Introduction
Alexander Campbell Martin was born in 1897, and he obtained a PhD from George Washington University in Washington, DC (USA), presumably in 1944; his doctorate thesis was submitted in December 1943. His PhD work was published in 1946 in The American Midland Naturalist, and the title was ‘The comparative internal morphology of seeds’. This paper contains drawings and information on embryos in 1287 genera of seed plants. Interestingly, Martin was 49 years old when this paper was published. While Martin worked on his PhD research, he was employed at the Patuxent Research Refuge of the USA Fish and Wildlife Service near Laurel, Maryland. He worked at this Refuge from 1940 until his retirement in about 1963. As we approach the 125 year anniversary of his birth and the 75th year since the publication of his classic paper on the internal morphology of seeds, we think it is appropriate to reflect on Martin's contributions to seed science.
The major contributions of Martin's Reference Martin1946 paper, include (1) development of a comprehensive database on embryo morphology of seeds, (2) a family tree of seed phylogeny based on morphological traits and (3) discussion and questions about some of the kinds of embryos, e.g. the peripheral embryo, that still provide challenges for seed biologists today. Martin was not the first person to pay attention to seed anatomy (see Netolitzky, Reference Netolitzky1926). However, his comprehensive survey gave him the background information with which to contemplate relationships between kinds of embryos and allowed him to construct the first family tree of seed phylogeny.
The information in Martin's Reference Martin1946 paper has been widely used by seed biologists/ecologists (e.g. Keeley, Reference Keeley1991; Finch-Savage and Leubner-Metzger, Reference Finch-Savage and Leubner-Metzger2006; Vyshenskaya, Reference Vyshenskaya and Batygina2006; Baskin and Baskin, Reference Baskin and Baskin2014). His work has played a role in studies of seed anatomy (Vaughan and Whitehouse, Reference Vaughan and Whitehouse1971; Gunn, Reference Gunn1974; Boesewinkel and Bouman, Reference Boesewinkel, Bouman, Kigel and Galili1995; Werker, Reference Werker1997), development (Floyd and Friedman, Reference Floyd and Friedman2000), evolution (Grushvitskii, Reference Grushvitskii1961; Forbis et al., Reference Forbis, Floyd and Queiroz2002; Eriksson and Kainulainen, Reference Eriksson and Kainulainen2011; Willis et al., Reference Willis, Baskin, Baskin, Auld, Venable, Cavender-Bares, Donohue and Rubio de Casas2014) and technology/physiology (Lang, Reference Lang1965; Grabe, Reference Grabe1970; Justice, Reference Justice and Kozlowski1972; Bass et al., Reference Bass, Gunn, Hesterman and Roos1988). In addition, Martin's paper has been cited in studies on plant taxonomy (Reeder, Reference Reeder1957; Takhtajan, Reference Takhtajan1980, Reference Takhtajan1997), embryology (Raghaven, Reference Raghaven1986; Vyshenskaya, Reference Vyshenskaya and Batygina2006), evolution (Carlquist, Reference Carlquist1961; Dahlgren and Rasmussen, Reference Dahlgren, Rasmussen, Hecht, Wallace and Prance1983; Thorne, Reference Thorne2000), propagation (Hartman and Kester, Reference Hartman and Kester1975) and floristics/taxonomic revisions (Palser, Reference Palser1951; Fryxell, Reference Fryxell1978; Hodgson and Mackey, Reference Hodgson and Mackey1986; Harley et al., Reference Harley, Atkins, Budantsev, Cantino, Conn, Grayer, Harley, de Kok, Krestovskaja, Morales, Paton, Ryding, Upson and Kadereit2004). According to Google Scholar (29 July 2019), Martin's (Reference Martin1946) paper has been cited 846 times.
Martin had a productive career. However, except for a book co-authored with William D. Barkley (Martin and Barkley, Reference Martin and Barkley1961) entitled ‘Seed Identification Manual’ he did not publish any more work on seed embryos. He published several papers dealing with the food habits of waterfowl and a book (Martin et al., Reference Martin, Zim and Nelson1951) entitled ‘American Wildlife and Plants’, which had 500 pages and 300 illustrations of the genera of plants used as food by wildlife. Martin with co-authors Neil Hotchkiss, Francis Uhler and William Bourn (Martin et al., Reference Martin, Hotchkiss, Uhler and Bourn1953) published a paper entitled ‘Classification of wetlands in the United States’, which was the first attempt to classify wetlands in the USA. He also authored or co-authored four books (Weeds, Trees, Flowers and Wildflowers) in the ‘A Golden Guide’ series of books on natural history (published by St Martin's Press).
One of the intriguing things about Martin's Reference Martin1946 paper is that he was very frank about the aspects of his family tree of seed phylogeny that puzzled him. One of these puzzles is the peripheral embryo. Martin described the peripheral embryo as a ‘phylogenetic orphan’ and wrote several comments about it, two of which are provided here:
‘Peripheral division – Embryo ordinarily elongate and large, quarter to dominant, continuous in part at least to the testa and often curved; endosperm (actually perisperm) conspicuously starchy; central or in a few instances lateral. Cotyledons narrow or expanded. Dicots – but in several cases one of the cotyledons is abortive.’ [p. 519]
‘Peripheral division seems to resemble a blind alley leading nowhere beyond itself; though expanded cotyledons in some Peripheral families show at least superficial resemblance to the Foliate subdivision it appears to be a case of “parallelism” in fundamentally distinct groups. Even if the distinct kind of endosperm [perisperm] did not present an insuperable barrier it still would seem almost inconceivable that a peripheral embryo, with its characteristic position surrounding the endosperm [perisperm] could ever have given rise to central-embryoed members of the Axile group.’ [p. 526]
Not only did Martin consider the peripheral embryo to be a ‘phylogenetic orphan’, but he placed it at the base of his tree, showing it as a branch that originates from the side of something that looks like a tree stump (Fig. 1). Much research on seed morphology and on the phylogeny of angiosperms has been conducted since 1946, but the results of these studies have not been evaluated in terms of Martin's conclusion with regard to the peripheral embryo. In attempting to understand the origins and evolutionary relationships of the various kinds of embryos in seeds of angiosperms, it is important to know if the peripheral embryo is truly a phylogenetic orphan, or is it related to other kinds of embryos? As a tribute to Martin's pioneer work in seed phylogeny, we have revisited his peripheral embryo. We have carefully studied the details about the peripheral embryo provided in Martin's Reference Martin1946 paper and the results from relevant kinds of research conducted since 1946. We can conclude that Martin had great insight with regard to the peripheral embryo, but more can be added to the story, which we think would have fascinated him.
