Introduction
Ovarian follicular development involves a complex series of coordinated events, including primordial follicle activation, growth of primary and secondary follicles, formation of antrum cavity, oocyte maturation, steroidogenesis and ovulation (Van Den Hurk and Zhao, Reference Van Den Hurk and Zhao2005; Silva et al., Reference Silva, Figueiredo and Van Den Hurk2009, Reference Silva, Van Den Hurk and Figueiredo2016; Figueiredo et al., Reference Figueiredo, Lima, Silva and Santos2018). During these growth and differentiation processes, paracrine factors mediate the communication among oocyte, granulosa and theca cells (Hsueh et al., Reference Hsueh, Kawamura, Cheng and Fauser2015). Cytokines, such as tumour necrosis factor-α (TNF-α) and interleukin-1β (IL-1β) are involved in controlling follicular development and ovulation. Their functions include regulation of cellular proliferation/differentiation, follicular survival/atresia, and oocyte maturation (Field et al., Reference Field, Dasgupta, Cummings and Orsi2014).
TNF-α is a cytokine that was originally recognized for its action in inflammatory processes (Terranova and Rice, Reference Terranova and Rice1997). This factor acts by binding to one of its two receptors, namely a type I receptor (TNFR1), which is approximately 60 kDa and a type II receptor (TNFR2). Moreover, TNF-α is involved in the regulation of physiological processes such as follicular development, steroidogenesis, ovulation and corpus luteum function (Sakumoto and Okuda, Reference Sakumoto and Okuda2004; Glister et al., Reference Glister, Hatzirodos, Hummitzsch, Knight and Rodgers2014; Samir et al., Reference Samir, Glister, Mattar, Laird and Knight2017). It has also been reported that, depending on the stage of development, TNF-α may regulate differentiation of granulosa cells (Glister et al., Reference Glister, Hatzirodos, Hummitzsch, Knight and Rodgers2014) and apoptosis (Manabe et al., Reference Manabe, Matsuda-Minehata, Goto, Maeda, Cheng, Nakagawa, Inoue, Wongpanit, Jin, Gonda and Li2008).
IL-1β is another important pro-inflammatory cytokine that can stimulate T-cell proliferation and increase antibody production (Kool et al., Reference Kool, Fierens and Lambrecht2012). IL-1β synthesis occurs primarily in macrophages and monocytes when stimulated, but may be synthesized to a lesser extent in other cell types such as lymphocytes, neutrophils, fibroblasts, and endothelial cells (Gabay et al., Reference Gabay, Lamacchia and Palmer2010). Passos et al. (Reference Passos, Costa, Cunha, Silva, Ribeiro, Souza, Barroso, Dau, Saraiva, Gonçalves, Hurk and Silva2016) also reported that IL-1β and its receptors are expressed in bovine ovarian follicles at various stages of development. The IL-1 system has two receptor types, i.e. a type I receptor (IL-1RI) with a 213 amino acid domain in the intracytoplasmic region and a type II receptor (IL-1RII) with only a domain of 29 residues (Adashi, Reference Adashi1998). IL-1RII and IL-1R antagonist (IL-1Ra) are natural inhibitors that prevent excessive inflammatory responses caused by the IL-1 system (Gabay et al., Reference Gabay, Lamacchia and Palmer2010). It has been demonstrated that IL-1β acts in the control of follicle development by facilitating granulosa cells proliferation and preventing premature differentiation (Brännström, Reference Brännström, Leung and Adash2004). This factor also influences apoptosis in ovarian granulosa cells (Chun et al., Reference Chun, Eisenhauer, Kubo and Hsueh1995) and appears to be involved in a number of ovulation-associated events such as protease synthesis, regulation of plasminogen activator activity, and prostaglandin production (Brännström, Reference Brännström, Leung and Adash2004). Considering that TNF-α and IL-1β are produced locally in the ovary and, once secreted, diffuse to act in a paracrine/autocrine manner to regulate ovarian function, it is very important to discuss recent findings about these cytokines to better understand their role in ovarian folliculogenesis in domestic animals.
The review aims to show the expression sites of TNF-α and IL-1β system members in the ovary and to discuss their roles and mechanisms of action during follicle development, oocyte maturation and ovulation in domestic animal species.
