INTRODUCTION AND SITE BACKGROUND
The goal of the Azraq Marshes Archaeological and Paleoecological Project (AMAPP) is to examine how the fluctuating Azraq wetlands and surrounding Azraq Basin environment have been exploited by hominins during the middle (781–126 ka) and late (126–12 ka) Pleistocene. One particular focus of the project is to examine the role of the wetlands as a desert refugium during times of increased regional aridity, as well as how changing climates affected local ecology, lithic technology, and hominin occupations around the wetlands (Cordova et al., Reference Cordova, Nowell, Bisson, Pokines, Ames and al-Nahar2009, Reference Cordova, Nowell, Bisson, Ames, Pokines, Chang and al-Nahar2013; Ames, Reference Ames2014; Ames and Cordova, Reference Ames and Cordova2014, Reference Ames and Cordova2015; Ames et al., Reference Ames, Nowell, Cordova, Pokines and Bisson2014a, Reference Ames, Nowell, Pokines and Cordova2014b; Bisson et al., Reference Bisson, Nowell, Cordova, Poupart, Pokines and Ghaleb2014; Nowell, Reference Nowell2014; Pokines et al., Reference Pokines, Hoskins, Ames, Kravitz and Beck2014; Nowell et al., Reference Nowell, Walker, Cordova, Ames, Pokines, Stueber, DeWitt and al-Souliman2016; Walker, Reference Walker2017). AMAPP also aims to situate these results within the larger patterns of Pleistocene biogeography in Jordan and southwest Asia in general (Pokines and Ames, Reference Pokines and Ames2015a). The present research examines the faunal remains derived from ongoing excavations at Shishan Marsh 1 (SM1), which is situated along the former wetlands margin and includes undisturbed Pleistocene deposits. These archaeological deposits span a period when multiple hominin taxa may have occupied this region, which is an area that intermittently served as a part of a corridor for hominin and other taxa at a crossroads between Africa and Eurasia (Bisson et al., Reference Bisson, Nowell, Cordova and Kalchgruber2007a, Reference Bisson, Nowell, Cordova and Kalchgruber2007b; Bar-Yosef and Belmaker, Reference Bar-Yosef and Belmaker2011; Bar-Yosef and Belfer-Cohen, Reference Bar-Yosef and Belfer-Cohen2013; Stewart et al., Reference Stewart, Louys, Price, Drake, Groucutt and Petraglia2017). The undisturbed Pleistocene deposits in the Azraq wetlands have yielded abundant lithic industries associated with faunal remains in generally poor condition because of postdepositional taphonomic processes.
Regional context
The greater Azraq Oasis area is located in the Azraq Basin in Jordan’s Eastern Desert. This large inland basin is located almost completely within Jordan’s borders, with small areas extending into southern Syria and northern Saudi Arabia. The total area approaches 13,000 km2 (El-Naqa, Reference El-Naqa2010). The mean annual precipitation registered at Azraq in the center of the basin is 54 mm, with most precipitation concentrated in December through January (Shatnawi et al., Reference Shatnawi, AlAyyash and Abdellhadi2014). Seasonal winter rainfall produces temporary but extensive pools inundating the low-lying salty mudflat (‘qa in Arabic). The arid environment around the oasis at the center of the basin sustains only Saharo-Arabian vegetation (Al-Eisawi, Reference Al-Eisawi1996; Woolfenden and Ababneh, Reference Woolfenden and Ababneh2011).
Until only a few decades ago, the wetlands were a thriving ecosystem supporting a diverse local fauna that included multiple mammalian species, abundant migratory avian species, and endemic and introduced fish (Nelson, Reference Nelson1973, Reference Nelson1985; Villwock et al., Reference Villwock, Scholl and Krupp1983; Andrews, Reference Andrews1995; Amr, Reference Amr2000; Ramsey et al., Reference Ramsey, Jones, Richter and Rosen2015). The springs were fed by the uppermost aquifer that is recharged through the Jebel Druze basalt massif less than 100 km north in Syria (El-Naqa, Reference El-Naqa2010). The two major Azraq wetlands consisted of the Druze Marsh in the north and the Shishan Marsh in the south, with their former extents approximately 2 km2 and 6 km2, respectively (Ames and Cordova, Reference Ames and Cordova2015). Water pumping to feed the country’s growing urban areas and agricultural needs far exceeded the natural ability of the aquifer to recharge, causing a large drop in the water table (Al-Kharabsheh, Reference Al-Kharabsheh2000). This drop led to the complete drying up of the spring-fed pools and a large loss of biodiversity (Al-Eisawi, Reference Al-Eisawi2005). In 1978, the Royal Society for the Conservation of Nature (RSCN) established the Azraq Wetland Reserve, and the implementation of artificial pumping allowed the restoration of a fragment of the former wetlands expanse as a nature preserve (France, Reference France2010; RSCN, http://www.rscn.org.jo/ [accessed 17 October 2017]). Open water today therefore is present only in the southern Shishan Marsh because of this pumping, and the northern Druze Marsh remains dry. The loss of permanent surface water has had the effect of exposing large areas of Paleolithic archaeological deposits, some of which have been previously surveyed and excavated. These include the Early Epipaleolithic site of ‘Ayn Qasiyya (Richter et al., Reference Richter, Maher, Stock, Allcock, Jones, Martin and Thorne2009; Jones and Richter, Reference Jones and Richter2011; Ramsey et al., Reference Ramsey, Jones, Richter and Rosen2015), the Lower Paleolithic site of ‘Ain Soda (Rollefson et al., Reference Rollefson, Quintero, Low, Schnurrenberger and Watson1996, Reference Rollefson, Quintero, Wilke, Schnurrenburger, Low and Watson1997a, Reference Rollefson, Schnurrenburger, Quintero, Watson and Low1997b; Dirks, Reference Dirks1998; Cordova et al., Reference Cordova, Rollefson, Kalchgruber, Wilke and Quintero2008), and the Druze Marsh Paleolithic site, also excavated under AMAPP (Ames et al., Reference Ames, Nowell, Cordova, Pokines and Bisson2014a, Reference Ames, Nowell, Pokines and Cordova2014b; Ames and Cordova, Reference Ames and Cordova2015).
Shishan Marsh 1 (SM1)
The site of Shishan Marsh 1 (SM1) is located within the boundaries of the Azraq Wetland Reserve, at coordinates 31°49.93′N 36°49.13′E (Figs. 1 and 2). The northern edge of the site was exposed years earlier by mechanical earthmoving during attempts to create pooling spaces for water as the marshes began to dry up from the excessive groundwater pumping. Test excavations of SM1 began in 2013, with subsequent excavation in the 2014 and 2015 seasons. More than 20 m2 of deposits have been excavated down to sterile sediment. Exposures SM2 through SM13 also have been examined in the immediate area to reconstruct the overall stratigraphy. The Lower Paleolithic site of ‘Ain Soda is less than 100 m north of SM1, while the Early Epipaleolithic site of ‘Ayn Qasiyya is less than 200 m northwest. Other sites in the area include a basalt wall adjacent to the extant marshes to the east of SM1, tentatively assigned to the Umayyad (early Islamic) period (Watson and Burnett, Reference Watson and Burnett2001), although additional research is underway to explore its origins.

Figure 1. (color online) Map of southwest Asia (A) and the Azraq region (B) showing the Near East sites named in the text: Qesem (1); Neve David, El Wad, Skhul, Tabun, Qafzeh, Meged, Ubeidiya, Ma’ayan Baruch (2–9); Tor Hamar (10); Wadi Hasa (11); Wadi Jilat 6 (12); Kharaneh IV (13); Uwaynid 14, 18 (14, 15); SM1, SM13, ‘Ain Soda, ‘Ayn Qasiyya, Azraq 18, C-Spring (16–21); Druze Marsh (22); Douara, El Kowm (23, 24); Sihi (25); Palegawra (26); Wezmeh (27); Baynunah (28); Al Sufouh 2 (29); and Shar-i-Sokhta (30). The black rectangle in panel A identifies the area shown in panel B.

Figure 2. Google Earth Pro (Google Earth, 2018) image of the Shishan Marsh 1 (SM1) site area (1), with nearby SM13 (2), ‘Ain Soda (3), and ‘Ayn Qasiyya (4). The satellite image date is March 3, 2016.
Although the site’s stratigraphy is currently undergoing analysis, some components of the depositional environment have been interpreted (Fig. 3). The middle Pleistocene occupation levels are located in a transitional environment between a receding lake margin and shallow ponds/marshy wetlands at the edge of a fan delta. The local bedrock is chert limestone of the Umm Rijam formation; level 10 on top of this consists of lacustrine clay-loam deposits, corresponding to lake recession. Level 9 is a high-energy facies of a fan delta that corresponds to alluvial influx. Level 8 is a low-energy fan delta facies optically stimulated luminescence (OSL) dated to 266±40 ka (ECU-0019; Nowell et al., Reference Nowell, Walker, Cordova, Ames, Pokines, Stueber, DeWitt and al-Souliman2016). Level 7b was formed through alluvial deposition, sheet erosion, and eolian silt accretion and was OSL dated to 125±12 ka (ECU-0018). Level 7a was formed through eolian sandy silt accretion and has been OSL dated to 119±40 ka (ECU-0017). The environments interpreted from these layers suggest that levels 8, 7c, and 7b correspond to marshes/pools receiving low-energy alluvial influx, and level 7a corresponds to subsequent desiccation of the wetlands margin. Levels 3 through 6 represent archaeologically sterile lacustrine and palustrine deposits. Level 2 is composed of heavily mixed Holocene deposits near the surface, with inclusions ranging from Acheulean artifacts to recent trash. This level has a high mollusc shell content from small marsh taxa, primarily gastropods (Kalbe et al., Reference Kalbe, Ames, Nowell, Cordova and Pokines2014).

