Introduction
As in many fields of biology, the primary goals of molecular and biochemical investigations of parasitic helminths have been directed towards characterizing the functional biology of their proteins and peptides. Studies on the contributions of helminth glycans, or carbohydrates, to protein structure and function, to parasite biology and to host–parasite interplay has lagged somewhat, although some pioneering work has called attention to these molecules. Norden and Strand (Reference Norden and Strand1985), for example, observed the relevance of a glycan present in Schistosoma mansoni extracts, strongly recognized by lectin concanavalin A, to the diagnosis of schistosomiasis. Methodological improvements in studies of glycans since the 1980s have allowed researchers to gain key insights into these molecules among various helminths and protozoans. Notably, the roles that glycans play in several aspects of parasitism, including contributions to antigenicity, pathogenicity, signalling, recognition, attachment and evasion of host defences, are being progressively taken into consideration. In this review we have compiled information about glycans of a wide range of parasites, including protozoans and helminths, discussing their involvement in pathogenicity, host–parasite interactions and their potential application for the development of diagnostic methods.
Glycans: an overview
Carbohydrates are organic molecules consisting of carbon, hydrogen and oxygen. These molecules are commonly called saccharides, glycans or sugars. Besides their central role in metabolism, carbohydrates are essential components of a broad range of biological processes that occur in eukaryotes and prokaryotes, including cell recognition, signalling and interaction, fertilization, virus invasion and replication and host–pathogen interactions (Handel et al., Reference Handel, Johnson, Crown, Lau and Proudfoot2005; van Liempt et al., Reference van Liempt, van Vliet, Engering, García Vallejo, Bank, Sanchez-Hernandez, van Kooyk and van Die2007; Fincher, Reference Fincher2009).
Glycans are often bound to proteins and lipids and the modified molecules are identified collectively as glycoconjugates. Proteins are glycosylated through complex biochemical pathways reliant on sequential action of a variety of enzymes, mainly glycosidases and glycosyltransferases. The attachment of oligo- or polysaccharides to the polypeptide structure is the most frequent post-translational modification observed in all living organisms (Spiro, Reference Spiro2002). By modifying the form of glycan linkage, and the structure of the glycan itself can directly influence the properties of a glycoprotein (Cipollo et al., Reference Cipollo, Awad, Costello and Hirschberg2005; Varki, Reference Varki2017).
In eukaryotic cells, the several reactions necessary for protein glycosylation are compartmentalized in the endoplasmic reticulum and Golgi apparatus. In these organelles, key glycosylation enzymes may generate a wide diversity of structures. N- and O-glycosylation are the two major forms of protein glycosylation. During these processes, carbohydrates are attached to specific glycosylation sites in the protein backbone. For N-glycosylation, the first residue of N-acetylglucosamine (GlcNAc) of a chitobiose core (Man3GlcNAc2) is attached to an asparagine (Asn) residue of a consensus sequence composed of Asn-X-serine/threonine (Ser/Thr) (where X can be any amino acid except proline). For O-glycosylation, in most instances, a residue of N-acetylgalactosamine (GalNAc) is attached firstly to Ser/Thr residues of mucin and mucin-like proteins (Haslam et al., Reference Haslam, Morris and Dell2001). However O-glycans with mannose, GlcNAc or fucose residues occupying the first position are also observed in glycoproteins (Thaysen-Andersen and Packer, Reference Thaysen-Andersen and Packer2014). Following this step, a residue of Gal(β1-3) or GlcNAc(β1-6) is added into the first GalNAc giving rise to the core type 1 [Gal(β1-3)GalNAc] or type 2 [GlcNAc(β1-6)GalNAc] glycans, respectively. Subsequent additions of different monosaccharides or other glycan modifications may happen to the non-reducing termini, in specific configurations (Spiro, Reference Spiro2002; Varki, Reference Varki2017). The availability of enzymes, carbohydrates and the characteristics of the protein undergoing glycosylation will define the repertoire of glycans that a specific protein, cell or organism will express. An individual protein can contain either N- or O-glycans, or a combination of both (Haslam et al., Reference Haslam, Morris and Dell2001; Thaysen-Andersen and Packer, Reference Thaysen-Andersen and Packer2014).
Parasite glycans and the host–parasite relationship
The mechanisms of protein glycosylation are highly conserved among eukaryotes, despite the genetic diversity of member taxa. Nonetheless, structural variation within the glycan cores and termini of glycoproteins and glycolipids of parasites have been observed (Fig. 1) (Haslam et al., Reference Haslam, Morris and Dell2001; Hokke et al., Reference Hokke, Deelder, Hoffmann and Wuhrer2007). Therefore, is not surprising that diverse eukaryotic parasites were observed to express a wide diversity of glycans or glycoconjugates containing distinctive and unusual monosaccharides residues or terminal modifications (caps), or even oligosaccharides linked in rare configurations (Table 1). The generation of such specific molecules will depend on the cellular glycosylation machinery, which comprises enzymes such as nucleotide sugar synthases, glycosidases and glycosyltransferases, which perform the necessary glycosylation reactions (Haslam et al., Reference Haslam, Morris and Dell2001; Nyame et al., Reference Nyame, Kawar and Cummings2004). On the other hand, protozoan parasites often display oligosaccharides attached to phosphatidylinositol, forming what is known as glycosylphosphatidylinositol (GPI) anchors at their surface. These anchors are the most important and common post-translational modifications in proteins across the vast diversity of protozoan parasites (Mendonça-Previato et al., Reference Mendonça-Previato, Todeschini, Heise and Previato2003).
