INTRODUCTION
Geological heritage, relative sea-level variations, and climatic changes determine topography, coastal orientation, sediment production and availability, and accommodation space, as well as wind, wave, and coastal currents (Murray-Wallace and Woodroffe Reference Murray-Wallace and Woodroffe2014; Khan et al. Reference Khan, Ashe, Shaw, Vacchi, Walker, Peltier, Kopp and Horton2015). The balance among these factors establishes the evolution of coastal systems. In densely urbanized coastal areas, human interventions interfere with the landscape evolution by altering the sedimentation conditions from the source to the depositional environments. In beach systems, permanent buildings and engineering works prevent the natural mobility of the sediments, which intensifies erosion, making it difficult to understand the past evolution and the current coastal processes.
The area of this study is situated at Barra da Tijuca, a highly urbanized location at the eastern end of Jacarepaguá coastal plain, in the city of Rio de Janeiro, southeastern Brazil (Figure 1). The coastal plain of Jacarepaguá has approximately 140 km2, a coastline of 20 km, and exhibits a lagoon complex composed of Jacarepaguá, Tijuca, Camorim, and Marapendi lagoons (Figure 1). The studied site was a building construction site approximately 700 m from the current coastline and 200 m from the Joatinga channel, which connects the Tijuca lagoon with the sea.

Figure 1 Jacarepaguá coastal plain, with the location of the study site (at Barra da Tijuca), indicated in the insert, at right-hand below, by the circle B1 (drilling) and the hatched polygon (excavated area for the construction of the commercial building). Hillshade terrain from SRTM/USGS/TOPODATA (Valeriano Reference Valeriano2008). Ortho-photo from IBGE (the Brazilian Institute of Geography and Statistics).
By acknowledging prior studies developed in the region and at the Australian coast, the objective of the present work is to contribute to the knowledge of the evolution of the wave-dominated barrier-lagoon systems associated with the relative sea-level variations. Analyses were performed on sediment samples collected after a percussion drilling in the area next to the construction site.
Previous geotechnical studies for the construction indicated that the groundwater level was approximately 2.7 m below the surface. To permit excavation to a 10-m depth for foundation construction, it was essential to build an underground containment wall around the entire area of 2500 m2 and drain it using hydraulic pumps before executing the excavation. This prevented the analysis of stratigraphy in profile, but traces of a 30-cm-thick muddy sediment horizon remained in place attached to the wall, at –6.3 m. Moreover, at the bottom of the excavation (at –10 m), a sandy-quartz deposit was exposed with a great abundance of in situ mollusk shells and echinoderm specimens of “sand dollars”. It was possible to recover a 7.84-m-long sediment core by percussion drilling. Three samples were dated by 14C AMS. The core stratigraphy was analyzed for organic matter content (OM), calcium carbonate content (CaCO3), and the physical characteristics of the sediment. Mollusk shells and echinoderm skeletons collected were also taxonomically identified.
BACKGROUND
Continental rifting and normal faulting (from Jurassic to Paleogene) controlled the development of Rio de Janeiro bedrock into a mountain range, coastal massifs and semi-grabens, all parallel to the coastline (Zalán and Oliveira Reference Zalán and Oliveira2005). This tectonic process was responsible for the settlement of the WSW-ENE regional drainage system that does not flow into the study area, but a few kilometers to the interior. According to Dias and Kjerfve (Reference Dias, Kjerfve, Dillenburg and Hesp2009), the Jacarepaguá coastal plain is part of the geomorphologic unit classified as “the low-lying Fluminense sedimentary plain”, characterized by the presence of several coastal lagoons. The river discharge and sediment input directly from the lagoons to the ocean are relatively low.
In the wave-dominated, microtidal Rio de Janeiro central coast, double transgressive barriers separate lagoons from the Atlantic Ocean (Dias and Kjerfve Reference Dias, Kjerfve, Dillenburg and Hesp2009). According to Turcq et al. (Reference Turcq, Martin, Flexor, Suguio and Tasayaco-Ortega1999), the source of sand that formed the barriers, during the Upper Pleistocene and Holocene, was the shallow and low gradient continental shelf (maximum 0.2º, according to Reis et al. Reference Reis, Maia, Silva, Rabineau, Guerra, Gorini, Ayres, Arantes-Oliveira, Benabdellouahed, Simões and Tardin2013; Dias et al. Reference Dias, Dias, Ternes, Dias, Bastos and Vital2019). In the Jacarepaguá coastal plain, the inner barrier, which isolates the lagoons of Camorim, Jacarepaguá, and Tijuca, is 17.5 km long, 950 m wide to the west and 150 m wide to the east, 9.5 m high to the west, 11.5 m to the center and 8.5 m to the east. The outer barrier, which isolates the lagoon of Marapendi, is 18 km long, 300 m wide to the west, 20 m wide to the east, and its height varies from 4.5 to 6.5 m (Dias and Kjerfve Reference Dias, Kjerfve, Dillenburg and Hesp2009).
