Introduction
Over the last 20 years, radiotherapy (RT) has played a significant role in the treatment of head-and-neck cancers. About 74% of neck-and-neck patients need to undergo either definitive or postoperative radiation therapy.Reference Ghosh, Gupta, Malviya and Saroj1,Reference Delaney, Jacob and Barton2 The radiation delivery technique is making progress with transition from two-dimensional conventional radiotherapy (2D-RT) to three-dimensional conformal radiotherapy (3D-CRT), field-in-field (FIF) and at present intensity-modulated radiation therapy (IMRT).Reference Ercan, Iğdem and Alço3 IMRT is one of the most common delivery techniques that is known for providing accurate, reliable and more homogenous and conformal dose to the planning target volume (PTV). IMRT is delivered mostly with multi-leaf collimators, and sometimes with compensators.Reference Bortfeld4 Although IMRT is widely implemented in many cancer centres and hospitals, some other techniques such as 2D, 3D-CRT and FIF are still in use. FIF and IMRT techniques are mostly used at Vietnam cancer centres so that the photon field can be adjusted to conform to the irregularly shaped target volume with extremely high precision.Reference Tai, Son, Loan and Anson5,Reference Tai, Son, Loan and Trang6 This can reduce the radiation delivered to the surrounding organs-at-risk (OARs) such as spinal cord, brainstem, parotid glands, eyes, optic nerves, optic chiasma and mandible in head-and-neck cancer.Reference Nishimura and Ritsuko7,Reference Mundt and Roeske8 The IMRT technique reduces the toxicity level at the OARs. Acute and late toxicity are also reduced significantly. In fact, the ability of sparing the OARs while maintaining or increasing the dose coverage at the target volume makes IMRT the better treatment option than the FIF. The comparison of IMRT and FIF techniques has been published in research works.Reference Hosseini and Momennezhad9,Reference Koksal, Kesen and Akbas10 Hosseini et al.Reference Hosseini and Momennezhad9 performed the dosimetric comparison of conventional and FIF techniques in early-stage breast cancer RT. They concluded that FIF technique produced significantly lower dose homogeneity index (HI), lower maximum doses and higher median doses in PTV than 3D-CRT. Koksal et al.Reference Koksal, Kesen and Akbas10 compared the differences of the dosimetric parameters between 3D-CRT and simultaneous-integrated boost intensity-modulated radiotherapy (SIB-IMRT) techniques in the prone and supine positions for breast irradiation. Their results showed SIB-IMRT allowed a more conformal dose distribution regardless of position than 3D-CRT. Recently, the FIF and IMRT techniques have been firstly applied in Baichay Hospital, Vietnam. While studies related to comparison of the IMRT and FIF techniques have been published in breast RT,Reference Ercan, Iğdem and Alço3,Reference Herrassi, Bentayeb and Malisan11 there is no work undertaking the dosimetric comparison between the IMRT and FIF technique for head-and neck RT. The objective of this study is to compare the differences of dosimetric parameters of IMRT and FIF techniques for head-and neck-cancer using Elekta Monaco treatment planning system (TPS).
Materials and Methods
Treatment planning
A total of 20 cases of head-and-neck cancer patients with stages I–IVA including 10 nasopharynx cases, 5 hypopharynx cases and 5 oral cavity cases were treated by prescription of 60–70 Gy at Baichay Hospital. Patients were scanned by Somatom 16-slice computed tomography (CT) scanner (Siemens Medical System, Germany). Parameters of CT imaging for all patients were scanned from head to upper thorax, above orbits, to 5 cm below collarbone with 2-mm slice thickness and the photon beam voltage was 130 kVp. The head-and-neck immobilisation device for the patients included iBeam Overlay with shoulder retractors and thermoplastic mask. After the simulation, the data were transferred to the TPS through Digital Imaging and Communications in Medicine (DICOM). The Monaco TPS (Elekta, Stockholm, Sweden) was used in this study. Each patient was planned using both FIF and dMLC-IMRT techniques after radiation oncologist defined the target and OAR12 and dMLC-IMRT techniques were selected for treatment.
dMLC-IMRT technique
A total of 20 plans were created using Monte Carlo algorithm as the dose calculation engine. The plans used the 6-MV photon beams with the isocenters at PTVC (PTVC = PTV1 + PTV2 + PTV3), in which PTV1s were defined as the primary target volume, PTV2s were defined as the treatment volume of high-risk nodal and PTV3s were defined as low-risk nodal. dMLC-IMRT plans were created using seven photon fields with gantry angles equal to 0, 51, 102, 153, 204, 255 and 306°.
FIF technique
A total of 20 plans were created with dose distribution calculated by the collapsed cone algorithm, based on the 6-MV photon beams. The isocenter was defined as a marker at the coronal slice of PTV3 (which is after PTV2). FIF plans were created using six photon fields with five gantry angles 180, 125, 90, 270 and 230° for PTV2 and PTV3, and one field with a gantry angle at 0° for PTV1. Smaller fields were created inside a big field as beam segments. Two to four segmental fields were created in a field to decrease the dose at OAR and increase the dose at the target. Patients were prescribed 66–70 Gy for the target and the dose limitation or OAR followed the RTOG-0225 protocol.Reference Lee, Kramer and Xia13
Treatment planning evaluation
Clinically approved treatment plans were reviewed and restored from the TPS. Normal tissue avoidance planning was based on both the OAR hard constraints and individualised OAR planning objectives specified by the radiation oncologist. Treatment plans were evaluated on the basis of the dose distribution of target on each slice and the dose–volume histograms (DVHs). The treatment plans were optimised to ensure 95% of the PTVs (D95%), and 98% of the clinical target volume (D98%) received the prescription doses. Dosimetric data included the volume of PTV receiving greater than 95–107% of prescribed dose (V95% and V107%); the dose delivered to 98% (Dnear-min, D98%) and 2% (Dnear-max, D2%) of the PTV; and mean dose of the PTV (Dmean) from the DVHs [3]. HI and conformity index (CI) were calculated according to definition proposed by the International Commission on Radiation Units and Measurements (ICRU) Report 83.12
CI is defined as the ratio of volume of tissue receiving at least 95% of the prescribed dose divided by the volume of the PTV. The plan is considered more conformal if CI is near to 1:12,Reference Paddick14