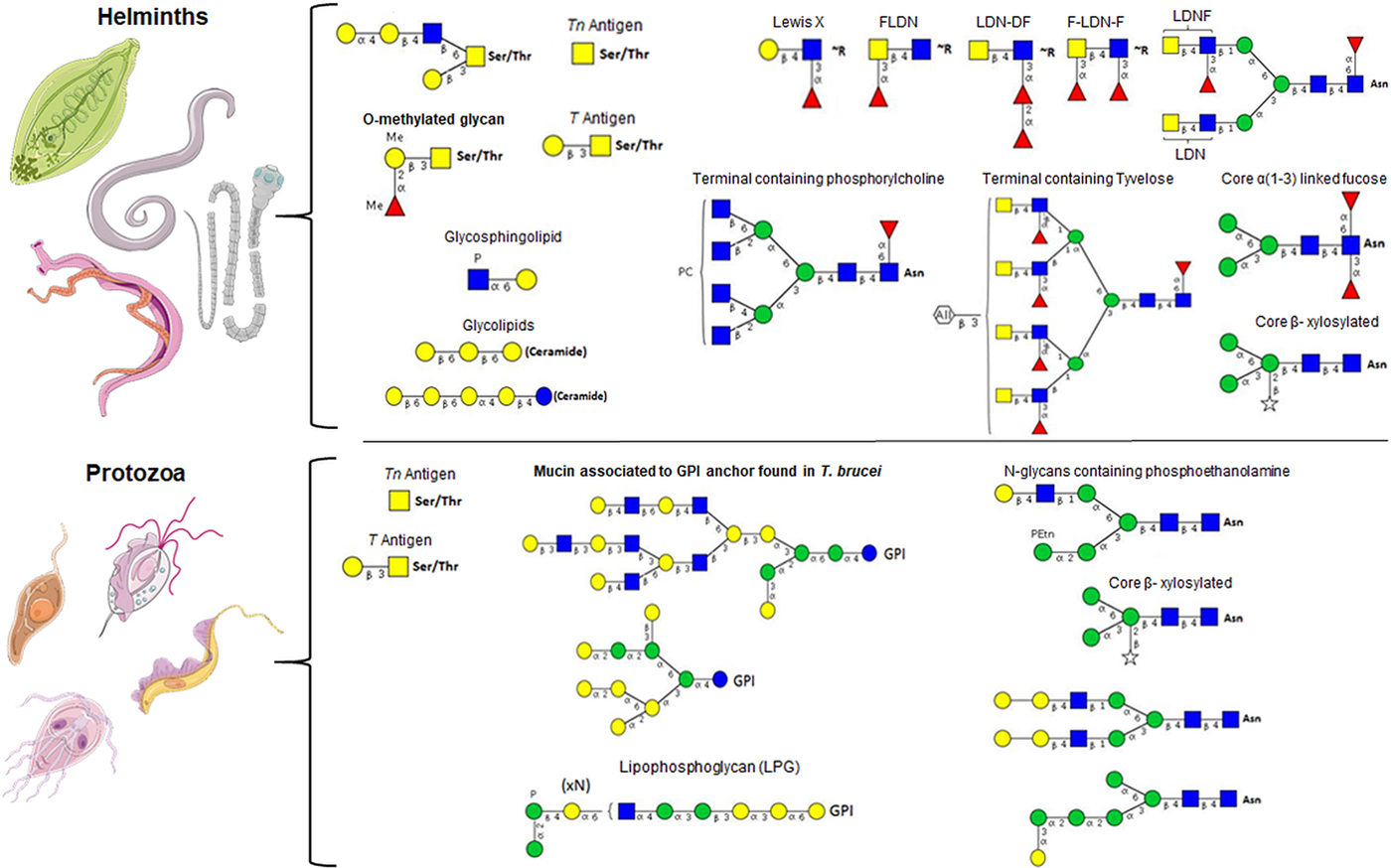
Fig. 1. Main glycan moieties found in helminth and protozoan parasites.
Table 1. Main glycan structures described in helminths and protozoa

a Possible sample contamination.
The diverse glycan repertoire of parasites often include moieties that also form the so-called ‘host-like’ glycans (van Die and Cummings, Reference van Die and Cummings2010). Motifs such as Lewis X [Gal1,4(Fucα1-3)GlcNAc; LeX], LacdiNAc (GalNAcβ1,4GlcNAc; LDN), fucosylated LDN [GalNAcβ1,4(Fucα1-3)GlcNAc; LDNF], truncated O-glycans, known as T antigen (Galβ1-3GalNAcα1-O-Thr/Ser), as well as Tn antigen (GalNAc-O-Ser/Thr), and GPI anchors modified by different glycans are examples of structures found both in parasites and humans. Nevertheless, helminth parasites in general present Tn antigen in greater abundance than do human cells, whereas no sialic acid termini, other than the trans-sialidase-mediated sialylation in trypanosomatids (Schauer et al., Reference Schauer, Reuter, Muhlpfordt, Andrade and Pereira1983), are observed in glycans isolated from parasites (Cummings, Reference Cummings2009; Hokke and van Diepen, Reference Hokke and van Diepen2017).