Roncarati and Neves (Reference Roncarati and Neves1976) presented a seminal model of the evolution of the Jacarepaguá coastal plain based on stratigraphy and geomorphology and assumed that the double transgressive barriers developed during the Holocene. They followed the hypothesis of Lamego (Reference Lamego1945), based on Gilbert (Reference Gilbert1885), and argued that the lagoons would have been isolated by sand spits that migrated longitudinally at the coast by longshore currents transport, forming elongated barriers. Muehe and Correa (Reference Muehe and Corrêa1989) opposed this model and agreed with Hoyt (Reference Hoyt1967), Schwartz (Reference Schwartz1971), Field and Duane (Reference Field and Duane1976), stating that the prevalence of frontal waves leads to only moderate longshore currents, and the formation of lagoons on the back-barrier might be predominantly a consequence of landward beach migration caused by marine transgression.
Maia et al. (Reference Maia, Martin, Flexor and Azevedo1984) suggested that the double barriers are transgressive features. However, based on radiocarbon (14C) dating of surface lagoon samples and the oscillating Holocene relative sea-level curve developed by Martin et al. (Reference Martin, Suguio and Flexor1979), they proposed that the inner barrier formed between 7000 and 5100 BP and the outer barrier between 3800 and 3500 BP. These sea-level oscillations were later refuted by Angulo and Lessa (Reference Angulo and Lessa1997), Angulo et al. (Reference Angulo, Lessa and Souza2006), and Angulo et al. (Reference Angulo, Giannini, De Souza and Lessa2016), showing that from 4400 BP the sea-level was lowered to the present mean sea-level (PMSL) without the significant oscillations showed by Martin et al. (Reference Martin, Suguio and Flexor1979).
The model proposed by Turcq et al. (Reference Turcq, Martin, Flexor, Suguio and Tasayaco-Ortega1999) is considered, in the present research, the most applicable to the current concepts and scientific knowledge, regarding the relative variations of sea-level during the Holocene for the southeastern Brazilian coast. Turcq et al. (Reference Turcq, Martin, Flexor, Suguio and Tasayaco-Ortega1999) recognized that the inner barrier formed in the Upper Pleistocene as a result of the penultimate transgression of around 123,000 BP (based on analogy analysis of Th/U dating of corals found underlying a beach terrace at the eastern Brazilian coast by Martin et al. Reference Martin, Bittencourt and Vilas-Boas1982). After carrying out 14C dating, Turcq et al. (Reference Turcq, Martin, Flexor, Suguio and Tasayaco-Ortega1999) showed that the outer barrier-lagoon system began to form in the Mid-Holocene, as a result of the most recent post-glacial marine transgression (PMT), between 7000 and 5000 BP. In the same direction, relied on diatoms analysis and 14C dating from Itaipu and Ponta Negra lagoons, less than 70 km east of Barra da Tijuca (see Figure 1), Ireland (Reference Ireland1987) had already concluded that the inner barrier-lagoon systems were formed in the Pleistocene and the outer was formed after about 7150 BP. These allowed the development of semi-enclosed coastal lagoons, connected to the sea through a single channel (Joatinga channel, at Barra da Tijuca), typical wave-dominated environments.
Muehe and Lins-de-Barros (Reference Muehe, Lins-de-Barros, Short and Klein2016) pointed out that during the penultimate transgression, the climate was similar to the current one and the sea-level reached between approximately +7 and +8 m (above PMSL), at the Rio de Janeiro state coast. According to Angulo et al. (Reference Angulo, Lessa and Souza2006), Muehe and Lins-de-Barros (Reference Muehe, Lins-de-Barros, Short and Klein2016), and Angulo et al. (Reference Angulo, Giannini, De Souza and Lessa2016), the most recent PMT surpassed the PMSL on the coast of Rio de Janeiro state ca. 7000 BP and reached the elevation between +3 to +4 m, within 5800 and 5000 BP. In the last 4400 cal BP the relative sea-level has dropped smoothly to the PMSL (Angulo et al. Reference Angulo, Giannini, De Souza and Lessa2016).
The sea-level variation in the Rio de Janeiro coast during the Upper Pleistocene and Mid-Holocene is similar to the observed at the eastern and northeastern Australian coast, also on a relatively tectonically stable continental margin (Sloss et al. Reference Sloss, Nothdurft, Hue, O’Connor, Moss, Rosendahl, Petherick, Nanson, McKenzie, Sternes, Jacobsen and Ulm2018). The geomorphological evolution of double transgressive barriers separated by lagoons is similarly described by Roy and Thom (Reference Roy and Thom1981) on the northern coast of New South Wales (NSW), Australia. The wave-dominated transgressive double barriers cut by the Joatinga channel also correspond to the barrier estuary described by Sloss et al. (Reference Sloss, Jones and Murray-Wallace2005) at Lake Illawarra (NSW), even though in that case the Pleistocene inner barrier was not preserved.