Figure 3. (color online) Stratigraphic profile of Shishan Marsh 1 (SM1) (Cordova et al., Reference Cordova, Nowell, Ames, Pokines, DeWitt and Al-Souliman2015).
The stone tool industries are undergoing analysis, with approximately 10,000 lithic artifacts excavated as of the 2015 field season. The sources of raw material are local flint nodules and small fluvial clasts from nearby wadi gravels. The lithic assemblage is characterized by small to moderately sized ovate and discoid bifaces and a predominance of small flake tools. Preliminary results of an ongoing site formation analysis, including the lack of postdepositional edge damage and the random distribution of object orientations, suggest that fluvial transport was not a significant site formation process—that is, the artifacts and faunal remains appear to be in their primary contexts (Ames et al., Reference Ames, Nowell, Pokines and Cordova2014b).
Faunal history
Historically and prehistorically, the Azraq Basin supported multiple species of mammalian megafauna from the Palearctic (Europe, most of Asia, and North Africa) and Afrotropic (sub-Saharan Africa and the Arabian Peninsula) biogeographic ecological zones. Palearctic mammalian megafaunal species inhabiting the region included the steppe-dwelling Equus caballus (horse), E. hemionus (onager or Asiatic wild ass; locally extinct), and E. hydruntinus (European wild ass; extinct) (Ducos, Reference Ducos1975; Davis, Reference Davis1980; Eisenmann, Reference Eisenmann1986; Eisenmann and Beckouche, Reference Eisenmann and Beckouche1986; Forsten, Reference Forsten1986; Groves, Reference Groves1986; Uerpmann, Reference Uerpmann1987; Bennett and Hoffman, Reference Bennett and Hoffman1999). Morphological analysis (Burke et al., Reference Burke, Eisenmann and Ambler2003) and new genetic data (Orlando et al., Reference Orlando, Mashkour, Burke, Douady, Eisenmann and Hänni2006), however, indicate that E. hydruntinus may be a subspecies of E. hemionus.
The fauna also included Elephas hysudricus, the extinct ancestor of the modern Asian elephant (E. maximus), the core distribution of which is in the Indo-Malayan zone (Maglio, Reference Maglio1973; Shoshani and Eisenberg, Reference Shoshani and Eisenberg1982; Fleischer et al., Reference Fleischer, Perry, Muralidharan, Stevens and Wemmer2001; Nanda, Reference Nanda2002, Reference Nanda2013; Vidya et al., Reference Vidya, Sukumar and Melnick2009; Albayrak and Lister, Reference Albayrak and Lister2012; Lister et al., Reference Lister, Dirks, Assaf, Chazan, Goldberg, Applbaum, Greenbaum and Horwitz2013); Stephanorhinus hemitoechus, an extinct rhinoceros species associated with grassland/intermediate environments (Clutton-Brock, Reference Clutton-Brock1970, Reference Clutton-Brock1989; Fortelius et al., Reference Fortelius, Mazza and Sala1993; Pandolfi and Tagliacozzo, Reference Pandolfi and Tagliacozzo2015; Rivals and Lister, Reference Rivals and Lister2016); and Bos primigenius (aurochs), the extinct ancestor of domesticated cattle (B. taurus) (van Vuure, Reference van Vuure2003; Hall, Reference Hall2008). Relatively little is known about the wild ancestor of domesticated dromedary camels (Camelus dromedarius), but their range likely included much of the southwest Asian region as indicated by their limited fossil history and adaptations to arid environments (Compagnoni and Tosi, Reference Compagnoni and Tosi1978; Gauthier-Pilters and Dagg, Reference Gauthier-Pilters and Dagg1981; Smuts and Bezuidenhout, Reference Smuts and Bezuidenhout1987; Grigson et al., Reference Grigson, Gowlett and Zarins1989; Köhler-Rollefson, Reference Köhler-Rollefson1991, Reference Köhler-Rollefson1993; Stanley et al., Reference Stanley, Kadwell and Wheeler1994; von den Driesch and Obermaier, Reference von den Driesch and Obermaier2007; von den Driesch et al., Reference von den Driesch, Bruckner, Obermaier and Zander2008; Beech et al., Reference Beech, Mashkour, Huels and Zazzo2009). Other ungulate species formerly common in the region include three species of gazelle (Gazella gazella, G. subgutturosa, and G. dorcas) and the antelope species hartebeest (Alcelaphus buselaphus) (Osborn and Helmy, Reference Osborn and Helmy1980; Harrison and Bates, Reference Harrison and Bates1991; Amr, Reference Amr2000; Jones, Reference Jones2012). Gazella dorcas and A. buselaphus, however, have today/historically a primarily Afrotropic range, as do the majority of antelope species.
Afrotropic carnivore species whose ranges also formerly extended into this region include lion (Panthera leo), spotted hyena (Crocuta crocuta), and cheetah (Acinonyx jubatus); the latter species was made locally extinct in Jordan and surrounding areas only in the past century (Harrison and Bates, Reference Harrison and Bates1991). Striped hyena (Hyaena hyaena) has a low population density in Jordan, and leopard (Panthera pardus) may be locally extinct; both carnivore species were formerly more abundant (Harrison and Bates, Reference Harrison and Bates1991; Amr, Reference Amr2000). Multiple efforts also have been made to reintroduce Arabian oryx (Oryx leucoryx) into the region from captive stock (Jungius, Reference Jungius1985; Abu-Zinada et al., Reference Abu-Zinada, Habibi and Seitre1988; Gillet, Reference Gillet1989; Asmodé and Khoja, Reference Asmodé and Khoja1990). The Azraq environment that sustained the examined mammalian faunal community possibly had characteristics similar to modern East African environments, but with lower biodiversity. The local and migratory avian community was highly diverse, as is the modern one, with numerous species attracted to the wetlands (Andrews, Reference Andrews1995; Tyrberg, Reference Tyrberg1998) and with terrestrial species such as ostrich (Struthio camelus) (Garrard et al., Reference Garrard, Betts, Byrd, Hunt, Colledge, Copeland, Montague and West1988a, Reference Garrard, Colledge, Montague and Hunt1988b; Martin et al., Reference Martin, Edwards and Garrard2013). Multiple mammalian microfaunal species are found in the Azraq Basin that are adapted to the desert environment (Harrison and Bates, Reference Harrison and Bates1991; Amr et al., Reference Amr, Al-Melhim and Yousef1997; Amr, Reference Amr2000; Rifai et al., Reference Rifai, Al-Melhim, Gharaibeh and Amr2000; Abu Baker et al., Reference Abu Baker, Qarqaz and Amr2005; Shehab and Ciach, Reference Shehab and Ciach2008).
Excavation and analytical methods
Levels 7a–c and 8 were excavated in 1 m units divided into quadrants and following natural stratigraphic levels. For thicker levels, quadrants were divided into vertical spits of 5 cm within the natural levels, meaning the maximum excavated context volume is a 50×50 cm area with a depth of 5 cm. The level 2 deposits were excavated in places by full 1 m unit to their full depth, because these deposits were mixed, and then screened. Site features and all artifacts, bone, and other objects at least 2.5 cm in maximum dimension were mapped using a Leica total station. Multiple points were taken to record the orientation on all mapped items where the length was at least 1.5 times greater than its width (i.e., where a true measurable orientation axis was present). All individual sediment lots were dry-screened through a 2 mm mesh. Where necessary, bone preservation in the field and during later analysis was aided by coating the surfaces with a dilute solution of Acryloid B-72 in acetone. Faunal identifications were facilitated by comparative collections at the Mammalogy Department, Peabody Museum, Harvard University (Cambridge, MA) and the Department of Mammalogy and the Division of Paleontology, American Museum of Natural History (New York). Where necessary, published sources also were consulted (e.g., Smuts and Bezuidenhout, Reference Smuts and Bezuidenhout1987; Olsen, Reference Olsen1988; Steiger, Reference Steiger1990; Peters, Reference Peters1998). Faunal size classes (I, II, etc.) follow those developed by Brain (Reference Brain1974) as based on adult live masses for southern African antelope fauna, and these are extended to all large vertebrates.
SM1 SITE CONTEXTS
Level 2
Level 2 was unique at the site of SM1 in that the remains of vertebrate microfauna, including fish, birds, herpetofauna, and Crustacea were also recovered. The bird and fish remains are undergoing analysis. The mammalian fauna also consisted largely of domesticated species or was consistent with these species. These deposits, however, are highly mixed, and their fauna can be used only as a general indicator of the presence of these taxa during the Holocene. Level 2b at exposure SM13, however, appears to be undisturbed deposits dating to ca. 20,000 yr BP and may correlate to Epipalaeolithic occupations at nearby ‘Ayn Qasiyya (Richter et al., Reference Richter, Maher, Stock, Allcock, Jones, Martin and Thorne2009).
Level 2 at SM1 also yielded a single piece of worked bone, a rounded portion of large mammal long bone shaft broken at the other end (Fig. 4). The maximum length is 5.5 cm. Its function and age are unknown, as it is consistent in form with multiple artifact types, including projectile tips or awls from the broader region (Edwards, Reference Edwards1991; Abuhelaleh et al., Reference Abuhelaleh, Al Nahar, Hohenstein, Berruti and Cancellieri2015).

Figure 4. (color online) Worked bone from level 2 Shishan Marsh 1 (SM1).
Levels 7b, 7c, and 8
The level 7b deposits were contiguous across most of the excavated area, whereas the level 7c deposits excavated to date were not extensive and had only limited fauna recovered. The level 8 deposits were contiguous across all of the excavated area. Analysis of the lithic material from these levels at SM1 is Late Acheulean of the Azraq facies, which is characterized by small- to moderately sized ovate and discoid bifaces and a predominance of flake tools (Copeland, Reference Copeland1988). Level 7b and 7c deposits are dominated by both modified and unmodified Levallois flakes and noticeably fewer biface thinning flakes. By contrast, level 8 is characterized by more bifaces and biface reduction flakes.
The majority (73%) of complete tools and utilized flakes from SM1, except for bifaces and Levallois products, are smaller than 50 mm, with an average length of 42.02 mm and a median value of 39.85 mm. The majority of these small tools are made from fluvial clasts from local wadis. Included in this small-tool assemblage are a large number of tool types normally associated with the Upper Paleolithic, such as perçoires (borers), burins, and endscrapers. Some of these tool types have been noted in other Lower Paleolithic small-tool assemblages as well (e.g., Brühl, Reference Brühl2003; Chazan et al., Reference Chazan, Avery, Bamford, Berna, Brink, Fernandez-Jalvo and Goldberg2012; Zaidner, Reference Zaidner2013), although they are normally quite rare.
Disturbed deposits
Although multiple faunal remains were noted among the disturbed deposits, only the fragments referred to Elephas cf. hysudricus are analyzed here, as their zoogeographic/temporal association with the levels 7b, 7c, and 8 deposits is likely, and their preservation state generally matches the faunal remains recovered from the intact deposits and in particular the dental remains of Palaeoloxodon cf. recki (see Proboscidea, below).
Other SM exposures
Other vertical exposures near SM1 yielded limited amounts of faunal remains that were collected for analysis. Their taphonomic condition was similar throughout to the SM1 fauna, and their stratigraphic levels could be linked into the sequence of SM1. One of these, SM13 yielded small amounts of identifiable faunal remains that are noted subsequently.
TAXONOMIC AND OSTEOLOGICAL REPRESENTATION
The preliminary data for faunal identifications from SM1 (and SM13) are presented in Table 1. Overall faunal preservation, with the exception of level 2 at SM1, was poor and likely created a significant bias favoring the representation of larger species over smaller ones. Subfossil bone integrity was typically poor and highly friable, making only limited identifications possible. Multiple instances were noted of bone degrading until only a “ghost” (Gordon and Buikstra, Reference Gordon and Buikstra1981) remained visible in the sediments. The poor preservation was likely attributable in large part to low pH in the deposits. Bone surfaces were usually degraded, so evidence of butchery practices in the form of cut marks if formerly present was not obtainable. The dentin portions of the recovered teeth were often in very poor condition or largely eroded away relative to the enamel portions, leading to high amounts of tooth fragmentation. Fragments of bones and teeth therefore formed a large proportion of the faunal sample, and the majority of these were tooth and long bone shaft fragments, as the postdepositional destructive processes selectively eliminated the more fragile cortical and cancellous structures of the epiphyses as well as bones with low structural densities (e.g., skulls, vertebrae, and ribs; Lyman, Reference Lyman1994). Some recovered elements also were relatively highly identifiable despite heavy fragmentation, especially in the case of tooth fragments of elephants. As noted, fragments from disturbed deposits adjacent to the site consistent with having derived from a single partial molar of Elephas cf. hysudricus were able to be refit. All results discussed subsequently pertain to SM1 unless otherwise noted.
Table 1. Faunal representation from Shishan Marsh 1 (SM1) and SM13. MNI, minimum number of individuals; NISP, number of identified specimens.

