INTRODUCTION
Burial and dwelling mounds were the very first features subjected to incipient geoarcheological studies (Jefferson Reference Jefferson1783; Forchhammer et al. Reference Forchhammer, Steenstrup and Worsaae1851; Vanuxem Reference Vanuxem1843). Mounds are among the first studied objects of Hungarian archeology too (Rómer Reference Rómer1868a, Reference Rómer1868b, Reference Rómer1868c, Reference Rómer1878). Since the early period of archaeological excavations determination of the date of origin has been the most important aspect of mound research, as not only the type but also the time of construction displayed large-scale variance. The Hungarian term “kunhalom”Footnote 1 (Győrffy Reference Győrffy1821; Horváth Reference Horváth1825; Jerney Reference Jerney1851; Gyárfás Reference Gyárfás1870; Gárdonyi Reference Gárdonyi [Nagy]1893, 1914) is a catch-all category into which all types of mounds have been thrown without consideration to their function or date of origin (Makkay Reference Makkay1964; Tóth and Tóth Reference Tóth and Tóth2003; Tóth Reference Tóth2006; Barczi et al. Reference Barczi, Tóth, Tóth and Pető2009; Pető and Barczi Reference Pető and Barczi2011; Dani and Horváth Reference Dani and Horváth2012). This issue can only be tackled if the initial phase of each research includes stratigraphical and chronological analyses, so the very function and age of the mound are revealed (Sümegi et al. Reference Sümegi, Bede and Szilágyi2015a, Reference Sümegi, Náfrádi, Molnár and Sávia2015b). After setting up a stratigraphy using sedimentological, geochemical, and geophysical methods, a chronology is set up using absolute dates. A precise chronology can only be achieved by radiocarbon analyses (Gazdapusztai Reference Gazdapusztai1966–1967; Ecsedy Reference Ecsedy1979; Sümegi and Hertelendi Reference Sümegi and Hertelendi1998; Molnár et al. Reference Molnár, Joó, Barczi, Szántó, Futó, Palcsu and Rinyu2004; Gulyás et al. Reference Gulyás, Sümegi and Molnár2010; Molnár and Svingor Reference Molnár and Svingor2011; Dani and Horváth Reference Dani and Horváth2012). Additional information can be obtained with the OSL analyses (Liritzis et al. Reference Liritzis, Singhvi, Feathers, Wagner, Kadereit, Zacharias and Li2013) of wattle-and-daub fragments or pottery remains conserved in the layers. It is important to note though that pottery and wattle-and-daub fragments piled up with the soil can originate from the very same culture that created the mound itself, but also from earlier cultures. Thus, OSL analysis of the pottery and wattle-and-daub fragments may result in the incorrect conclusion that some layers are older than their actual age (Gazdapusztai Reference Gazdapusztai1966–1967). In addition, uncertainty of OSL measurements is too high compared to 14C analysis rendering them unsuitable for the construction of some hundred or a couple of thousand year-resolution chronologies for archeological periods of the younger Holocene. These skewed results can be rectified by mass radiocarbon or AMS dating on organic materials (Molnár et al. Reference Molnár, Joó, Barczi, Szántó, Futó, Palcsu and Rinyu2004, Reference Molnár, Rinyu, Veres, Seiler, Wacker and Synal2013; Barczi et al. Reference Barczi, Horváth, Pető and Dani2012; Dani and Horváth Reference Dani and Horváth2012) derived from the grave (wooden structure, bulrush shroud covering the corpse) or on the humus content of the piled-up soil.
In this paper results of a comprehensive stratigraphic work complemented by absolute chronology based on 14C AMS analyses on samples from the Ecse Mound (Sümegi Reference Sümegi2012) are discussed along with age-depth models built via Bayesian analysis using models that seem to be best suited for capturing the deposition rates characterizing mound formation.