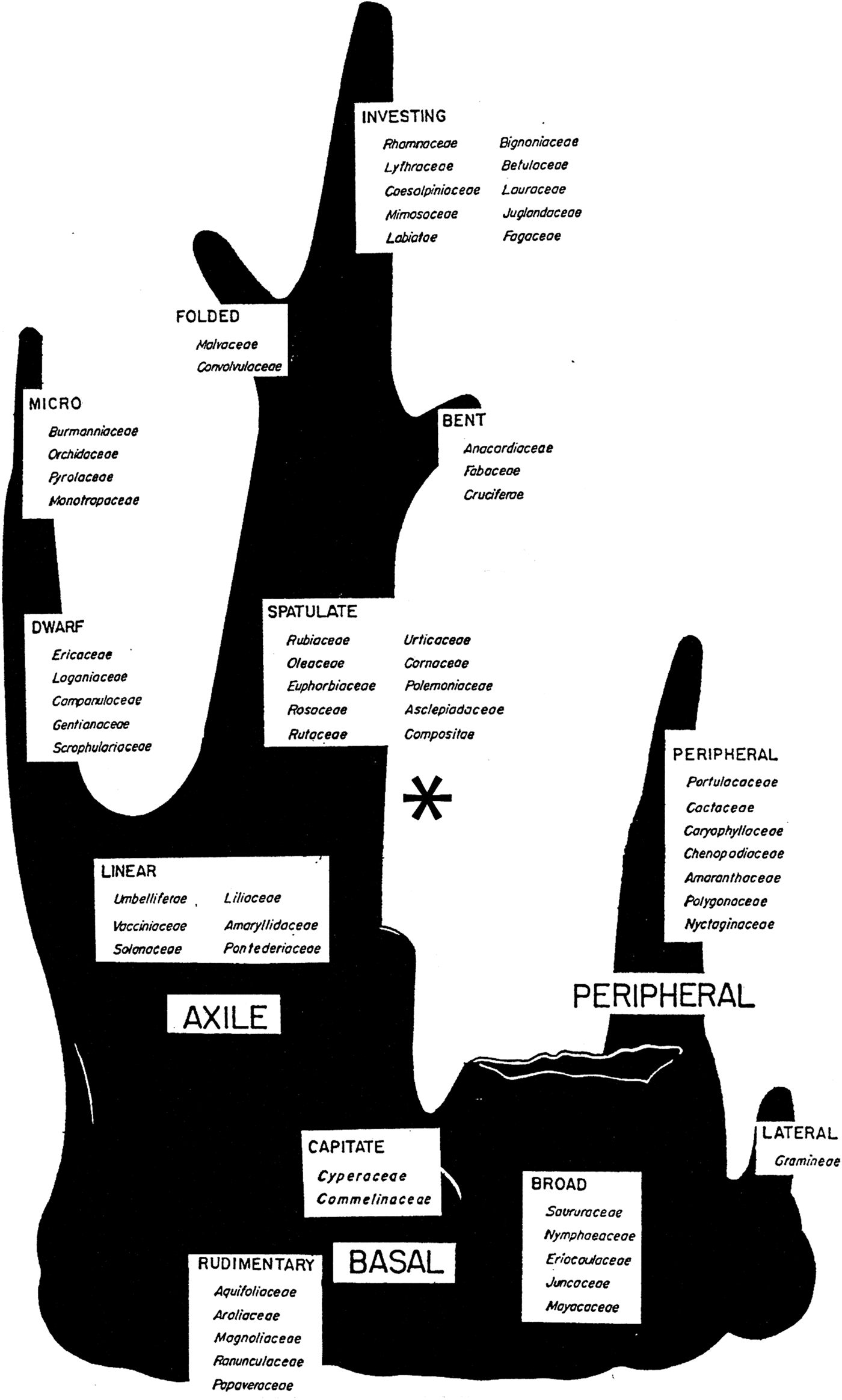
Figure 1. Martin's (Reference Martin1946) family tree of seed phylogeny. *Proposed position on Martin's tree for the peripheral embryo. Reproduced with permission.
From studying the shape and location of the peripheral embryo in the seed, as illustrated in Martin (Reference Martin1946), we hypothesized that seeds with this kind of embryo have distinct morphological and developmental characteristics. Furthermore, based on the variation within Martin's families with peripheral embryos with regard to cotyledon morphology, his comments about the peripheral embryo and expansion of our knowledge of seed morphology and plant taxonomy/phylogeny since 1946, we hypothesize that the peripheral embryo does not belong at the base of Martin's family tree of seed phylogeny.
To address these hypotheses, the literature was reviewed to find answers to various questions: (1) What families of angiosperms have a peripheral embryo? (2) What traits do families with peripheral embryos have in common, and are any of these traits found in families that do not have a peripheral embryo? (3) What kind of ovule/seed development and cotyledon morphology occurs in taxa with peripheral embryos? (4) What is the phylogenetic position of families with peripheral embryos and of families that share traits with those known to have peripheral embryos?
Plant families with peripheral embryos
Martin (Reference Martin1946) illustrated a peripheral embryo in seeds of 11 families and listed them in the approximate order ‘of extent of expansion of their cotyledons’: Aizoaceae, Portulacaceae, Cactaceae, Caryophyllaceae, Chenopodiaceae, Frankeniaceae, Scleranthaceae, Amaranthaceae, Phytolaccaceae, Polygonaceae and Nyctaginaceae. All these families belong to the Caryophyllales (Takhtajan, Reference Takhtajan1997); thus, we need to investigate the characteristics of this order.