a Estimated.
b MNI not estimated; skeletal remains under analysis.
Crustacea
A single element (a distal pincer) identified to Crustacea was identified from level 2. Only one species of decapod is reported as endemic to Azraq, Potamon potamios (Scates, Reference Scates1968), and this element is consistent with this species. This freshwater crab species is reported as having been abundant in the Azraq pools in the past, and its present eastern Mediterranean range includes Sinai, Jordan, southern Anatolia, and Greece (Bilgin and Fidanbaş, Reference Bilgin and Fidanbaş2011). Potamon potamios has been identified from Paleolithic and Neolithic sites in the region (Turnbull and Reed, Reference Turnbull and Reed1974; Edwards, Reference Edwards1991; Simmons et al., Reference Simmons, Rollefson, Kafafi, Mandel, al-Nahar, Cooper, Köhler-Rollefson and Durand2001; Munro et al., Reference Munro, Kennerty, Meier, Samei, al-Nahar and Olszewski2016) and was a potential dietary resource for the Paleolithic inhabitants of Azraq.
Osteichthyes
All fish remains (n=56) identified to date come from level 2, likely because of preferential postdepositional destruction of smaller or less dense bones in the Pleistocene levels. Only one fish species is endemic to Azraq, the Azraq toothcarp (Aphanius sirhani) (Nelson, Reference Nelson1973; Villwock et al., Reference Villwock, Scholl and Krupp1983), and the fish remains are generally too large to have come from this species. Multiple potentially commercial species have been deliberately introduced into the Azraq marshes or into privately excavated pools in the area over the years, including African sharptooth catfish (Clarias gariepinus), redbelly tilapia (Tilapia zillii), blue tilapia (Oreochromis aureus), common carp (Cyprinus carpio), hound’s barbel (Barbus canis), and Jordan bream (Acanthobrama lissneri) (Nelson, Reference Nelson1973; Khoury et al., Reference Khoury, Amr, Hamidan, Al Hassani, Mir, Eid and Bolad2012). Not all of these species are still found in the area, as in the case of the African sharptooth catfish that was introduced into the Azraq marsh system, but this species has not been seen since the year 2000; Jordan bream, however, still breeds in the Shishan Marsh (Khoury et al., Reference Khoury, Amr, Hamidan, Al Hassani, Mir, Eid and Bolad2012). Multiple introduced fish species therefore may be represented in the Holocene deposits.
Amphibian
A single element from level 2 (a partial left humerus) was identified to Amphibia. The preservation of this element was poor, but it is most consistent in morphology with the widespread species green toad (Bufo viridis). This species and the Levantine frog (Rana bedriagae) are the only amphibians currently found in Azraq (Disi et al., Reference Disi, Modry, Necas and Rifai2001). Either of these species would have required the wetlands environment to reproduce, although the adults of the toad species can live away from water.
Aves
Multiple avian elements (n=58) were recovered from level 2, and these are under analysis. The Azraq Oasis is a home to multiple species of breeding bird and is a stopping place for multiple migratory species (Nelson, Reference Nelson1973, Reference Nelson1985). Bird remains have been identified from multiple Paleolithic sites within the Azraq Basin (Garrard et al., Reference Garrard, Betts, Byrd, Hunt, Colledge, Copeland, Montague and West1988a, Reference Garrard, Colledge, Montague and Hunt1988b; Byrd and Garrard, Reference Byrd and Garrard1990; Tyrberg, Reference Tyrberg1998; Martin et al., Reference Martin, Edwards and Garrard2010, Reference Martin, Edwards and Garrard2013) and the broader southwest Asian region (Tchernov, Reference Tchernov1993), but the preservation conditions in levels 7b, 7c, and 8 at SM1 have not been conducive to the survival of these relatively gracile elements. Proteins from avian tissue, subfamily Anatinae (ducks, etc.), have been detected on three stone tool cutting edges from SM1 level 7b (Nowell et al., Reference Nowell, Walker, Cordova, Ames, Pokines, Stueber, DeWitt and al-Souliman2016), so this resource clearly was exploited during those earlier occupations.
Bovidae
Multiple bovid species have been identified from SM1. Level 2 has yielded multiple elements identified to domesticated sheep/goat (Ovis/Capra; n=24; minimum number of individuals [MNI]=2) and cattle (Bos taurus; n=8; MNI=1) that require no further elaboration here. Additional bovid remains (n=14) from this level are consistent in size and morphology with these taxa.
Level 8 yielded a single element (nearly complete, fused, first row phalanx; Fig. 5) of gazelle (Gazella sp.) morphologically consistent with goitered gazelle (G. subgutturosa) or mountain gazelle (G. gazella). The latter species, however, has a current distribution in the higher terrain and coastal plains of the Levant and the Arabian Peninsula (Harrison and Bates, Reference Harrison and Bates1991; Rabinovich and Hovers, Reference Rabinovich and Hovers2004). Goitered gazelle was formerly common in many arid environments within Jordan, including sand deserts, but today is encountered only rarely (Amr Reference Amr2000); its range includes the Levant, the Arabian Peninsula, and eastern Turkey, extending east through the Iranian region as far as western China (Harrison and Bates, Reference Harrison and Bates1991). Its diet consists largely of grasses and perennial dwarf shrubs, and large herds of 50–100 individuals are known (Harrison and Bates, Reference Harrison and Bates1991; Amr, Reference Amr2000). This species was hunted in large numbers at the Azraq region Epipaleolithic sites of Kharaneh IV and Wadi Jilat 6 (Byrd and Garrard, Reference Byrd and Garrard1990; Martin et al., Reference Martin, Edwards and Garrard2010) and also has been identified from the Upper Paleolithic site 784X at Wadi Hasa (Clark et al., Reference Clark, Lindly, Donaldson, Garrard, Coinman, Schuldenrein, Fish and Olszewski1988) and the Epipaleolithic levels at Wadi Uwaynid 18 (Garrard et al., Reference Garrard, Betts, Byrd, Hunt, Colledge, Copeland, Montague and West1988a, Reference Garrard, Colledge, Montague and Hunt1988b; Byrd and Garrard, Reference Byrd and Garrard1990) and Tor Hamar (Henry and Garrard, Reference Henry and Garrard1988). Goitered gazelle is known also from other sites in the broader region, as at the late Pleistocene site of Palegawra Cave in Iraq (Bakken, Reference Bakken2000) and among Pleistocene contexts at El Kowm in Syria (Jagher et al., Reference Jagher, Elsuede and Le Tensorer2015). Gazelle species can subsist without sources of water and obtain the moisture that they require from their diet alone, including metabolic water, although these species will exploit water sources when available (Harrison and Bates, Reference Harrison and Bates1991). The wetlands margins therefore may have attracted large herds of these animals at once. The fragility of their skeletal elements relative to the larger mammalian species, however, may have reduced their recovery from the SM1 deposits.

Figure 5. (color online) First phalanx of Gazella sp., level 8, Shishan Marsh 1 (SM1).
Remains identifiable to wild cattle or aurochs (Bos primigenius) were recovered in small amounts from SM1 levels 7b and 8. The osseous remains consist only of a fragmentary maxillary premolar and a partial mandibular second molar from level 7b and a fragmentary molar from level 8 of SM1; all teeth were adult. The preservation is such that no other firm conclusions can be drawn about wear stage or other parameters. In addition, a single partial molar (a fragment of an M2 or M3) was identified from level 7b of SM13.
Cattle diet consists primarily of grasses, and their range during the Pleistocene and early Holocene included open, mixed, and more closed environments throughout much of the subarctic Palearctic, from western Europe through East Asia and India, including the Levantine Near East and North Africa but not desert Arabia (van Vuure, Reference van Vuure2003). Bos primigenius has been recovered from other nearby sites, including the Acheulean deposits at C-Spring (Clutton-Brock, Reference Clutton-Brock1970, Reference Clutton-Brock1989; Copeland, Reference Copeland1991) and ‘Ain Soda (Lister et al., Reference Lister, Dirks, Assaf, Chazan, Goldberg, Applbaum, Greenbaum and Horwitz2013), and the Epipaleolithic deposits at Kharaneh IV and Wadi Jilat 6 (Martin et al., Reference Martin, Edwards and Garrard2010), ‘Ayn Qasiyya (Jones and Richter, Reference Jones and Richter2011), and Wadi Uwaynid 14 and 18 and Azraq 18 (Garrard et al., Reference Garrard, Betts, Byrd, Hunt, Colledge, Copeland, Montague and West1988a, Reference Garrard, Colledge, Montague and Hunt1988b; Byrd and Garrard, Reference Byrd and Garrard1990; Garrard, Reference Garrard1991). Multiple sites in the coastal Levant also have yielded this species, including the Geometric Kebaran deposits at Neve David Cave (Bar-Oz et al., Reference Bar-Oz, Dayan and Kaufman1998); Kebaran deposits at Ein Gev I (Davis, Reference Davis1974; Marom and Bar-Oz, Reference Marom and Bar-Oz2008); Natufian, Kebaran, Aurignacian, and Mousterian deposits at Hayonim and Upper Paleolithic deposits at Meged (Stiner, Reference Stiner2005); Mousterian deposits at Qafzeh (Bouchud, Reference Bouchud1974) and Amud (Rabinovich and Hovers, Reference Rabinovich and Hovers2004); and middle Pleistocene deposits at Qesem Cave (Stiner et al., Reference Stiner, Barkaib and Gopher2009).
Unidentified bovid remains are also present from levels 7b and 8. The size range of these includes Bos primigenius, but some fragments may correspond to smaller bovid species in the size class II or III range. Some of these therefore could have come from Gazella (size class II). Other medium-sized (size class III) bovids that have been identified in the area include hartebeest (Alcelaphus buselaphus) and possible Boselaphus sp. from the Acheulean site of C-Spring (Clutton-Brock, Reference Clutton-Brock1970, Reference Clutton-Brock1989; Copeland, Reference Copeland1991). Alcelaphus buselaphus used to be present throughout the southwest Asian region, including the coastal Levant, as at the Geometric Kebaran deposits at Neve David Cave (Bar Oz et al., Reference Bar-Oz, Dayan and Kaufman1998), the Aurignacian at Hayonim D (Stiner, Reference Stiner2005), and the Upper Paleolithic and Mousterian deposits at Qafzeh (Bouchud, Reference Bouchud1974).
Camelidae
Multiple elements (n=9) of the domesticated species dromedary camel (Camelus dromedarius) were identified from level 2 and require no further elaboration of this common desert pack animal. Other remains of the size and morphology closest to modern C. dromedarius were identified from levels 7b, 7c, and 8 (Table 2) and require further description, as the evolutionary history of the wild antecedent of domesticated dromedary camel is poorly known (Uerpmann, Reference Uerpmann1987; Martini et al., Reference Martini, Costeur, Le Tensorer and Schmid2015). The wild antecedent of domesticated dromedary camel is presumed to have inhabited the Arabian Peninsula area, but fossil remains substantiating this hypothesis have been limited, possibly because of the destructive taphonomic processes of remains deposited in desert environments (Pokines and Ames, Reference Pokines and Ames2015b; Stewart et al., Reference Stewart, Louys, Price, Drake, Groucutt and Petraglia2017). The nomenclature Camelus dromedarius was originally applied (Linné) to the domesticated form of this species, with no other name assigned to its wild ancestral form, as none was known (Uerpmann, Reference Uerpmann1987). To differentiate the two, the modifier “wild” will be applied when discussing the nondomesticated form recovered from sites clearly predating its domestication.
Table 2. Remains identified to wild Camelus from Shishan Marsh 1 (SM1), Pleistocene levels 7b, 7c, and 8.