Expression of TNF-α systems in ovarian follicles and its mechanism of action
The non-inflammatory effects to which TNF-α is related include control of follicular development (Samir et al., Reference Samir, Glister, Mattar, Laird and Knight2017), ovulation, and, depending on its mechanism of action, it may further regulate differentiation (Glister et al., Reference Glister, Hatzirodos, Hummitzsch, Knight and Rodgers2014) or apoptosis (Manabe et al., Reference Manabe, Matsuda-Minehata, Goto, Maeda, Cheng, Nakagawa, Inoue, Wongpanit, Jin, Gonda and Li2008). In bovine ovaries, Silva et al. (Reference Nakayama2017b) showed that TNF-α system proteins (TNF-α/TNFR1/TNFR2) are expressed in various follicular compartments. These authors reported the presence of TNF-α and TNFR1 in primary follicle oocytes, as well as in oocyte, granulosa and theca cells of secondary and antral follicles. TNFR2 proteins are expressed in oocytes of primordial, primary and secondary follicles, as well as in granulosa and theca cells of secondary and antral follicles. TNF-α receptor expression was demonstrated in granulosa cells from small (1–5 mm) and large (> 8 mm) bovine antral follicles (Spicer, Reference Spicer2001). In addition, mRNA and/or protein for TNF-α are also localized in different follicular compartments of sheep preovulatory follicles (Johnson et al., Reference Johnson, Murdoch, Van Kirk, Kaltenbach and Murdoch1999). Human oocytes and cumulus cells from large antral follicles express both mRNA and protein for TNF-α and its TNFR2 receptor (Naz et al., Reference Naz, Zhu and Menge1997). In pig antral follicles, intense signals for TNF-α and its mRNAs were demonstrated in granulosa cells (Nakayama, Reference Nakayama2003). In rat ovaries, TNF-α localization sites include oocytes (Marcinkiewicz et al., Reference Marcinkiewicz, Krishna, Cheung and Terranova1994), granulosa cells (Roby and Terranova, Reference Roby, Terranova and Hirshfield1989), corpora lutea and macrophages (Sancho-Tello et al., Reference Sancho-Tello, Tash, Roby and Terranova1993). In rabbits, TNF-α production and accumulation were reported in the corpus luteum of pseudopregnant and pregnant animals. These data highlight an autocrine and paracrine effect of TNF-α during follicular development. Figure 1 summarizes the sites of expression of TNF-α system in ovarian follicles.

Figure 1. Expression of proteins of IL-1β, TNF-α and their receptors in bovine ovarian follicles.
TNF-α acts by binding to one of its two receptors, i.e. TNFR1 or TNFR2. These receptors are transmembrane proteins with cytoplasmic domains that initiate signal transduction after TNF-α binding (Fig. 2). After activation of TNFR1, signalling begins with the recruitment of various adapter proteins to form complex I, consisting of TNFR1-associated DEATH domain protein (TRADD), TNF-associated factor 2 (TRAF2), and the interacting receptor with protein 1 (RIP1). Then, complex II is formed by association of complex I plus Fas-associated death domain (FADD) and pro-caspase 8/10, which are responsible for apoptosis (Micheau and Tschopp, Reference Micheau and Tschopp2003; Wullaert et al., Reference Wullaert, van Loo, Heyninck and Beyaert2007). However, the TRADD protein that interacts with the intracellular domain of TNFR1 can be suppressed by the crmA gene (Hsu et al., Reference Hsu, Xiong and Goeddel1995). In addition, TNFR1 receptor may also activate transcription factor nuclear factor-kappaB (NF-κB) and promote cell survival by permanence of the caspase-8 inhibitor (FLIP) (Micheau and Tschopp, Reference Micheau and Tschopp2003). The p65 (RelA), RelB, c-Rel, p50/p105 and p52/p100 members of the NF-κB family appear in cells as homodimers or heterodimers linked to IκB proteins (Hayden and Ghosh, Reference Hayden and Ghosh2004). NF-κB factor, when not stimulated, is bound to IκB (one of the NF-κB inhibitory proteins), as phosphorylation and degradation of IκB are required to promote translocation of NF-κB (p65 and p50) to the nucleus (Baeuerle and Baltimore, Reference Baeuerle and Baltimore1996). After IκB kinase (IKK) stimulation, IKKα and IKKβ promote the classical pathway of NF-κB translocation to the nucleus (Rauert et al., Reference Rauert, Wicovsky, Muller, Siegmund, Spindler, Waschke, Kneitz and Wajant2010). The classical pathway can be activated by both TNFR1 and TNFR2. The NF-κB modifier (NEMO) signals activate the IκB kinase complex beta subunit (IKKβ), which then phosphorylates the IκB protein into two N-terminal serine residues. This complex is recognized by ubiquitin ligase, leading to its activation. polyubiquitination and subsequent degradation by proteasomes (Fig. 2) (Hayden and Ghosh, Reference Hayden and Ghosh2004).
