet biomechanical requirements without compromising portability. The system provides maximum torque in the elbow module that is greater than the required torque for daily activities [Reference Murray and Johnson65]. In addition, the torque values allow the device to be used by patients with any level of spasticity, confirming the possibility of using the PPRE orthosis in patients with different levels of upper limb involvement.
The presence of two modules (elbow and hand) in the orthosis reinforces the concept of a modular device. A reconfigurable and modular rehabilitation robot can be more efficient and economical, but it is challenging to increase its portability [Reference Khor, Chin, Yeong, Su, Narayanan, Rahman and Khan8]. Thus, the PPRE orthosis presents a new modulation proposal without losing the characteristics necessary for portability [Reference Khor, Chin, Yeong, Su, Narayanan, Rahman and Khan8], since the use of modules does not restrict the use of the orthosis, and it is possible to use it anywhere. Furthermore, the therapist responsible for the treatment can adjust the system parameters to change the amount of mechanical assistance the device provides as needed [Reference Page, Hill and White58]. This versatility allows for a greater range of movement during rehabilitation. Consequently, the patient can train individual movements with different configuration options to simulate daily activities [Reference Khor, Chin, Yeong, Su, Narayanan, Rahman and Khan8], reinforcing the assistive potential of the device. In this way, it will provide, besides an improvement in motor recovery, a functional improvement [Reference Basteris, Nijenhuis, Stienen, Buurke, Prange and Amirabdollahian72, Reference Vaida, Carbone, Major, Major, Plitea and Pisla73]. In addition, the apparatus can be adapted for different users who need specific training for a certain period, with infrequent changes, adding or connecting the modules as they progress in the rehabilitation process [Reference Khor, Chin, Yeong, Su, Narayanan, Rahman and Khan8]. It can have a positive impact on the setup time and cost of rehabilitation [Reference Stewart, Pretty and Chen71].
The modular characteristic also contributes to the ease of placing and removing the device, confirmed by the time spent during the dressing process. In terms of safety, the joints have a mechanical system that prevents an operational range above the anatomical limits, restricting the movement of the joints [Reference Manna and Dubey22]. Considering that the device has human–machine interaction, this design parameter becomes even more relevant [Reference Khor, Chin, Yeong, Su, Narayanan, Rahman and Khan8]. Besides, the application has a communication interrupt button, and the electronic board has an emergency button to de-energizes the entire system that can be activated at any time. Therefore, communication between the orthosis and the control driver or software is monitored continuously. These points are another positive factor of PREP given that the functional activity requires rehabilitation therapy that not only provides medical benefits but also ensures comfort and safety for users [Reference Manna and Dubey22].
Using an application provides other advantages besides security. Although post-stroke individuals tends to be older and possibly do not make as much use of computer software, an application on a robotic device can simplify the use of the device. Furthermore, the proposal of an intuitive interface for a robotic therapy device can provide protocols that are easier to follow [Reference Khor, Chin, Yeong, Su, Narayanan, Rahman and Khan8]. The presence of Bluetooth® for remote control of the device provides better control of the settings by the therapist [Reference Page, Hill and White58]. In Myomo Orthosis [Reference Page, Hill and White58], the app’s functionalities allow people with impaired upper limbs to participate in functionally relevant tasks while using robotic therapy independently. Consequently, the use of the application tends to increase acceptance by users, assist the therapist’s work, and increase adaptation to new robotic rehabilitation devices. It corroborates the idea that the software-based solution is the best option for portable orthoses [Reference Manna and Dubey22].
Regardless of the results of this study indicating a high potential of the PPRE orthosis for its assistive use, further clinical tests are needed to assess and quantify the effects of use on upper limb rehabilitation. The randomized controlled clinical trial should be performed on a large and heterogeneous sample over a more extended period. For this, the orthosis will be improved with the development of the wrist and thumb joints. An adjustment protocol for use outside the clinical environment will be created given that there is currently a need for a health professional to shape the rigid splints, wear the orthosis on individuals, and operate it with appropriate graduation of parameters (speed, angulation, time). Objective (kinematic as a quantitative outcome measure) and subjective (behavioral) assessments can be implemented. Finally, it is suggested to use functional electrical stimulation to replace the motors of the hand module, transforming it into a hybrid robotic system, thus alleviating the energy demand of the robotic device and reducing the weight of the system.
5. Conclusions
The existing robotic strategies aimed at the upper limbs are limited by their effectiveness, portability, and integration into daily activities. This study presents a new and promising portable robotic device that differentiates it from other technologies in the world. The apparatus can assist the movement of the upper limb of people with motor impairment, expanding their functional skills, and making them more independent, with greater autonomy and quality of life. The mechanical structure of the orthosis has been changed to a previous study. One of the consequences was the better distribution of the mass, correcting the discomfort of the weight presented in the previous study. Furthermore, changes were implemented in terms of material and mechanical layout. Then, the adaptation of the motor coupling system made it possible to use orthosis in different environments. Through the clinical test, the robotic orthosis was validated, demonstrating correct biomechanical functioning, the ability to pick up and hold objects, and ease of installation and operation of the device. The device proved suitable and safe for use in different contexts and environments. As a significant differential, the potential for portability for home use stands out. In a larger sample, future studies are needed to assess the effect of robotic orthosis on the level of rehabilitation in individuals with upper limb impairment. Future results can favor the resumption, as much as possible, of the previous level of functionality of people with disabilities and their active involvement in meaningful life roles.
Acknowledgments
This prototype was named in memory of Professor Marcos Pinotti Barbosa (in Memoriam). The authors would like to thank Professor Daniel Neves Rocha and the Instituto Federal de Minas (IFMG) for contributing to developing the smartphone application for the portable robotic orthosis.
Financial support
This work was supported by Coordenação de Aperfeiçoamento de Pessoal de Nível Superior – Capes (grant number 001); Conselho Nacional de Desenvolvimento Científico e Tecnológico – CNPq (grant number 203345/2019-3); and Financiadora de Estudos e Projetos – FINEP (grant number 01.12.0476.01).
Author contributions
Conceptualization: Fernanda Marcia Rodrigues Martins Ferreira; Guilherme de Paula Rúbio; Rina Mariane Alves Dutra. Data curation: Fernanda Marcia Rodrigues Martins Ferreira; Guilherme de Paula Rúbio. Writing and editing: Fernanda Marcia Rodrigues Martins Ferreira; Guilherme de Paula Rúbio; Rina Mariane Alves Dutra. Supervision: Claysson Bruno Santos Vimieiro; Adriana Maria Valladão Novais Van Petten. Resources: Claysson Bruno Santos Vimieiro. Project administration: Claysson Bruno Santos Vimieiro.
Conflicts of interest
The authors declare none.