1. Introduction
Throughout most of Cambrian and Ordovician times, Baltica was an isolated continent with distinctive and largely endemic faunas different from those of contemporary palaeoplates elsewhere. This substantial terrane encompasses much of northern Europe and is bounded by the Ural Mountains in the east, the Caledonides in the northwest and the Trans-European Suture Zone in the southwest (e.g. Cocks & Fortey, Reference Cocks and Fortey1998; Torsvik & Cocks, Reference Torsvik and Cocks2005, Reference Torsvik, Cocks, Harper and Servais2013). Palaeomagnetic data indicate that Baltica was geographically inverted relative to its present configuration and lay at temperate to subtropical latitudes (35–65° south of the palaeoequator) during Cambrian times (Torsvik & Rehnström, Reference Torsvik and Rehnström2001; Torsvik & Cocks, Reference Torsvik and Cocks2005, Reference Torsvik and Cocks2017; Cocks & Torsvik, Reference Cocks and Torsvik2005; Álvaro et al. Reference Álvaro, Ahlberg, Babcock, Bordonaro, Choi, Cooper, Ergaliev, Gapp, Ghobadi Pour, Hughes, Jago, Korovnikov, Laurie, Lieberman, Paterson, Pegel, Popov, Rushton, Sukhov, Tortello, Zhou, Żylińska, Harper and Servais2013). Much of the craton was submerged under a shallow to moderately deep epeiric sea for long periods of Early Palaeozoic time (e.g. Cocks & Torsvik, Reference Cocks and Torsvik2005; Calner et al. Reference Calner, Ahlberg, Lehnert and Erlström2013; Torsvik & Cocks, Reference Torsvik and Cocks2017). Extensive weathering and erosion during late Neoproterozoic time resulted in a low topography, and hence sediment starvation, for most of Cambrian and Early–Middle Ordovician times. The Cambrian through Middle Ordovician sedimentary cover of Baltoscandia (sensu Martinsson, Reference Martinsson and Holland1974) is therefore condensed and relatively thin, commonly less than 300 m in total.
Outcrop areas with lower Palaeozoic sedimentary successions are widely distributed in Baltoscandia, from Finnmark in northernmost Norway to the island of Bornholm, Denmark, in the south (Fig. 1a; Nielsen & Schovsbo, Reference Nielsen and Schovsbo2007, fig. 1, Reference Nielsen and Schovsbo2011, fig. 1, Reference Nielsen and Schovsbo2015, fig. 1). Most outcrops occur within the East European (or Russian) Platform or in scattered outliers in Norway, on the mainland of Sweden, and on Bornholm. Numerous outcrops are also present in a relatively narrow belt with (par-)autochthonous and allochthonous rocks along the Caledonian thrust front. The preserved deposits are erosional remnants of what originally was a broad sedimentary blanket that covered most of Baltoscandia.
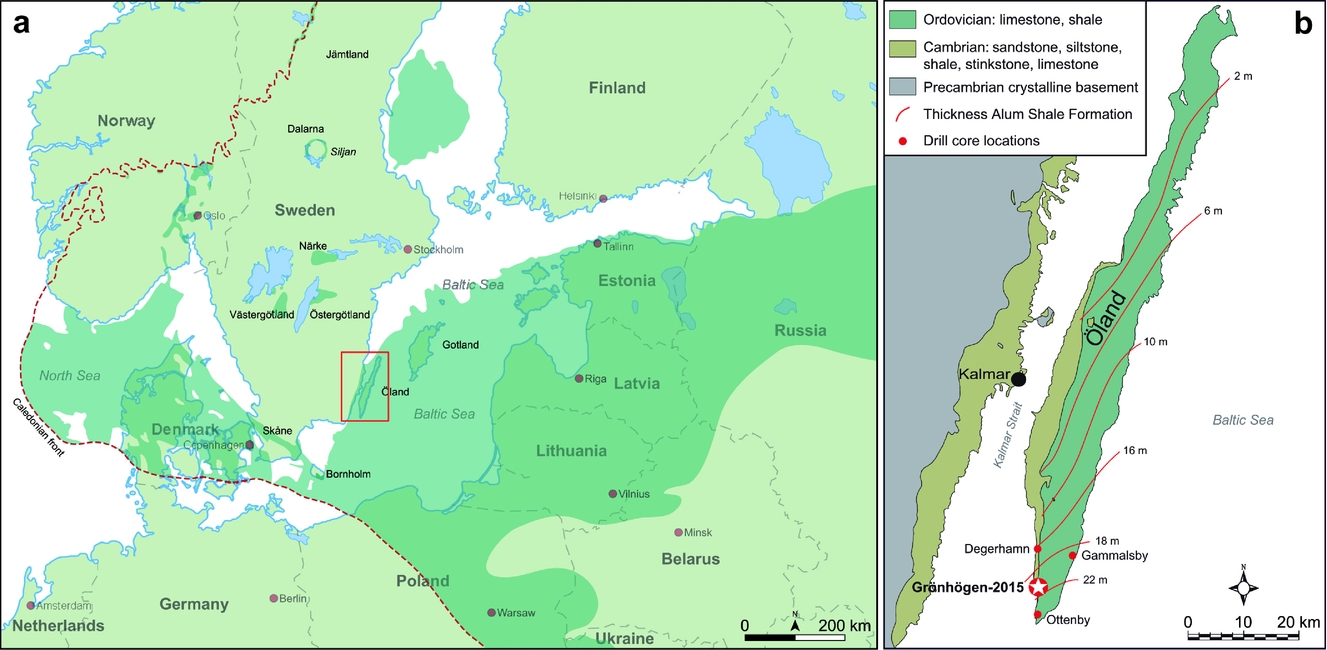
Figure 1. (a) Map of southern Sweden and the surrounding Baltoscandian region showing the distribution of lower Palaeozoic rocks (green shading). Modified from Lindskog & Eriksson (Reference Lindskog and Eriksson2017). (b) Simplified geological map of Öland, southern Sweden, showing the location of the Grönhögen-2015 drill site and the location of other core drillings referred to in the text. Unbroken lines represent isopachytes for the Cambrian Series 3 through Lower Ordovician (Tremadocian) Alum Shale Formation. Modified from Erlström (Reference Erlström2016, fig. 1).