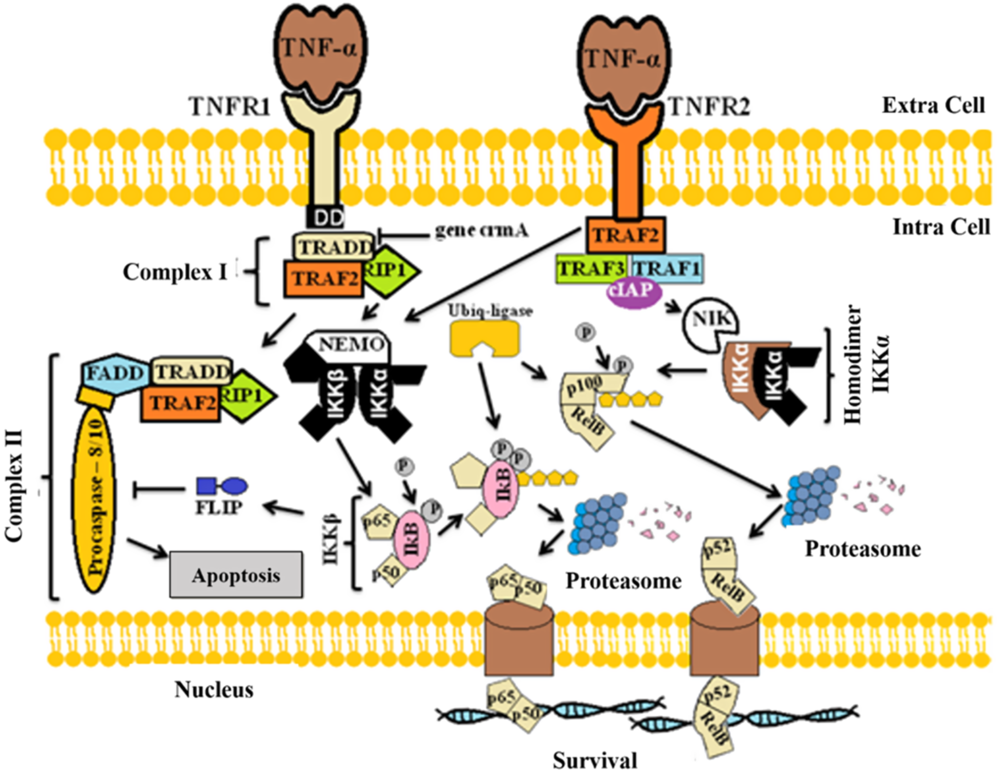
Figure 2. Mechanisms of action of TNF-α to promote cell signal transduction.
The alternative NF-κB pathway through phosphorylation of the IKKα complex (composed of p100 and RelB) is independent of NEMO stimulation (Sun and Ley, Reference Sun and Ley2008). First, TNFR2 signals through TRAF2 that binds to TNF-associated factor 1 (TRAF1), TNF-associated factor 3 (TRAF3) and apoptosis inhibitor protein (cIAP) (ROTHE et al., Reference Rothe, Sarma, Dixit and Goeddel1995). This complex activates the inducing kinase NF-κB (NIK), responsible for phosphorylation and activation of the IKKα homodimer (Senftleben et al., Reference Senftleben, Cao, Xiao, Greten, Krahn, Bonizzi, Chen, Hu, Fong, Sun and Karin2001). Then, two IKKα C-terminals are phosphorylated for proteosomal degradation, generating p52 that is translocated to the nucleus (Fig. 2) (Rauert et al., Reference Rauert, Wicovsky, Muller, Siegmund, Spindler, Waschke, Kneitz and Wajant2010). Studies suggest that the classical and alternative pathways of NF-κB have distinct regulatory functions (Bonizzi and Karin, Reference Bonizzi and Karin2004).
Expression of IL system in ovarian follicles and its mechanisms of action
In bovine species, Passos et al. (Reference Passos, Costa, Cunha, Silva, Ribeiro, Souza, Barroso, Dau, Saraiva, Gonçalves, Hurk and Silva2016) showed that IL-1β protein is expressed in oocytes and granulosa cells of primordial follicles, as well as in oocyte, granulosa and theca cells from primary, secondary and antral follicles. These authors showed that the protein for IL-1RA is found in granulosa cells of primary follicles and in oocyte and granulosa cells of secondary and antral follicles. Oocytes and granulosa cells from primordial and primary follicles express IL-1RI, while this protein is found in oocyte, granulosa and theca cells from secondary and small antral follicles. For IL-1RII, the protein is observed in oocytes and granulosa cells of all follicular categories. Figure 1 summarizes the sites of expression of IL-1β system in ovarian follicles.