The glycan repertoire that a parasite presents throughout its life cycle is thought to be of fundamental importance to avoid detection and clearing from the host, ultimately enabling the establishment of a chronic infection. Many helminth-glycans can subvert host immune responses by suppressing it or by inducing T-helper 2 (Th2) type response, activate T regulatory cells (Tregs) and alternatively activate macrophages (Sher et al., Reference Sher, Pearce and Kaye2003; Maizels et al., Reference Maizels, Balic, GomezEscobar, Nair, Taylor and Allen2004; Tundup et al., Reference Tundup, Srivastava and Harn2012). To mimic host glycan is, therefore, a parasite strategy for survival. The mimicked antigens modulate immune responses through direct interaction with receptors on the surface of antigen-presenting cells (APCs), such as dendritic cells (DCs) and macrophages, presenting themselves as ‘self-like’ molecules and not as foreign antigens, consequently preventing the host from attacking the parasite (van Die and Cummings, Reference van Die and Cummings2010).
Indeed, during the development and differentiation of the parasites within their mammal hosts, DCs are exposed to various parasite-derived antigens that modulate their functions and maturation (Linehan et al., Reference Linehan, Coulson, Wilson, Mountford, Brombacher, Martínez-Pomares and Gordon2003; Van Liempt et al., Reference van Liempt, van Vliet, Engering, García Vallejo, Bank, Sanchez-Hernandez, van Kooyk and van Die2007; Terrazas et al., Reference Terrazas, Terrazas and Gómez-García2010). Such modulation occurs through DC receptors, including the classes of Toll-like (TLR), the C-type Lectin receptor (CLRs) and other lectin receptors, that recognize specific glycan motifs (Akira et al., Reference Akira2006; Diebold, Reference Diebold2009; Terrazas et al., Reference Terrazas, Terrazas and Gómez-García2010). It has been proposed that CLRs induce an intracellular signalling pathway upon the recognition of carbohydrates that might lead to impairment of TLR-mediated signalling (Geijtenbeek and Gringhuis, Reference Geijtenbeek and Gringhuis2009).
Each different class of lectin receptors, including DC-SIGN (dendritic cell-specific ICAM3-grabbing non-integrin), MGL (macrophages galactose-type lectin) and MBL (mannose-binding lectin) have been demonstrated to recognize specific host-like or parasite-specific glycan moieties. DC-SIGN receptors, for example, interact with Lewis-X (Lex), LDNF and high mannose N-glycans that are commonly found in extracts of various parasites (Table 1), but which are also expressed by human cells (Okano et al., Reference Okano, Satoskar, Nishizaki and Harn2001; Geijtenbeek et al., Reference Geijtenbeek, Van Vliet, Koppel, Sanchez-Hernandez, Vandenbroucke-Grauls, Appelmelk and Van Kooyk2003; Gomez-Garcia et al., Reference Gómez-García, Rivera-Montoya, Rodríguez-Sosa and Terrazas2006). Therefore, upon their recognition by DC receptors, TLR-mediated signalling and immune response against the parasite presenting these motifs will be dampened. Even though the mechanism of immune modulation through glycan receptors is not fully understood, it is known that DCs bind and internalize glycans derived from S. mansoni eggs through MGL, mannose receptor and DC-SIGN receptors, ultimately driving a T-helper 2 (Th2) response fundamental for the establishment of infection (Van Liempt et al., Reference van Liempt, van Vliet, Engering, García Vallejo, Bank, Sanchez-Hernandez, van Kooyk and van Die2007).
Alternatively, at the parasite surface, carbohydrates may present as a rigid and resilient layer that aid the parasite in evading cellular immune responses (Taratuto and Venturello, Reference Taratuto and Venturiello1997; Kusel et al., Reference Kusel, Al-Adhami and Doenhoff2007). A classic example of this mechanism is observed during infection with the Trypanosoma brucei species complex in Africa. Trypanosoma encodes many genes belonging to the variant surface glycoprotein (VSG) family of proteins (discussed further below), each of them giving origin to a distinct glycoprotein that will present a unique immune signature to the host (Ferguson, Reference Ferguson1999). Thereby, this parasite can constantly stall immune mediated killing of newly members of the blood-resident population. In the same context, changes of the glycan surface of schistosome cercariae, which completely replace their surface membrane of the tegumentary syncytium after host invasion, is thought to allow Schistosoma to survive and complete its life cycle within its definitive host after skin penetration (Jones et al., Reference Jones, Gobert, Zhang, Sunderland and McManus2004).
Glycoconjugates of protozoan parasites
Trypanosoma
Trypanosoma (Euglenozoa: Kinetoplastida: Trypanosomatidae) is a widespread genus of flagellated parasites of vertebrates and invertebrates. Two species of Trypanosoma primarily cause disease in humans, T. cruzi in the Americas causing Chagas disease and members of the T. brucei species complex in Africa. Two sub-species of the latter, T. brucei gambiense and T. brucei rhodesiense, cause blood infection that eventually reaches the central nervous system causing sleeping sickness in humans and nagana in cattle. Trypanosoma brucei survives freely in the bloodstream of its hosts and, as previously mentioned, is covered by GPI-linked variant surface glycoprotein (VSG). This molecule contains a glycan portion that is immunogenic, and through an intriguing molecular switch, the VSG present in the parasite surface can be replaced randomly and repeatedly by another VSG that will contain a distinct glycan profile. The new VSG will also be expressed in the progeny of the original parasite, produced through asexual reproduction, and the new clonal population will be initially resistant to the host immune response. More than a thousand VSG variants have already been identified (Bangs, Reference Bangs2018).