The Cambrian through lowermost Ordovician (Tremadocian) succession of Scandinavia can be broadly divided into two divisions: a lower division dominated by Terreneuvian and Cambrian Series 2 coarse-grained siliciclastic rocks, generally resting on Precambrian crystalline rocks, and an upper division consisting predominantly of Cambrian Series 3 through lower Tremadocian mudstones and shales with subordinate limestone beds and lenses. The boundary between these broad divisions is marked by a prominent unconformity ascribed to non-deposition and erosion during a eustatic sea-level fall that at least partially correlates with the regressive ‘Hawke Bay Event’ (Bergström & Ahlberg, Reference Bergström and Ahlberg1981; Nielsen & Schovsbo, Reference Nielsen and Schovsbo2007, Reference Nielsen and Schovsbo2011, Reference Nielsen and Schovsbo2015). In western Baltica (Scandinavia) this sea-level fall coincided with epeirogenic uplift (Nielsen & Schovsbo, Reference Nielsen and Schovsbo2007, Reference Nielsen and Schovsbo2015). The upper division comprises the silt- and mudstone-dominated Borgholm Formation (Cambrian Series 3) followed by dark grey to black, organic-rich siliciclastic mudstones and shales of the Alum Shale Formation (Nielsen & Schovsbo, Reference Nielsen and Schovsbo2007). The latter formation ranges from the upper part of Cambrian Series 3 through the lower Tremadocian and contains subordinate limestone beds and concretionary lenses, referred to as orsten or stinkstone (for general reviews, see Martinsson, Reference Martinsson and Holland1974; Bergström & Gee, Reference Bergström, Gee, Gee and Sturt1985; Andersson et al. Reference Andersson, Dahlman, Gee and Snäll1985; Thickpenny, Reference Thickpenny, Leggett and Zuffa1987; Buchardt, Nielsen & Schovsbo, Reference Buchardt, Nielsen and Schovsbo1997).
The epeiric sea that covered the Baltoscandian (or Baltic) palaeobasin was characterized by significant spatial and temporal variations in the redox state of the seafloor and water column. The Terreneuvian and Cambrian Series 2 sandstone-dominated succession was deposited in shallow marine and well-oxygenated environments, whereas the kerogen-rich strata of the Alum Shale Formation suggest deposition under poorly oxygenated (dysoxic to anoxic) conditions in a shallow to moderately deep sea (e.g. Westergård, Reference Westergård1922; Henningsmoen, Reference Henningsmoen1957; Thickpenny, Reference Thickpenny, Stow and Piper1984, Reference Thickpenny, Leggett and Zuffa1987; Buchardt, Nielsen & Schovsbo, Reference Buchardt, Nielsen and Schovsbo1997; Schovsbo, Reference Schovsbo2001, Reference Schovsbo2002; Nielsen & Schovsbo, Reference Nielsen, Schovsbo, Lindskog and Mehlqvist2013; Egenhoff et al. Reference Egenhoff, Fishman, Ahlberg, Maletz, Jackson, Kolte, Lowers, Mackie, Newby and Petrowsky2015).
This paper focuses on the Cambrian succession in a new drill core, Grönhögen-2015, from the classical geological outcrop area of the island of Öland, southern Sweden. The purpose of the paper is to describe the general stratigraphy of the Grönhögen-2015 core, and to present a high-resolution biostratigraphy and a δ13Corg isotope stratigraphy of its Cambrian portion. The biostratigraphy is based on agnostoids and polymerid trilobites. The δ13Corg chemostratigraphy is tied directly to the biostratigraphy, and its relations to that of coeval successions in Baltoscandia and elsewhere are discussed. This is one of the very few δ13Corg investigations in the Cambrian Series 3 through Lower Ordovician (Tremadocian) successions in Baltoscandia and also one of the few δ13Corg studies dealing with this interval in the world.
2. Location and general remarks
In the spring of 2015, a core drilling, Grönhögen-2015, was performed adjacent to Mörbylånga municipality's groundwater wells at Grönhögen, southern Öland, Sweden (Erlström, Reference Erlström2016; Fig. 1b). The drilling penetrated a 50.15 m thick succession of Lower–Middle Ordovician (0–~ 19.0 m), Furongian (~ 19.0−25.73 m) and Cambrian Series 3 (25.73−50.15 m) strata. All depths are measured from the ground level. The core, which has a diameter of 83 mm down to 15.00 m and 61 mm between 15.00 and 50.15 m, is housed at the Geological Survey of Sweden, Lund, Sweden. The recovery of the tectonically undisturbed, essentially horizontal, core rock succession is close to 100%.
The drilling was made by the Engineering Geology group of the Department of Measurement Technology and Industrial Electrical Engineering, Lund University, with an Atlas Copco CT20 drill rig (Riksriggen). The purpose of the drilling was to obtain information on the subsurface bedrock geology and to collect sample material for a chemical characterization of the bedrock. The core drilling included geophysical borehole logging, for example gamma ray logging, and a detailed XRF-scanning of the core (see Erlström Reference Erlström2016). The XRF (X-ray fluorescence) concentrations of more than 30 elements were determined. These elements include molybdenum and vanadium that are important proxies for redox conditions during deposition (for Mo and V logs, see Erlström Reference Erlström2016, fig. 11).
The major portion of the drill core is represented by the Cambrian Series 3 (Stage 5) Borgholm Formation and the Cambrian Series 3 (Guzhangian) through Lower Ordovician (Tremadocian) Alum Shale Formation (Erlström, Reference Erlström2016; Fig. 2). The succession shows no tectonic disturbances or major late diagenetic alteration, and has most likely not been buried below the oil window (cf. Buchardt, Nielsen & Schovsbo, Reference Buchardt, Nielsen and Schovsbo1997, fig. 19).

Figure 2. Lithological succession and formation classification of the Grönhögen-2015 drill core, Öland, Sweden. The m-figures to the left of the columns refer to drilling depth.