IL-1β was previously demonstrated in oocytes, granulosa, theca and cumulus cells in ovaries of human (Zolti et al., Reference Zolti, Ben-Rafael, Meirom, Shemesh, Bider, Mashiach and Apte1991; Barak et al., Reference Barak, Yanai, Trevers, Roisman, Simón and Laufer1992; De Los Santos et al., Reference De Los Santos, Anderson, Racowsky, Simón and Hill1998; Carlberg et al., Reference Carlberg, Nejaty, Fröysa, Guan, Söder and Bergqvist2000), mouse (Simón et al., Reference Simón, Frances, Piquette and Polan1994; Terranova and Rice, Reference Terranova and Rice1997), rat (Brännström et al., Reference Brännström, Pascoe, Norman and McClure1994) and mare (Martoriati et al., Reference Martoriati, Lalmanach, Goudet and Gérard2002). IL-1α protein was previously detected in mouse (Simón et al., Reference Simón, Frances, Piquette and Polan1994; Terranova and Rice, Reference Terranova and Rice1997) and human (De Los Santos et al., Reference De Los Santos, Anderson, Racowsky, Simón and Hill1998) oocytes, theca and cumulus cells (Kol et al., Reference Kol, Donesky, Ruutiainen-Altman, Ben-Shlomo, Irahara, Ando, Rohan and Adashi1999), showing that ovarian cells synthesize IL-1α. Moreover, IL-1β was found to be produced by ovarian granulosa cells of preovulatory follicles (Salamonsen et al., Reference Salamonsen, Hannan and Dimitriadis2007; Trundley and Moffett, Reference Trundley and Moffett2004). The presence of IL-1RA was demonstrated in granulosa and cumulus cells of mares (Martoriati et al., Reference Martoriati, Lalmanach, Goudet and Gérard2002) and human (De Los Santos et al., Reference De Los Santos, Anderson, Racowsky, Simón and Hill1998). The presence of IL-1RI protein was previously demonstrated in oocytes, granulosa, theca and cumulus cells in ovarian tissues of mouse (Simón et al., Reference Simón, Frances, Piquette and Polan1994), rat (Kol et al., Reference Kol, Donesky, Ruutiainen-Altman, Ben-Shlomo, Irahara, Ando, Rohan and Adashi1999; Wang et al., Reference Wang, Brännström, Cui, Simula, Hart, Maddocks and Norman1997), human (De Los Santos et al., Reference De Los Santos, Anderson, Racowsky, Simón and Hill1998; Wang et al., Reference Wang, Brännström, Cui, Simula, Hart, Maddocks and Norman1997) and mares (Martoriati et al., Reference Martoriati, Lalmanach, Goudet and Gérard2002). In mouse, the expression of IL-1RI varies with follicular development (Terranova and Rice, Reference Terranova and Rice1997). In human, it has been demonstrated that mRNA for IL-1RI is absent in primordial follicles, but present in granulosa and theca cells from growing secondary and antral follicles (Wang et al., Reference Wang, Brännström, Cui, Simula, Hart, Maddocks and Norman1997).