Two forms of camel exist today, one-humped domesticated dromedaries and two-humped domesticated bactrian camels (Camelus bactrianus). Multiple theories existed in the past regarding their relationship, but it is clearer now that they are the domesticated forms of two distinct species likely derived from separate wild forms (Peters, Reference Peters1998; Uerpmann and Uerpmann, Reference Uerpmann and Uerpmann2002). A remnant population of wild (potentially feral) C. bactrianus still exists in the Gobi Desert. These domesticated forms, however, are closely related (Steiger, Reference Steiger1990; Stanley et al., Reference Stanley, Kadwell and Wheeler1994) and can produce at least partially fertile offspring (Köhler-Rollefson, Reference Köhler-Rollefson1993; Çakirlar and Berthon, Reference Çakirlar and Berthon2014). Domesticated dromedaries are remarkably adapted to warm, arid environments (Köhler-Rollefson, Reference Köhler-Rollefson1991, Reference Köhler-Rollefson1993), whereas bactrian camels are adapted for colder environments. Domestication of the dromedary may have taken place in Arabia at least by the second millennium BC (Kӧhler, Reference Kӧhler1984; Wapnish, Reference Wapnish1984; Uerpmann and Uerpmann, Reference Uerpmann and Uerpmann2002; Spassov and Stoytchev, Reference Spassov and Stoytchev2004), and the bactrian camel was domesticated in the Iranian region, as at the site of Shar-i-Sokhta (Compagnoni and Tosi, Reference Compagnoni and Tosi1978; Olsen, Reference Olsen1988; Köhler-Rollefson, Reference Köhler-Rollefson1993) or farther east (Peters and von den Driesch, Reference Peters and von den Driesch1997). Domesticated dromedaries spread rapidly to the surrounding semiarid and arid regions including coastal Levant and North Africa as their value for transportation and other uses was recognized (Kӧhler, Reference Kӧhler1984; Wapnish, Reference Wapnish1984; Köhler-Rollefson, Reference Köhler-Rollefson1993; Grigson, Reference Grigson2002; Sapir-Hen and Ben-Yosef, Reference Sapir-Hen and Ben-Yosef2013). Domesticated bactrian camels were spread west at least as far as the Mesopotamian civilizations (Heide, Reference Heide2011). The two species are difficult to differentiate osteologically (Wapnish, Reference Wapnish1984; Olsen, Reference Olsen1988; Steiger, Reference Steiger1990), further confusing their recent fossil history.
The fossil history of the Camelidae in the broader region also includes an extinct giant camel, Camelus thomasi (Gautier, Reference Gautier1966; Uerpmann, Reference Uerpmann1987; Peters, Reference Peters1998), and other large, not fully described forms (Martini et al., Reference Martini, Costeur, Le Tensorer and Schmid2015). Camelus thomasi was considerably larger than extant C. dromedarius, by 20% or more in body size (Peters, Reference Peters1998). This species is known from North Africa, including Tighennif in Algeria (Martini and Geraads, Reference Martini and Geraads2018), and it or other large-bodied camelid is known from sites in the Negev Desert (Grigson, Reference Grigson1983) and Nadaouiyeh Aïn Askar (El Kowm) in Syria (Reynaud Savioz and Morel, Reference Reynaud Savioz and Morel2005). It has been suggested that this species is more closely related on morphological grounds to C. bactrianus (Gautier, Reference Gautier1966), but this has been rejected by other authors who have found that it is closer morphologically to C. dromedarius (Peters, Reference Peters1988). Its evolutionary relationship to the two modern domesticated species is unknown (Martini and Geraads, Reference Martini and Geraads2018), and it became extinct by the end of the Pleistocene (Peters, Reference Peters1998).
Other nearby sites that have remains identified to wild ancestral Camelus dromedarius include the Acheulean deposits at C-Spring (Clutton-Brock, Reference Clutton-Brock1970, Reference Clutton-Brock1989; Copeland, Reference Copeland1991), the Early Upper Paleolithic deposits at Wadi Uwaynid 18 (Garrard et al., Reference Garrard, Betts, Byrd, Hunt, Colledge, Copeland, Montague and West1998a, Reference Garrard, Colledge, Montague and Hunt1988b), and the Pre-Pottery Neolithic B (PPNB) deposits at Ain El-Assad (Turnbull, Reference Turnbull1989). It may also have been present in the Mousterian deposits of Mugharet-el-Emireh (Hedges et al., Reference Hedges, Housley, Law, Perry and Gowlett1987), Qafzeh (Bouchud, Reference Bouchud1974), and Tabun (Payne and Garrard, Reference Payne and Garrard1983) in the coastal Levant. Multiple sites in the El Kowm Basin in Syria have yielded relatively abundant Camelidae remains from the Pleistocene (Boëda et al., Reference Boëda, Noël-Soriano and Griggo2001; Reynaud Savioz and Morel, Reference Reynaud Savioz and Morel2005; Jagher et al., Reference Jagher, Elsuede and Le Tensorer2015), and these remains are under study (Martini et al., Reference Martini, Costeur, Le Tensorer and Schmid2015); Payne (Reference Payne1983) also reported Camelus from Douara Cave in Syria. Intrusion of more recent camel remains into deeper site deposits may have been a factor in site formation at multiple sites, because accelerator mass spectrometry dating on bone of the Camelus remains from Tabun, Mugharet-el-Emireh, and C-Spring indicated that some mixing from more recent deposits has occurred (Hedges et al., Reference Hedges, Housley, Law, Perry and Gowlett1987). It is important to note that the Pleistocene deposits at SM1 were sealed by multiple undisturbed sterile levels, so the intrusion of recent domesticated dromedary camel remains into the lower deposits of this site effectively can be ruled out.
The remains of large numbers of wild dromedary camels that were hunted are known from some Arabian Peninsula sites, including Al Sufouh 2 in the United Arab Emirates, dated by artifact association to the second half of the third to second half of the second millennium AD (von den Driesch and Obermaier, Reference von den Driesch and Obermaier2007; von den Driesch et al., Reference von den Driesch, Bruckner, Obermaier and Zander2008). The site of Baynunah, also in the United Arab Emirates, has the remains of wild dromedaries directly 14C dated to the second half of the fifth millennium BC (Beech et al., Reference Beech, Mashkour, Huels and Zazzo2009). A dromedary mandible from the shell midden site of Sihi in Saudi Arabia yielded a direct 14C date of ca. 7000 BC, which may come from a Neolithic level (Grigson et al., Reference Grigson, Gowlett and Zarins1989), although the dating of this find may be flawed (Peters, Reference Peters1998). Wild dromedaries may have died out in various areas during the Bronze Age, with their replacement among faunal assemblages by the domesticated form (Uerpmann and Uerpmann, Reference Uerpmann and Uerpmann2002).
The wild Camelus remains from levels 7b, 7c, and 8 of SM1 include teeth and postcranial elements (complete inventory in Table 2). The taphonomic conditions at the site mostly favored the preservation of small, dense elements, although in contradiction to the overall pattern for these levels, some portions of vertebrae also were identifiable to this species. All elements where determinable were fully fused and/or of adult size. The identified elements include a partial upper right third molar (M3) (Fig. 6A) in poor condition from level 7b; a partial molar from level 7c; and a metacarpal midshaft fragment (Fig. 6B) and an approximately complete right external and middle cuneiform (Fig. 6C) from level 8.
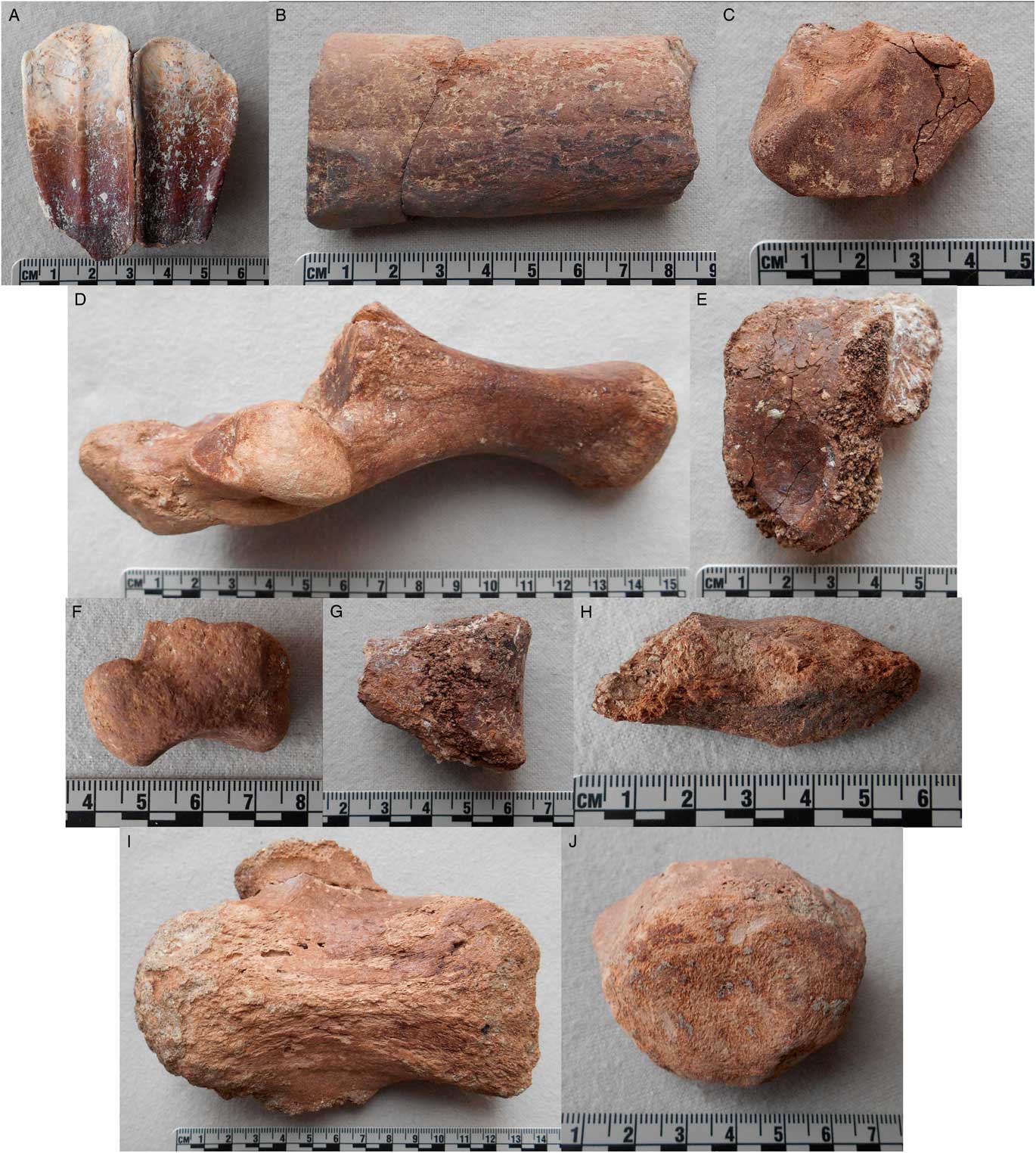
Figure 6. (color online) Examples of wild Camelus remains from Shishan Marsh 1 (SM1): partial right M3 (A), metacarpal midshaft fragment (B), right external and middle cuneiform (C), left calcaneus (D), right unciform (E), right fibula (F), distal first phalanx (G), C5/C6 vertebra anterior articular process (H), C7 vertebra body (I), and C2 vertebra body, caudal portion (J). (A three-dimensional image of the left calcaneus is available for free download; see the Supplementary Materials).
The left calcaneus (Fig. 6D) from level 8 has a maximum length of 141 mm and maximum antero-posterior breadth of 47 mm, following the methods described by von den Driesch (Reference von den Driesch1976). Gautier (Reference Gautier1966) also measured the antero-posterior and transverse (medio-lateral) breadth inferior to the proximal tuberosity of the calcaneus. The maximum length and two additional calcaneus dimensions place it well below the size reported for Camelus thomasi by Gautier (Reference Gautier1966) and closest to the measurements for Camelus dromedarius (Table 3). The distal articular facet measures 42 mm, following the method described by Steiger (Reference Steiger1990, p. 88). This measurement places the SM1 calcaneus closer to the average for Camelus bactrianus (44.5 mm) than C. dromedarius (38.6 mm) (Steiger, Reference Steiger1990, p. 100). In addition, the comparative morphology of the calcaneus of C. thomasi, C. dromedarius, and C. bactrianus was detailed by Peters (Reference Peters1998) and Steiger (Reference Steiger1990, p. 61). The SM1 calcaneus has some postmortem eroded areas, including the sustentaculum tali, so not all points could be compared. Overall, the calcaneus is most consistent in morphology with C. dromedarius, including the relatively narrow shaft, lesser width of the proximal end, shape of the posterior margin, and pattern of the disto-medial articular surfaces.
Table 3. Comparison of measurements of Camelus left calcaneus from Shishan Marsh 1 (SM1), Pleistocene level 8. All comparative data follow Gautier (Reference Gautier1966). All measurements are in millimeters and follow von den Driesch (Reference von den Driesch1976) and Gautier (Reference Gautier1966).