3. Materials and methods
The core was split up and examined at the centimetre scale in the laboratory. Fossils and macroscopic lithological characteristics were recorded and examined under a binocular light microscope. Subsequently, each fossil was meticulously studied and identified, generally to species level. Selected diagnostic fossils were painted with opaque matt black and then lightly coated with a sublimate of ammonium chloride prior to being photographed using a digital camera (Canon 550D) mounted on a table-set camera holder with four external light sources. Figured specimens are stored in the type collections of the Department of Geology, Lund University, Sweden (LO, which signifies Lund Original).
A total of 73 samples were collected from a 41.16 m thick rock interval (50.11–8.95 m) of the Grönhögen-2015 drill core. All samples were subjected to processing for δ13Corg following the procedure described by Ahlberg et al. (Reference Ahlberg, Axheimer, Babcock, Eriksson, Schmitz and Terfelt2009) and Terfelt, Eriksson & Schmitz (Reference Terfelt, Eriksson and Schmitz2014). Carbon isotope analyses of organic carbon were performed with a Flash EA 2000 elemental analyser connected online to a ThermoFinnigan Delta V Plus mass spectrometer. All carbon isotope values are reported in the conventional δ-notation in per mil relative to the V-PDB (Vienna-Pee Dee Belemnite). Accuracy and reproducibility of the analyses were checked by replicate analyses of laboratory standards calibrated to international standards USGS 40 and 41. Reproducibility was ±0.05‰ (1σ). The obtained δ13Corg values are listed in Table 1 and used for the isotope curve described and discussed below.
Table 1. Stable isotope data from organic matter (δ13Corg) from the Grönhögen-2015 drill core. All values are reported relative to Vienna-Pee Dee Belemnite (V-PDB)

4. Lithologic succession
The lowermost 18.45 m (50.15−31.70 m) of the Grönhögen-2015 drill core consists of a relatively uniform succession of alternating grey or reddish grey siltstones and siliciclastic mudstones with thin shale partings (Fig. 2). This succession represents the Äleklinta Member of the Borgholm Formation (see Nielsen & Schovsbo, Reference Nielsen and Schovsbo2007, Reference Nielsen and Schovsbo2015). Bioturbation and small-scale cross-bedding occur frequently throughout this interval, particularly in siltstone beds in the upper part (cf. Erlström, Reference Erlström2016).
The Äleklinta Member is disconformably overlain by the Cambrian Series 3 (Guzhangian) through Lower Ordovician (Tremadocian) Alum Shale Formation, which has a thickness of 22.30 m (31.70−9.40 m). The lowermost part of the Alum Shale Formation is represented by a c. 7 cm thick basal conglomerate with mudstone clasts, the Exporrecta Conglomerate Bed. This calcareous conglomerate rests with a distinct disconformity on the Äleklinta Member and is in turn disconformably overlain by dark grey silt-rich mudstones (31.63−30.42 m).
The remainder of the Alum Shale Formation (30.42−9.40 m) consists of dark grey to black shales and siliciclastic mudstones with several concretionary limestone lenses and prominent limestone beds, including the Kakeled Limestone Bed, which comprises several beds separated by black shale (cf. Nielsen & Schovsbo, Reference Nielsen and Schovsbo2007; Rasmussen, Rasmussen & Nielsen, Reference Rasmussen, Rasmussen and Nielsen2017). Some of the limestone beds are conglomeratic or brecciated, the most conspicuous of them between 20.38 and 20.12 m.
The upper 9.40 m of the core succession includes the Lower Ordovician Bjørkåsholmen Formation (Tremadocian) and ‘Latorp Limestone’ (?Tremadocian−Floian, provisional topoformation), which in turn are overlain by the Middle Ordovician (Dapingian−Darriwilian) ‘Lanna’ and ‘Holen’ limestones (topoformations; see Lindskog & Eriksson, Reference Lindskog and Eriksson2017).
5. Biostratigraphy
Cambrian Series 3 and Furongian strata of Scandinavia are generally richly fossiliferous. The faunas are commonly dominated by polymerid trilobites and agnostoid arthropods, which provide a firm basis for the biostratigraphic classification (e.g. Westergård, Reference Westergård1922, Reference Westergård1946, Reference Westergård1947a; Henningsmoen, Reference Henningsmoen1957; Ahlberg, Reference Ahlberg2003; Axheimer & Ahlberg, Reference Axheimer and Ahlberg2003; Axheimer et al. Reference Axheimer, Eriksson, Ahlberg and Bengtsson2006; Høyberget & Bruton, Reference Høyberget and Bruton2008). Recent efforts to produce a high-resolution trilobite zonation of the Series 3 and Furongian in Scandinavia, especially in southern Sweden and southern Norway, have resulted in new zonal nomenclature, and because of significant differences in ecologic and geographic distributions, separate zonal schemes are now being used for the polymerid trilobites and the agnostoids of Scandinavia (e.g. Terfelt et al. Reference Terfelt, Eriksson, Ahlberg and Babcock2008; Terfelt, Ahlberg & Eriksson, Reference Terfelt, Ahlberg and Eriksson2011; Ahlberg & Terfelt, Reference Ahlberg and Terfelt2012; Nielsen et al. Reference Nielsen, Weidner, Terfelt and Høyberget2014; Rasmussen, Nielsen & Schovsbo, Reference Rasmussen, Nielsen and Schovsbo2015; Babcock, Peng & Ahlberg, Reference Babcock, Peng and Ahlberg2017).
The succession and ranges of agnostoids and trilobites in the Cambrian of Öland have been studied by, for example, Westergård (Reference Westergård1922, Reference Westergård1936, Reference Westergård1944, Reference Westergård1947b), Wærn (Reference Wærn1952), Weidner & Nielsen (Reference Weidner and Nielsen2009) and Rasmussen, Rasmussen & Nielsen (Reference Rasmussen, Rasmussen and Nielsen2017). Their studies have shown that there are several unconformities and substantial gaps both in Series 3 and in the Furongian.
The preservation of fossils in the Grönhögen-2015 drill core is often excellent in the limestones, but less good in the shales and mudstones. In addition to agnostoids and polymerid trilobites, the Cambrian succession of the drill core also contains brachiopods, phosphatocopine arthropods and trace fossils.