In bovine follicles, Passos et al. (Reference Passos, Costa, Cunha, Silva, Ribeiro, Souza, Barroso, Dau, Saraiva, Gonçalves, Hurk and Silva2016) reported that the growth from secondary to small antral follicles is followed by an increase in the levels of mRNA for IL-1α, IL-1β and IL-1RA (Fig. 1). In equine species, Martoriati et al. (Reference Martoriati, Lalmanach, Goudet and Gérard2002) reported the presence of IL-1β and IL-1RI transcripts in oocytes of antral follicles, while transcripts for IL-1β, IL-1RA, IL-1RI and IL-1RII were found in cumulus cells. Other studies have shown that the members of the IL-1 system are located in various types of ovarian cells, i.e. in oocytes, granulosa and theca cells of several mammalian species (Sirotkin, Reference Sirotkin2011). The transcript for IL-1β is located in mural granulosa cells, the most inner layers of the theca, and in the oocytes of rat preantral and early antral follicles (Kol et al., Reference Kol, Donesky, Ruutiainen-Altman, Ben-Shlomo, Irahara, Ando, Rohan and Adashi1999). IL-1RI mRNA is also expressed in murine oocytes (Deyerle et al., Reference Deyerle, Sims, Dower and Bothwell1992) and abundant levels of IL-1RA mRNA are found in the granulosa of rat primordial and growing follicles (Wang et al., Reference Wang, Brännström, Cui, Simula, Hart, Maddocks and Norman1997). Within antral follicles, Passos et al. (Reference Passos, Costa, Cunha, Silva, Ribeiro, Souza, Barroso, Dau, Saraiva, Gonçalves, Hurk and Silva2016) showed that the follicular wall is the main site of production of mRNA for IL-1α, IL-1β, IL-1RA and IL-1RI. With the growth from small to large antral follicles, a reduction in expression of mRNA for IL-1α is observed but, in general, large follicles had higher expression of IL-1β, IL-1RA and IL-1RII. Other studies have shown that mare cumulus–oocyte complexes (COCs) contain IL-1β mRNA (Martoriati et al., Reference Martoriati, Lalmanach, Goudet and Gérard2002), and that the protein for IL-1β is secreted by cultured human granulosa cells (Carlberg et al., Reference Carlberg, Nejaty, Fröysa, Guan, Söder and Bergqvist2000). Production of IL and their receptors is also observed in both granulosa and theca cells, while maximal production occurred in preovulatory follicles after gonadotropin action (Ingman and Jones, Reference Ingman and Jones2008).
Regarding the IL mechanism of action (Fig. 3), there are inhibitors, such as IL-1 receptor antagonist (IL-1Ra) and type II receptor (IL-1RII) that regulates the responses to IL-1β (Dinarello, Reference Dinarello2010). In humans, IL-1β binds to two receptor types, a type I (IL-1RI) and a type II receptor (IL-1RII) (Adashi, Reference Adashi1998). IL-1RII and IL-1R antagonist (IL-1Ra) are natural inhibitors that prevent excessive inflammatory responses caused by the IL-1 system (Gabay et al., Reference Gabay, Lamacchia and Palmer2010). Additionally, it was shown that IL-1RA blocks IL-1β mechanisms (Martoriati et al., Reference Martoriati, Caillaud, Goudet and Gérard2003a). The IL-1β receptor is a complex formed by the type I receptor and an accessory receptor protein (IL-1RAcP) that form an IL-1 heterodimer (Boraschi and Tagliabue, Reference Boraschi and Tagliabue2013). Myeloid differentiation protein (MyD88) is an molecule that acts as a transient adapter or regulator in the IL-1R signalling complex (Muzio et al., Reference Muzio, Ni, Feng and Dixit1997) and is capable of recruiting interleukin receptor-associated kinase type 1 (IRAK1) and type 2 (IRAK2) (Wesche et al., Reference Wesche, Henzel, Shillinglaw, Li and Cao1997). In addition, receptor-associated factor 6 (TRAF6) is recruited by IL-1R and requires MyD88 to activate NF-κB (Wu and Arron, Reference Wu and Arron2003). The classical NF-κB pathway occurs with the phosphorylation of two serine residues (ser32 and ser36) of the IκB protein by IκB kinase activity (IKK), the polyubiquitination of IKKβ and then degradation by the 26S proteosome, releasing NF-κB dimers (p65 and p50) (Nishikori, Reference Nishikori2005; Karin and Ben-Neriah, Reference Karin and Ben-Neriah2000). IKKβ-dependent activation of NF-κB plays an important role in the transcriptional control of acute and chronic inflammation (Bonizzi and Karin, Reference Bonizzi and Karin2004). However, studies show that IL-1-induced, but not TNF-α induced, NEMO and IKKα are sufficient for NF-κB activation without requiring the IKKβ dimer (Solt et al., Reference Solt, Madge, Orange and May2007). Therefore, IL-1 can induce both the classical pathway through IKKβ and IKKα through NEMO (Solt et al., Reference Solt, Madge, Orange and May2007; Bonizzi and Karin, Reference Bonizzi and Karin2004; Nishikori, Reference Nishikori2005). Following activation by the IKKα complex, there is the transfer of p52 and RelB to the nucleus (Rauert et al., Reference Rauert, Wicovsky, Muller, Siegmund, Spindler, Waschke, Kneitz and Wajant2010) and the IKKβ complex translocation of p65 and p50 (Baeuerle and Baltimore, Reference Baeuerle and Baltimore1996).