Remarkably, T. brucei can also vary its glycan profile throughout the multiple morphological forms that constitute its life cycle. Complex N-glycans are absent or expressed at a very low level in procyclic forms found in the vector mid-gut, while trypomastigote forms present in the bloodstream of its mammal host express these glycans in substantial amount (Hwa and Khoo, Reference Hwa and Khoo2000). Additionally, T. brucei has been demonstrated to express moieties containing oligomannose, paucimannose and complex N-linked glycans that contain large poly-N-acetyllactosamine structures.
Poly-N-acetyllactosamine is produced by N-acetylglucosaminyltransferases. A search of the T. brucei genome did not identify any orthologues of conventional N-acetylglucosaminyltransferases, a perplexing result given the abundance of this glycan within the parasite. Damerow et al. (Reference Damerow, Rodrigues, Wu, Güther, Mehlert and Ferguson2014, Reference Damerow, Graalfs, Güther, Mehlert, Izquierdo and Ferguson2016) resolved this conundrum when they characterized two highly divergent genes encoding members of the β3-glycosyltransferase family in T. brucei, namely N-acetylglucosaminyltransferases I and II (TbGnTI and II) (Damerow et al., Reference Damerow, Rodrigues, Wu, Güther, Mehlert and Ferguson2014; Reference Damerow, Graalfs, Güther, Mehlert, Izquierdo and Ferguson2016). Surprisingly, TbGnTI and TbGnTII are not essential for parasite survival in vitro and trypanosomes adapted their β3-glycosyltransferases members to catalyse specific glycosidic linkages (Damerow et al., Reference Damerow, Rodrigues, Wu, Güther, Mehlert and Ferguson2014; Reference Damerow, Graalfs, Güther, Mehlert, Izquierdo and Ferguson2016). These data show essential aspects of Trypanosoma protein glycosylation that may assist in identifying druggable targets. Other glycoconjugates of trypanosomatid parasites include various glycoinositolphospholipids (GIPLs), and an unusual GPI anchored mucin. Trypanosoma cruzi O-glycans contain a quite unique mix of GlcNAc, galactose, galactopyranose, galactofuranose and other linked carbohydrates residues (Todeschini et al., Reference Todeschini, da Silveira, Jones, Wait, Previato and Mendonça-Previato2001). In addition, T. cruzi, present an unusual glycan with phosphate attached to Galp terminal residues is found in the gp72 glycoprotein of the epimastigote form possibly having a role in inhibiting parasite to differentiate into metacyclic trypomastigote stage (Allen et al., Reference Allen, Richardson, Mehlert and Ferguson2013).
Although trypanosomatids do not synthesize sialic acid, they do express trans-sialidase (TS) enzymes that catalyse the transfer of sialic acid from host glycoconjugates onto the parasite surface (Costa et al., Reference Costa, Franchin, Pereira-Chioccola, Ribeirão, Schenkman and Rodrigues1998), an unique ability that is thought to be critical for the success of the infection and virulence of the parasite (San Francisco et al., Reference San Francisco, Barría, Gutiérrez, Neira, Muñoz, Sagua, Araya, Andrade, Zailberger, Catalán, Remonsellez, Vega and González2017). Indeed, the activity of TS seems to guarantee the survival of T. cruzi by suppression of host responses, as the sialylated glycans on the parasite surface interact with DC receptors to suppress response, mainly via the lectin receptor sialic acid-binding protein (Siglec-E) (Erdmann et al., Reference Erdmann, Steeg, Koch-Nolte, Fleischer and Jacobs2009). This interaction leads to lower production of IL-12 and IFN-γ. Vaccines against T. cruzi based on recombinant TS have demonstrated 60% efficacy in experimental infections in A/As rats (Pereira-Chioccola et al., Reference Pereira-Chioccola, Costa, Ribeirão, Soares, Arena, Schenkman and Rodrigues1999). Similarly, Hoft et al. (Reference Hoft, Eickhoff, Giddings, Vasconcelos and Rodrigues2007) used only the enzymatic domain of TS to formulate another vaccine that showed what the author considered a good protection against the acute T. cruzi infection, a response also maintained during the chronic phase. In addition, Hoft and colleagues demonstrated that intranasal delivery of a soluble recombinant TS induced both TS-specific CD4(+) and CD8(+) T cells associated with protective immunity, results further confirmed by Giddings et al. (Reference Giddings, Eickhoff, Sullivan and Hoft2010). Even though these results are promising, further vaccine trials still necessary to guarantee that TS is a suitable vaccine candidate to Chagas disease.
The immune modulation observed during Chagas disease is dependent on the genetic backgrounds of both host and parasite (Terrazas et al., Reference Terrazas, Terrazas and Gómez-García2010). By expressing antigens, among them glycoconjugates that target DCs receptors, T. cruzi has developed an important strategy for immune evasion that unbalances the host–parasite relationship at various levels depending on the virulence of the parasite strain. In general, T. cruzi infection is characterized by reduced levels of pro-inflammatory cytokines, such as IL-12 and TNF-α and a lack of mature DCs (Alba Soto et al., Reference Alba Soto, Mirkin, Solana and González Cappa2003). This last effect is one observed on splenic and marrow-derived DCs when the cells were exposed to parasite-GIPLs, indicating that this glycoconjugate plays a primary role in modulating the host cellular response (Brodskyn et al., Reference Brodskyn, Patricio, Oliveira, Lobo, Arnholdt, Mendonça-Previato, Barral and Barral-Netto2002; Poncini et al., Reference Poncini, Alba Soto, Batalla, Solana and Gonzalez Cappa2008). Trypanosoma cruzi GIPLs were also shown to activate DCs through TLR signalling, which contributed to IFN-β production and clearance of infection (Campos et al., Reference Campos, Almeida, Takeuchi, Akira, Valente, Procópio, Travassos, Smith, Golenbock and Gazzinelli2001; Koga et al., Reference Koga, Hamano, Kuwata, Atarashi, Ogawa, Hisaeda, Yamamoto, Akira, Himeno, Matsumoto and Takeda2006).