5.a. Cambrian Series 3
Cambrian Series 3 is currently subdivided into three superzones (the Acadoparadoxides oelandicus, Paradoxides paradoxissimus and Paradoxides forchhammeri superzones) and seven agnostoid zones (Høyberget & Bruton, Reference Høyberget and Bruton2008; Nielsen et al. Reference Nielsen, Weidner, Terfelt and Høyberget2014; Babcock, Peng & Ahlberg, Reference Babcock, Peng and Ahlberg2017). The A. oelandicus Superzone is well developed on Öland, whereas the P. paradoxissimus and P. forchhammeri superzones are incomplete (Westergård, Reference Westergård1946; Martinsson, Reference Martinsson and Holland1974).
The Cambrian Series 3 succession is incomplete in the Grönhögen-2015 drill core and only represented by upper Stage 5 and Guzhangian strata (Fig. 3). Trace fossils occur in abundance in the thin-bedded mudstones and siltstones of the Äleklinta Member, but no body fossils were found. Elsewhere on Öland, the Äleklinta Member has locally yielded a fairly diverse fauna indicative of the Ptychagnostus gibbus Zone (upper Stage 5; Weidner & Nielsen, Reference Weidner and Nielsen2009). The Exporrecta Conglomerate is poorly constrained biostratigraphically, but generally considered equivalent to the Andrarum Limestone Bed (Guzhangian Stage; lower Lejopyge laevigata Zone) of Scania (Skåne), southern Sweden. The interval between 31.63 m and 25.73 m is assigned to the Agnostus pisiformis Zone (Fig. 3). The lower boundary of the zone is, however, difficult to firmly establish since the lowermost c. 1.6 m of this interval is largely unfossiliferous, except for a few trace fossils. The eponymous species (Fig. 4a) ranges from 30.00 m to 28.30 m and is abundant at some levels. The upper part of the A. pisiformis Zone is barren of body fossils, apart from a few phosphatocopine arthropods (Cyclotron sp.) and linguliformean brachiopods near the top of the zone.

Figure 3. Biostratigraphy and ranges of fossils in the Alum Shale Formation of the Grönhögen-2015 drill core, Öland, Sweden.

Figure 4. Fossils from Cambrian Series 3 and the Furongian in the Grönhögen-2015 drill core. Scale bars correspond to 1 mm. (a) Agnostus pisiformis (Wahlenberg, Reference Wahlenberg1818), cephala and pygidia from the A. pisiformis Zone (29.90 m), LO 12418t. (b–d) Olenus truncatus (Brünnich, Reference Brünnich1781) from the O. truncatus Zone: (b) cranidium (25.15–25.18 m), LO 12419t; (c) cranidium (25.25 m), LO 12420t; (d) cranidia and librigenae (25.15–25.18 m), LO 12421t. (e) Olenus gibbosus (Wahlenberg, Reference Wahlenberg1818), pygidium from the O. gibbosus Zone (25.73 m), LO 12422t. (f) Cyclotron cf. angelini (Linnarsson, Reference Linnarsson1875) from the O. truncatus Zone (25.18–25.20 m), LO 12423t. (g, h) Agnostus (Homagnostus) obesus (Belt, Reference Belt1867) from the O. truncatus Zone (25.15–25.18 m): (g) cephalon, LO 12424t; (h) pygidium, LO 12425t. (i) Orusia lenticularis (Wahlenberg, Reference Wahlenberg1818), abundant specimens from the P. spinulosa Zone (23.65 m), LO 12426t. (j) Ctenopyge directa Lake, Reference Lake1919, cranidium from the base of the C. linnarssoni Zone (20.65–20.70 m), LO 12427t. (k) Sphaerophthalmus sp., cranidium from the base of the Ctenopyge tumida Zone (21.88 m), LO 12428t. (l–n) Sphaerophthalmus alatus (Boeck, Reference Boeck and Keilhau1838) from the Ctenopyge tumida Zone (21.05–21.10 m): (l) cranidium, LO 12429t; (m) cranidium, LO 12430t; (n) cranidium, LO 12431t. (o) Triangulopyge humilis (Phillips, Reference Phillips1848), cranidium from the base of the C. linnarssoni Zone (20.65–20.70 m), LO 12432t. (p) Peltura sp., cranidium from the base of the C. linnarssoni Zone (20.65–20.70 m), LO 12433t. (q, r) Peltura scarabaeoides westergaardi Henningsmoen, Reference Henningsmoen1957 from the Parabolina lobata Zone: (q) pygidium (19.65 m), 12434t; (r) pygidium (19.90–19.92 m), LO 12435t. (s) Parabolina cf. lobata, cranidium from the base of the P. lobata Zone (19.90–19.92 m), LO 12436t.
5.b. Furongian
The Furongian biostratigraphy of Scandinavia is largely based on the succession of olenid trilobites. The rate of faunal turnover is very high, which enabled Westergård (Reference Westergård1922, Reference Westergård1947a) and Henningsmoen (Reference Henningsmoen1957) to establish a high-resolution biostratigraphy. Their biostratigraphical scheme has subsequently been slightly modified (Terfelt et al. Reference Terfelt, Eriksson, Ahlberg and Babcock2008; Terfelt, Ahlberg & Eriksson, Reference Terfelt, Ahlberg and Eriksson2011; Høyberget & Bruton, Reference Høyberget and Bruton2012; Weidner & Nielsen, Reference Weidner and Nielsen2013; Rasmussen, Nielsen & Schovsbo, Reference Rasmussen, Nielsen and Schovsbo2015) and the Furongian of Scandinavia is now being subdivided into six superzones and 26 polymerid (olenid) trilobite zones that can be linked to four parallel agnostoid zones (Nielsen et al. Reference Nielsen, Weidner, Terfelt and Høyberget2014; Rasmussen, Rasmussen & Nielsen, Reference Rasmussen, Rasmussen and Nielsen2017; Babcock, Peng & Ahlberg, Reference Babcock, Peng and Ahlberg2017, fig. 3). In ascending order, the Furongian Series includes: the Olenus, Parabolina, Leptoplastus, Protopeltura, Peltura and Acerocarina superzones (Nielsen et al. Reference Nielsen, Weidner, Terfelt and Høyberget2014). All superzones have been recorded on Öland but they are partially or largely incomplete (Westergård, Reference Westergård1947a; Rasmussen, Rasmussen & Nielsen, Reference Rasmussen, Rasmussen and Nielsen2017).