The right unciform from level 8 identified to Camelus is largely intact but in poor condition, with some areas of erosion (Fig. 6E). The morphology of this element also is more consistent with C. dromedarius than C. bactrianus (Steiger, Reference Steiger1990, p. 41), including the more rectangular shape of the proximal articular facet/body. The other carpals identified to Camelus are too eroded to be diagnostic. The Camelus fibulae from levels 7b and 8 are partially eroded and lack their proximal processes, but their overall morphology also is more consistent with C. dromedarius (Steiger, Reference Steiger1990, p. 57). The right Camelus fibula from level 7b (Fig. 6F) was intact enough for measurement, and the maximum width of its proximal articular surface is 19 mm, following the method described by Steiger (Reference Steiger1990, p. 88). The right fibula from level 8 was more eroded and yielded a maximum width of 22 mm. These dimensions place the SM1 right fibulae closer to the average for C. dromedarius (22.2 mm) than C. bactrianus (26.1 mm), and below the minimum value (23.0 mm; n=8) for the latter species (Steiger, Reference Steiger1990, p. 98). The distal first row phalanx (Fig. 6G) was partially eroded and has a maximum surviving distal breadth of 37 mm. The Camelus tooth and vertebral fragments are too fragmentary to supply sufficient species information. The partial M2 and M3 (Fig. 6A) from level 7b are large and consistent in size with modern examples of adult male C. dromedarius; the maximum surviving mesiodistal length of the M3 is 50 mm. These molars display minimal occlusal wear, as does the partial probable M3 from level 8.
The overall recovery pattern for the identifiable wild Camelus dromedarius remains largely reflects density-mediated destruction, whereby most elements surviving consist of teeth and small, dense bones of the front and rear feet/ankles. The exception to this pattern is the recovery of three identifiable cervical vertebra portions (Fig. 6H, I, and J), which was atypical for the Pleistocene levels. The camel teeth were also substantially smaller than those of the elephant species and Stephanorhinus hemitoechus yet substantially larger than those of Bos primigenius, expediting their assignment to Camelus; this artifact of the species representing the faunal size classes present may have served to inflate the number of elements that could be assigned to this genus.
Carnivora
Remains (n=2) from domesticated dog (Canis familiaris) have been identified only from level 2 and require no further description. This species is ubiquitous in the area, and golden jackal (Canis aureus) has been spotted near the site within the Azraq Wetland Reserve during excavations of SM1 (personal observation, JTP, 2015).
A single tooth, a partial lower right adult canine in highly fragmented condition (Fig. 7) from level 8, has been tentatively identified to lion (Panthera leo). In addition to its African range, this species was present through the Near East region and into southeastern Europe well into historical times, and a protected remnant population still exists in India (Haas et al., Reference Haas, Hayssen and Krausman2005). The site of Nadaouiyeh Ain Askar at El Kowm in Syria has also yielded the remains of P. leo (Morel, Reference Morel1997; Reynaud Savioz and Morel, Reference Reynaud Savioz and Morel2005), and Payne (Reference Payne1983) reports Panthera cf. leo from Douara Cave in Syria. This large predator likely exploited some of the same mammalian faunal resources as the hominins utilizing the SM1 site, which was located near the wetlands margin and a therefore natural attractant for herds of herbivores. Felid protein also was tested for on the stone tool sample (Nowell et al., Reference Nowell, Walker, Cordova, Ames, Pokines, Stueber, DeWitt and al-Souliman2016), but no results were consistent with felid.
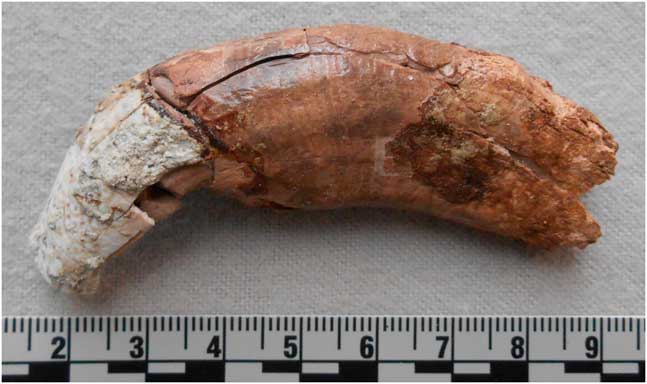
Figure 7. (color online) Partial lower right adult canine (C1), cf. Panthera leo, level 8, Shishan Marsh 1 (SM1).
An additional canine tooth (adult, partial, probable upper right) from level 7b was identified to large carnivore (Fig. 8). The species represented is most consistent with spotted hyena (Crocuta crocuta), but not to the exclusion of other large carnivores likely present in the area, including leopard (Panthera pardus); other taxa including lion and cheetah (Acinonyx jubatus) can be excluded by their size (too large and too small, respectively). The range of spotted hyena and leopard formerly included this area (Harrison and Bates, Reference Harrison and Bates1991), and either would have been attracted to the abundant mammalian faunal species at the water margins. The poor condition of this tooth precludes definitive morphological assessment at the present time.

Figure 8. (color online) Canine tooth (adult, partial, probable upper right) of unidentified large carnivore, level 7b, Shishan Marsh 1 (SM1).
Proboscidea
Elephantid remains were identified from level 7b (n=23) and 8 (n=27). All elements except one consist of molar fragments, some of which could be reassembled into portions of individual teeth. Preservation conditions favored dissolution/degradation of the cementum, so molars tended to fragment into individual enamel lamellae with associated dentin. In addition to the dental remains, one fragment of vertebral body consistent with an elephant and inconsistent with any other species from the site was identified from level 8.
The fragments of molar from level 8 consist of five large portions and numerous smaller fragments. The large pieces could be partially reconstructed into two paired maxillary molars. The left one (Fig. 9) was reconstructed from four pieces (anterior to posterior: SM1-3398, SM1-3418, SM1-3419, and SM1-3420). These sections have six, four, two, and two lamellae, respectively, plus an anteriormost element that appears to be the talon, so the lamellar formula is x14-. Occlusal wear extends posteriorly to the 11th or 12th lamella. The matching right molar (SM1-2915; Fig. 10) consists of seven anterior lamellae, all with occlusal wear, and is interpreted as x7-. The detailed shape and wear stage of the left and right molars are closely similar. The identification of these teeth as the third (last) molars is supported by their large size (especially length of the left molar), number of lamellae (significantly >14 when complete) on any plausible specific determination, and the visible narrowing of the crown toward the posterior: maximum width of the crown, on the 6th or 7th lamella, is 100 mm (Table 4), but on the 14th lamella, it is only 75 mm.
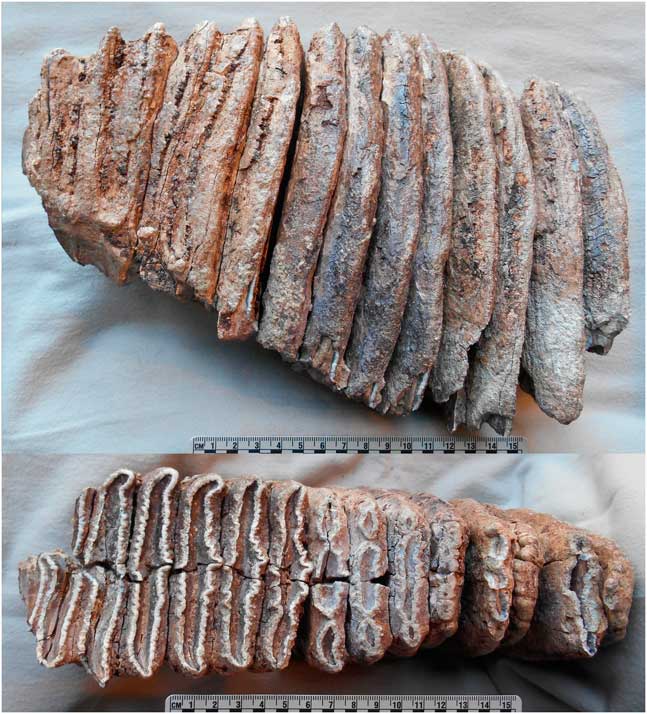
Figure 9. (color online) Upper left partial (probable third) molar, Palaeoloxodon cf. recki, assembled from four pieces (left to right: SM1-3398, SM1-3418, SM1-3419, and SM1-3420), level 8, lateral (top) and occlusal (bottom) views. Anterior is to the left. SM1, Shishan Marsh 1. (Three-dimensional images of the molar fragments are available for free download; see the Supplementary Materials).

Figure 10. (color online) Upper right partial (probable third) molar (SM1-2915), Palaeoloxodon cf. recki, level 8, medial (top) and occlusal (bottom) views. Anterior is to the left. SM1, Shishan Marsh 1. (A three-dimensional image of the molar fragment is available for free download; see the Supplementary Materials).
Table 4. Measurements of elephantid molars from Shishan Marsh. SM1 3398 and 2915 and associated fragments are identified as Palaeoloxodon cf. recki; SM1 Dist A+B, as Elephas cf. hysudricus; SM1 Dist C, as elephantid indet. (see text). Abbreviations: ET, median enamel thickness; H, crown height; HI, hypsodonty index; L, crown length; LF, lamellar frequency; LFB, basal lamellar frequency; LM3, left upper third molar; PF, plate (lamellar) formula; RM3, right upper third molar; RM2/3, right lower second or third molar, RM1/2, right lower first or second molar; W, crown width. For further details, see Lister and Sher (Reference Lister and Sher2015, supplement). Square brackets: incomplete measurements; round brackets: lamella number (l) on which the measurement was taken. All measurements are in millimeters.