Caryophyllales
Plant families with free-central or basal placentation, mostly campylotropous ovules (i.e. curved embryos) and perisperm in the mature seed have long been regarded as a natural unit of classification (Bittrich, Reference Bittrich, Kubitzki, Rohwer and Bittrich1993). This group of families has been called the Curvembryae, Centrospermae and Caryophyllales (Harms, Reference Harms, Engler and Harms1934; Takhtajan, Reference Takhtajan1997). Harms (Reference Harms, Engler and Harms1934) listed 12 families in the Centrospermae: Achatocarpaceae, Aizoaceae, Amaranthaceae, Basellaceae, Caryophyllaceae, Chenopodiaceae, Dysphaniaceae, Gyrostemonaceae (now in Brassicales), Nyctaginaceae, Phytolaccaceae, Portulacaceae and Theligonaceae (now in Gentianales). As various kinds of research have been conducted, the list of families in the Centrospermae (now Caryophyllales) has been modified.
By the late 1980s, 12 families were included in the Caryophyllales: Achatocarpaceae, Aizoaceae, Amaranthaceae, Basellaceae, Cactaceae, Caryophyllaceae, Chenopodiaceae, Didiereaceae, Molluginaceae, Nyctaginaceae, Phytolaccaceae and Portulacaceae and about 10,000 species (Cronquist, Reference Cronquist1988). However, studies on the phylogenetic relationships of angiosperms using gene sequence data have provided much new insight on angiosperms in general (e.g. Downie et al., Reference Downie, Katz-Downie and Cho1997; Soltis et al., Reference Soltis, Soltis and Chase1999, Reference Soltis, Soltis, Chase, Mort, Albach, Zanis, Savolainen, Hahn, Hoot, Fay, Axtell, Swensen, Prince, Kress, Nixon and Farris2000; Hilu et al., Reference Hilu, Borsch, Müller, Soltis, Soltis, Savolainen, Chase, Powell, Alice, Evans, Sauquet, Neinhuis, Slotta, Rohwer, Campbell and Chatrou2003; Moore et al., Reference Moore, Soltis, Bell, Burleigh and Soltis2010) and on Caryophyllales in particular (Giannasi et al., Reference Giannasi, Zurawski, Learn and Clegg1992; Retting et al., Reference Retting, Wilson and Manhart1992; Morton et al., Reference Morton, Karol and Chase1997; Cuénoud et al., Reference Cuénoud, Savolainen, Chatrou, Powell, Grayer and Chase2002; Greenberg and Donoghue, Reference Greenberg and Donoghue2011; Yang et al., Reference Yang, Moore, Brockington, Soltis, Wong, Carpenter, Zhang, Chen, Yan, Xie, Sage, Covshoff, Hibberd, Nelson and Smith2015; Smith et al., Reference Smith, Brown, Yang, Bruenn, Drummond, Brockington, Walker, Last, Douglas and Moore2018). In some cases, the molecular studies have yielded some surprising results, e.g. Droseraceae belongs in a clade with Caryophyllidae and Nepenthaceae (Williams et al., Reference Williams, Albert and Chase1994). The number of families presently recognized in the Caryophyllales is 38 (APG IV, 2016). Hernández-Ledesma et al. (Reference Hernández-Ledesma, Berendsohn, Bosch, Mering, Akhani, Arias, Castañeda-Noa, Eggli, Eriksson, Flores-Olvera, Fuentes-Bazán, Kadereit, Klak, Korotkova, Nyffeler, Ocampo, Ochoterena, Oxelman, Rabeler, Sanchez, Schlumpberger and Uotila2015) recognized Amaranthaceae and Chenopodiaceae as separate families and thus have 39 families on their list, while APG IV (2016) combined these two families into Amaranthaceae, resulting in 38 families. The number of species in the Caryophyllales is about 12,500 (Hernández-Ledesma et al., Reference Hernández-Ledesma, Berendsohn, Bosch, Mering, Akhani, Arias, Castañeda-Noa, Eggli, Eriksson, Flores-Olvera, Fuentes-Bazán, Kadereit, Klak, Korotkova, Nyffeler, Ocampo, Ochoterena, Oxelman, Rabeler, Sanchez, Schlumpberger and Uotila2015).
According to placement of the Caryophyllales on the angiosperm phylogeny diagram (APG IV, 2016), the Caryophyllales along with Berberidopsidales and Santalales make up the Superasterid clade, which is sister to the Asterids. As such, the Caryophyllales are placed between the Malvids and Campanulids on the phylogenetic diagram.
Another result of the molecular phylogeny studies is that the Caryophyllales have been divided into two major clades: Caryophyllales I (core) and Caryophyllales II (non-core) (Cuénoud et al., Reference Cuénoud, Savolainen, Chatrou, Powell, Grayer and Chase2002; Hilu et al., Reference Hilu, Borsch, Müller, Soltis, Soltis, Savolainen, Chase, Powell, Alice, Evans, Sauquet, Neinhuis, Slotta, Rohwer, Campbell and Chatrou2003; Soltis et al., Reference Soltis, Soltis, Endress and Chase2005; Brockington et al., Reference Brockington, Alexandre, Ramdial, Moore, Crawley, Dhingra, Hilu, Soltis and Soltis2009; Endress, Reference Endress2010; Lee et al., Reference Lee, Kim, Park and Ali2013; Walker et al., Reference Walker, Yang, Feng, Timondea, Mikenas, Hutchison, Edwards, Wang, Ahluwalia, Olivieri, Walker-Hale, Majure, Puente, Kadereeit, Lauterbach, Eggli, Flores-Olvera, Ochoterena, Brockington, Moore and Smith2018). The core Caryophyllales consist of the families traditionally included in this order, along with the Agdestidaceae, Barbeuiaceae, Gisekiaceae, Halophytaceae, Lophiocarpaceae, Petiveriaceae, Sarcobataceae and Stegnospermataceae (Table 1). The noncore families include the Ancistrocladaceae, Droseraceae, Drosophyllaceae, Dioncophyllaceae, Frankeniace, Nepenthaceae, Plumbaginaceae, Polygonaceae and Tamaricaceae (Table 1).