Agnostoids and trilobites allowed subdivision of the Furongian succession of the Grönhögen-2015 drill core into seven biozones, in ascending order: the Olenus gibbosus, O. truncatus, Parabolina spinulosa, Sphaerophthalmus? flagellifer, Ctenopyge tumida, C. linnarssoni and Parabolina lobata zones (Fig. 3). The biostratigraphy and conspicuously developed unconformities show that the Alum Shale Formation is incomplete and that there are several substantial gaps of variable magnitudes. The Leptoplastus (Jiangshanian) and Acerocarina (uppermost Stage 10) superzones appear to be missing, and the Olenus (Paibian) and Protopeltura (upper Jiangshanian) superzones are incomplete (Fig. 5).

Figure 5. Stratigraphical subdivision of the Cambrian succession in the Grönhögen-2015 drill core, Öland, Sweden. Grey shading indicates unconformities. Biostratigraphy based on Axheimer et al. (Reference Axheimer, Eriksson, Ahlberg and Bengtsson2006), Høyberget & Bruton (Reference Høyberget and Bruton2008), Terfelt et al. (Reference Terfelt, Eriksson, Ahlberg and Babcock2008), Terfelt, Ahlberg & Eriksson (Reference Terfelt, Ahlberg and Eriksson2011), Nielsen et al. (Reference Nielsen, Weidner, Terfelt and Høyberget2014), Rasmussen, Nielsen & Schovsbo (Reference Rasmussen, Nielsen and Schovsbo2015) and Babcock, Peng & Ahlberg (Reference Babcock, Peng and Ahlberg2017).
The base of the Furongian Series and the Paibian Stage is placed at the lowest occurrence Olenus gibbosus (Fig. 4e). This species occurs at 25.73–25.35 m and is indicative of the O. gibbosus Zone, the base of which coincides with the first appearance datum (FAD) of Glyptagnostus reticulatus (see Peng et al. Reference Peng, Babcock, Robison, Lin, Rees and Saltzman2004; Ahlberg & Terfelt, Reference Ahlberg and Terfelt2012; Nielsen et al. Reference Nielsen, Weidner, Terfelt and Høyberget2014). The O. gibbosus Zone is succeeded by a 20 cm thick succession with O. truncatus (Fig. 4b–d), Agnostus (Homagnostus) obesus (Fig. 4g, h) and the phosphatocopine Cyclotron cf. angelini (Fig. 4f).
The base of the Parabolina Superzone, which roughly coincides with the base of the Jiangshanian Stage (Ahlberg & Terfelt, Reference Ahlberg and Terfelt2012), cannot be positively identified in the drill core, as there is a barren interval between the lower Paibian O. truncatus Zone and the Jiangshanian Parabolina spinulosa Zone. However, the orthid brachiopod Orusia lenticularis (Fig. 4i) is most commonly associated with Parabolina spinulosa (Westergård, Reference Westergård1922; Terfelt, Reference Terfelt2003) and its presence in the 23.65–22.49 m interval is suggestive of the P. spinulosa Zone. Following the Parabolina Superzone there is a substantial hiatus and the Leptoplastus Superzone and most of the Protopeltura Superzone are missing; only the Sphaerophthalmus? flagellifer Zone has been positively identified in the middle and upper Jiangshanian, with the eponymous species occurring at 22.10 m.
Cambrian Stage 10 strata are represented by the Ctenopyge tumida, C. linnarssoni and Parabolina lobata zones. The base of the C. tumida Zone is placed at the first occurrence of a species of Sphaerophthalmus at 21.88 m (Fig. 4k; cf. Terfelt, Ahlberg & Eriksson, Reference Terfelt, Ahlberg and Eriksson2011). The lower and middle part of this zone has yielded S. alatus (Fig. 4l–n) and Peltura cf. minor. The Ctenopyge bisulcata Zone appears to be missing and the C. tumida Zone is followed by the C. linnarssoni Zone, the base of which is placed at 20.70 m and at the lowest occurrences of Triangulopyge humilis (Fig. 4o), Ctenopyge directa (Fig. 4j) and C. cf. pecten. The first occurrence of Peltura scarabaeoides westergaardi (Fig. 4r) and Parabolina cf. lobata (Fig. 4s) at 19.92–19.90 m is indicative of the base of the P. lobata Zone. The top of this zone is placed at the last occurrence of P. scarabaeoides westergaardi (Fig. 4q) at 19.65 m. The Parabolina lobata Zone is overlain by a thin (0.4 m) succession of unfossiliferous shales that may represent the Peltura paradoxa Zone. A prominent hiatus, probably comprising the uppermost four zones in the Furongian (the Acerocarina granulata Zone through the Acerocare ecorne Zone), is present between the top of the Parabolina lobata/Peltura paradoxa Zone and the lowermost Ordovician (Tremadocian) succession (Fig. 5). The base of the Ordovician is poorly constrained biostratigraphically but is tentatively placed at c. 19.0 m, below the first graptolite (Adelograptus? sp. at 17.95 m) and where there is a distinctive negative jump in the carbon isotopic curve.
6. δ13Corg chemostratigraphy
During the last three decades, the potential of carbon isotopes for global stratigraphical correlation of Cambrian strata has attracted a great deal of international interest (e.g. Brasier Reference Brasier, Hailwood and Kidd1993; Montañez et al. Reference Montañez, Osleger, Banner, Mack and Masgrove2000; Saltzman et al. Reference Saltzman, Ripperdan, Brasier, Lohmann, Robison, Chang, Peng, Ergaliev and Runnegar2000, Reference Saltzman, Cowan, Runkel, Runnegar, Stewart and Palmer2004; Zhu et al. Reference Zhu, Zhang, Li and Yang2004; Zhu, Babcock & Peng, Reference Zhu, Babcock and Peng2006; Peng, Babcock & Cooper, Reference Peng, Babcock, Cooper, Gradstein, Ogg, Schmitz and Ogg2012 and references therein). Although many studies on Cambrian chemostratigraphy have been carried out, most investigations focused on δ13Ccarb using samples from carbonate-dominated or carbonate-rich successions. The Cambrian Series 3 through Furongian succession of Scandinavia is dominated by shales and siliciclastic mudstones. δ13Corg has previously been used in the Cambrian of Scandinavia by Ahlberg et al. (Reference Ahlberg, Axheimer, Babcock, Eriksson, Schmitz and Terfelt2009), Lehnert et al. (Reference Lehnert, Ahlberg, Calner, Joachimski, Lindskog and Mehlqvist2013), Terfelt, Eriksson & Schmitz (Reference Terfelt, Eriksson and Schmitz2014), Lundberg et al. (Reference Lundberg, Ahlberg, Eriksson, Lindskog, Laurie, Kruse, García-Bellido and Holmes2016) and Hammer & Svensen (Reference Hammer and Svensen2017). These studies and the present one show that δ13Corg chemostratigraphy is useful for correlations between the siliciclastic-dominated successions of Scandinavia and carbonate successions elsewhere in the world.