HI is defined as the difference between the near-maximum and near-minimum dose normalised to the median dose:12

where D2% and D98% are the doses received by 2% (Dnear-max) and 98% (Dnear-min) volume of PTV, respectively, and Dp is the prescribed dose. The ideal value of HI is zero; an HI value approaching zero indicates a more homogenous dose distribution within the PTV and it increases as homogeneity decreases.
Statistical analysis
Statistical analysis was performed using SPSS Statistics 22 (IBM SPSS, Chicago, IL, USA) to assess statistical significance between FIF and IMRT plan. The differences were considered statistically significant at p-values < 0·05.
Treatment plan verification
Quality assurance (QA) plans before treating
The OmniPro I’mRT+ software, the MatriXX detector and MULTICube Phantom were used to evaluate the plan accuracy, ensuring the safe and accurate delivery of a prescribed radiation dose. A plan specified for QA was created for each IMRT treatment plan and transferred to the linear accelerator console for re-treatment delivery.
The MatriXX Evolution consists of 1,020 air-vented ionisation chambers located on a 32 × 32 grid. Each of the 2D array ionisation chambers has a sensitive volume, diameter, height and detector spacing of 0·08 cm3, 4·50, 5·00 and 7·62 mm, respectively. It operates at a voltage of 500 V and has a sensitive area of 24 × 24 cm2. The MatriXX Evolution functions as pretreatment QA system whereby it verifies the patient’s treatment plan offline (Figure 1). Our established constraint is that IMRT plan will be accepted with a pass rate of 95% at gamma criterion of 3%/3 mm. Otherwise, IMRT plan will be considered to be failed and be recreated.