MATERIALS AND METHODS
Percussion drilling was performed in the area outside the building excavation at the coordinates 43°18′18.4"W/23°0′31.7"S (see Figure 1, insert), using a Rammkernsonden sampler (RKS). The position of the borehole and the terrain altimetry were obtained using a GNSS receiver (TechGeo GTR-G2), set to WGS 1984 datum. The altitude data were post-processed and corrected concerning the PMSL with the GTR Processor software and data from the Brazilian Network for Continuous Monitoring of GNSS (RBMC), provided by IBGE (Brazilian Institute of Geography and Statistics). The RBMC supports the Brazilian Geodetic System and defines the geodetic height at different locations considering a geoid model (MAPGEO2015), referenced to the Brazilian Vertical Datum (Imbituba) to determine the local PMSL (Fortes et al. Reference Fortes, Costa, Abreu, Silva, Júnior, Monico, Santos, Tétreault and Sideris2009; IBGE 2010).
The recovered sections were photographed and visually described in the field before being sub-sampled. At the Laboratory of Sedimentology of Universidade Federal Fluminense, the steps for the analysis of the samples were: (i) lyophilization; (ii) quantification of OM by loss-on-ignition at 410°C for 16 hr, according to Schumacher (Reference Schumacher2002); (iii) quantification of CaCO3 by weight difference after dissolution in hydrochloric acid (10% HCl), according to Gross (Reference Gross and Carver1971); (iv) grain size analysis and morphometry of sand samples with CAMSIZER particle analyzer (Retsch Technology) and mud samples with Mastersizer 2000 Hydro G (Malvern Instruments).
The CAMSIZER analyzer is convenient to characterize dry, free-flowing materials, by simultaneously measuring particle size and shape using dynamic image analysis. It optically measures grain sizes ranging from 20 µm (very coarse silt) to 30 mm (coarse gravel) using two CCD (charge-coupled device) cameras with 30 fps (60 images/s) and 1.3 Mpixel each, and a LED strobe light source. The Mastersizer 2000 Hydro G measures particles in suspension by laser diffraction, from clay (< 2 µm) to coarse sand (1 mm).
The distribution of grain size and sorting were obtained through the software Gradistat 8.0 (Blott and Pye Reference Blott and Pye2001), which bases its statistics on Folk and Ward (Reference Folk and Ward1957). Sphericity and roundness of the sandy samples and the sandy fraction contained in the finer sediment samples measured by CAMSIZER (based on Krumbein Reference Krumbein1941) were evaluated according to the standards published in Blott and Pye (Reference Blott and Pye2008).
Calcareous shells of mollusks were manually removed from the drilling sampler using sterilized tweezers and along with samples collected from the excavation site were taxonomically identified to support paleoenvironmental reconstruction. The scientific nomenclature has been updated according to the definitions of the WoRMS Editorial Board (2018). The shells and animal remains were photographed, and three samples were picked for dating by 14C AMS. The material was prepared at the Radiocarbon Laboratory of Universidade Federal Fluminense (LAC-UFF) (Macario et al. Reference Macario, Gomes, Anjos, Carvalho, Linares, Alves, Oliveira, Castro, Chanca and Silveira2013) and sent for measurement by accelerator mass spectrometry (AMS) to CAIS (Center for Applied Isotope Studies), University of Georgia, USA (Cherkinsky et al. Reference Cherkinsky, Culp, Dvoracek and Noakes2010). For dating, the most intact samples were chosen to reflect the death of the organism in situ, ensuring that there was no considerable reworking. The sub-sampling procedure and pre-treatment were performed according to Macario et al. (Reference Macario, Souza, Trindade, Decco, Lima, Aguilera, Alves, Oliveira, Chanca, Carvalho, Anjos, Pamplona and Silva2014). Sample preparation started with separation of ca. 30 mg of carbonate from individual shells and followed acid etching with 0.1M HCl until removal of 50% in mass. Samples were converted to carbon dioxide by acid hydrolysis by injecting 85% H3PO4 in evacuated vials. Gas samples were purified using cryogenic traps to remove water (dry ice/ethanol mixture) and segregate pure carbon dioxide, using liquid nitrogen. After being transferred and frozen into Pyrex tubes, these were individually torch sealed and heated in a muffle oven at 550°C. Graphite was formed over iron powder using the Zn/TiH2 method (Xu et al. Reference Xu, Trumbore, Zheng, Southon, McDuffee, Luttgen and Liu2007; Macario et al. Reference Macario, Oliveira, Moreira, Alves, Carvalho, Jou, Oliveira, Pereira, Hammerschlag and Netto2017).