Figure 3. Mechanisms of action of IL-1β to promote cell signal transduction. (a) IL-1β system heterodimer complex capable of promoting signal transduction (classical pathway by NEMO stimulation). (b) Natural IL-1β antagonist (IL-1Ra) promoting blockade of signal transduction. (c) Type II receptor (IL-1RII) inhibiting signal transduction by the small peptide chain that does not cross the membrane completely.
Effects of TNF-α during folliculogenesis, oocyte maturation and ovulation
Silva et al. (Reference Nakayama2017b) showed that that TNF-α increases the number of apoptotic cells in cultured bovine ovarian tissue and reduces primordial follicle survival. Other studies reported that TNF-α induces oocyte, granulosa and luteal cell death (Chen et al., Reference Chen, Marcinkiewicz, Sancho-Tello, Hunt and Terranova1993; Kaipia et al., Reference Kaipia, Chun, Eisenhauer and Hsueh1996; Sasson et al., Reference Sasson, Winder, Kees and Amsterdam2002; Abdo et al., Reference Abdo, Hisheh and Dharmarajan2003), as well as apoptosis in granulosa cells of rat preovulatory follicles (Sasson et al., Reference Sasson, Winder, Kees and Amsterdam2002). In mice, Nilsson et al. (Reference Nilsson, Stanfield and Skinner2006) demonstrated that TNF-α can interact with P4 to regulate the growth of primordial follicles. In bovine secondary follicles in vitro, Paulino et al. (Reference Paulino, Cunha, Silva, Souza, Lopes, Donato, Peixoto, Matos-Brito, Van Den Hurk and Silva2018) showed that TNF-α promotes growth, antrum formation and maintains the ultrastructure of secondary follicles in vitro. These studies show that the effect of TNF-α on ovarian cells is dependent on the stage of follicle development, which can be associated with different levels of TNF-α receptor expression (Witty et al., Reference Witty, Bridgham and Johnson1996). The ability of TNF-α to promote the increase in follicular diameter could be because these follicles have receptors (TNFR1 and TNFR2) for TNF-α in their oocyte and granulosa cells (Silva et al., Reference Nakayama2017b). Exogenous TNF-α added into culture medium can bind to the TNFR2 and promote follicular growth, instead of apoptosis. As reported by Wajant et al. (Reference Wajant, Pfizenmaier and Scheurich2003), TNFR2 can induce gene transcription associated with cell survival, growth and differentiation. Fischer et al. (Reference Fischer, Maier, Siegemund, Wajant, Scheurich and Pfizenmaier2011) showed that protection from cell death is dependent on TNFR2 activation of the PI3K–PKB/Akt pathway. TNF-α has also been shown to activate NF-κB, which in turn regulates the expression of proteins associated with cell survival and cell proliferation (Aggarwal et al., Reference Aggarwal, Takada, Mhashilkar, Sieger, Chada and Aggarwal2004).
TNF-α can also modulate steroidogenesis by granulosa, thecal and luteal cells (Chun and Hsueh, Reference Chun and Hsueh1998), as some studies reported that TNF-α inhibits secretion of estradiol and P4 in murine, porcine, bovine and human granulosa cells in vitro (Spicer, Reference Spicer1998; Veldhuis et al., Reference Veldhuis, Garmey, Urban, Demers and Aggarwal1991; Rice et al., Reference Rice, Williams, Limback and Terranova1996). TNF-α also exerts an inhibitory effect on luteinization of pig granulosa cells and influences the balance between follicular growth (proliferation) and atresia (apoptosis) (Prange-Kiel et al., Reference Prange-Kiel, Kreutzkamm, Wehrenberg and Rune2001).
Regarding the effects of TNF-α on oocyte maturation, Ma et al. (Reference Ma, Yan, Qiao, Sha, Li, Chen and Sun2010) showed that exposure of porcine oocytes to TNF-α causes a reduction in oocyte maturation and abnormalities in chromosomal alignment. Conversely, in bovine species, this factor promotes in vitro oocyte growth during 48 h of culture of COCs from antral follicles and influenced the distribution of mitochondria in pre-matured oocytes (Lima et al., Reference Lima, Bezerra, Souza, Matos, Van Den Hurk and Silva2018). TNF-α also reduces CASP3 and CASP6 mRNA levels in bovine cumulus cells after 12 h of culture (Silva et al., Reference Muzio, Ni, Feng and Dixit2017a). This fact suggests that, in bovine oocyte and cumulus cells, TNF-α is mainly acting by binding to the TNFR2, thereby reducing the apoptotic process. Silva et al., (Reference Muzio, Ni, Feng and Dixit2017a) also showed that TNF-α reduces HAS-2 mRNA levels in cumulus cells after culturing bovine COCs for 12 h, but this reduction in expression did not interfere with cumulus expansion at the end of maturation. Recently, Kong et al. (Reference Kong, Wang, Xiao, Lin, Zhu, Sun, Luo and Tan2018) showed that ageing mouse cumulus cells secrete TNF-α, which accelerates oocyte ageing by interacting with TNFR.