Table 1. Families in the core and non-core Caryophyllales and information for each family for various seed and plant characters (P, protein; S, starch)

1. Martin, Reference Martin1946; 2. Baskin and Baskin, unpublished embryo database; 3. Eckardt, Reference Eckardt1976; 4. Mabberley, Reference Mabberley2008; 5. Shepherd et al., Reference Shepherd, Macfarlane and Colmer2005; 6. Johansen, Reference Johansen1950; 7. AP (Angiosperm Phylogeny) website; 8. Woodcock, Reference Woodcock1914; 9. Houk, Reference Houk1938; 10. Liao and Wu, Reference Liao and Wu2000; 11. Anderson, Reference Anderson1975; 12. Ayele et al., Reference Ayele, Magnus, Mihaljević, Prebeg, Čož-Rakovac, Ozga, Reinecke, Mander, Kamiya, Yamaguchi and Salopek-Sondi2010; 13. Grayum, Reference Grayum1991; 14. Iwashina, Reference Iwashina2013; 15. Brockington et al., Reference Brockington, Walker, Glover, Soltis and Soltis2011; 16. Brockington et al., Reference Brockington, Alexandre, Ramdial, Moore, Crawley, Dhingra, Hilu, Soltis and Soltis2009; 17. Watson and Dallwitz, Reference Watson and Dallwitz1992 onwards; 18. Thulin et al., Reference Thulin, Moore, El-Seedi, Larsson, Christin and Edwards2016; 19. Hartley and Harris, Reference Hartley and Harris1981; 20. Hakki, Reference Hakki2013; 21. Behnke, Reference Behnke1972; 22. Behnke et al., Reference Behnke, Chang, Eifert and Mabry1974; 23. Behnke, Reference Behnke1976; 24. Behnke, Reference Behnke1991; 25. Fay et al., Reference Fay, Cameron, Prance, Lledó and Chase1997
The Asteropeiaceae, Physenaceae, Rhabdodendraceae and Simmondsiaceae are included in the core Caryophyllales; however, the seeds of these families do not have a peripheral embryo and only scant endosperm and no perisperm, except for scant perisperm in Rhabdodendraceae (Table 1). According to Cuénoud et al. (Reference Cuénoud, Savolainen, Chatrou, Powell, Grayer and Chase2002), the Simmondsiaceae is sister to the core, and Rhabdondendraceae is sister to the noncore Caryophyllales. Carlquist (Reference Carlquist2010) followed Cuénoud et al., (Reference Cuénoud, Savolainen, Chatrou, Powell, Grayer and Chase2002), but Crawley and Hilu (Reference Crawley and Hilu2012) considered these three families to be sister to the core lineages. According to Brockington et al. (Reference Brockington, Alexandre, Ramdial, Moore, Crawley, Dhingra, Hilu, Soltis and Soltis2009) and Magallón et al. (Reference Magallón, Gómez-Acevedo, Sánchez-Reyes and Hernández-Hernández2015), Rhabdodendraceae and Simmondsiaceae are sister to the core, and Asteropeiaceae and Physenaceae form a clade that is sister to the rest of the core Caryophyllales. In studies of the one-seeded fruits of the core Caryophyllales, Sukhorukov et al. (Reference Sukhorukov, Mavrodiev, Struwig, Nilova, Dzhalilova, Balandin, Erst and Krinitsyna2015) found that the early-diverging lineages, i.e. Asteropeiaceae, Physenaceae, Rhabdodendraceae and Simmondsiaceae, have fruit and seed coats that were different from those of other Caryophyllales. Fruits of these four families have a thick pericarp that is divided into several distinct zones, and the seed coat also is divided into several layers.
Chemotaxonomy research on the Caryophyllales has revealed that members of the Aizoaceae, Amaranthaceae, Basellaceae, Cactaceae, Chenopodiaceae, Didiereaceae, Halophytaceae, Nyctaginaceae, Phytolaccaceae, Portulaceae and Stegnospermaceae have the pigment betalain, while the Caryophyllaceae and Molluginaceae have anthocyanin (Mabry et al., Reference Mabry, Taylor and Turner1963; Mabry, Reference Mabry1976, Reference Mabry1977; Mabry and Dreiding, Reference Mabry and Dreiding1969) (Table 1). Subsequent lineage-specific gene radiation studies have shown that betalain-specific isoforms in the CYP76AD1 and DODA lineages were lost or down-regulated in Caryophyllaceae and Molluginaceae (Brockington et al., Reference Brockington, Yang, Gandia-Herrero, Covshoff, Hibberd, Sage, Wong, Moore and Smith2015), resulting in production of anthocyanin instead of betalain. Betalains have been found only in the Caryophyllales (Mabry and Dreiding, Reference Mabry and Dreiding1969; Stafford, Reference Stafford1994; Brockington et al., Reference Brockington, Walker, Glover, Soltis and Soltis2011; Thulin et al., Reference Thulin, Moore, El-Seedi, Larsson, Christin and Edwards2016).
Another chemical compound of interest in our consideration of the Caryophyllales is ferulic acid, which is ester-linked to polysaccharides in the nonlignified (primary) cell wall. Ultraviolet fluorescence microscopy is used to examine primary cell walls, and if ferulic acid is present the cell walls autofluoresce blue at low pH and green at high pH (Harris and Hartley, Reference Harris and Hartley1980). Hartley and Harris (Reference Hartley and Harris1981) examined the primary cell wall of 251 species in 150 families and 46 orders of basal angiosperms and eudicots and found ferulic acid only in core Caryophyllales (Table 1). In the Commelinid monocots and core Caryophyllales, the concentration of ferulic acid is >3.5 mg (g cell wall)–1, but in eudicots such as Arabidopsis thaliana, Daucus carota, Medicago sativa and Populus trichocarpa the concentration is <3.5 mg (g cell wall)–1 (Harris and Trethewey, Reference Harris and Trethewey2010).
Some families in the Caryophyllales have a unique kind of sieve-element plastid in which there are ring-shaped bundles of filaments that contain protein; these plastids are called P-type plastids (Behnke, Reference Behnke1972). There is another basic kind of sieve-element plastid in which starch is accumulated, and it is called an S-type plastid (Behnke, Reference Behnke1972, Reference Behnke1976, Reference Behnke1991; Behnke et al., Reference Behnke, Chang, Eifert and Mabry1974). The non-core families in the Caryophyllales have S-type sieve-element plastids, and the core families have P-type, except the Asteropediaceae, Physenaceae and Simmondsiaceae, which have S-type (Table 1).