The calibration of results was done using OxCal v4.3.1 (Bronk Ramsey Reference Bronk Ramsey2017), with the Marine13 curve (Reimer et al. Reference Reimer, Bard, Bayliss, Beck, Blackwell, Bronk Ramsey, Buck, Cheng, Edwards and Friedrich2013), and taking into account the closest available local reservoir offset ΔR = 32 ± 44 14C yr (Alves et al. Reference Alves, Macario, Souza, Pimenta, Douka, Oliveira, Chanca and Angulo2015), and 2 σ of error (95.4% reliability). Results are presented as calibrated age intervals. Previous published 14C ages have also been analyzed (Ireland Reference Ireland1987; Mansur et al. Reference Mansur, Ramos, Godoy and Nascimento2011; Silva et al. Reference Silva, Silva, Souza and Pinto2014; Angulo et al. Reference Angulo, Giannini, De Souza and Lessa2016; Jesus et al. Reference Jesus, Dias, Muniz, Macario, Seoane, Quattrociocchi, Cassab, Aguilera, Souza and Alves2017). For the discussion, all 14C ages consulted in the literature had the calibration updated, except when the conventional ages were not published in the source.
RESULTS
The terrain altitude concerning the PMSL was measured at +1.953 m (±1.4 cm). A 7.84-m-long sediment core was recovered, but some intervals were lost during drilling (Figure 2). The quartzose sediment of the bottom of the excavation site (at –9 m) presented moderately well-sorted gravelly coarse sand with unimodal distribution, rounded grains, and high sphericity. The sediment taken from the base of the core, from –7.84 m up to –7.2 m, showed the predominance of moderately sorted fine sand, with unimodal distribution, sub-rounded to rounded, and high sphericity (Figure 2).

Figure 2 Profile of the core collected, with grain size distribution, organic matter (OM) and calcium carbonate (CaCO3) contents, sorting, and shape parameters of sand fraction. On the left, the 14C ages obtained are shown in the positions indicated by arrows (see Table 1). The data of the base at –9 m correspond to the sample collected at the bottom of the excavation site.
Two muddy layers were observed from –7.2 to –6.3 m interspersed with narrow fine sand intervals. The lower mud layer, at –7.2 m, is moderately sorted and contains shell fragments, while the upper mud layer, at –6.3 m, is very poorly sorted and contains plant traces. The lower layer has a smaller proportion of fine sediments in relation to sand and smaller content of OM and CaCO3 (Figure 2). At –7.2 m, the muddy fraction is divided, on average, into 2.5% of silt and 0.5% of clay. At –6.3 m, this ratio is 26.3% of silt and 1.4% of clay. The sand roundness of the two layers does not differ considerably, being sub-rounded to angular. Even though both layers present moderate sand grain sphericity, the upper layer presents notable less spherical grains.
Above –6.3 m to the core top (at –1.4 m), the grain size becomes medium to coarse sand, with unimodal distribution. Between –6 and –3 m, the sandy layers presented the lowest OM and CaCO3 content and the absence of stratification, plant traces, and shell fragments. Within this range, sands are coarser between –5.2 and –4.7 m, varying from medium to coarse. Between –6.15 and –3.8 m, the sands are well sorted, sub-rounded to rounded, and with high sphericity (Figure 2). From –1.2 m to the surface, a landfill and debris layer was present.
A fragment of bivalve shell from a sandy sample at –6.6 m was dated at 4969 ± 29 BP (5440–5070 cal BP). Another shell sample from –2.1 m was dated at 6552 ± 28 BP (7170–6880 cal BP), indicating an age inversion (Figure 2 and Table 1). In some sectors untouched by backhoes at the base of the excavation, there were concentrations of echinoderms Encope emarginata (sand dollars) in living position (Figure 3). Nine specimens of Encope emarginata were collected between –10 and –9 m. A skeleton sample collected at –9 m was dated at 7205 ± 28 BP (7770–7540 cal BP) (Table 1). Regarding the inconsistency of age inversion, it is considered that the dating of the echinoderm is reliable, due to the grouped skeletons found in living position. The same is attributed to the bivalve sample at –6.6 m due to the lateral continuity observed between the sediment core and the excavation site. The age obtained from the sample at –2.1 m is the only doubtful result.
Table 1 14C ages of three samples from the study area. The first two were picked from the core and the third was obtained from an organism in living position (echinoderm) taken from the bottom of the excavation.


Figure 3 (a) and (b): Skeletons of Encope emarginata found in situ in living position; (c), (d), (e), and (f): Some of the specimens collected.