Location and Characteristics of the Site
The Ecse Mound is in NE Hungary in the Hortobágy 12 km north-northeast to the town of Karcag (N47°25′31.11′′, E20°57′47.71′′) at a height of 93.5 m ASL. The mound is 5.5 m high with a length of 75.5 m and width of 67.5 m (Figure 1). The mound occupies a Pleistocene lag-surface wedging into the Holocene alluvia of the Hortobágy. It rises on the eastern end of a slightly elevated, elongated loess ridge that is clearly separable from its surroundings based on its vegetation and geomorphology. Traces of a ditch that was created when the earth was piled up on the mound are barely perceivable (Sümegi Reference Sümegi2012).

Figure 1 Location, geomorphology and observed stratigraphy of the Ecse Mound in the Hortobágy, NE Hungary.
Ecse Mound is mentioned (Gyárfás Reference Gyárfás1883; Benedek and Zádorné Reference Benedek and Zádorné1998) first in a charter describing village borders from 1521 (in the form “Echehalma”). In the Early Modern Era it was the border point between the villages of Asszonyszállás and Kápolnás. Today it lies on the administrative border between Karcag and Kunmadaras; the borderline breaks in an angle on the top of the mound.
Manuscript maps from the 18th–19th centuries and later printed maps consistently represent the whole area of the mound as pasture (Bede et al. Reference Bede, Csathó, Czukor and Sümegi2016). In the beginning of the 20th century, however, its southern half was plowed due to the increased demand for arable land, and in 1943, this is the picture presented. Socialist large-scale agriculture and the consequent large-scale landscape transformations did not spare the Ecse Mound, either: in the 1950s rice parcels were established on its southern side, traces of which are still visible. In the 1960s the area served again as pasture and has been used the same way until today (Bede et al. Reference Bede, Csathó, Czukor and Sümegi2016). In the wider vicinity of the mound farmsteads, dirt roads, ditches, embankments, grasslands and lower lying swamps can be found. The mound rises above its marshy, alkaline environment, thus most of its surface is covered by a loess grassland association (Salvio nemorosae-Festucetum rupicolae) and its derivatives. Arboreal vegetation is only sparsely present in the area. The mounds are characteristic refugia for the survival of such habitat types, having a significant conservation value, even the plant association itself (Joó Reference Joó2003; Illyés and Bölöni Reference Illyés and Bölöni2007; Horváth et al. Reference Horváth2011).
MATERIAL AND METHODS
Field Survey, Sampling
The first step of our work included the collection of historical and high-resolution regional maps of the area to create a digital elevation model of the surrounding landscape (Figure 1). This was followed by a field survey during which mapping data points have been recorded on the mound itself to provide an up-to-date DEM of the mound for further geomorphological studies. This was complemented by probe coring to reveal the spatial variability of the stratigraphy before actual sampling via undisturbed cores.
Sediment types were determined and described on the field using the Troels-Smith (Reference Troels-Smith1955) system internationally accredited for paleoecological works. Both wet and dry colors were determined (Munsell Reference Munsell2000).
Magnetic Susceptibility
Measuring magnetic susceptibility (MS) has proved to be one of the best methods to yield reliable stratigraphic data in case of studies of mounds (Sümegi Reference Sümegi2012, Reference Sümegi2013; Bede et al. Reference Bede, Csathó, Czukor, Páll, Szilágyi and Sümegi2014, Reference Bede, Salisbury, Csathó, Czukor, Páll, Szilágyi and Sümegi2015; Sümegi et al. Reference Sümegi, Bede and Szilágyi2015a). For this study samples were taken at 2–4-cm intervals. Prior to the start of the measurement, all samples were crushed in a glass mortar after weighing. Then samples were cased in plastic boxes and dried in air in an oven at 40°C for 24 hr. Afterwards, magnetic susceptibilities were measured at a frequency of 2 kHz using an MS2 Bartington magnetic susceptibility meter with a MS2E high resolution sensor (Dearing Reference Dearing1994). All the samples were measured three times and the average values of magnetic susceptibility were computed and reported.