The 15 stratigraphically lowest samples from the Grönhögen-2015 drill are from the Äleklinta Member (upper Stage 5) and show δ13Corg values between –29.1 and –28.4‰ (Fig. 6). At the base of the Agnostus pisiformis Zone (31.6 m), there is a significant negative shift in δ13Corg of c. −2.5‰. This is followed by a positive trend with minor fluctuations in the A. pisiformis Zone (Fig. 6).

Figure 6. Lithologic log and plot of δ13Corg values through the Cambrian and Lower Ordovician (Tremadocian) of the Grönhögen-2015 drill core. Note the positions of the SPICE and TOCE excursions recognized in the present study. Jiangsh. – Jiangshanian.
The carbon isotopic curve through the Furongian shows a distinctive positive excursion in the Paibian (core depth 25.7−23.6 m), which is tied closely to the biostratigraphy (Fig. 6). The amplitude and stratigraphic position of this excursion strongly suggests that it is the Steptoean Positive Carbon Isotopic Excursion (SPICE; e.g. Saltzman et al. Reference Saltzman, Ripperdan, Brasier, Lohmann, Robison, Chang, Peng, Ergaliev and Runnegar2000; Kouchinsky et al. Reference Kouchinsky, Bengtson, Gallet, Korovnikov, Pavlov, Runnegar, Shields, Veizer, Young and Ziegler2008; Ahlberg et al. Reference Ahlberg, Axheimer, Babcock, Eriksson, Schmitz and Terfelt2009; Gill et al. Reference Gill, Lyons, Young, Kump, Knoll and Saltzman2011; Wotte & Strauss, Reference Wotte and Strauss2015; Schiffbauer et al. Reference Schiffbauer, Huntley, Fike, Jeffrey, Gregg and Shelton2017). It has an amplitude of nearly +2‰, begins near the first appearance of Olenus gibbosus (base of the Paibian), and extends upward into the O. truncatus Zone and slightly younger beds (?upper Olenus Superzone). A relatively minor (c. −0.5‰) but consistent trend to more negative δ13Corg values near the top of the Cambrian is seen near the base of the Ctenopyge linnarssoni Zone. It displays nadir values just below and above the Parabolina lobata Zone. Based on its stratigraphic position, we interpret this interval (21.0−~ 19.0 m) as an equivalent to the Top of Cambrian Excursion (TOCE; Zhu, Babcock & Peng, Reference Zhu, Babcock and Peng2006). The end of the putative TOCE cannot be precisely recognized because there is likely a gap between the Parabolina lobata/Peltura paradoxa Zone and the basal Ordovician. The transition between the Cambrian and the Ordovician is marked by a c. −0.6‰ shift in the carbon isotope values. In the lowermost Ordovician (lower Tremadocian) part of the drill core, δ13Corg values are around –30‰ (Fig. 6).
7. Discussion
The Cambrian succession in the Grönhögen-2015 drill core is lithologically and stratigraphically similar to coeval intervals in other drill cores from southern Öland (see, e.g., Westergård, Reference Westergård1944, Reference Westergård1947b; Erlström, Reference Erlström2016). The Äleklinta Member is generally barren of body fossils and hence biostratigraphically poorly constrained. However, recent studies show that it should be assigned to the Ptychagnostus gibbus Zone (Weidner & Nielsen, Reference Weidner and Nielsen2009; Nielsen & Schovsbo, Reference Nielsen and Schovsbo2015). The Äleklinta Member is disconformably overlain by the Exporrecta Conglomerate (Guzhangian Stage, probably lower Lejopyge laevigata Zone; Axheimer et al. Reference Axheimer, Eriksson, Ahlberg and Bengtsson2006), which in turn is overlain by the upper Guzhangian Agnostus pisiformis Zone. Thus, the entire Drumian Stage seems to be missing in the Grönhögen-2015 drill core (Fig. 5). The Alum Shale Formation has a thickness of 22.3 m. This figure is closely comparable to the thickness of this formation at Ottenby, 8 km south of Grönhögen (23.3 m; Westergård, Reference Westergård1944) and Gammalsby, 18 km northeast of Grönhögen (18.8 m; Westergård, Reference Westergård1944). The Alum Shale Formation gradually thins out towards the NNW of Öland (Westergård, Reference Westergård1944, Reference Westergård1947b; Erlström, Reference Erlström2016). On southernmost Öland, the top of the Cambrian is generally formed by a thin, less than 0.6 m thick, succession assigned to the Acerocarina granulata Zone of Weidner & Nielsen (Reference Weidner and Nielsen2013) (Westergård Reference Westergård1944, Reference Westergård1947a,b). This zone and the underlying Peltura paradoxa Zone cannot be positively identified in the Grönhögen-2015 drill core owing to lack of fossils in the 19.6−19.0 m interval overlying the Parabolina lobata Zone. At the Degerhamn quarry, 15 km north of Grönhögen, the top of the Cambrian consists of strata assigned to the P. lobata Zone (Rasmussen, Rasmussen & Nielsen, Reference Rasmussen, Rasmussen and Nielsen2017).