Peripheral embryo
Development
To understand the relatively long length of the peripheral embryo and its position in the seed, information about the ovule in which it develops is required. An ovule is a sac-like structure that contains the megasporangium in which the egg and eventually the embryo are produced. The oldest known ovules are those of Elkinsia polymorpha (Late Devonian, 365 ma) (Gillespie et al., Reference Gillespie, Rothwell and Scheckler1981; Rothwell et al., Reference Rothwell, Scheckler and Gillespie1989). The ovule of many orders of angiosperms has two integuments (bitegmic) and a relatively large amount of nucellus (crassinucellate) (Bouman, Reference Bouman and Johri1984; Endress Reference Endress2011a). In some angiosperms, e.g. the asterids, the ovule has one integument (unitegmic) and a thin nucellus (tenuinucellate), but ovules can be bitegmic and tenuinucellate, e.g. various families in the Rosiidae and Dilleniidae (Philipson, Reference Philipson1974). Furthermore, there is much diversity in crassinucellate and tenuinucellate ovules (Endress, Reference Endress2011a). The Caryophyllales have ovules that are bitegmic and crassinucellate (Eckardt, Reference Eckardt1976).
The amount of curvature of the ovule that has occurred by the time the embryo sac has formed varies greatly, and the most common kinds of ovules are orthotropous (atropous), anatropous, campylotropous, hemianatropous and amphitropous (Fahn, Reference Fahn1974; Endress, Reference Endress2011a); there are variations of these five common kinds of ovules. An orthotropous ovule is straight with the micropyle opposite the funiculus, while an anatropous ovule is bent so that the micropyle is adjacent to the funiculus. The anatropous ovule is the most common kind in extant angiosperms (Endress, Reference Endress2011a), and it is probably the most ancestral (Endress, Reference Endress2011b). The hemianatropous ovule is bent so that the micropyle is at a 90 degree angle in relation to the funiculus. In orthotropous, anatropous and hemianatropous ovules, the nucellus and embryo sac are straight (Endress, Reference Endress2011b). Campylotropous and amphitropous ovules are bent, and the micropyle is pointed toward the base of the funiculus. The campylotropous ovule has a bulge on the side, while the amphitropous ovule has a bulge on top. Thus, in campylotropous and amphitropous ovules the nucellus and embryo sac are curved, which results in a curved embryo (Rau, Reference Rau1940; Takaso and Bouman, Reference Takaso and Bouman1984). Furthermore, there is more space in campylotropous and amphitropous ovules for the embryo to elongate than in the other kinds of ovules. In campylotropous ovules, the embryo can be up to two times as long as the seed.
Taxonomic occurrence
With the exception of the Asteropeiaceae, Physenaceae, Rhabdodendraceae and Simmondsiaceae, the core families of the Caryophyllales have seeds with a peripheral embryo, while non-core families mostly do not have a peripheral embryo (Table 1). Seeds of Frankeniaceae and Polygonaceae (listed by Martin as having a peripheral embryo) are non-core, but they have endosperm (Takhtajan, Reference Takhtajan1997). The Frankeniaceae have a long narrow embryo (Martin, Reference Martin1946), and the Polygonaceae have embryos that are long and narrow, spatulate or folded in appearance (Stevens, Reference Stevens1912; Martin, Reference Martin1946). With the exception of Asteropeiaceae, Simmondsiaceae and Stegnospermataceae, the core families of Caryophyllales have campylotropous ovules (Table 1). All the non-core families have anatropous ovules except the Polygonaceae, which has orthotropous ovules.
To gain a better understanding of the relevance of ovule morphology in our consideration of the peripheral embryo, information on family occurrence of campylotropous and amphitropous ovules was obtained from Johansen (Reference Johansen1950) and Takhtajan (Reference Takhtajan1997) and plotted on the APG IV (2016) phylogenetic diagram (Fig. 2). We found campylotropous and/or amphitropous ovules in 77 families, including 26 families in the Caryophyllales. Campylotropous ovules are widely distributed phylogenetically, and their occurrence ranges from the Austrobaileyales (an ANA grade angiosperm) to the Boraginales (a Lamiid) (Fig. 2). The occurrence of amphitropous ovules ranges from the Fabales (a Fabid) to the Gentianales (a Lamiid). Thus, clearly ovules with the capacity to produce seeds with elongated embryos occur throughout the extant angiosperms.

Figure 2. Angiosperm phylogenetic diagram showing number of families with campylotropous (C) and amphitropous (A) ovules, perisperm (P) and linear-full embryos (LF) (modified from APG IV, 2016).
Perisperm
Development
A young angiosperm ovule consists of integuments, nucellus and female gametophyte. The cells of the nucellus represent the remnants of the megasporangium, and they surround the female gametophyte (embryo sac) and contain stored food that is used for embryo growth. In many plant species, as the ovule grows the amount of nucellus increases (Maheshwari and Chopra, Reference Maheshwari and Chopra1955; Kellman-Sopyła et al., Reference Kellmann-Sopyła, Koc, Górecki, Domaciuk and Giełwanowsak2017). After fertilization and formation of the embryo and endosperm, embryo growth occurs at the expense of the endosperm (Werker, Reference Werker1997; Burrieza et al., Reference Burrieza, López-Fernández and Maldonado2014), and the endosperm may transfer food from the nucellus to the embryo (Mohana Rao et al., Reference Mohana Rao, Guignard and Duret1988). Depending on the species, all the stored food in the nucellus may be consumed before or after fertilization or during germination (Mohana Rao et al., Reference Mohana Rao, Guignard and Duret1988; Werker, Reference Werker1997). Following fertilization, developing seeds of Chenopodium quinoa simultaneously accumulate (starch) and use food in the nucellus (López-Fernández and Maldonado, Reference López-Fernández and Maldonado2013). If any nucellar tissue remains after seed development is completed, it is called perisperm (Burrieza et al., Reference Burrieza, López-Fernández and Maldonado2014; Jiménez-Durán et al., Reference Jiménez-Durán, Arias-Montes, Cortés-Palomec and Márquez-Guzmán2014). Development of perisperm takes place in the ovule, and it helps to ensure a good supply of food and water for the developing seed (Wilms, Reference Wilms1980; Mohana Rao et al., Reference Mohana Rao, Guignard and Duret1988). As the perisperm in campylotropous ovules develops on the lower side of the embryo sac (above the chalaza), the embryo is pushed to the periphery of the ovule as the mass of the perisperm increases (Gibbs, Reference Gibbs1907; Buell, Reference Buell1952; Wilms, Reference Wilms1980; Mohana Rao et al., Reference Mohana Rao, Guignard and Duret1988; Zheng et al., Reference Zheng, Ma and Chai2010).