Leishmania
The genus Leishmania (Euglenozoa: Kinetoplastida: Trypanosomatidae) includes over 20 species able to infect humans, causing different forms of leishmaniasis endemic in 97 countries. Forms of the disease include the potentially fatal visceral (kala-azar) leishmaniasis, the disfiguring cutaneous leishmaniasis and mucocutaneous leishmaniasis (WHO, 2017).
The complex set of glycoconjugates forming the glycocalyx covering Leishmania cells have been extensively studied because of their immunomodulatory properties. These molecules may include different types of mucin glycan, GIPLs, GPI-linked carbohydrate, phosphoglycans (PGs) and the group of molecules variously identified as lipophosphoglycans (LPG), lipopeptidophosphoglycan (LPPG) or proteophosphoglycan (PPG) by different authors. Moreover, phospholipids and glycoproteins are found attached to the GPI anchors, while Leishmania presents a LPG-containing mannose residue attached in uncommon positions, among other structural modifications (Descoteaux and Turco, Reference Descoteaux and Turco1999; Guha-Niyogi et al., Reference Guha-Niyogi, Sullivan and Turco2001).
Glycan metabolism in Leishmania species is highly complex. It has been demonstrated that both the terminal oligosaccharide motifs and the carbohydrate chains that decorate the GPIs vary among species and various life-stages of the parasites (Guha-Niyogi et al., Reference Guha-Niyogi, Sullivan and Turco2001). Glycoproteins and LPG also decrease in abundance in the coated surface of promastigotes as they differentiate into amastigotes, so that GIPL is the predominant glycoconjugate in the latter stage (Naderer et al., Reference Naderer, Vince and McConville2004). Intraspecific variation in LPG has been observed from different field isolates (Coelho-Finamore et al., Reference Coelho-Finamore, Freitas, Assis, Melo, Novozhilova, Secundino, Pimenta, Turco and Soares2011). Although still poorly understood, this variable glycan profile could be responsible for the success of Leishmania species in evading and modulating host responses throughout the whole life cycle. An understanding of the variation may assist in resolving specific attributes of infections among different species. For a complete review of the role of LPG in leishmaniasis infection, see Forestier et al. (Reference Forestier, Gao and Boons2015).
To maintain infection in mammals, Leishmania must manipulate DC activity so as to avoid the mature DC phenotype and, therefore, the Th1 pattern of immune response (Reiner and Locksley, Reference Reiner and Locksley1995; Chakir et al., Reference Chakir, Campos-Neto, Mojibian and Webb2003). The strategies the parasites adopt to manipulate the host responses vary among species and strains of Leishmania and are associated with the sets of glycoconjugates expressed by different parasites. L. major inhibits DC motility through the interaction of LPG with cell receptors (Jebbari et al., Reference Jebbari, Stagg, Davidson and Knight2002), while LPGs from L. mexicana reduce IL-12, thus limiting DC activation (Bennett et al., Reference Bennett, Misslitz, Colledge, Aebischer and Blackburn2001; Argueta-Donohue et al., Reference Argueta-Donohué, Carrillo, Valdés-Reyes, Zentella, Aguirre-García, Becker and Gutiérrez-Kobeh2008). Likewise, PGs expressed by this parasite ultimately impaired the ability of DCs to induce Th1 responses (von Stebut et al., Reference von Stebut, Belkaid, Jakob, Sacks and Udey1998; Konecny et al., Reference Konecny, Stagg, Jebbari, English, Davidson and Knight1999). PG produced by L. donovani affects DCs maturation and migration (Tejle et al., Reference Tejle, Lindroth, Magnusson and Rasmusson2008).
Glycoconjugates of Leishmania have also been shown to interact with TLRs. Even though the effect of this interaction on DC modulation is yet to be understood, it was demonstrated that LPG binds to TLR-2 and 4 on macrophages and natural killer cells, leading to a switch in the profile of pro-inflammatory molecules in these host cells that ultimately allows the intracellular parasite to survive (De Veer et al., Reference De Veer, Curtis, Baldwin, Didonato, Sexton, Mcconville, Handman and Schofield2003; Rojas-Bernabe et al., Reference Rojas-Bernabe, Garcia-Hernandez, Maldonado-Bernal, DelegadoDominguez, Ortega, Gutierrez-Kobeh, Becker and Aguirre-Garcia2014). Accordingly, it was suggested that differences in the LPGs found on L. braziliensis and L. infantum surfaces may impact host cell modulation each species is able to stimulate (Ibraim et al., Reference Ibraim, de Assis, Pessoa, Campos, Melo, Turco and Soares2013).
Curiously, in natural infections, LPG does not seem to be highly immunogenic (Goel et al., Reference Goel, Vohra and Varshney1999), although a different study showed that it could at least in part prevent complement-mediated lysis of Leishmania by blocking the binding of the membrane attack complex C5b-9 to the promastigote membrane (Descoteaux and Turco, Reference Descoteaux and Turco1999). However, when preparations of LPG were used to vaccinate rats, the animals developed a protective immune response, verified by the presence of specific anti-LPG serum antibodies (Russell and Alexander, Reference Russell and Alexander1988). Parasites exposed to these antibodies were incapable of infecting the phlebotomine vectors, suggesting at the time that LPGs were promising candidates antigens for transmission-blocking vaccines (Tonui et al., Reference Tonui, Mbati, Anjili, Orago, Turco, Githure and Koech2001).