Despite some scatter in the δ13Corg values (Fig. 6) in parts of the drill core succession, two globally significant excursions can be identified, the SPICE and a subdued TOCE (previously referred to as the HERB Event; Ripperdan, Reference Ripperdan2002), both of which are generally considered as large and rapid excursions indicative of perturbations in the oceanic carbon cycle (e.g. Ripperdan et al. Reference Ripperdan, Magaritz, Nicoll and Shergold1992; Saltzman et al. Reference Saltzman, Ripperdan, Brasier, Lohmann, Robison, Chang, Peng, Ergaliev and Runnegar2000, Reference Saltzman, Cowan, Runkel, Runnegar, Stewart and Palmer2004; Miller et al. Reference Miller, Ripperdan, Loch, Freeman, Evans, Taylor and Tolbart2015). The onset of the SPICE is associated with the base of the Furongian Series (Peng et al. Reference Peng, Babcock, Robison, Lin, Rees and Saltzman2004), whereas the TOCE occurs in the lower Eoconodontus Conodont Zone near the top of the Cambrian (e.g. Miller et al. Reference Miller, Evans, Freeman, Ripperdan and Taylor2014, Reference Miller, Ripperdan, Loch, Freeman, Evans, Taylor and Tolbart2015). The SPICE and TOCE excursions have been recorded from most Cambrian palaeocontinents and have great potential for global correlation in the Paibian and Cambrian Stage 10, respectively (e.g. Saltzman et al. Reference Saltzman, Ripperdan, Brasier, Lohmann, Robison, Chang, Peng, Ergaliev and Runnegar2000; Sial et al. Reference Sial, Peralta, Ferreira, Toselli, Aceñolaza, Parada, Gaucher, Alonso and Pimentel2008, Reference Sial, Peralta, Gaucher, Toselli, Ferreira, Frei, Parada, Pimentel and Pereira2013; Landing, Westrop & Adrain, Reference Landing, Westrop and Adrain2011; Woods et al. Reference Woods, Wilby, Leng, Rushton and Williams2011; Gill et al. Reference Gill, Lyons, Young, Kump, Knoll and Saltzman2011; Miller et al. Reference Miller, Evans, Freeman, Ripperdan and Taylor2011, Reference Miller, Evans, Freeman, Ripperdan and Taylor2014, Reference Miller, Ripperdan, Loch, Freeman, Evans, Taylor and Tolbart2015; Ng, Yuan & Lin, Reference Ng, Yuan and Lin2014; Lim et al. Reference Lim, Chung, Park and Lee2016; Azmy, Reference Azmy2018). Although it has been argued (e.g. Landing, Westrop & Adrain, Reference Landing, Westrop and Adrain2011) that the HERB Event is different from the TOCE excursion of Zhu, Babcock & Peng, (Reference Zhu, Babcock and Peng2006), we follow Peng, Babcock & Cooper (Reference Peng, Babcock, Cooper, Gradstein, Ogg, Schmitz and Ogg2012), Terfelt, Eriksson & Schmitz (Reference Terfelt, Eriksson and Schmitz2014), Miller et al. (Reference Miller, Ripperdan, Loch, Freeman, Evans, Taylor and Tolbart2015) and Li et al. (Reference Li, Zhang, Chen, Zhang, Chen, Huang, Peng and Shen2017) in considering them as the same carbon isotopic excursion.
The magnitude of the SPICE and TOCE in the Grönhögen-2015 succession is comparable to δ13Corg curves from drill cores retrieved from Scania, southern Sweden (SPICE from the Andrarum-3 drill core and TOCE from the Håslöv-1 drill core; Ahlberg et al. Reference Ahlberg, Axheimer, Babcock, Eriksson, Schmitz and Terfelt2009; Terfelt, Eriksson & Schmitz, Reference Terfelt, Eriksson and Schmitz2014; Fig. 7) and at Krekling, southern Norway (SPICE; Hammer & Svensen, Reference Hammer and Svensen2017). However, the amplitude and expression of the identified isotopic excursions, especially the TOCE, in Swedish successions are typically quite subdued compared to equivalents recorded in other areas (see below). In our drill core, the SPICE begins near the first appearance of Olenus gibbosus, which is considered to coincide with the first appearance of Glyptagnostus reticulatus and the base of the Furongian Series and the Paibian Stage (Terfelt et al. Reference Terfelt, Eriksson, Ahlberg and Babcock2008; Terfelt, Ahlberg & Eriksson, Reference Terfelt, Ahlberg and Eriksson2011). It extends upward into the O. truncatus Zone and through unfossiliferous shales that may represent the middle and upper Olenus Superzone of Nielsen et al. (Reference Nielsen, Weidner, Terfelt and Høyberget2014). Hence, the SPICE from the Grönhögen-2015 drill core spans a biostratigraphical interval approximately equivalent to that recorded in the Alum Shale of Scandinavia (Andrarum-3 drill core, southern Sweden, and at Krekling, southern Norway; Ahlberg et al. Reference Ahlberg, Axheimer, Babcock, Eriksson, Schmitz and Terfelt2009; Hammer & Svensen, Reference Hammer and Svensen2017) and the Outwoods Shale Formation in Warwickshire, England (Woods et al. Reference Woods, Wilby, Leng, Rushton and Williams2011). This biostratigraphical interval can be assigned to the lower−middle Paibian Stage. In the Grönhögen-2015 core, the putative TOCE begins near the base of the Ctenopyge linnarssoni Zone and has its nadir immediately below and above the Parabolina lobata Zone. In the Håslöv-1 drill core, the TOCE interval displays two peaks, a lower one in the upper P. lobata Zone and an upper one straddling the Parabolina lobata−Peltura paradoxa zonal boundary (Fig. 7; Terfelt, Eriksson & Schmitz, Reference Terfelt, Eriksson and Schmitz2014). A double peak has also been recognized in, e.g., western Newfoundland (Stouge, Bagnoli & Azmi, Reference Stouge, Bagnoli, Azmi, Laurie, Kruse, García-Bellido and Holmes2016). The TOCE seemingly begins slightly earlier in our drill core and two peaks cannot be identified, probably because of condensation and/or too few data points. In terms of the Baltoscandian conodont biostratigraphy, the TOCE excursion spans the upper Proconodontus muelleri Zone and the Cordylodus? andresi Zone, i.e. an interval that can be correlated with the lower Eoconodontus Conodont Zone in Laurentia and elsewhere (Bagnoli & Stouge, Reference Bagnoli and Stouge2014).