In the excavation site, many well-preserved shells indicated that there was no intense reworking of the sediments, although articulated pairs of bivalve shells in living position were not found. Muddy sediments left traces of a dark grey layer adhered to the surface of the retaining wall at 6.3 m deep (Figure 4a), the same depth as the mud layers identified at the core. The abundance of mollusk shells was remarkable, predominantly between –10 and –9 m (Figures 4b and 4c). Twenty-two taxa of Bivalvia and Gastropoda classes (Table 2 and Figure 5) were identified, distinctive of the marine environment, basically of the beach, but with rocky shore contributors (gastropods).

Figure 4 (a) Quartzose sand intercalated by a dark muddy layer (indicated by red arrows) that left traces in place on the surface of the retaining wall, at 6.3 m depth underground. (b) and (c) Shells found on the excavation site between –10 and –9 m. Photographs kindly provided by engineer Roberto Almeida.
Table 2 List of taxonomic identification of shells (see Figure 5).


Figure 5 Photographs of mollusk shells collected (identified in Table 2). The photographs of the bivalves show the external and internal faces of the same valve.
DISCUSSION
Correlations with Holocene Paleo Sea-Levels on the Coast of Rio de Janeiro State
The benthic community of Encope emarginata found in living position between –9 and –10 m represents an indication of a pre-existing marine surface. Considering the 14C age of 7770–7540 cal BP, it is necessary to infer the vertical position of these organisms to the paleo sea-level and consequently verify the correspondent paleoenvironment. For that, the Holocene sea-level oscillation on the coast of Rio de Janeiro state is described below, as retrieved from recently published literature.
To establish when the relative sea-level during the last post-glacial marine transgression (PMT) crossed the present mean sea-level (PMSL) on the coast of Rio de Janeiro state, Angulo et al. (Reference Angulo, Giannini, De Souza and Lessa2016) considered the 14C ages published by Mansur et al. (Reference Mansur, Ramos, Godoy and Nascimento2011) in Jaconé, an area located only 70 km to the east of the study area (Figure 1). The ages presented were 9190 ± 30 BP and 7410 ± 30 BP, the first relative to shell samples and the second relative to carbonate cement of a mapped beach rock in the region. These ages were reported as calibrated respectively as 8198–7827 cal BP and 6008–5786 cal BP, but even though these calibrations were expressed in cal BP, the values correspond in fact to AD/BC years. In the present study, after recalculating the calibrations, the results found were 10,134–9874 cal BP and 7945–7795 cal BP.
Regarding the beach rocks as indicators of paleo sea-levels, Angulo et al. (Reference Angulo, Giannini, De Souza and Lessa2016) pointed out that the shells that compose them may be older than the rock formation and thus the dating can indicate only a maximum age. For this reason, the dating of the carbonate cement obtained by Mansur et al. (Reference Mansur, Ramos, Godoy and Nascimento2011) is more reliable to compute the age of the beach rock formation itself and therefore designate a more consistent paleo sea-level. So here we used the recalibrated carbonate cement age in our sea-level curve (Figure 6).
