I. INTRODUCTION
Trisodium hexachlorometalates (Na3
MCl6, M = metal ion) were reported to crystallize in three crystal structure types namely the Na3AlF6 (cryolite) structure type (for M = Dy, Er, Ho, Lu, Sc, Ti, Tm, Y, Yb) (space group P21/n), the Na3CrCl6 structure type (for M = Cr, In, Mo, V) (space group P
$\bar 3$
1c), and the Na3GdCl6 structure type (M = Eu, Gd, Tb) (space group R
$\bar 3$
H) (an overview and related literature can be found in Table I). Interestingly, another trisodium hexachlorometalate, trisodium hexachlororhodate (Na3RhCl6) was reported in a diffraction study by Krylov et al. (Reference Krylov, Danilov, Stepareva and Kotlyar1983) (ICDD PDF4 + ID # 00-036-0754); however, neither the crystal structure nor the indexing of the reported X-ray diffraction (XRD) pattern was given by them.
Table I. Overview of Na3MCl6 (M = metal ion) compounds and related literature.

Here, the crystal structure of Na3RhCl6 was examined using laboratory X-ray powder diffraction.
II. EXPERIMENTAL
A. Sample preparation
Commercially available trisodium hexachlororhodate(III) was bought from the Sigma-Aldrich Chemie GmbH (Taufkirchen, Germany). The bright red powder was ground in an agate mortar with an agate pestle to a fine powder and subsequently filled in a quartz capillary of 0.5 mm diameter (Hilgenberg GmbH, Malsfeld, Germany). The dehydration of the sample was done by mounting the capillary on the diffractometer and by heating it for half an hour at a constant temperature of 350 °C with an MRI capillary heater. The heating and the cooling rate from room temperature to 350 °C and back was 0.5 °C/s. After the heat treatment, the color of the powder turned into a darker red.
B. XRD data collection
Room temperature X-ray powder diffraction measurements of the Na3RhCl6 specimen were carried out using a Bruker D8 Advance diffractometer in Debye–Scherrer geometry. The diffractometer was equipped with a sealed tube Molybdenum X-ray source (operated at 50 kV and 40 mA) and a Ge(220) primary beam monochromator to obtain pure MoKα 1 radiation at a wavelength of λ = 0.7093 Å. Detection of diffracted X-rays was done using a Lynxeye detector. The diffractogram was collected from 2 to 45.0° 2θ with a resolution of 0.01° 2θ and a total integration time of 8 h.
C. Data treatment
Crystal structure determination and subsequent Rietveld refinements of the measured powder diffraction pattern of Na3RhCl6 were performed using the TOPAS 4.2 software from Bruker AXS Inc. (Cheary et al., Reference Cheary, Coelho and Cline2004). The background of the pattern was modeled by Chebyshev polynomials plus five broad Lorentzian peak shapes, whereas phase peaks where modeled by the fundamental parameter approach.
III. RESULTS AND DISCUSSION
The first indexing attempts (Coelho, Reference Coelho2003) of the measured powder diffraction pattern at room temperature suggested either monoclinic (e.g. P2, P21), trigonal (e.g. P31c, P3), or orthorhombic (e.g. Ccc2, C2221, Cmc21) space groups for Na3RhCl6. From the suggested solutions, the monoclinic ones provided the smallest volume and the best figure of merit (de Wolff, Reference de Wolff1968); however, subsequent whole powder pattern decomposition refinements (Le Bail et al., Reference Le Bail, Duroy and Fourquet1988) revealed that not all reflections could be satisfactorily modeled. In contrast to the monoclinic solutions, trigonal and orthorhombic solutions (which had an exactly double unit-cell volume compared with the trigonal ones) did only match under the assumption, that two of the observed reflections have to be classified as impurity lines. Subsequent whole powder pattern decomposition refinements of the trigonal solutions unveiled several possible impurity lines. Therefore, possible by-products, which could emerge because of the heating process, were checked. It was found that ordinary sodium chloride (NaCl) could be reasonably fitted by a Rietveld refinement to the impurity reflections. Interestingly, no other crystalline rhodium containing impurity phase could be identified. As the trigonal solutions and the corresponding lattice parameters suggested a close structural relationship to the Na3CrCl6 structure type, Na3CrCl6 was used as starting model for a Rietveld refinement (Rietveld, Reference Rietveld1969), exchanging the chromium ion by a rhodium one. Together with the NaCl phase, a successful Rietveld refinement of all reflections could be performed (Figure 1). Intriguingly in this refinement, anisotropic displacement parameters could be utilized for all atoms within the Na3RhCl6 phase, as the quality of the collected data was insomuch excellent, that the R wp dropped by a significant value of 0.3% compared with the usage of sole isotropic displacement parameters. The reasonability of the values of the anisotropic displacement parameters was subsequently confirmed by drawing ellipsoidal atomic models of the crystal structure (see Figure S2 in the online Supplementary material). The final agreement factors of this refinement are R p = 2.651%, R wp = 3.602%, R B(Na3RhCl6) = 1.309%, R B(NaCl) = 1.232%, and GOF = 2.085 (all agreement factors as defined in TOPAS 4.2). The calculated weight percentage of the Na3RhCl6 phase is wt% = 96.03(24), whereas that of the NaCl impurity could be determined to wt% = 3.97(24). The results of the final Rietveld refinement of the Na3RhCl6 phase (lattice parameters, agreement factors, etc.) are summarized in Table II, whereas atomic positions, occupancies, and anisotropic displacement parameters of this phase are listed in Table III. Selected bond lengths are listed in Table IV and the powder diffraction data are given in Table V. The crystal structure of Na3RhCl6 is presented in Figure 2.