Figure 7. Comparison of the δ13Corg curve from the Grönhögen-2015 drill core with δ13Corg curves from the apparently continuous successions in the Andrarum-3 and Håslöv-1 drill cores from Skåne (Scania), southern Sweden. The Andrarum-3 curve is after Ahlberg et al. (Reference Ahlberg, Axheimer, Babcock, Eriksson, Schmitz and Terfelt2009) and the Håslöv-1 curve is after Terfelt, Eriksson & Schmitz (Reference Terfelt, Eriksson and Schmitz2014). Note the closely similar stratigraphic position of the SPICE and TOCE excursions in these successions. Jiang. – Jiangshanian.
The negative −2.5‰ shift at the base of the Alum Shale Formation coincides with a substantial hiatus and a shift in lithology from mudstones and siltstones in the Äleklinta Member (upper Stage 5) to dark grey mudstone and shales in the lower Alum Shale Formation (upper Guzhangian). Throughout the overlying Agnostus pisiformis Zone (upper Guzhangian), δ13Corg values increase until the base of the SPICE (Fig. 6). This positive trend has also been recorded from the pre-SPICE interval in the Andrarum-3 drill core from Scania, southern Sweden (Ahlberg et al. Reference Ahlberg, Axheimer, Babcock, Eriksson, Schmitz and Terfelt2009), and elsewhere in the world, notably in South China, Kazakhstan and Australia (e.g. Saltzman et al. Reference Saltzman, Ripperdan, Brasier, Lohmann, Robison, Chang, Peng, Ergaliev and Runnegar2000; Wotte & Strauss, Reference Wotte and Strauss2015). The post-SPICE and pre-TOCE δ13Corg curve in the Grönhögen-2015 drill core displays variable values (between –28.8 and –29.7‰), with two ‘cycles’ in δ13C being apparent. It is, however, worth noting that significant parts of the post-SPICE and pre-TOCE isotope curve are cut out by gaps in the Furongian succession, the most prominent one being in the middle−upper Jiangshanian Stage.
The overall trends in the presented isotope curve are similar to those present in some published δ13Ccarb curves through coeval stratigraphic intervals in other parts of the world. The shift of the δ13Corg in the excursions recorded in the Grönhögen-2015 drill core is approximately half (SPICE) or less than one-fourth (TOCE) the magnitude of coeval δ13Ccarb excursions documented from other regions (see also Terfelt, Eriksson & Schmitz, Reference Terfelt, Eriksson and Schmitz2014). This difference may be related to spatial and temporal variations in the origin, composition, alteration and diagenesis of the organic matter analysed (Ahlberg et al. Reference Ahlberg, Axheimer, Babcock, Eriksson, Schmitz and Terfelt2009), with different geographic areas hosting unique geochemical conditions that influence and partly overprint the global δ13C signal. Still, the present study shows that the SPICE and TOCE are useful for long-distance correlations in both shaly and carbonate successions.
8. Conclusions
The Grönhögen-2015 core drilling penetrated 50.15 m of Cambrian Series 3, Furongian and Lower–Middle Ordovician strata. The lower part of the drill core succession belongs to the upper Äleklinta Member (Borgholm Formation; Cambrian Series 3), which is disconformably overlain by the Cambrian Series 3 (Guzhangian) through Lower Ordovician (Tremadocian) Alum Shale Formation. The upper part of the drill core includes the Lower Ordovician Bjørkåsholmen Formation (Tremadocian) and ‘Latorp Limestone’ (?Tremadocian−Floian, topoformation), which in turn are overlain by the Middle Ordovician (Dapingian−Darriwilian) ‘Lanna’ and ‘Holen’ limestones (topoformations).
Agnostoids and trilobites allowed subdivision of the succession into eight biozones (in ascending order): the uppermost Cambrian Series 3 (Guzhangian) Agnostus pisiformis Zone and the Furongian Olenus gibbosus, O. truncatus, Parabolina spinulosa, Sphaerophthalmus? flagellifer, Ctenopyge tumida, C. linnarssoni and Parabolina lobata zones. The biostratigraphy and conspicuous unconformities show that the Alum Shale Formation is incomplete and that there are several substantial gaps of variable magnitudes. The Furongian Leptoplastus Superzone (Jiangshanian) and Acerocarina Superzone (Stage 10) appear to be missing, and the Paibian Olenus and upper Jiangshanian Protopeltura superzones are incomplete.
The Grönhögen-2015 drill core offers an excellent opportunity to calibrate the Furongian δ13Corg curve with the Furongian standard trilobite and agnostoid zone succession of Baltoscandia. Carbon isotopic analyses (δ13Corg) through the Alum Shale Formation show two globally significant excursions, the Steptoean Positive Carbon Isotopic Excursion (SPICE) and the Top of Cambrian Carbon isotopic Excursion (TOCE), previously referred to as the HERB Event. The SPICE has an amplitude of c. +1.5−2‰, begins near the first appearance of Olenus gibbosus (base of the Furongian Series and the Paibian Stage), and extends upward into the O. truncatus Zone and slightly younger beds (middle and ?upper Olenus Superzone). The negative TOCE, which is poorly expressed in the studied succession (net shift c. −0.5‰), occurs in Stage 10, begins near the base of the Ctenopyge linnarssoni Zone and displays nadir values immediately below and above the Parabolina lobata Zone. The net shifts of the excursions are comparable to those recorded in drill cores retrieved from Scania, southern Sweden (SPICE from the Andrarum-3 drill core and TOCE from the Håslöv-1 drill core), but they are subdued compared to international counterparts. The occurrence of the TOCE δ13Corg excursion in Stage 10 in southern Sweden has potential for global correlation of the uppermost Cambrian in Baltoscandia with coeval successions elsewhere in the world.
Acknowledgements
This research was supported in part by The Gyllenstierna Krapperup's Foundation grant 2014-0100 to Ahlberg. The municipality of Mörbylånga is thanked for their support and contribution to the drilling operations in 2015. Magne Høyberget kindly commented upon the identification of some trilobites. We are also indebted to two anonymous reviewers for useful comments that helped to improve the paper.