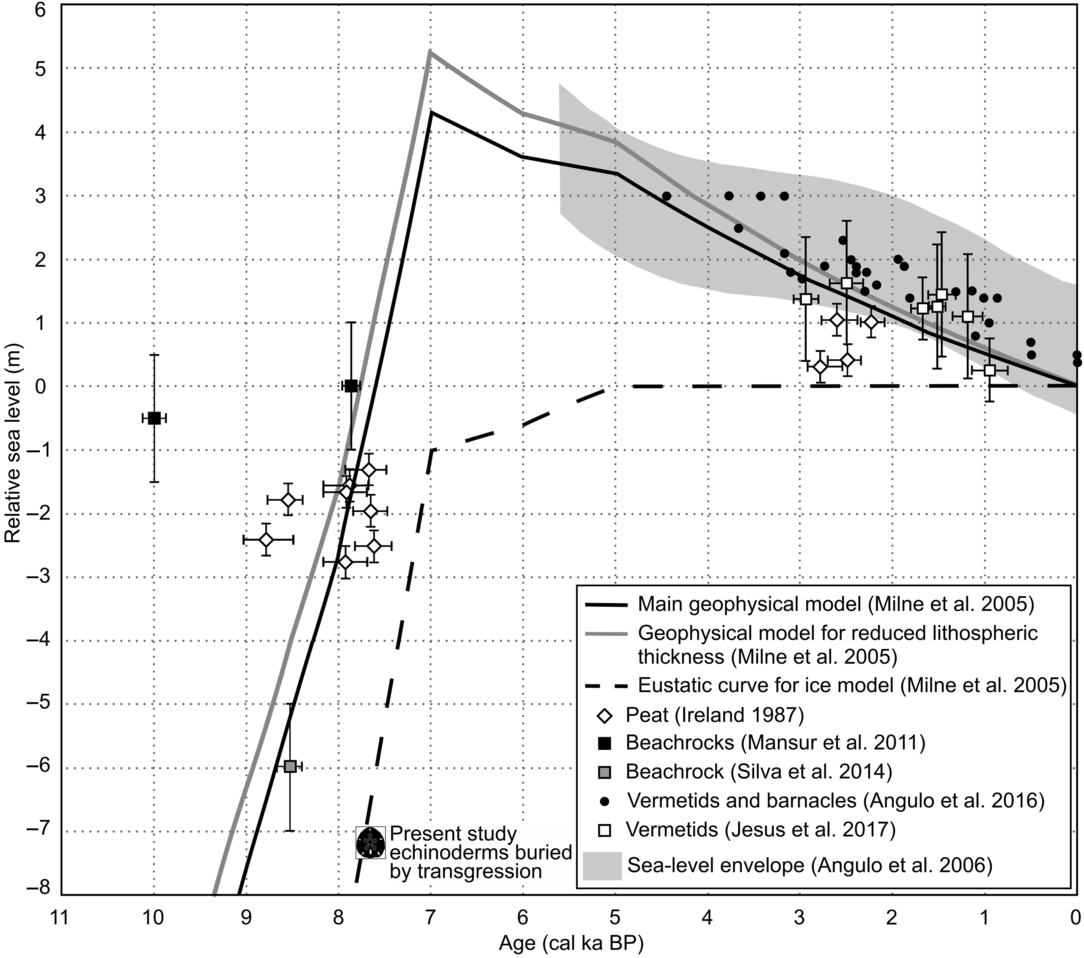
Figure 6 Revised variation curves of relative sea-level and paleo sea-level indicators exclusively for the coast of Rio de Janeiro state in the last 10,000 cal BP, compiled from Ireland (Reference Ireland1987), Milne et al. (Reference Milne, Long and Basset2005), Mansur et al. (Reference Mansur, Ramos, Godoy and Nascimento2011), Silva et al. (Reference Silva, Silva, Souza and Pinto2014), Angulo et al. (Reference Angulo, Giannini, De Souza and Lessa2016), and Jesus et al. (Reference Jesus, Dias, Muniz, Macario, Seoane, Quattrociocchi, Cassab, Aguilera, Souza and Alves2017). Present study echinoderms (sand dollars) buried by transgression must have lived under a water depth of about 7–8 m.
Angulo et al. (Reference Angulo, Giannini, De Souza and Lessa2016) emphasized that the reconstruction of paleo sea-levels for the coast of Rio de Janeiro state using vermetids and barnacles (Tetraclita stalactifera) from the southern coast (from Parati to Búzios; see location in Figure 1) is compatible with the geophysical model of glacial isostatic adjustment proposed by Milne et al. (Reference Milne, Long and Basset2005) for the same region. Angulo et al. (Reference Angulo, Giannini, De Souza and Lessa2016) gathered data of several authors (Delibrias and Laborel Reference Delibrias and Laborel1969; Laborel Reference Laborel1969; Martin and Suguio Reference Martin and Suguio1978; Martin et al. Reference Martin, Suguio and Flexor1979; Martin et al. Reference Martin, Suguio, Flexor, Bittencourt and Vilas-Boas1979/80; Martin and Suguio Reference Martin and Suguio1989; Martin et al. Reference Martin, Suguio, Dominguez and Flexor1997; Angulo et al. Reference Angulo, Lessa and Souza2006), with ages ranging from about 4700 cal BP to the present, showing a decline in relative sea-level in this period from +3.0 ± 1.0 m to the PMSL. These data were also used by Milne et al. (Reference Milne, Long and Basset2005) to validate their models with the peat samples dated by Ireland (Reference Ireland1987).
Using the same approach, a data compilation of Ireland (Reference Ireland1987), Mansur et al. (Reference Mansur, Ramos, Godoy and Nascimento2011) and Angulo et al. (Reference Angulo, Giannini, De Souza and Lessa2016) were superimposed on the models of Milne et al. (Reference Milne, Long and Basset2005) (Figure 6). Other data obtained in the literature were added, such as:
(i) Silva et al. (Reference Silva, Silva, Souza and Pinto2014), related to the formation of Itaipuaçu beach rock (see location in Figure 1), with 14C age of 8110 ± 30 BP (8682–8400 cal BP), placing the beach position at the time between –5 and –7 m depth (below PMSL);
(ii) Jesus et al. (Reference Jesus, Dias, Muniz, Macario, Seoane, Quattrociocchi, Cassab, Aguilera, Souza and Alves2017), with seven samples of vermetids collected in the region of Búzios between +0.243 ± 0.5 m and +1.622 ± 1.0 m, dated from 1397 ± 81 BP (1137–752 cal BP) to 3139 ± 64 BP (3097–2753 cal BP), indicating higher past sea-levels.