Figure 1. (Color online) Rietveld plot of the measured powder X-ray diffraction data. Two phases, Na3RhCl6 [wt% = 96.03(2)] and NaCl [wt% = 3.97(2)] were refined. At low 2Theta values the background is increased because of amorphous scattering of the quartz capillary.

Figure 2. (Color online) Trigonal crystal structure of Na3RhCl6. (a) View along the crystallographic (110)-direction, (b) view along the crystallographic c-direction.
Table II. Crystal structure data obtained from the Rietveld refinement for Na3RhCl6 at room temperature (303 K).

Table III. Refined atomic coordinates, occupancy, and atomic anisotropic displacement parameters for Na3RhCl6 at room temperature (303 K).

Table IV. Selected bond lengths for Na3RhCl6.

Table V. Powder X-ray diffraction data for Na3RhCl6. Calculated values are from the Rietveld refinement, whereas observed values are determined by single peak fittings. Lines which are uniquely identified as NaCl reflections are omitted here.
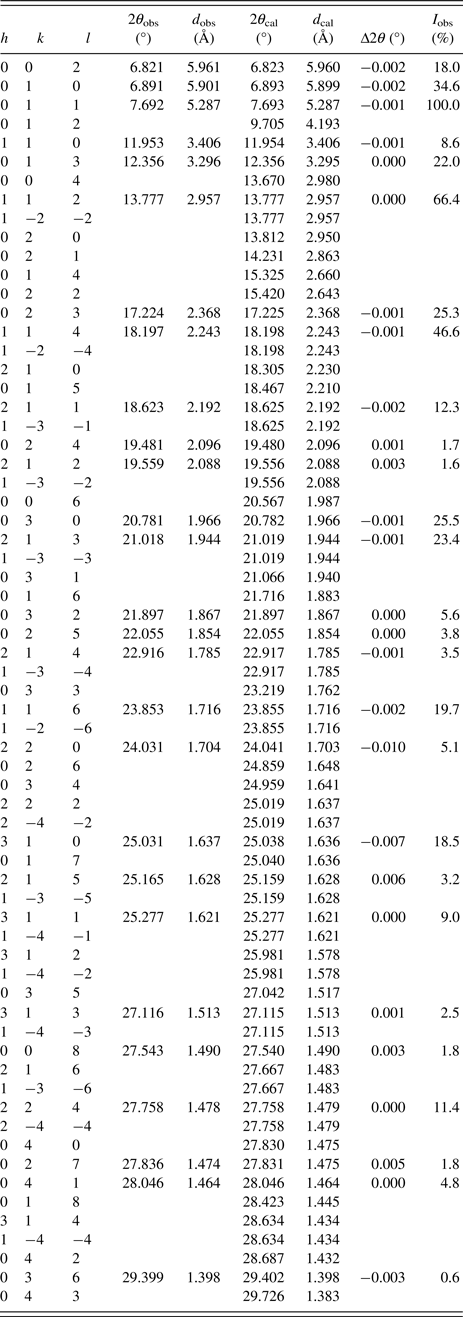
IV. CONCLUSION
Commercially available trisodium hexachlororhodate (Na3RhCl6) was dehydrated and characterized by laboratory X-ray powder diffraction. The crystal structure is isostructural to the Na3CrCl6 structure type with space group P
$\bar 31$
c. Unit-cell parameters are a = 6.8116(1) Å, c = 11.9196(2) Å, V = 478.95(2) Å3, and Z = 2.
SUPPLEMENTARY MATERIAL
The supplementary material for this article can be found at https://doi.org/10.1017/S0885715618000155
ACKNOWLEDGEMENT
The author acknowledges Christine Stefani, Max Planck Institute for Solid State Research (Stuttgart, Germany) for carrying out the powder X-ray diffraction experiments.