Grain-Size Distribution and Loss on Ignition
The grain size composition of sedimentological samples was carried out using the laser-sedigraph method. First the samples were pretreated with 1 M HCl and H2O2 to remove CaCO3 and organic content respectively. A more detailed description of the pretreatment process is given by Konert and Vandenberghe (Reference Konert and Vandenberghe1997). All the samples were measured for 42 intervals between 0.0001 and 0.5 mm using an Easy Laser Particle Sizer 2.0 and Fritsch sieves in Szeged (Hungary). For LOI examination sub-samples were taken at every 2–4-cm intervals and the loss on ignition method was applied, commonly used for the analysis of the organic and carbonate content on calcareous sediments (Dean Reference Dean1974).
Radiocarbon Dating
Six shell samples were submitted for radiocarbon dating taken from major stratigraphic units as depicted in Table 1 and Figure 1. AMS 14C dating measurements were done in the internationally referenced AMS laboratory of Seattle, WA, USA (Table 1). The dead carbon effect was negligible in case of our chosen taxa because certain herbivorous gastropods are known to yield reliable ages for dating deposits of the past 40 ka with minimal measurement error on the scale of perhaps a couple of decades (Pigati et al. Reference Pigati, Quade, Shanahan and Haynes2004, Reference Pigati, Rech and Nekola2010, Reference Pigati, McGeehin, Muhs and Bettis2013; Sümegi and Újvári et al. Reference Újvári, Molnár, Novothny, Páll-Gergely, Kovács and Várhegyi2014). This uncertainty is preserved even after calibration yielding us dates on the sub-centennial scale. Considering the presently available multicentennial resolution of prehistoric archeochronology this level of uncertainty suited our needs.
Table 1 Conventional (year BP) 14C ages for Ecse Mound.

Preparation of the samples and the actual steps of the measurement followed the methods of Hertelendi et al. (1989, 1992) and Molnár et al. (Reference Molnár, Rinyu, Veres, Seiler, Wacker and Synal2013). Shells were ultrasonically washed and dried at room temperature. Surficial contaminations and carbonate coatings were removed by pretreatment with weak acid etching (2% HCl) before graphitization. Conventional radiocarbon ages were converted to calendar ages using the software OxCal 4.2 (Bronk Ramsey Reference Bronk Ramsey2009) and the most recent IntCal13 calibration curve (Reimer et al. Reference Reimer, Bard, Bayliss, Beck, Blackwell, Bronk Ramsey, Buck, Cheng, Edwards, Friedrich, Grootes, Guil- derson, Haflidason, Hajdas, Hatté, Heaton, Hoffmann, Hogg, Hughen, Kaiser, Kromer, Manning, Niu, Reimer, Richards, Scott, Southon, Staff, Turney and van der Plicht2013). Calibrated ages are reported as age ranges at the 2-sigma confidence level (95.4%). A U_Sequence age-depth model was constructed for the upper part of the sequence representing the actual mound via Bayesian modeling using OxCal (Bronk Ramsey Reference Bronk Ramsey2009). As these layers were artificially built up we may presume a relatively uniform deposition rate related to the events of mound formation. In our models we used a U_Sequence model assuming strictly uniform deposition for the anthropogenic stratigraphic horizons.
RESULTS
Lithology and Stratigraphy
The substrate sediment is comprised of fine sand and coarse-grained silt with substantial carbonate, low clay and organic content between 10 and 8 m. The sediment also contained tiny iron-manganese precipitation particles in the form of granules and coating. The overlying stratigraphic unit (8–7.8 m) is composed of very fine to fine sands with medium sand intercalations. This unit is overlain by yellowish brown calcareous clayey silt (7.8–5.8 m.) Between 5.8 and 4.15 m a meadow chernozem soil was encountered with well-developed A and B horizons. This is seen in a sudden increase in Corg values as well (Figure S1). This soil gave the base of the artificial mound. Start of the first man-made horizon was noted between the depths of 4.15 and 4.10 m also seen in elevated Fe content and magnetic susceptibility values (Figure S1). This horizon is overlain by another soil layer marking a different disturbance phase starting around 2.9–2.8 m. Start of this second soil layer is recorded in a drop of magnetic susceptibility and soluble Fe values and a peak in Corg (Figure S1).