Taxonomic occurrence
Except for Asteropeiaceae, Physenaceae and Simmondsiaceae with scant endosperm and Rhabdodendraceae with scant endosperm and scant perisperm, seeds of families in the core Caryophyllales have copious starchy perisperm (Table 1). However, a small amount of endosperm may occur around the radicle end of the embryo in seeds of some core Caryophyllales, e.g. Amaranthaceae (Kajale, Reference Kajale1940, Reference Kajale1954; Pal et al., Reference Pal, Singh and Pal1990), Caryophyllaceae (Buell, Reference Buell1952; Wagner and Tengg, Reference Wagner and Tengg1993; Kellman-Sopyła et al., Reference Kellmann-Sopyła, Koc, Górecki, Domaciuk and Giełwanowsak2017) and Lophiocarpaceae (Hakki, Reference Hakki2013). Seeds of the non-core Caryophyllales families have endosperm, and those of Tamaricaceae have a thin layer of perisperm in addition to scant endosperm.
To gain a better understanding of the family occurrence of perisperm in seeds, we obtained information from Woodcock (Reference Woodcock1914), West et al. (Reference West, Flannigan and Lott1995), Takhtajan (Reference Takhtajan1997), Lu (Reference Lu1985), Goldberg (Reference Goldberg1986, Reference Goldberg1989) and Plakhine et al. (Reference Plakhine, Tadmor, Ziadne and Joel2012) and plotted it on the APG IV (2016) phylogenetic diagram (Fig. 2). Perisperm is widely distributed in the angiosperms and ranges from the Nymphaeales to Gentianales. However, the amount of perisperm varies from copious in seeds of Nymphaeales, Piperales, Zingeriberales and Caryophyllales (Goldberg, Reference Goldberg1986, Reference Goldberg1989) to a single layer of cells in Brassicales, Malvales and Lamiales (Werker, Reference Werker1997; Plakhine et al., Reference Plakhine, Tadmor, Ziadne and Joel2012). The only families, in addition to those in the Caryophyllales, with both campylotropous and/or amphitropous ovules and perisperm are the Marantaceae, Papaveraceae, Ranunculaceae, Rubiaceae and Simaroubaceae.
Embryo morphology/shape
Aside from the peripheral embryo being located adjacent to the seed coat, how can we describe/characterize it? For various other kinds of embryos in Martin's (Reference Martin1946) paper such as the bent, folded, investing, linear and spatulate, relative width of the radicle and cotyledons is a useful identification character. For all the drawings of members of the Caryophyllales in Martin's paper, we measured the width of the radicle and cotyledons and calculated the cotyledon (C):radicle (R) ratio. A C:R ratio of 1 represents a long, narrow embryo with the radicle and cotyledons having the same width, whereas a C:R ratio >1 means the cotyledons are wider than the radicle. Furthermore, we determined the C:R ratio for seeds with bent, folded, investing, full-sized linear and spatulate embryos illustrated in Martin's paper.
Mean C:R ratio for the Aizoaceae, Amaranthaceae, Cactaceae, Caryophyllaceae, Chenopodiaceae, Frankeniaceae, Nyctaginaceae, Phytolaccaceae and Portulaceae, which are in the core Caryophyllales, ranged from 1.0 to 1.82, and the mean C:R ratio for full-sized linear embryos was 1.06 (Table 2). As small linear embryos grow inside the seed prior to germination and full-sized linear embryos do not, we refer to them as linear-underdeveloped and linear-fully developed (linear-full), respectively (Baskin and Baskin, Reference Baskin and Baskin2007). All families included by Martin that belong to the core Caryophyllales have embryos that easily fit his full-sized linear embryo. All families in the core Caryophyllales, except Asteropeiaceae, Physenaceae, Rhabdodendraceae and Simmondsiaceae, have long thin linear or peripheral embryos (Table 1).
Table 2. Mean (mm ± s.d.) and range of cotyledon (C) width:radicle (R) width ratios calculated from measurements made on drawings of embryos in Martin's (Reference Martin1946) paper.

However, the mean C:R ratio for the Polygonaceae (2.56) most closely matches the mean C:R ratio (2.98) for spatulate embryos. Families in the non-core Caryophyllales have a diversity of embryo types, including peripheral [Polygonaceae and Frankeniaceae (Martin, Reference Martin1946)], linear-full, spatulate and investing, with spatulate being the most common (Table 1).
As the majority of Martin's families with a peripheral embryo have linear-full embryos, we queried our embryo database (Baskin and Baskin, unpublished) for other families with linear-full embryos. Linear-full embryos occur in 153 families, including 29 in the Caryophyllales, throughout the extant angiosperms from Laurales (Magnoliids) to Boraginales (Lamiids) (Fig. 2). In addition to the Caryophyllales, ≥5 families in the Alismatales, Asparagales, Brassicales, Ericales, Lamiales, Malpighiales, Saxifragales and Zingiberales have linear-full embryos. Linear-full embryos occur in one or more families in all orders with campylotropous and/or amphitropous ovules, except the Austrobaileyales, Dilleniales, Fabales, Saxifragales and Zygophyllales; however, some families in the Saxifragales have linear-underdeveloped embryos (Baskin and Baskin, unpublished embryo database).