The 14C-dated beach rocks and vermetids are indicative of paleo-shoreline positions and are used here to reconstruct sea-level variation. Figure 6 shows that the age of the Jaconé beach rock carbonate cement (Mansur et al. Reference Mansur, Ramos, Godoy and Nascimento2011) conforms to the geophysical modelling of Milne et al. (Reference Milne, Long and Basset2005), while the shells age of the same beach rock departs considerably from the model. The age of the Itaipuaçu beach rock (Silva et al. Reference Silva, Silva, Souza and Pinto2014), although it was obtained from the dating of a shell sample, is also consistent with the curve of Milne et al. (Reference Milne, Long and Basset2005).
The relative sea-level variation at the end of the most recent PMT on the coast of Rio de Janeiro state is well delineated, with a good correlation with the geophysical models of Milne et al. (Reference Milne, Long and Basset2005) and the paleo level indicators used here. Thus, we argue that the age of Jaconé beach rock cement (Mansur et al. Reference Mansur, Ramos, Godoy and Nascimento2011) and the model by Milne et al. (Reference Milne, Long and Basset2005) represent the best parameters to infer the interval in which, during the last PMT, the relative sea-level crossed the PMSL on the coast of Rio de Janeiro state: between 7945 cal BP and approximately 7500 cal BP.
Correlating the calibrated age of the echinoderms (7770–7540 cal BP) with the curve shown in Figure 6, it is reasonable to state that the relative paleo sea-level was at that time equal to or very close to PMSL (0 m). The sand dollars are positioned between approximately –7 and –8 m below PMSL, which means they must have lived under a water depth of about 7–8 m, consistent with the environment where they are found today, from the intertidal to the subtidal zone, on sandy substrate. Therefore, it is interpreted that during a fast Holocene marine transgression, the sand dollars colonized the substrate on a shoreface environment. Roncarati and Neves (Reference Roncarati and Neves1976) recognized a correlated subsurface deposit in the center of the coastal plain between –9 and –11 m, levels compatible with the drilling and excavation analyzed in this research.
Interpretations of Stratigraphy Observed
Based on the model of Turcq et al. (Reference Turcq, Martin, Flexor, Suguio and Tasayaco-Ortega1999), it is interpreted that at the end of the most recent PMT (Figure 7c), when the relative sea-level exceeded the current level (PMSL), the Pleistocene inner barrier was partially reworked, leading to deposition of sand on the ancient shoreface. It is estimated that this stage promoted the burial of the echinoderms (Figures 3 and 6) and accumulation of almost 1.8 m thick of fine sand rich in shells of bivalves and gastropods (Figure 2). The occurrence of bivalves, mainly Anomalocardia flexuosa and Anadara notabilis, is indicative of the establishment of transgressive estuarine sand flats, analogously to the observations by Sloss et al. (Reference Sloss, Jones and Murray-Wallace2005) in Lake Illawarra (NSW, Australia) regarding the occurrence of Anadara trapezia and Ostrea angasi.

Figure 7 Evolutionary model of Jacarepaguá coastal plain as proposed by Turcq et al. (Reference Turcq, Martin, Flexor, Suguio and Tasayaco-Ortega1999), with the ages of phases (c), (d), and (e) calibrated and adjusted to the literature updates (see Figure 6 and corresponding text). The location of the survey site of the present study (B1) is superimposed on the model. 14C ages published by Maia et al. (Reference Maia, Martin, Flexor and Azevedo1984) are presented calibrated (2 σ) on age range BP, at (d), (e), and (f). Source: modified after Turcq et al. (Reference Turcq, Martin, Flexor, Suguio and Tasayaco-Ortega1999).
According to Turcq et al. (Reference Turcq, Martin, Flexor, Suguio and Tasayaco-Ortega1999), after the drowning of the Pleistocene coastal plain, during the Holocene transgression, the outer barrier and the paleo-lagoon of Marapendi developed (Figure 7d). In this period, the marine dynamics ceased to act in isolation in the studied area, and the estuarine/lagoon dynamics began to prevail. The first indication of this phase in the analyzed core is the muddy-sand layer at –7.2 m. The increase of OM and CaCO3 content is consistent with an environment subject to lower hydrodynamic energy, but with a connection to the sea. The reduced sorting and the less rounded sand grains with moderate sphericity indicate decreased reworking, determining the characteristics of a lagoonal/estuary environment, where the sea waves do not act directly on the sediments. The 14C age of 5440–5070 cal BP, obtained from a sample collected at –6.6 m, demonstrates that the outer barrier-lagoon system began to form before this age, corroborating with the model of Turcq et al. (Reference Turcq, Martin, Flexor, Suguio and Tasayaco-Ortega1999) and with data from Ireland (Reference Ireland1987), less than 70 km east of the study area.