TNF-α is a mediator of ovulation in terms of oocyte release and death induction via apoptosis and autophagy of granulosa cells in ovarian tissue remodelling (Yamamoto et al., Reference Yamamoto, Kuwahara, Taniguchi, Yamasaki, Tanaka, Mukai, Yamashita, Matsuzaki, Yasui and Irahara2015). Crespo et al. (Reference Crespo, Mañanós, Roher, Mackenzie and Planas2012) showed that luteinizing hormone (LH) induces ovulation via TNF-α-dependent increases in prostaglandin F2α. TNF-α is secreted by mammalian preovulatory follicles (Brännström et al., Reference Brännström, Pascoe, Norman and McClure1994) and, in rats, its levels are increased by in vivo administration of hCG (Rice et al., Reference Rice, Williams, Limback and Terranova1996). TNF-α enhances ovulation rates in rat ovary (Brännström et al., Reference Brännström, Pascoe, Norman and McClure1994) and stimulates apoptosis (Murdoch et al., Reference Murdoch, Colgin and Ellis1997) and collagenolytic activity in preovulatory follicles (Johnson et al., Reference Johnson, Murdoch, Van Kirk, Kaltenbach and Murdoch1999).
Effects of IL-1 system members during folliculogenesis, oocyte maturation and ovulation
Regarding folliculogenesis, Passos et al. (Reference Passos, Costa, Cunha, Silva, Ribeiro, Souza, Barroso, Dau, Saraiva, Gonçalves, Hurk and Silva2016) demonstrated that IL-1β promotes the development and activation of primordial bovine follicles and contributes to maintain early follicle survival. These findings suggest that IL-1β is a survival factor for bovine ovarian follicles, its action being partially mediated via NO and cGMP generation (Chun et al., Reference Chun, Eisenhauer, Kubo and Hsueh1995). Previous studies have revealed that IL-1β controls the transition of follicular cells by facilitating their proliferation and differentiation (Brännström, Reference Brännström, Leung and Adash2004). For bovine secondary follicles, Paulino et al. (Reference Paulino, Cunha, Silva, Souza, Lopes, Donato, Peixoto, Matos-Brito, Van Den Hurk and Silva2018) showed that IL-1β does not influence their development in vitro. Probably, IL-1β acts mainly in large antral follicles. IL-1β is capable of promoting proliferation of bovine granulosa cells (Basini et al., Reference Basini, Baratta, Bussolati and Tamanini1998) and suppressing apoptosis of rat ovarian follicles (Chun et al., Reference Chun, Eisenhauer, Kubo and Hsueh1995), acting as a survival factor. Studies in murine (Karakji and Tsang, Reference Karakji and Tsang1995) and bovine (Baratta et al., Reference Baratta, Basini, Bussolati and Tamanini1996) species showed that the effects of IL-1 on granulosa cell proliferation in vitro are dependent on follicle size. IL-1β is also able to modulate steroidogenesis and promote the proliferation of granulosa cells in porcine (Fukuoka et al., Reference Fukuoka, Yasuda, Taii, Takakura and Mori1989) and human (Best and Hill, Reference Best and Hill1995) species. IL-1β promotes an increase in the production of cAMP, oestrogen, and progesterone in mice granulosa cells that are essential for growth and follicular development (Chun et al., Reference Chun, Eisenhauer, Kubo and Hsueh1995). In contrast, Uri-Belapolsky et al. (Reference Uri-Belapolsky, Shaish, Eliyahu, Grossman, Levi, Chuderland, Ninio-Many, Hasky, Shashar, Almog, Kandel-Kfi, Harats, Shalgi and Kamari2014) reported that IL-1β promotes apoptotic pathways and causes age-related exhaustion of ovarian reserves in mice.