On Martin's family tree of seed phylogeny, spatulate, bent, folded and investing embryos are placed above the linear-full (and in this order) (Fig. 1). Thus, in families with campylotropous and/or amphitropous ovules there is a strong association between linear-full and spatulate embryos, but linear-full also may occur with folded, investing and bent embryos. That is, the linear-full embryo is found in various families with campylotropous and/or amphitropous ovules along with the most advanced kinds of embryos (sensu Martin, Reference Martin1946).
Twelve kinds of embryos have been recorded in the 29 families with perisperm, with some families having as many as four kinds for a total of 46 embryo records. The importance of the kind of embryo in families with perisperm is bent (2.2%), broad (6.5%), capitate (8.7%), cupulate (4.3%), folded (4.3%), investing (13.0%), linear-full (19.6%), linear-underdeveloped (13.0%), rudimentary (4.3%), spatulate (17.4%), spatulate-underdeveloped (2.2%) and undifferentiated (organless) (4.3%).
Evaluation of hypotheses
Hypothesis 1: Seeds with a peripheral embryo have distinct morphological and developmental characteristics
With the exception of Asteropeiaceae, Physenaceae, Rhabdodendraceae and Simmondsiaceae, families in the core Caryophyllales have long narrow embryos that match the characteristics of Martin's linear-full embryos. These linear-full embryos develop in campylotropous and/or amphitropous ovules, and they encircle perisperm in the centre of the seed. This combination of characters (i.e. linear-full embryo, campylotropous and/or amphitropous ovules and perisperm) not only distinguishes the peripheral embryo but helps to characterize most of the core Caryophyllales. Individually, these three characters are widely distributed throughout the extant angiosperms, but they occur together in only seven orders (Alismatales, Gentianales, Lamiales, Malpighiales, Ranunculales, Sapindales and Zingiberales) in addition to the Caryophyllales (Fig. 2). However, in these orders (except for Caryophyllales), the three characters do not usually occur together in the same family. In addition to various families in the core Caryophyllales, this combination of characters is known to occur only in the Marantaceae and Rubiaceae. The Marantaceae have a linear-full embryo that is curved but located in the centre of the seed, and the Rubiaceae have folded, investing, linear-full, spatulate and spatulate-underdeveloped embryos (Martin, Reference Martin1946).
The combination of linear-full embryos, campylotropous and/or amphitropous ovules and perisperm supports our hypothesis that seeds with peripheral embryos have distinct characters. In addition, P-type sieve-elements, betalain pigment and ferulic acid in the primary cell wall are strongly associated with the core, but not the non-core, Caryophyllales (Table 1), further supporting Martin's (Reference Martin1946) contention that the peripheral embryo is unique among the angiosperms.
Hypothesis 2: The peripheral embryo does not belong at the base of Martin's family tree of seed phylogeny
We now have to ask the difficult question: where does the peripheral embryo belong on Martin's family tree of seed phylogeny? The answer lies in the embryo itself. We queried our embryo database (Baskin and Baskin, unpublished) and asked what kinds of embryos occur in the non-Caryophyllales families with campylotropous and/or amphitropous ovules. Among the 50 non-Caryophyllales families with campylotropous and/or amphitropous ovules, there are 11 kinds of embryos, with some families having as many as five kinds of embryos. In total, we have 116 embryo records for these 50 families: capitate (0.9%), bent (9.5%), folded (12.9%), investing (14.7%), linear-full (20.7%), lateral (0.9%), linear-underdeveloped (8.6%), rudimentary (6.0%), spatulate (22.4%), spatulate-underdeveloped (2.6%) and undifferentiated (0.9%). Fourteen of the families have linear-full as well as other kinds of embryos (31 records). In these 14 families, linear-full occurs with bent (9.7%), investing (16.1%), linear-underdeveloped (9.7%), spatulate (38.7%), spatulate-underdeveloped (3.2%) and undifferentiated (3.2%) embryos. In three families (Lecythidaceae, Ranunculaceae and Rutaceae), linear-full occurred with folded, investing and spatulate, and in the Myrtaceae it occurred with bent, folded, investing and spatulate. In the Rubiaceae, the linear-full embryo occurred with folded, investing, spatulate and spatulate-underdeveloped embryos. Thus, in the 14 families with linear-full as well as other kinds of embryos, there is clearly a strong association between the linear-full and spatulate embryos. Also, we ask what families have seeds with a linear-full embryo and perisperm? This combination of seed characters occurs only in seven monocot families (Asparagaceae, Araceae, Cannaceae, Marantaceae, Strelitziaceae, Tecophilaceae and Zingiberaceae) and three eudicot orders: Proteales (Proteaceae), Gentianales (Rubiaceae) and Caryophyllales (26 families; Table 1) for a total of 35 families. Thus, the combination of a linear-full embryo and perisperm is most common in the core families of the Caryophyllales.
Seeds of the core Caryophyllales with perisperm also have a linear-full (peripheral) embryo, except those of Rhabdodendraceae, which have scant perisperm, scant endosperm and an investing embryo (Table 1). In the non-core families, spatulate embryos and endosperm are the most common kind, but linear-full and endosperm are found in the Nepanthaceae, Frankeniaceae and Polygonaceae. Seeds of Tamaricaceae have seeds with spatulate embryos, scant perisperm and thin endosperm. Thus, seeds of the Caryophyllales may have endosperm and/or perisperm, and the embryo varies from linear-full to spatulate to investing.