The shape of the enamel lamellae, visible both on the worn surface of the teeth (Figs. 9 and 10) and in the CT scans (Fig. 11), shows several features that together are strongly suggestive of the genus Palaeoloxodon (straight-tusked elephants). These are as follows:

Figure 11. Three CT sections of upper left partial molar, Palaeoloxodon cf. recki, level 8, Shishan Marsh 1 (SM1) (molar shown in Fig. 9). Anterior is to the left. Sections progressively deeper in the crown, show lamella 4 (partial) to 14 (top image), lamella 3 (partial) to 14 (middle image), and talon (partial) plus lamellae 1–14 (bottom image).
(a) Fine, tightly packed, low amplitude crenulations along the length of the lamellae (Figs. 9, 10, and 11); (b) Lamellae in early wear (lamellae 7–10 on the occlusal surface) show a dot-dash-dot pattern (i.e., short lateral and medial loops, and a long central loop). On the occlusal surface, lamella 11 shows a row of ~7 digits that fuses to form central “dash” lower in the crown, with separate “dots” visible laterally and medially. In the highest CT scan (Fig. 11), this pattern can be seen on lamellae 10–14; (c) Median anterior and posterior sinuses. On the occlusal surface, lamellae 3–4 show sharp points, strongest posteriorly; lamellae 5–6 have a broader median expansion including two main points posteriorly and one anteriorly; and lamella 7 shows median points anteriorly and posteriorly on the central loop. In the CT scans (Fig. 11), anterior and posterior sinuses are particularly clear in lamellae 10–14, with double sinuses visible on more anterior lamellae.
Metrical data (Table 4) also support the attribution of this specimen to Palaeoloxodon. In Figure 12a, M3 hypsodonty index and lamellar frequency are plotted for the latest named African subspecies of P. recki, P. recki recki from East Africa (ca. 1.0–0.5 Ma), and European P. antiquus (ca. 0.4–0.12 Ma). In addition, samples of Elephas hysudricus from the Siwalik Hills of India/Pakistan (ca. 2.6–0.6 Ma; Nanda, Reference Nanda2002) and from the ‘Ain Soda site adjacent to SM1 (Lister et al., Reference Lister, Dirks, Assaf, Chazan, Goldberg, Applbaum, Greenbaum and Horwitz2013), and modern Elephas maximus, are plotted. Lamellar frequency is measured as the number of lamella-plus-cement intervals in a 10 cm length of molar and is a valuable proxy for lamellar number when the tooth is incomplete. The hypsodonty index is calculated as the maximum height of the unworn molar crown divided by maximum crown width (see Lister and Sher [Reference Lister and Sher2015, supplement] for detailed discussion and description of methodology).

Figure 12. Hypsodonty index and lamellar frequency (LF) plots of upper (A) and lower (B) third molars. Key: black circle, Shishan Marsh; black triangle, Siwalik Elephas hysudricus; black diamond, ‘Ain Soda E. cf. hysudricus left M2/3; white circle, modern E. maximus; gray triangle and ellipse, Palaeoloxodon recki recki; gray circle, P. antiquus; white triangle, Mammuthus. cf. trogontherii (Marine Oxygen Isotope Stage 7).
The SM1 molar (Fig. 12a; black circle) plots at the lower end of the range of P. antiquus and E. hysudricus for lamellar frequency, and intermediate between them (and beyond the observed range of each) in hypsodonty index. It is, however, in the middle of the range of P. recki recki (gray ellipse) on both variables. In enamel pattern, the molar could pertain to either P. antiquus or P. recki, but metrically it conforms better to the latter species, so is referred to Palaeoloxodon cf. recki.
The genus Palaeoloxodon arose in Africa and has a long history (as successive nominal subspecies of P. recki) from ca. 4.0–0.4 Ma (Todd, Reference Todd2005; Sanders et al., Reference Sanders, Gheerbrant, Harris, Saegusa and Delmer2010). The latest of these subspecies, P. r. recki, in the time range ca. 1.5–0.4 Ma, was selected for plotting in Figure 12, but is still earlier than the age of SM1. However, remains from North Africa, named as P. iolensis, are regarded as the terminal occurrence of this lineage in Africa (Maglio, Reference Maglio1973) and are plausibly considered the last subspecies of P. recki (P. recki iolensis); the fossils are poorly dated but are believed to extend into the middle and late Pleistocene, possibly as recently as ca. 35 ka (Sanders et al., Reference Sanders, Gheerbrant, Harris, Saegusa and Delmer2010). These remains are sparse, and none is complete enough to allow plotting in Figure 12. The genus entered Europe ca. 0.75 Ma (Lister, Reference Lister2016), where it is recognized as P. antiquus. The specific identity of Palaeoloxodon remains from the Middle East, the geographically intermediate region, is therefore unclear and of interest. Remains from Holon, Israel, ca. 200 ka, were identified as P. antiquus (Davies and Lister, Reference Davies and Lister2007), whereas recently discovered Palaeoloxodon from the Ti’s al Ghadah, Saudi Arabia (ca. 500 ka; Stimpson et al., Reference Stimpson, Lister, Parton, Clark-Balzan, Breeze, Drake and Groucutt2016), have been referred to P. recki; both of these species identifications are based more on location than morphology. Saegusa and Gilbert (Reference Saegusa and Gilbert2008) showed that the cranium from Gesher Benot Ya’aqov, Israel (ca. 0.8 Ma), originally described as P. antiquus (Goren-Inbar et al., Reference Goren-Inbar, Lister, Werker and Chech1994), was more likely, on the basis of cranial morphology, to represent P. recki. The data plotted in Figure 12 suggest that the two species can be separated on dental metrics; although they overlap on each variable, jointly their distributions are separate, at least in the samples available. This is a conservative estimate of their difference, because earlier subspecies of P. recki are less hypsodont still, and analysis of European P. antiquus reveals no significant metric change through its middle and late Pleistocene history (Davies and Lister, Reference Davies and Lister2007). Consideration of Loxodonta (African elephant lineage) for the identity of the SM1 molar is excluded on the basis of its high lamellar count and lack of the diagnostic diamond-shaped “loxodont” lamellar wear figure. Of particular significance, both the lamellar figures and the metric attributes of the SM1 molar are clearly distinct from the two upper molars from the adjacent ‘Ain Soda site (black diamonds in Fig. 12), referred to Elephas hysudricus (Lister et al., Reference Lister, Dirks, Assaf, Chazan, Goldberg, Applbaum, Greenbaum and Horwitz2013).
The upper molar of P. cf. recki also provides some paleoecological information. A recent study showed that dental mesowear on the occlusal surface of proboscidean molars is a reliable indicator of diet (Saarinen et al., Reference Saarinen, Karme, Cerling, Uno, Säilä, Kasiki and Ngene2015). In individuals with a more grass-dominated diet, the dentin platform extends less far below the enamel surface of the lamella, whereas in browsing individuals the dentin is worn more deeply relative to the enamel. This metric is quantified as the angle between the two enamel ridges of the lamella and the dentin platform in between. The relatively well-preserved occlusal surfaces of the SM1 molars show mean mesowear angles (averaged between three central lamellae) of 100.3° (right) and 99.3° (left). These are very steep angles that, by comparison with baseline data in Saarinen et al. (Reference Saarinen, Karme, Cerling, Uno, Säilä, Kasiki and Ngene2015), indicate a strongly browse-dominated diet and hence the availability of tree and/or shrub browse in the vicinity.
Most fragments of elephant molar from the disturbed deposits associated with SM1 could all be refitted or are consistent with having come from the same tooth (Fig. 13), and the majority of postmortem breakage was clearly recent in origin. All fragments were recovered in a small area less than 1 m in diameter. The overall taphonomic condition also was consistent with the Palaeoloxodon molar from undisturbed level 8. Some fragments could be refitted into two large sections of a left lower molar that had not been in occlusion. The anterior portion comprises the talonid, five complete lamellae, and part of the sixth; the posterior portion comprises the remainder of the sixth lamella and three more. The tooth is broken behind, so the total lamellar formula is x9-. Its size indicates that it is a second or third molar (M2 or M3), but it is not possible to determine which.