The soil built up in the first two anthropological horizons is of polygonal structure with hydromorphic qualities that is very similar to the A and B layers of the underlying meadow chernozem soil. The organic content is significantly higher in this horizon (Figure S1), also showing an abrupt change of the soluble elements and insignificant level of carbonate content. However, the elemental and carbon concentrations must have changed considerably during the soil development process, and later due to ground water table fluctuations and precipitation percolating into the soil. Another disturbance layer was noted just below the modern topsoil (1.5 m) containing pottery as well as wattle and daub fragments. The last 1.5 m is the modern chernozem type topsoil. The sedimentological and geochemical properties of the topsoil overlying the artificial horizons is completely different from the artificially built (Figure S1). This is in line with earlier pedological and sedimentological observations made on other kurgans of the Hortobágy and Nagykunság (Sümegi Reference Sümegi1992; Barczi et al. Reference Barczi, Sümegi and Joó2003, Reference Barczi, Sümegi and Joó2004, Reference Barczi, Tóth, Csanádi, Sümegi and Czinkota2006; Joó et al. Reference Joó, Barczi and Sümegi2007; Sümegi and Szilágyi Reference Sümegi and Szilágyi2011; Szilágyi et al. Reference Szilágyi, Sümegi, Molnár and Sávia2013), that chernozem soil has developed on top of the artificial pile of kurgans. This type of top soil and related loess grassland association (Salvio nemorosae-Festucetum rupicolae) form the topmost layer of the kurgan.
Chronology
The AMS-based chronology assessment of the Ecse Mound was investigated from the bedrock to the first few artificially built-up horizons. According to the dates obtained, the bedrock of the base soil of the mound is dated to the Early Holocene (10,207–9877 cal BC). The upper part of the base soil of polyhedral structure was placed into the Late Neolithic as seen from radiocarbon dates received for a local steppe dwelling mollusk (4723–4558 cal BC) (Figure 2; Table 3: Phase 1). The next age corresponds to the first date at the boundary of the lower anthropological horizon (Figure 2; Tables 1–2). According to the modeled ages this boundary could be placed between 4446–4263 cal BC; i.e. Early Copper Age (Vaday Reference Vaday2004). Shell fragments of the aquatic bivalve Unio crassus found in the top boundary of the first artificially piled layer of terrestrial sediment suggest external, most probably human influence. 14C studies on freshwater shells from Neolithic mounds in SE Hungary indicated no significant dead carbon effect on obtained ages at the centennial scale (Gulyás et al. Reference Gulyás, Sümegi and Molnár2010). Modeled ages for this level representing the uppermost part of the first anthropological layer yielded ages 2922–2878 cal. BC (Figure 2; Table 2). As these two ages are bracketing the first anthropological horizon we may presume that the first anthropological horizon must have been built up during the Early Copper Age. The 2nd anthropological horizon clearly postdates the level marking the start of this horizon (Figure 2; Table 2). This this must have been built up during the latest phase of the Late Copper Age. Thus, based on our dates the mound must have been constructed by a local community of the Pit grave (Yamna) Culture (Gazdapusztai Reference Gazdapusztai1966/1967; Ecsedy Reference Ecsedy1979; Vaday Reference Vaday2004; Horváth et al. Reference Horváth, Dani, Pető, Pospieszny and Svingor2013) inhabiting the area between the late Copper Age–Bronze Age period.
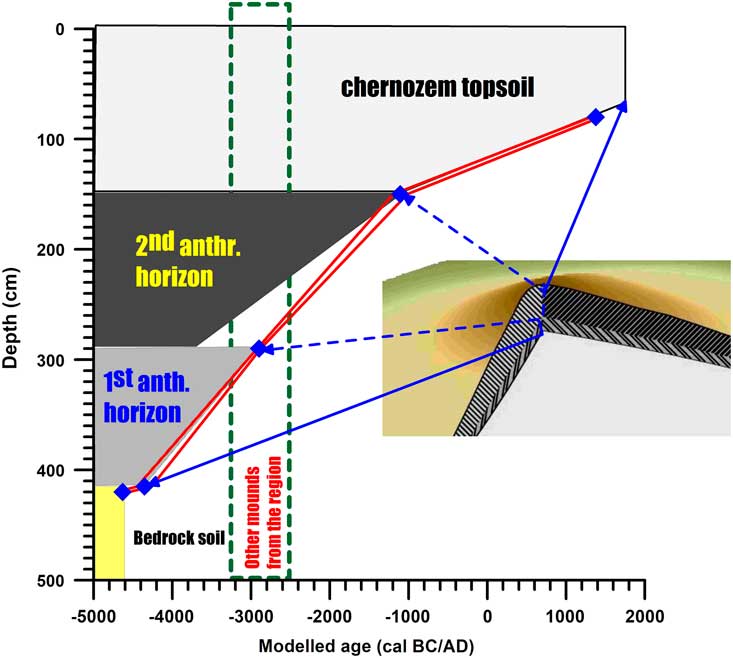
Figure 2 Constructed age-depth model for the mound.