The order Caryophyllales is very old. According to Magallón et al. (Reference Magallón, Gómez-Acevedo, Sánchez-Reyes and Hernández-Hernández2015), the median time of divergence of Berberidopsidales and Caryophyllales was 117.9 Ma, and the core and non-core Caryophyllales diverged 107.1 Ma. Within the non-core Caryophyllales, the clade that includes Frankeniaceae, Plumbaginaceae, Polygonaceae and Tamaricaceae diverged from the one that includes Ancistrocladaceae, Dioncophyllaceae, Droseraceae, Drosophyllaceae and Nepenthaceae 99.3 Ma. Within the core Caryophyllales, Rhabdodendraceae diverged 108.9 Ma and Asteropeiaceae/Physenaceae 96.2 Ma. Among the core Caryophyllales, the clade consisting of Achatocarpaceae, Amaranthaceae and Caryophyllaceae diverged 89.5 Ma; the clade consisting of Aizoaceae, Barbeuiaceae, Gisekiaceae, Lophiocarpaceae, Nyctaginaceae, Phytolaccaceae and Sarcobataceae 79.6 Ma; and the one consisting of Basellaceae, Cactaceae, Didieraceae, Halophytaceae, Montiaceae, Portulacaceae and Talinaceae 42.8 Ma (Magallón et al., Reference Magallón, Gómez-Acevedo, Sánchez-Reyes and Hernández-Hernández2015). Thus, the non-core families with endosperm and various kinds of embryos, including spatulate, investing and linear-full, and endosperm diverged from the other Caryophyllales 107.1 Ma, while the core families with peripheral embryos and perisperm did not diverge from the Asteropeiaceae with spiralled linear embryos and endosperm until 96.2 Ma. From 89.5 Ma, when Achatocarpaceae, Amaranthaceae and Caryophyllaceae diverged from the other Caryophyllales to 28.8 Ma, when Cactaceae and Portulacaceae diverged, 20 families of core Caryophyllales become distinct, and all have peripheral embryos and perisperm.
What we can surmise from this information about the age of divergence of Caryophyllales families is that those with peripheral embryos and perisperm are younger than those with endosperm and linear-full, spatulate or investing embryos. As the peripheral embryo is a linear-full embryo that is pushed to the side of the seed by the developing perisperm (Mohana Rao et al., Reference Mohana Rao, Guignard and Duret1988), we propose that the basal stock for the peripheral embryo had a linear-full embryo and endosperm. In seeds with a peripheral embryo, perisperm became the primary food-storage tissue, but the linear-full embryo is still present. Concomitantly with production of copious perisperm, it is conceivable that bending and bulging of the ovule may have occurred, i.e. formation of campylotropous and/or amphitropous ovules. According to Bouman (Reference Bouman and Johri1984), the long embryo in campylotropous ovules is a derived character, and the resulting seedling is large and highly developed when it emerges from the seed and thus has an increased possibility of survival. Following this line of thinking, it is worth noting that some species of Amaranthaceae (subfamilies Chenopodioideae and Salicornioidea) with long coiled embryos have very fast germination, i.e. seeds imbibe and germinate in <24 hours (Parsons, Reference Parsons2012; Liu et al., Reference Liu, Baskin, Baskin and Du2013; Parsons et al., Reference Parsons, Vandelook and Janssens2014). Nineteen of the 28 angiosperms reported to have very fast germination belong to the Caryophyllales, and 12 of them are in the Amaranthaceae. We suggest that the advantage gained by having seeds with highly developed embryos (some of which also had very fast germination) may have played a role in the high diversification of the core Caryophyllales and even in occupying extreme habitats such as saline areas and deserts.
For (an) unknown reason(s), perisperm is scattered throughout the extant angiosperms: Nymphalales, Magnoliids, monocots, Fabids, Malvids, Superasterids and Lamiids (Fig. 2), which suggests that it has become the main food-storage tissue in seeds at different times in various lineages. If perisperm developed in ancestral stock with linear-full embryos and endosperm, it might help explain the presence of endosperm around the radicle in various core families today. The presence of perisperm vs endosperm in seeds is of interest to theoretical ecologists who argue that 2n perisperm (2 maternal:0 paternal) gives the maternal parent complete control over resource distribution to the developing seeds. However, 3n endosperm (2 maternal:1 paternal) gives the paternal parent some control over resource allocation to the developing seeds (Westoby and Rice, Reference Westoby and Rice1982; Queller, Reference Queller1983; Haig and Westoby, Reference Haig and Westoby1989; Pires, Reference Pires2014; Povilus et al., Reference Povilus, Diggle and Friedman2018). We do not know what, if any, role a shift from endosperm to perisperm played in the evolution of the Caryophyllales. Nonetheless, after divergence of the lineage with perisperm much diversification occurred, as evidenced by the 25 families known today in the core Caryophyllales that have linear-full embryos and perisperm.
The embryo in the core Caryophyllales with perisperm is long, and both the cotyledons and radicle are narrow, resulting in a low C:R ratio. Interestingly, this embryo is very similar to the curved linear-full embryo in seeds of many species of Solanaceae that have endosperm (Martin, Reference Martin1946). The fact that the peripheral embryo is pushed to the side of the seed by the developing perisperm seems to have diverted attention away from the fact that it is a linear-full embryo. According to Martin (Reference Martin1946), the foliate embryo division (i.e. spatulate, bent, folded and investing) was derived from ‘…erect or nearly straight-embryoed Linear Dicots…’. Thus, we conclude that the peripheral embryo is unique and that it deserves recognition, i.e. a position on Martin's tree. The evidence we have collected reveals that the peripheral embryo is a curved linear-full embryo and as such should be placed on Martin's tree near his linear embryo. We propose that the peripheral embryo be depicted as a side branch (‘orphan’) from the right branch of Martin's tree and that it be located above the linear embryo and below the spatulate embryo (Fig. 1). Martin's peripheral embryo in the Frankeniaceae and Polygonaceae, both of which have endosperm, are best represented by his linear and/or spatulate embryos that are already on the tree.
Tribute
Martin's insight into the relationships between the kinds of embryos in seeds came from his analysis of morphological traits across many plant families and of many taxa in some of the families. To his great credit, Martin recognized that the families with peripheral embryos had a unique combination of characters, and thus he raised concerns about this kind of embryo. In light of all the seed- and phylogeny-related research conducted since 1946, the uniqueness of the families with peripheral embryos still holds today, i.e. a ‘phylogenetic orphan’. What we can add to Martin's puzzle about the peripheral embryo is that it is related to the linear-full embryo. However, without Martin's original concern about the peripheral embryo we might never have given this unique kind of embryo/seed the attention it deserves.
Acknowledgement
We thank Dr Matthew C. Perry and his colleagues Shannon Beliew, John French, Lynda Garrett and Jerry Longcore from the Patuxent Refuge for providing information about Dr A.C. Martin.