Figure 1. MatriXX Evolution and MULTICube Phantom.
Results
A dosimetric comparison between IMRT and FIF
The dose distributions on the transverse and coronal plane for IMRT and FIF plan on the same patient are shown in Figure 2. It shows that the isoline dose coverage of IMRT plan is better than FIF plans. Furthermore, as shown in Figure 3, the IMRT plans also showed a stronger capability of controlling the hot spots (V107%), and the OAR sparings of IMRT plan, such as parotid gland and tongue, are significantly reduced when compared to FIF plan. It can be seen that the dose coverage of PTVs, the capability of controlling the hot spots and the normal tissue sparing of IMRT plan are significantly better than the FIF plan, in particular, parotid glands in the IMRT plans are reduced about a half when comparing to FIF plans.
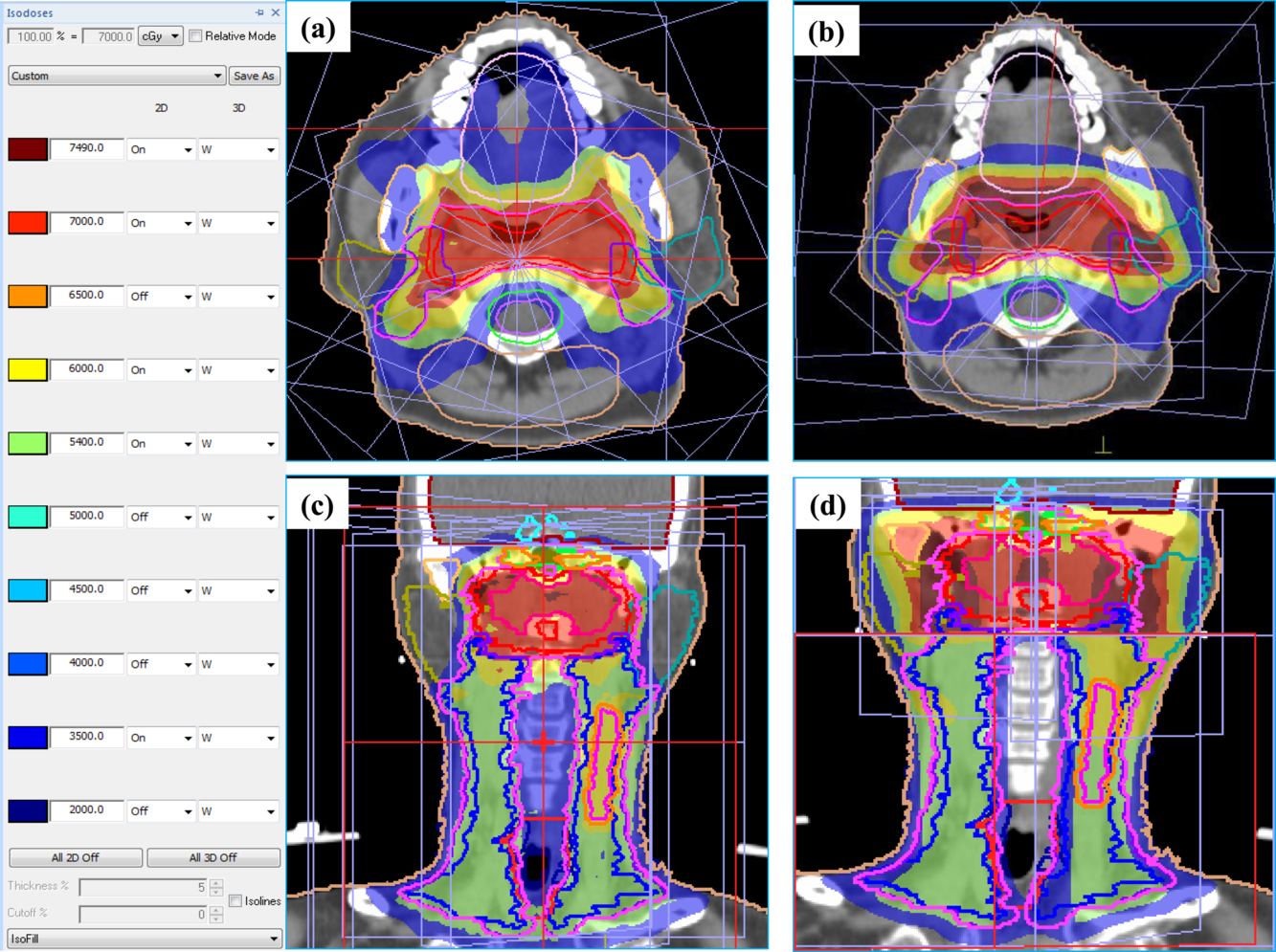
Figure 2. Comparison of dose distribution IMRT (a, c) and FIF plan (b, d) on transverse and coronal plane.

Figure 3. Comparison of DVHs of one patient in the group for the IMRT and FIF plan.
Treatment plan verification
Figure 4 shows an example of the analysis of clinical case as acquired with the MatriXX. A total of 20 treatment IMRT plans were verified with MatriXX Evolution and had the pass rate ≥ 95% at gamma criterion of 3%/3 mm. The results are displayed in Figure 5.

Figure 4. The results of treatment plan verification with MatriXX.