Figure 13. (color online) Partial left lower (second or third) molar, Elephas cf. hysudricus, disturbed deposits associated with SM1, medial view (disturbed deposits A and B remains). Anterior is to the right. (Three-dimensional images of the molar fragments are available for free download; see the Supplementary Materials).
Because of its unworn state, the enamel loop pattern is not visible on the specimen itself. However, the CT scans (Fig. 14) clearly show the development of lamellar form down the crown, and the revealed pattern does not demonstrate any of the features of Palaeoloxodon as seen in the molar from the intact SM1 deposits. The lamellae are more irregular in form and show only sporadic, variable crenulations. There are no clear median sinuses. In early wear, the lamellae progress from a row of digitations to three roughly equal loops. Of possible candidate species, this pattern is consistent with Elephas hysudricus (compare illustrations in Osborn, Reference Osborn1942, fig. 1203), or conceivably Mammuthus. Metrically, identification as E. hysudricus is corroborated (Fig. 12b); the relatively low crown of the SM molar places its hypsodonty index below the range of available P. recki, P. antiquus, and E. maximus, but consistent with the two available M3 of Siwalik E. hysudricus. The fragmentary M2 or M3 from ‘Ain Soda, referred to E. hysudricus (Lister et al., Reference Lister, Dirks, Assaf, Chazan, Goldberg, Applbaum, Greenbaum and Horwitz2013), is too incomplete to allow measurement of crown height, but its basal lamellar frequency (4.73) is effectively identical to that of the SM molar (4.72). Identity as Mammuthus is considered highly unlikely because a lamellar frequency as low as this is found at the latest in early middle Pleistocene Mammuthus trogontherii (ca. 700–500 ka; Lister and Stuart, Reference Lister and Stuart2010). A sample of European Mammuthus molars roughly contemporaneous with level 8 at SM1 (Marine Oxygen Isotope Stage 7, ca. 200 ka) is plotted in Figure 12b and shows the markedly higher lamellar frequency by this date. The SM molar is therefore referred to Elephas cf. hysudricus.
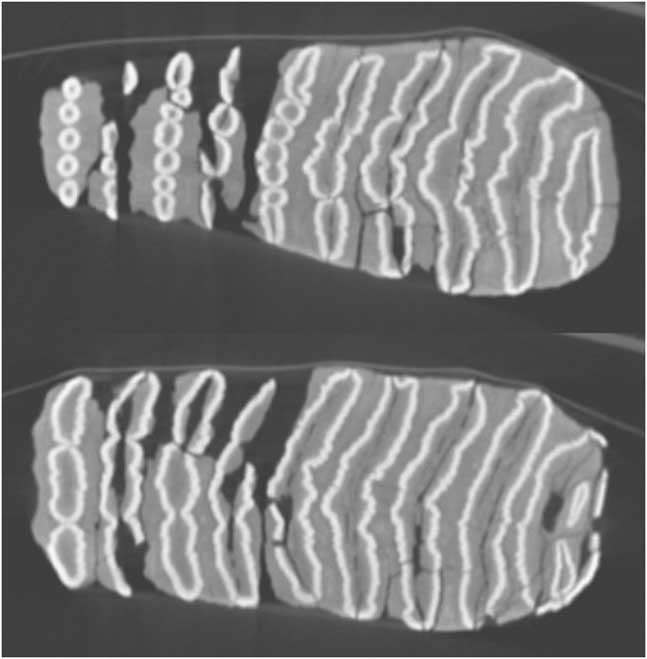
Figure 14. Two CT sections of partial left lower (second or third) molar, Elephas cf. hysudricus, disturbed deposits A and B remains associated with SM1 (molar shown in Fig. 13). Anterior is to the right. Sections (top) and (bottom) are higher and lower in the crown, respectively, and each shows the anterior talonid plus lamellae 1–9.
An additional fragment found associated with this molar could not be refitted with the other sections and, unlike them, was in occlusion (Fig. 15). It is a lower molar fragment comprising one full enamel loop and parts of one anterior and one posterior to it. It is probably the posterior end of a molar with -2x preserved. Because the M2 or M3 described previously was unworn, this fragment cannot belong to the same molar and is unlikely to belong to the immediately preceding molar of the same individual, also because its degree of wear would predict that the anterior part of the M2/M3 would also be in wear. The identity of this fragment as E. hysudricus therefore cannot be assumed and is otherwise indeterminate.
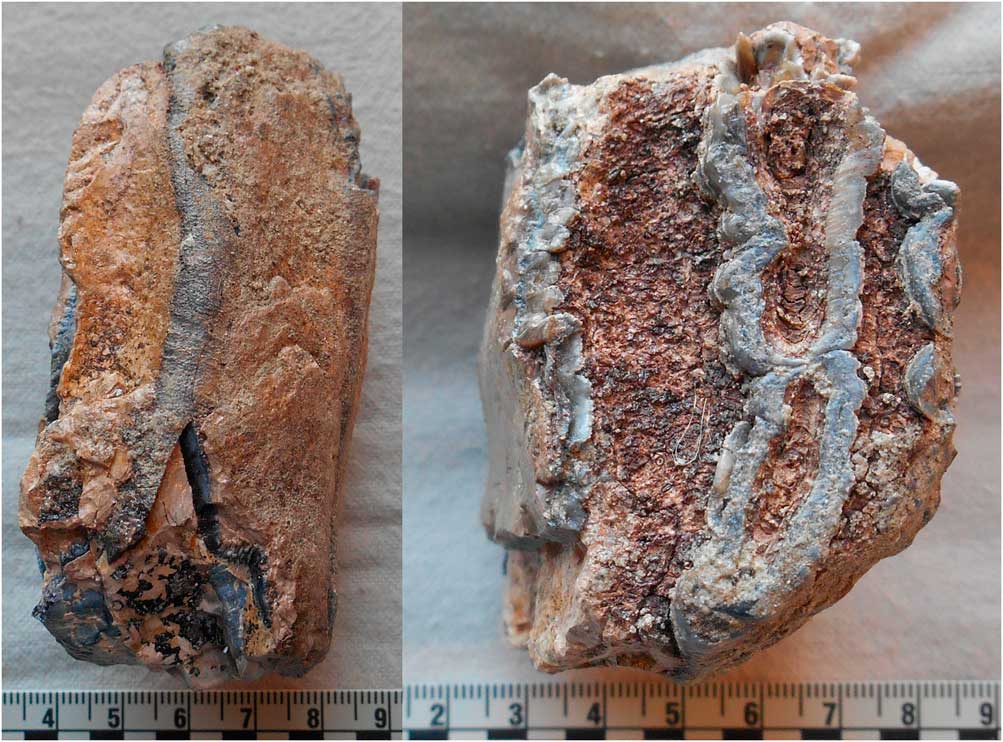
Figure 15. (color online) Lower molar fragment, indeterminate elephantid, disturbed deposits associated with SM1, side (left image) and occlusal (right image) views (disturbed deposits C remains). Anterior is to the right. (A three-dimensional image of the molar fragment is available for free download; see the Supplementary Materials).
The identification of both Palaeoloxodon cf. recki and Elephas cf. hysudricus in the Shishan Marsh deposits is of considerable interest. As discussed previously, African P. recki has been identified until 0.4 Ma, but North African “P. iolensis” extends this range into the late Pleistocene. It seems plausible that the Jordanian remains demonstrate an extended geographic range of this late population of the African Palaeoloxodon lineage.
The fossiliferous deposits of the Siwalik type area of E. hysudricus extend to ca. 600 ka at their younger end (Nanda, Reference Nanda2008), although there are debatable records from the late middle to late Pleistocene elsewhere in India (summarized in Lister et al., Reference Lister, Dirks, Assaf, Chazan, Goldberg, Applbaum, Greenbaum and Horwitz2013), and remains attributed to this species have been identified from the Late Acheulian of both ‘Ain Soda, Jordan, and Ma’ayan Baruch, Israel (Lister et al., Reference Lister, Dirks, Assaf, Chazan, Goldberg, Applbaum, Greenbaum and Horwitz2013). The remains from Shishan Marsh provide an important addition to these records, because although unstratified, they very likely derive from levels 7 or 8 and hence date to the interval ca. 300–100 ka, making them one of the latest records of the species. Because of the long time span of this species, however, and its uncertain terminal date (or date of transition to E. maximus), its presence at Shishan Marsh and ‘Ain Soda does not necessarily indicate a direct correlation between their respective fossiliferous levels. Because of stratigraphic uncertainty, nor can we be sure whether the SM P. cf. recki was contemporary with the E. cf. hysudricus there or at ‘Ain Soda. Their contemporaneity is not excluded; many fossil localities are known with two or more elephantid species apparently coexisting (e.g., Sanders et al., Reference Sanders, Gheerbrant, Harris, Saegusa and Delmer2010).
Equidae
The horse family is represented by multiple species in these levels, and fragmentation made species assignment difficult in many cases. Level 2 includes multiple elements (n=5; MNI=2) of domesticated donkey/ass (Equus asinus). An additional element from this level has been identified to horse (Equus caballus) and therefore is likely the domesticated form of this species; another element from level 2 is of unknown Equus species and is consistent with both E. asinus and E. caballus.
Wild Equus caballus has also been identified in small amounts from levels 7b (partial maxillary molar/premolar) and 8 (partial probable maxillary left third molar, partial unknown maxillary molar, and partial unknown molar), with all teeth in fair to poor condition. Equus caballus is associated with open grasslands but also mixed habitats, including semidesert and steppe-tundra. In the late Pleistocene, the range of wild E. caballus extended throughout the Palearctic from as far west as the Iberian Peninsula into the coastal portions of the Near East and possibly well into North Africa. It also extended into the Nearctic prior to its extinction there at the beginning of the Holocene (and before its reintroduction there as domesticates or feral populations) (Bennett and Hoffmann, Reference Bennett and Hoffman1999). Multiple Epipaleolithic/Natufian sites in the Azraq region include remains attributable only to Equus sp. (Garrard et al., Reference Garrard, Betts, Byrd, Hunt, Colledge, Copeland, Montague and West1988a, Reference Garrard, Colledge, Montague and Hunt1988b; Byrd and Garrard, Reference Byrd and Garrard1990; Richter et al., Reference Richter, Maher, Stock, Allcock, Jones, Martin and Thorne2009; Martin et al., Reference Martin, Edwards and Garrard2010, Reference Martin, Edwards and Garrard2013), and Martin et al. (Reference Martin, Edwards, Roe and Garrard2016) identify E. caballus in the Azraq area. Sites in the surrounding region have yielded remains of E. caballus, including the Natufian and Aurignacian levels at Hayonim (Stiner, Reference Stiner2005), a Mousterian occupation at Wadi Hasa in Jordan (Clark et al., Reference Clark, Lindly, Donaldson, Garrard, Coinman, Schuldenrein, Fish and Olszewski1988), middle Pleistocene at Qesem (Stiner et al., Reference Stiner, Barkaib and Gopher2009), multiple other sites (Davis, Reference Davis1980) in the coastal Levant, and the Wezmeh Cave late Pleistocene carnivore den site in Iran (Mashkour et al., Reference Mashkour, Monchot, Trinkaus, Reyss, Biglari, Bailon, Heydari and Abdi2009). Though this taxon did not typically inhabit true deserts, the relative lushness of the Azraq Oasis would have provided a rich environment in the midst of otherwise more marginal territory.
Remains of a smaller equid consistent with either Equus hemionus or E. hydruntinus were recovered from SM1 levels 7b (a sesamoid in good condition), 7c (a proximal plus shaft portion of a fused left metatarsal, fragmentary and eroded; Fig. 16), and 8 (medial fragment of a fused distal right humerus in good condition). In addition, an M2 and M3 were found adjacent in the nearby exposure SM13 and are tentatively assigned to level 2b. The M2 is most consistent in its enamel fold pattern (smooth and shallow linguaflexid) with the hemione dental pattern (Eisenmann, Reference Eisenmann1986).

Figure 16. (color online) Equus hemionus/hydruntinus left metatarsal, anterior view, level 8, Shishan Marsh 1 (SM1).
Equus hemionus (Asiatic wild ass or onager) still exists in the wild, and its former range extended from the Near East to East Asia in desert and semidesert environments (Groves, Reference Groves1986; Klingel, Reference Klingel1998). Equus hydruntinus (European ass) is now extinct, and its former range extended from Europe to western Asia, including the Near East (Burke et al., Reference Burke, Eisenmann and Ambler2003). Both taxa have been identified from the Azraq region. These finds include the Acheulean deposits at C-Spring in Azraq (Clutton-Brock, Reference Clutton-Brock1970, Reference Clutton-Brock1989; Copeland, Reference Copeland1991), and E. hydruntinus was identified from ‘Ain Soda (Dirks, Reference Dirks1998), although as indicated previously some researchers argue that these taxa may differ only at the subspecies level (Burke et al., Reference Burke, Eisenmann and Ambler2003; Orlando et al., Reference Orlando, Mashkour, Burke, Douady, Eisenmann and Hänni2006). Remains consistent with these two taxa also were recovered from the Late Epipaleolithic at Wadi Jilat 6 and Azraq 18 (Garrard et al., Reference Garrard, Betts, Byrd, Hunt, Colledge, Copeland, Montague and West1988a, Reference Garrard, Colledge, Montague and Hunt1988b; Byrd and Garrard, Reference Byrd and Garrard1990). Equus hemionus also has been identified from the Kebaran at Hayonim and Meged in the coastal Levant (Stiner, Reference Stiner2005) and was highly abundant in the late Pleistocene deposits at Palegawra Cave in Iraq (Bakken, Reference Bakken2000). Equus hydruntinus has been identified from the middle Pleistocene at Qesem Cave (Stiner et al., Reference Stiner, Barkaib and Gopher2009), from Lower Mousterian levels at Tabun and Qafzeh (Davis, Reference Davis1980), and from the Kebaran at Ein Gev I (Davis, Reference Davis1974; Marom and Bar-Oz, Reference Marom and Bar-Oz2008) in the coastal Levant.
Metrical analysis of the partial left metatarsal from level 7c (Fig. 17 and Table 5) of the proximal end indicates that the size much smaller than E. caballus and consistent with specimens assigned to both E. hemionus (specifically E. hemionus onager) and E. hydruntinus (Eisenmann and Beckouche, Reference Eisenmann and Beckouche1986). Its poor preservation does not allow for fuller metrical or morphological comparison. The tooth fragments and other elements also were poorly preserved.

Figure 17. Proximal metatarsal measurements diagram (redrawn after Eisenmann and Beckouche, Reference Eisenmann and Beckouche1986, p. 131) for Equus; the numbers correspond to the measurements in Table 5.
Table 5. Comparison of measurements of Equus left metatarsal from Shishan Marsh 1 (SM1), Pleistocene level 7c. All comparative data and measurement descriptions of the proximal end follow Eisenmann and Beckouche (Reference Eisenmann and Beckouche1986). All measurements are in millimeters, and the SM1 measurements are approximate because of postmortem erosion.
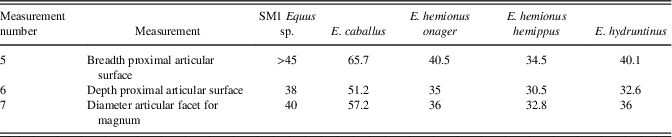
Stephanorhinus hemitoechus
Multiple tooth (molar or premolar) fragments (Fig. 18) have been identified to Rhinocerotidae, and these are all morphologically consistent with steppe rhinoceros (Stephanorhinus hemitoechus), the only rhinoceros species currently known from the Azraq region at this time. This species was two-horned, with hypsodont molars and relatively short limbs (Fortelius et al., Reference Fortelius, Mazza and Sala1993). There is much morphological overlap with other species, including S. etruscus, S. hundsheimensis, and S. kirchbergensis (Fortelius et al., Reference Fortelius, Mazza and Sala1993; Lacombat, Reference Lacombat2006; Ballatore and Breda, Reference Ballatore and Breda2013), but the limited, fragmentary materials available from SM1 do not allow a detailed analysis. The identified remains come from levels 7b (n=11) and 8 (n=6), and both levels have long bone fragments consistent with megafauna of this size or larger (bovid size class V, which also includes the remains of Elephantidae taxa identified). The largest and most intact fragment comes from level 7b and is the buccal enamel portion with some dentin intact of a probable left M2 molar (Fig. 18); the distinctive paracone fold (Ballatore and Breda, Reference Ballatore and Breda2013) is visible.