Further studies with the same molecule revealed that subcutaneous LPG injection failed to induce protection against L. amazonensis in animals, but the intranasal delivery of LPG resulted in protection (Pinheiro et al., Reference Pinheiro, Pinto, Lopes, Guedes, Fentanes and RossiBergmann2005; Reference Pinheiro, Pinto, de Matos Guedes, Filho, de Mattos, Saraiva, de Mendonça and Rossi-Bergmann2007). Although the mechanisms of LPG-mediated protection are still unknown other vaccine formulations have been better characterized. A dual stimulation using a peptidoglycan (PGN) and the soluble leishmanial antigen (SLA) was able to stimulate DCs during experimental visceral leishmaniasis (VL). The vaccinated animals showed a significant decrease in hepatic and splenic parasite burden, and an increased production of nitric oxide (NO) and pro-inflammatory cytokines such as IL-12, IFN-γ and IL-17, that ultimately resulted on increased number of Th17 cells (Jawed et al., Reference Jawed, Majumder, Bandyopadhyay, Biswas, Parveen and Majumdar2016).
Plasmodium
Plasmodium, a genus of parasites belonging to the phylum Apicomplexa within the SAR supergroup of eukaryotes, is the agent of malaria in humans and animals.
The intracellular apicomplexans, Plasmodium species synthesize GPI anchors that contain a highly conserved glycan core composed of a trimannosylglucosaminyl moiety with an additional mannose attached. These anchors are attached to several P. falciparum proteins and constitute 90% of the glycoconjugates identified on this parasite (Gowda and Davidson, Reference Gowda and Davidson1999). Not surprisingly, this glycoconjugate has been strongly associated with various symptoms observed during malaria infection (Schofield et al., Reference Schofield, McConville, Hansen, Campbell, Fraser-Reid, Grusby and Tachado1999; Clark and Schofield, Reference Clark and Schofield2000; Jaurigue and Seeberger, Reference Jaurigue and Seeberger2017). GPI anchors can activate macrophages and cells of the vascular endothelium through several signalling pathways that result in the production of chemical mediators, such as NO, tumour necrosis factor (TNFα) and intracellular adhesion molecules I (ICAM-I). This observation led tp exploration of this molecule as a vaccine target (Schofield et al., Reference Schofield, Hewitt, Evans, Siomos and Seeberger2002), a concept later reinforced by a study that demonstrated that presence of specific antibodies to P. falciparum GPI could neutralize the strong inflammatory response that GPI stimulates (de Souza et al., Reference de Souza, Runglall, Corran, Okell, Kumar, Gowda, Couper and Riley2010).
Indeed, most GPI-vaccines aim to neutralize or decrease the inflammatory response observed during infection rather than produce a sterilizing immunity. Rats immunized with a synthetic GPI based on the P. falciparum anchor displayed high titres of IgG antibodies that neutralized pro-inflammatory effects caused by activated macrophages, despite no significant protection being observed after challenge with P. berghei ANKA. Even though animals immunized did not present a reduction in parasitaemia, the results suggest that GPI anchors have a conserved structure among the different species of Plasmodium (Schofield et al., Reference Schofield, Hewitt, Evans, Siomos and Seeberger2002). Another GPI formulation used as vaccine induced an IgG antibody response able to neutralize the parasite pathogenesis in vitro (Taylor et al., Reference Taylor, Cobb, RittenhouseOlson, Paulson and Schreiber2012). Currently, the P. falciparum GPI vaccine is being tested in phase II clinical trials (see Anchora Pharmaceutical, MA https://www.sbir.gov/sbirsearch/detail/89678).
It is important to highlight here that many studies have shown that P. falciparum produces a restricted glycan repertoire. Technical limitations in isolating sufficient quantities of parasite molecules have rendered it difficult to determine with clarity whether the parasites produce O-glycans or complex N-glycans (Kimura et al., Reference Kimura, Couto, Peres, Casal and Katzin1996; Cova et al., Reference Cova, Rodrigues, Smith and Izquierdo2015). A recent work using a mass spectrometry technique by electron transfer dissociation was able to show different glycoforms of thrombospondin type 1 repeats-containing proteins (TSR) (Swearingen et al., Reference Swearingen, Eng, Shteynberg, Vigdorovich, Springer, Mendoza, Sather, Deutsch, Kappe and Moritz2019). TSRs are implicated in the invasion process of Plasmodium and may be modified with O-linked fucose (O-Fuc) and C-linked mannose (C-Man).
It seems to be certain, however, that in addition to the GPI anchors, P. falciparum produces truncated N-glycans, GlcNAc and GlcNAc2 (Cova et al., Reference Cova, Rodrigues, Smith and Izquierdo2015). The relevance of these glycans in the host–parasite interplay, if any, remains to be elucidated.
Entamoeba histolytica
Entamoeba histolytica (Amoebozoa: Endamoebidae) is a pathogenic amoeba responsible for significant food-borne infections in humans. The species causes intestinal amoebiasis and may affect other organs through disseminated infection. Amoebiasis is among the leading causes of death in the world attributed to a protozoan parasite (Ralston and Petri, Reference Ralston and Petri2011).