Table 2 Modeled 14C ages (cal BC/AD) for the individual stratigraphic horizons at the 2σ (95.4%) confidence level.

DISCUSSION
Mounds were constructed in the Hortobágy and its wider region as early as 3300 BC (first appearance of the peoples of the Pit-Grave Culture or Pre-Pit Grave Culture) until as late as the 15th century, which is a 4900–5000-yr timespan.
There have been assumptions (Bede et al. Reference Bede, Csathó, Czukor, Páll, Szilágyi and Sümegi2014, Reference Bede, Salisbury, Csathó, Czukor, Páll, Szilágyi and Sümegi2015) before the current chronological study that the Ecse Mound had been built by communities of the Pit-grave (Yamna) culture (Merpert Reference Merpert1974; Gimbutas Reference Gimbutas1980; Rassamakin Reference Rassamakin1994) considering the similarities in shape, orientation and stratigraphy of this earth-pyramid, and comparative geomorphological research of other mounds in the Hortobágy region (Sümegi Reference Sümegi1992; Barczi et al. Reference Barczi, Sümegi and Joó2003, Reference Barczi, Sümegi and Joó2004, Reference Barczi, Tóth, Csanádi, Sümegi and Czinkota2006; Joó et al. Reference Joó, Barczi and Sümegi2007; Sümegi and Szilágyi Reference Sümegi and Szilágyi2011; Szilágyi et al. Reference Szilágyi, Sümegi, Molnár and Sávia2013). According to our investigations the first phase of mound construction could be placed to the Early Copper Age, while the second part dates to the the Late Copper Age, i.e. the time of first infiltration of the Yamna (Pit Grave or Ochre Grave) Culture. It is interesting to note that 14C ages from surrounding mounds covering a period of the Pre-Pit, Early Pit, and Classical Pit Cultures, clearly overlap with our ages obtained for the base of the second anthropogenic unit of Ecse Mound (Table 3; Figure 2).
Table 3 Conventional (year BP) and calibrated (cal BC/AD) 14C ages from different kurgans around Ecse Mound in the Great Hungarian Plain (Gazdapusztai Reference Gazdapusztai1966–1967; Ecsed 1973, Reference Ecsedy1979; Dani and Nepper 2006; Horváth et al. 2008, Reference Horváth, Dani, Pető, Pospieszny and Svingor2013; Dani and Horváth Reference Dani and Horváth2012).

CONCLUSION
This paper can be considered as a pilot research to a much larger scale scientific program with the objective of studying kurgans by the means of undisturbed core sampling. By publishing these preliminary data, we also wanted to draw attention to the need of concentrated and focused research efforts, and using standardized methodology in kurgan research, so the results from different researches done by different research groups are consistent and comparable. As of today, comparative studies are virtually impossible not only due to the different drilling and sampling techniques, but also for the lack of standardized methodology in fine stratigraphy and common understanding of geology, paleoecology, and geoarchaeology.
ACKNOWLEDGMENTS
The authors would like to thank to Balázs Pál Sümegi and Tünde Törőcsik for assisting in the sedimentological and magnetic susceptibility measurements, as well as to Dr Dávid Gergely Pál for assisting in the geochemical measurements. Special thanks should be passed to the Hortobágy National Park Directorate for the financial support provided.
Supplementary material
To view supplementary material for this article, please visit https://doi.org/10.1017/RDC.2018.107