In large antral follicles, intra-follicular injection of IL-1β in dominant follicles induces oocyte maturation in vivo (Martoriati et al., Reference Martoriati, Duchamp and Gérard2003b). Chaubey et al. (Reference Chaubey, Kumar, Kumar, Sarwalia, Kumaresan, De, Kumar and Datta2018) showed that IL-1β improves cumulus expansion and developmental ability of poor quality buffalo oocytes. In bovine species, IL-1β also improved the percentage of oocytes developing to the blastocyst stage. Recently, Javvaji et al. (Reference Javvaji, Dhali, Francis, Kolte, Mech, Sathish and Roy2019) showed that IL-7 at low concentrations is beneficial for oocyte maturation, through the favourable level of intracellular reactive oxygen species and antioxidant mechanisms. Other studies have indicated that IL-7 promotes survival and multiplication of granulosa cells, germinal vesicle breakdown and quality and nuclear maturation of oocytes in murine species (Cakmak et al., Reference Cakmak, Franciosi, Zamah, Cedars and Conti2016; Franciosi et al., Reference Franciosi, Manandhar and Conti2016; Cheng et al., Reference Cheng, Yata, Klein, Cho, Deguchi and Hsueh2011). Caillaud et al. (Reference Caillaud, Duchamp and Gérard2005) showed that IL-1β alone is not able to promote cytoplasmic maturation of equine oocyte, but it may play an essential role in the physiology of equine oocytes by acting on meiosis resumption. Additionally, there is an important interdependence between IL-1α and follicle-stimulating hormone receptor (FSHR), as IL-1 receptor inactivation increases FSHR expression in rat granulosa cells (Uri-Belapolsky et al., Reference Uri-Belapolsky, Miller, Shaish, Levi, Harats, Ninio-Many, Kamari and Shalgi2017). IL-1β reduces the expression of LH receptors in rat granulosa cells in vitro (Gottschall et al., Reference Gottschall, Katsuura, Hoffmann and Arimura1988) and promotes the switch in granulosa cell proliferation to differentiation (Karakji and Tsang, Reference Karakji and Tsang1995), indicating that this cytokine participates in determining whether follicles undergo atresia or progress to ovulation. IL-1β can also upregulate granulosa cell and intraovarian macrophage nitric oxide (NO) production, thereby influencing cellular growth or apoptosis (Matsumi et al., Reference Matsumi, Yano, Osuga, Kugu, Tang, Xu, Yano, Kurashima, Ogura, Tsutsumi, Koji, Esumi and Taketani2000).
Both IL-1β and TNF-α are known to induce ovulation in rats and rabbits (Machelon and Emilie, Reference Machelon and Emilie1997). The acceleration of ovulation has been reported to occur by neutrophil infiltration into the theca cells layer, which induces IL-1β synthesis and promotes synergy to stimulate ovulation (Tanaka et al., Reference Tanaka, Kuwahara, Ushigoe, Yano, Taniguchi, Yamamoto, Matsuzaki, Yasui and Irahara2017). In mice and humans, IL-1β mRNA levels increase in granulosa cells as the follicle nears rupture (Machelon et al., Reference Machelon, Nome, Durand-Gasselin and Emilie1995; Adashi, Reference Adashi1998), emphasizing the role of IL-1β in ovulation. Furthermore, IL-1β modulates total prostaglandin E (PGE) production by granulosa cells, therefore providing a mechanism to determine the site of follicle rupture (Hurwitz et al., Reference Hurwitz, Ricciarelli, Botero, Rohan, Hernandez and Adashi1991, Reference Hurwitz, Loukides, Ricciarelli, Botero, Katz, Mcallister, Garcia, Rohan, Adashi and Hernandez1992, Reference Hurwitz, Finci-Yeheskel, Dushnik, Milwidsky, Shimonovitz, Yagel, Adashi and Mayer1995; Duffy et al., Reference Duffy, Ko, Jo, Brannstrom and Curry2019).
Final considerations
TNF-α and IL-1β systems are expressed in ovarian follicles of different species and influence follicular development, oocyte maturation and ovulation. TNF-α is related to cell growth and differentiation and apoptosis, depending on which receptors it binds. IL-1β is related to granulosa cell proliferation, acting on the transition of follicular cells and facilitating the process of differentiation and proliferation. Both TNF-α and IL-1β are also involved in the nuclear and/or cytoplasmic oocyte maturation process, as well as in mechanisms that determine follicle ovulation.
Financial Support
This research received no specific grant from any funding agency, commercial or not-for-profit sectors.
Conflicts of Interest
The authors declare no conflicts of interest.
Ethical Standards
Not applicable.