The most abundant glycan found in E. histolytica is aggregated into caps on the surface of the cell by the N-glycan-specific, anti-retroviral lectin cyanovirin-N. Complex N-glycans contain α1,2-linked Gal to both arms of small oligomannose glycans, and Gal residues are capped by one or more Glc (Magnelli et al., Reference Magnelli, Cipollo, Ratner, Cui, Kelleher, Gilmore, Costello, Robbins and Samuelson2008). The motile trophozoite stage of the parasite has a coat of lipid-linked glycoconjugates including the LPGs. The Entamoeba LPG has an unusual GPI anchor on its surface, which uses an ethanolamine phosphate bridge to anchor a residue of α-Gal onto the C-terminus of the protein. Several other linear glycans [Glcα1-6(n) Glcβ1-6Gal] are found attached to the LPG via phosphoserine residues (Moody-Haupt et al., Reference Moody-Haupt, Patterson, Mirelman and McConville2000).
The pathogenesis observed during amoebiasis appears to result from the cytotoxic activity of the parasite, which depends mainly on the adhesive interactions between the parasite and the glycoconjugates present on the surface of host cells, and of the interaction of the parasite glycoconjugates with the host immune system. The LPG has been strongly associated with Entamoeba virulence, since specific antibodies to this antigen could prevent trophozoite adhesion to cells and the consequent cytolysis in vitro, and liver abscess formation in experimental infections in mice (Marinets et al., Reference Marinets, Zhang, Guillen, Gounon, Bohle, Vollmann, Scheiner, Wiedermann, Stanley and Duchene1997). The same LPG also appears to have a central role in the first steps of infection, when the adherent E. histolytica trophozoites transfer their LPG to the apical side of host enterocytes causing dysfunction of the tight junctions (Lauwaet et al., Reference Lauwaet, Oliveira, De Bruyne, Bruchhaus, Duche ne, Mareel and Leroy2004). LPGs were demonstrated to interact with TLR receptors and stimulate the production of IL-10, IL-12 and TNF-α (Maldonado-Bernal et al., Reference Maldonado-Bernal, Kirschning, Rosenstein, Rocha, Rios-Sarabia, Espinosa-Cantellano, Becker, Estrada, Salazar-González, López-Macías, Wagner, Sánchez and Isibasi2005) and, together with parasite surface Gal/GalNAc lectins, are considered fundamental for regulating host cell adhesion and cytolysis. Both LPG and Gal/GalNAc lectin were observed to activate and induce maturation of DCs, leading to a Th1 response during amoebiasis (Ivory and Chadee, Reference Ivory and Chadee2007; Vivanco-Cid et al., Reference Vivanco-Cid, Alpuche-Aranda, Wong-Baeza, Rocha-Ramírez, Rios-Sarabia, Estrada-Garcia, Villasis-Keever, Lopez-Macias and Isibasi2007; Aguirre García et al., Reference Aguirre García, Gutiérrez-Kobeh and López Vancell2015).
Several LPGs have been applied in diagnosis tests and vaccines of protozoan infections. Species of Entamoeba and Leishmania can be distinguished by the type of LPG they are expressing (Coelho-Finamore et al., Reference Coelho-Finamore, Freitas, Assis, Melo, Novozhilova, Secundino, Pimenta, Turco and Soares2011). Moreover, LPG identification is suitable for differential diagnosis of the pathogenic E. histolytica from non-pathogenic E. dispar, as it was demonstrated that those LPGs expressed by pathogenic strains of E. histolytica are unique for this species (Moody et al., Reference Moody, Becker, Nuchamowitz and Mirelman1997; Bhattacharya et al., Reference Bhattacharya, Arya, Clark and Ackers2000).
Glycoconjugates in helminth parasites: phylum Nematoda
Trichinella spiralis
Trichinella spiralis (Nematoda: Adenophorea: Trichinelloidea) is the causative agent of trichinellosis in mammals, a disease leading to severe debilitation and pain, and sometimes death. Humans become infected by consuming raw or under-cooked meat of an infected animal. The life cycle is maintained in the wild through predation and meat-scavenging behaviours.
Trichinella spiralis produces many glycans, but one of the most remarkable is a motif containing a tyvelose (Tyv) (3,6-dideoxy-D-Arabino-hexose) residue that is found attached to the glycoprotein TSL-1 (T. spiralis larva-1), present at the surface of the first stage larvae of T. spiralis and its ES products (Reason et al., Reference Reason, Ellis, Appleton, Wisnewski, Grieve, McNeil, Wassom, Morris and Dell1994). Reason and colleagues claimed that a major proportion of the N-glycans antennae of the TSL-1 are capped with Tyv and the uniqueness of this monosaccharide encouraged further studies. This represents an excellent case where a glycan motif that appears to be restricted to an organism renders the molecule as a potentially valuable target for immunodiagnostic tests. Indeed, moved by the previous results, Forbes et al. (Reference Forbes, Appleyard and Gajadhar2004) used a synthetic β-Tyv antigen to develop an enzyme-linked immunosorbent assay (ELISA) that presented a sensitivity of 94.3% and a specificity of 96.7% when applied to the diagnosis of swine trichinosis.