Figure 18. (color online) Molar fragment (probable left M2) of Stephanorhinus hemitoechus, level 7b, Shishan Marsh 1 (SM1).
The fossil history of this Palearctic species in the Near East extends back to ca. 1.2 Ma (Bar-Yosef and Belmaker, Reference Bar-Yosef and Belmaker2011) and persists at least until the Kebaran at Hayonim (Stiner, Reference Stiner2005), with the species having gone extinct before the Holocene (Groves, Reference Groves1983; Uerpmann, Reference Uerpmann1987; Cerdeño, Reference Cerdeño1998). Its range included Europe, Asia, and North Africa (Cerdeño, Reference Cerdeño1998; Pandolfi and Tagliacozzo, Reference Pandolfi and Tagliacozzo2015). Stephanorhinus hemitoechus (Dicerorhinus hemitoechus) or remains consistent with this species also have been identified from the Acheulean deposits of the nearby sites of C-Spring (Clutton-Brock, Reference Clutton-Brock1970, Reference Clutton-Brock1989; Copeland, Reference Copeland1991) and ‘Ain Soda (Dirks, Reference Dirks1998; Lister et al., Reference Lister, Dirks, Assaf, Chazan, Goldberg, Applbaum, Greenbaum and Horwitz2013). Sites in the Levantine region that have yielded remains of this species also include the Mousterian deposits at Qafzeh (Bouchud, Reference Bouchud1974) and the middle Pleistocene deposits of Qesem Cave (Stiner et al., Reference Stiner, Barkaib and Gopher2009), with multiple rhinoceros taxa identified from Pleistocene deposits at Ubeidiya and other Levantine sites (Bar-Yosef and Tchernov, Reference Bar-Yosef and Tchernov1972). Sites yielding this species in the broader region include the late Pleistocene carnivore den Wezmeh Cave in Iran (Mashkour et al., Reference Mashkour, Monchot, Trinkaus, Reyss, Biglari, Bailon, Heydari and Abdi2009).
Stephanorhinus hemitoechus is associated with steppe habitats and likely fed on grasses and forbs (Rivals and Lister, Reference Rivals and Lister2016). Both types of forage would have been abundant in the broader Azraq Oasis environment (Woolfenden and Ababneh, Reference Woolfenden and Ababneh2011). Blood proteins matching Rhinocerotidae were found on three stone tools tested from the Pleistocene levels at SM1 (Nowell et al., Reference Nowell, Walker, Cordova, Ames, Pokines, Stueber, DeWitt and al-Souliman2016), supplying direct evidence that this species was exploited by the hominins living there, either as hunted or scavenged prey (see Paleoecology of SM1, below).
Sus scrofa
A single element (partial pelvis) was identified to pig (Sus scrofa) in level 2, so the species represented likely is the domesticated form and not wild boar, based on biostratigraphy. Consumption of pig meat sometimes occurs among nonreligious members of the Druze in other areas (Grantham, Reference Grantham1995), and this group has a large presence in modern Azraq; foreign visitors to the region also may have deposited these remains. Wild boar formerly inhabited the Azraq region, as at the adjacent Azraq Epipaleolithic site of ‘Ayn Qasiyya (Richter et al., Reference Richter, Maher, Stock, Allcock, Jones, Martin and Thorne2009; Jones and Richter, Reference Jones and Richter2011) and Wadi Jilat 6 (Garrard et al., Reference Garrard, Betts, Byrd, Hunt, Colledge, Copeland, Montague and West1988a, Reference Garrard, Colledge, Montague and Hunt1988b; Martin et al., Reference Martin, Edwards and Garrard2010), and it is known from coastal Levant sites, as at the Mousterian of Amud (Rabinovich and Hovers, Reference Rabinovich and Hovers2004) and other sites in the region (Edwards et al., Reference Edwards, Bourke, Colledge, Head and Macumber1988; Edwards, Reference Edwards1991; Bar Oz et al., Reference Bar-Oz, Dayan and Kaufman1998; Stiner, Reference Stiner2005; Stiner et al., Reference Stiner, Barkaib and Gopher2009). This species can inhabit semiarid regions and was formerly widespread in nearby areas (Harrison and Bates, Reference Harrison and Bates1991), although it avoids true deserts (Uerpmann, Reference Uerpmann1987).
PALEOECOLOGY OF SM1
Because of the poor overall preservation of the faunal remains from the Pleistocene levels at SM1, only limited paleoecological and paleoenvironmental reconstruction can be inferred from these data, especially given the likely subtraction from the faunal record of the smaller vertebrate taxa. The Pleistocene environment indicated by these species is variable, which is not surprising given the unique ecological setting of a lush wetlands environment, expanded seasonally by rainfall into shallow pools (‘qa), in the middle of an otherwise arid basin. Although Bos primigenius is associated with more closed habitats, it is primarily a grazer (van Vuure, Reference van Vuure2003). The equid taxa are associated with grasslands and also semideserts. The diet of the probable Gazella species present (G. subgutturosa) consists largely of grasses and perennial dwarf shrubs, also indicating a mostly open environment. Analysis of Stephanorhinus hemitoechus dental wear patterns indicates that feeding may have been adapted toward graze-dominated mixed feeding (van Asperen and Kahlke, Reference van Asperen and Kahlke2015; Rivals and Lister, Reference Rivals and Lister2016). Stable isotope and dental wear analysis generally indicate a graze-dominated diet for P. recki (Lister, Reference Lister2013; Saarinen et al., Reference Saarinen, Karme, Cerling, Uno, Säilä, Kasiki and Ngene2015), although the morphology and occlusal wear of the Azraq Palaeoloxodon recki molars indicate a strongly browse-dominated diet from trees and/or shrubs at this locality. The overall Azraq habitat therefore may have been most similar to a savanna ecosystem, with a mixture of open grassland/shrub habitats and more closed vegetation along the wetlands margins.
The mammalian faunal remains from SM1 may have been hunted by the hominins exploiting the marsh environment at that time. The nearby site of ‘Ain Soda has been interpreted as a butchery site (Rollefson et al., Reference Rollefson, Quintero, Wilke, Schnurrenburger, Low and Watson1997a, Reference Rollefson, Schnurrenburger, Quintero, Watson and Low1997b; Lister et al., Reference Lister, Dirks, Assaf, Chazan, Goldberg, Applbaum, Greenbaum and Horwitz2013). Direct evidence of butchery is lacking on the faunal remains from SM1, possibly attributable in part to their generally poor preservation and loss of original surfaces. Other evidence of butchery at SM1 has been recovered, however, in the form of preserved blood protein residues on multiple tested stone tools (Nowell et al., Reference Nowell, Walker, Cordova, Ames, Pokines, Stueber, DeWitt and al-Souliman2016; Walker, Reference Walker2017). Using crossover immunoelectrophoresis, 20 tools from levels 7b, 7c, and 8 at SM1 tested positive for elephant (3), rhinoceros (3), duck (3), horse (5), camel (3), and bovine (3). The results were such that close relatives of the modern species could be represented; thus, modern rhinoceros was used to test for Stephanorhinus hemitoechus. The stone tool types tested were chosen because they displayed pronounced evidence of wear (microflaking, rounding, and polish as documented through low and high power microscopy) on their cutting edges. The tools were typical of the Levantine Lower Paleolithic (scrapers, utilized flakes, Levallois points, and bifaces) made from local flint. The site therefore has direct evidence of butchery and exploitation of these taxa, spanning size class V ungulates (Stephanorhinus hemitoechus, Palaeoloxodon recki, and Elephas hysudricus) to smaller taxa in size classes II–IV and waterfowl. As the water margin attracted these faunal species, the location would have been suitable for preying on them as they drank or exploited the lush vegetation or other resources. The wetlands margins also may have served to slow large ungulate prey and make them more easily ambushed, while the water body itself eliminated one direction of escape. The SM1 faunal assemblage therefore need not have undergone any transportation, selective or otherwise, by the hominins, as the animals may have been killed and processed in this location. The accumulation of some of the faunal remains in this location also may have been because of mortality from other sources, but the artifactual and blood protein evidence makes it clear that carcass processing in general occurred in this area.
Hunting along the wetlands margins may have brought the hominins into direct competition with the other large predators in the oasis area as the latter taxa also relied on the concentration of prey along the water margin to increase their chances of hunting success. Large carnivores known from the region include Panthera leo identified from SM1, as well as the additional unknown large carnivore that is consistent with Crocuta crocuta. Panthera pardus and Acinonyx jubatus also may have inhabited the greater wetlands environment and surrounding territory, along with smaller carnivores including Canis aureus and Hyaena hyaena. Although the smaller carnivores likely were little direct threat to hominins (except for competition as scavengers for the same resources), the pack-hunting techniques of the lions (Schaller, Reference Schaller1972) and spotted hyenas (Kruuk, Reference Kruuk1972) may have necessitated specialized predator-avoidance responses on the part of the hominins (Kruuk, Reference Kruuk2002). This likely included group cooperation of hominins during hunts or carcass processing, which would have been necessary regardless to take the largest prey species. The dense vegetation surrounding the water margins also would have favored ambush predators like felids, so hominins may have been at an even greater disadvantage. Interaction with other predators also may have included competition for fresh carcasses killed by any species or any natural deaths in the environment. It is likely, however, that adult Palaeoloxodon recki, Elephas hysudricus, and Stephanorhinus hemitoechus were largely predation-proof from lions and other large carnivores, and hominins may have been the only predator capable of hunting species this large successfully. Their predation repertoire could have included ambush tactics of their own along the water margin, with predation, butchery, and some consumption occurring at the SM1 and ‘Ain Soda sites. Habitation, especially during sleep hours, may have been less dangerous in locations more distant from the constant large mammal traffic of the wetlands margins. The wetlands margins would have been a focus of activity for many species, and additional research in this area is planned to examine more fully the interactions of hominin taxa with this environment.
SUPPLEMENTAL INFORMATION
Multiple three-dimensional images of the materials (all Proboscidea molars described previously and the Camelus left calcaneus) are available for free download from the senior author: https://doi.org/10.1017/qua.2018.113. Use of these files must follow the restrictions outlined in the accompanying document.
ACKNOWLEDGMENTS
The authors thank Judith Chupasko and Mark Omura of the Mammalogy Department, Peabody Museum, Harvard University (Cambridge, MA) for their access to collections and research help. The authors also thank Eileen Westwig at the Division of Vertebrate Zoology and Judy Galkin at the Division of Paleontology at the American Museum of Natural History (New York) for their kind access to and assistance with comparative collections. AML thanks Advait Jukar for the loan of E. hysudricus data, and Juha Saarinen for mesowear measurement. The CT scanning was performed by the Department of Radiology, Boston Medical Center, with special thanks to Stephan Anderson, Margaret Lavoye, Christine Seay, Mark Pomerleau, Bindu Setty, Mohamad Abdal Kader, Rutuparna Sarangi, and Nicholas Wilson. The authors also thank the Department of Antiquities of Jordan for permission to conduct our research, especially Aktham Oweidi, Ahmad Lash, and Wesam Esaid; Hazem Hreisha and the staff of the Azraq Wetland Reserve; Dr. Barbara Porter and the staff at the American Center of Oriental Research, Amman; Amer Al-Souliman, Stuart Lipkin, Jade de la Paz, Alexandra Maller, Jeremy Beller, and John Murray for their participation in the excavations; and the two peer reviewers for their helpful comments. Dr. Victoria Reed provided translation assistance. The present research was fully funded by an Insight Grant from the Social Sciences and Humanities Research Council of Canada.