Figure 5. Gamma pass rate measured with MatriXX.
Discussion
In Table 1, the total treatment monitor units (MUs) of IMRT plans were approximately double as compared to FIF plans. The patients treated by IMRT technique needed to lie longer on the treatment couch; the patients also had to be immobilised longer and be provided with a reliable means of reproducing the patient’s position from one treatment to another. Modern RT such as IMRT generally requires additional immobilisation accessories during the treatment of patients. IMRT plans were found to provide more homogenous dose distribution within the PTV than the FIF plan by an average of HI = 0·08 and 0·19, respectively. The average of CI values of IMRT plans showed a better PTV coverage by 0·981 comparing to 0·975 for the FIF plans. IMRT doses to the surrounding healthy tissues and critical structures displayed in Table 2 such as the spinal cord, brainstem, parotid glands, lens, eyes, optic nerves, optic chiasm and mandible were reduced significantly compared to FIF plans. When following recommendations for the mean dose of parotid glands and oral cavity, 50% of parotid gland volume did not receive a mean dose greater than 26 Gy. If the parotid gland volumes received a mean dose of more than 50 Gy, they will be seriously damaged. The mean parotid gland doses for patients treated with IMRT were significantly lower compared with the mean parotid gland doses of patients treated with conventional RT (Mann–Whitney, p = 0·016). Numerous studiesReference Chao, Deasy and Markman15–Reference Parliament, Scrimger and Anderson21 have also reported significant correlation between the mean parotid dose and salivary flow after RT and the rate of patient suffering from xerostomia. Their results clearly demonstrated the superiority of IMRT technique in terms of toxicity, mainly due to the parotid gland sparing. Tham et al.Reference Tham and Jiade22 performed a study of two patient groups including 26 cases treated by conventional technique and 30 cases treated by IMRT technique. Tham et al. found that the mean doses of the parotid glands were 48·1 Gy for the group using conventional technique, and 33·7 Gy for the group using IMRT technique, respectively. After 6 weeks of treatment, the patient groups were treated by IMRT and conventional technique who had side effect by 55 and 87%, respectively. Our study showed that the mean dose of parotid glands for IMRT plan is reduced 1·8 times compared to the FIF plans (by 27·27 and 48·69 Gy, respectively).
Table 1. Summary of dosimetric comparison of IMRT and FIF

Table 2. Maximum dose (Dmax) and mean dose (Dmean) for OARs sparing

In some Vietnam oncology centres, oral cavity is rarely considered as a structure to reduce the dose. However, according to published researches, the mean dose of oral cavity sparing was reduced under 40 Gy that improved the toxicity level.Reference Bortfeld4,Reference Lee, Kramer and Xia13,Reference Tai, Oanh, Son, Loan and Chow23,Reference Paudel, Narayanasamy and Han24 Our study showed that the mean oral cavity dose of IMRT plans was reduced significantly compared with FIF plans with 37·8 and 43·24 Gy, respectively.
The IMRT technique is relatively new but not widely used as 3D-CRT, FIF in our hospital, but it is a valuable tool for clinics to consider adopting in the future. IMRT is limited to the use of a fix number or radiation beams during the therapy. Also, this technique surged treatment delivery time that may cause problem in dose accuracy due to MLC movement and scattering of small subfields. As a single field is further divided into subfields, this technique provides a more homogenous and conformal dose to the target volume, with less dose to the surrounding healthy tissues. There are many ways to evaluate a radiation treatment plan using conventional dose indexes in this study such as CI and HI. However, the radiobiological parameters such as the Niemierko’s EUD-based tumour control probability and normal tissue complication probability have not yet applied in this research.
The QA is very important to consider the feasibility of the application of this technique before treatment.Reference Tai, Oanh, Son and Loan25 Our measurements showed that the dose distributions planned using IMRT TPS are accurate and suitable for treatment delivery. It is interesting to note that all 20 plans of this study passed the requirements based on our established pass criteria (γ-index criterion of 3%/3 mm and pass rates of 96·65 ± 0·86%).
Conclusion
This study showed that the PTV coverage, homogenous dose distribution within the PTV, the capability of controlling the hot spots and normal tissue sparing were better for the IMRT plans compared to FIF in head-and-neck cancer. The average MUs for the IMRT plans are also larger than FIF plans.
Acknowledgements
The authors would like to thank Professor James C. L. Chow, University of Toronto, Ontario, Canada, for his contributions. The authors also would like to extend thanks to Chief of Radiation Oncology Center, BaiChay Hospital.
Financial Disclosure
None declared.
Confict of Interest
The authors declare no conflict of interest.