The stabilization of the relative sea-level after the Holocene maximum transgression around 6000 cal BP led to the isolation of the outer lagoon system through the build-up of the Holocene barrier (Figure 7d) (in agreement with Turcq et al. Reference Turcq, Martin, Flexor, Suguio and Tasayaco-Ortega1999), followed by the sea-level regression that accelerated the infill/shallowing of the outer lagoon (Figure 7e). The distinction among the muddy layers identified in the core reveals the evolution of the lagoonal environment. The muddy layer at –6.3 m presents OM and CaCO3 content about two times higher than the muddy layer bellow, has vegetal remains and is almost ten times muddier (see Figure 5), which may represent the transition between estuarine conditions and infill/isolation of the Marapendi lagoon around core B1/excavation site.
Between –7.2 and –6.3 m, the muddy sediments interlayered with lagoonal sands reflect a typical process of a flood tidal delta, as characterized by Dalrymple et al. (Reference Dalrymple, Zaitlin and Boyd1992) and Dalrymple and Choi (Reference Dalrymple and Choi2007). The flood tidal deposit is then buried by the build-up of the Holocene barrier. Between –6.3 and –6 m, the fine sand layer with shell fragments suggests the last stage of sedimentation before complete isolation of the paleo-lagoon of Marapendi. Thus, the estuarine-lagoon phase is recorded in at least 1.2 m thick on the core (see Figure 5).
According to Turcq et al. (Reference Turcq, Martin, Flexor, Suguio and Tasayaco-Ortega1999), a marine regression began around 5200 cal BP. Maia et al. (Reference Maia, Martin, Flexor and Azevedo1984) presented 14C ages of samples from paleo-lagoonal beaches that revealed the reduction of the size of the Jacarepaguá and Marapendi lagoons during regression (see Figures 7e and 7f). In the present research, the regressive build-up of the Holocene barrier is interpreted as promoting sandy sedimentation between –6 and –2.3 m on the studied area, which became medium and coarse sand, well-sorted, and without calcareous bioclasts (see Figure 5). From –2.3 m anomalies are observed in all parameters analyzed and are probably related to human intervention, which also resulted in the 14C age inversion from the sample collected at –2.1 m (7170–6880 cal BP).
CONCLUSIONS
Marine transgressions and regressions developed barrier-lagoon systems along the central coast of Rio de Janeiro since Late Pleistocene on a tectonically stable continental margin. The low gradient continental shelf acted as the main sediment source due to the low fluvial sediment input on this wave-dominated coast. Based on the revised sea-level curve, the postglacial marine transgression (PMT) crossed the present mean sea-level between 7945 and 7500 cal BP on the region increasing the accommodation space.
In the studied area, at Barra da Tijuca (Jacarepaguá coastal plain), the PMT allowed the sedimentary deposition of a shoreface paleoenvironment between 7 and 8 m water column evidenced by the presence of a marine paleo-surface colonized by Encope emarginata. These echinoderms lived in front of the inner Pleistocene coastal barrier where they were buried due to the sub-aqueous rework of the barrier at 7770–7540 cal BP.
The PMT developed the outer Holocene barrier and back-barrier lagoonal deposits of the Marapendi lagoon since at least 5440–5070 cal BP, and the deposition of flood tidal delta sediments. The incipient base-level lowering enhanced the lagoon shallowing and infill, followed by relative sea-level stability that allowed the regressive widening/progradation of the Holocene barrier.
ACKNOWLEDGMENTS
The authors are deeply grateful to the engineer Roberto Almeida for motivating this research and kindly providing access to the study area. We give thanks also to Dr Alexandre Pimenta (Museu Nacional/UFRJ) for the taxonomic identification of shells, to Professor Dr Alberto Figueiredo Jr. (LAGEMAR/UFF) for the permission to use the pickup truck and the drilling equipment, and to the anonymous reviewers who helped to improve the manuscript. The authors are thankful to Secretaria Nacional de Portos for subsidizing the fieldwork and to the Development Agencies, CNPq (Conselho Nacional de Desenvolvimento Científico e Tecnológico, 307771/2017-2, INCT-FNA, 464898/2014-5) and FAPERJ (Fundação Carlos Chagas Filho de Amparo à Pesquisa do Estado do Rio de Janeiro, E-26/110.138/2014 and E26/203.019/2016), for the support. This study was financed in part by Coordenação de Aperfeiçoamento de Pessoal de Nível Superior - Brasil (CAPES) - Finance Code 001.