TSL-1 and Tyv have been proposed to have immunomodulatory effects. Niborski et al. (Reference Niborski, Vallée, Fonseca-Liñán, Boireau, Enciso, Ortega-Pierres and Yépez-Mulia2004) demonstrated a Th2 pattern of response during T. spiralis infections. Complementary in vitro experiments using rat mast cells exposed to TSL-1, demonstrated an association of the glycoprotein with increased mRNA levels for IL-4, IL-5, IL-6 and TNFα. During the intestinal (adult) phase of the infection, the TSL-1 antigen is thought to stimulate mast cells in an IgE-independent manner, ultimately leading to increased levels of histamine that could contribute to worm expulsion from the intestine (Yépez-Mulia et al., Reference Yépez-Mulia, Hernández-Bello, Arizmendi-Puga, Fonseca-Liñán and Ortega-Pierres2007). A different study, aiming to understand the role of the glycan portion of TSL-1, demonstrated that mice immunized with tyvelose-BSA develop high levels of IL-5 but not IFN-γ, which reinforced the idea that this glycan motif plays a central role to drive the Th2 response observed during trichinellosis (Goyal et al., Reference Goyal, Wheatcroft and Wakelin2002).
More recently, however, studies focusing on the Tv antigen have become sparse, while other Trichinella glycans are being prospected. A T. spiralis glycoconjugate containing LDNF, for example, was thought to be a suitable target for immunodiagnosis of trichinellosis, showing a sensitivity of 96% and a specificity of 67% in diagnostic tests (Aranzamendi et al., Reference Aranzamendi, Tefsen, Jansen, Chiumiento, Bruschi, Kortbeek, Smith, Cummings, Pinelli and Van Die2011). Interestingly, T. spiralis adult and L1 larvae have multiple antennae decorated with phosphorylcholine (PC)-modified LDNF that is found linked to residues of GlcNAc or GalNAc (Morelle et al., Reference Morelle, Haslam, Olivier, Appleton, Morris and Dell2000). The presence of this modification could explain the low specificity observed in tests using T. spiralis LDNF antigen since PC terminals have been associated with cross-reactivity observed in serological diagnostic tests to Wuchereria bancrofti, Brugia malayi, Onchocerca volvulus and Achanthocheilonema viteae (Dell et al., Reference Dell, Haslam, Morris and Khoo1999; Goodridge et al., Reference Goodridge, Marshall, Else, Houston, Egan, Al-Riyami, Liew, Harnett and Harnett2005).
Haemonchus contortus
Haemonchus contortus (Nematoda: Secernentea: Trichostrongylidae) is a highly pathogenic parasite of sheep and goats. The parasite is an avid blood-feeder in the abomasum of its ruminant hosts. Haemonchus contortus expresses a broad variety of glycans, including motifs containing LDN, LDNF motifs and N-glycans with core α1,3-fucose, which were mainly identified in ES products. A more recent and detailed mass spectrometry analysis of H. contortus N-glycans revealed a dominant trifucosylated Hex3HexNAc2Fuc3 structure containing α1,6- and α1,3-fucose on the proximal core GlcNAc, and a galactosylated distal α1,3-fucose (Table 1). Other motifs displayed galactosylation of the core α1,6-fucose, antennal fucosylation or PC modification (Haslam et al., Reference Haslam, Coles, Munn, Smith, Smith, Morris and Dell1996; Paschinger and Wilson, Reference Paschinger and Wilson2015).
The immunogenicity of glycan motifs of Haemonchus was highlighted by glycan array experiments using samples from lambs vaccinated with parasite ES products. The vaccinated animals presented high levels of IgG antibodies to Gal(α1-3)GalNAc motif that was correlated with the protection observed (van Stijn et al., Reference van Stijn, van den Broek, Vervelde, Alvarez, Cummings, Tefsen and van Die2010). Heim et al. (Reference Heim, Hertzberg, Butschi, Bleuler-Martinez, Aebi, Deplazes, Künzler and Štefanić2015) demonstrated that larval development could be arrested in vitro with the lectin Marasmius oreades agglutinin (MOA), that specifically binds to Gal(α1-3)GalNAc. Larvae exposed to the lectin presented severe malformations in body shape and died quickly, indicating a potential application of MOA for novel chemotherapeutic strategies (Heim et al., Reference Heim, Hertzberg, Butschi, Bleuler-Martinez, Aebi, Deplazes, Künzler and Štefanić2015). A galactose-containing glycoprotein complex (H-gal-GP), purified from the gut membrane of Haemonchus, inspired many studies on vaccination, leading to the first commercial vaccine available to protect sheep against the Barber's pole worm (Kearney et al., Reference Kearney, Murray, Hoy, Hohenhaus and Kotze2016). The success of this vaccine is possibly related to the fact that they use a native H-gal-GP purified from worms recovered from naturally infected sheep, rather than a recombinant protein. Although successful, this native vaccine emphasizes a major limitation in vaccinology, namely, the difficulties in producing recombinant glycoproteins that preserving the glycan configuration of the native antigens.
Angiostrongylus cantonensis
Angiostrongylus cantonensis (Nematoda: Secernentea: Metastrongylidae) is the main causative agent of eosinophilic meningoencephalitis in humans in many parts of the world (Morassutti et al., Reference Morassutti, Levert, Perelygin, da Silva, Wilkins and Graeff-Teixeira2012). The glycan repertoire of A. cantonensis female worms includes complex (Fuc0–1Hex3–5HexNAc3–5), high mannose (Hex5–7HexNAc2) and truncated structures (Fuc0–1Hex3HexNAc2) N-glycans, while O-glycans were not identified in any analyses performed so far (Veríssimo et al., Reference Veríssimo, Morassutti, von Itzstein, Sutov, Hartley-Tassell, McAtamney, Dell, Haslam and Graeff-Teixeira2016). Glycans with terminal containing galactose (Gal) and GalNAc are not commonly observed among nematode parasites and further investigations of the glycan repertoire of Angiostrongylus parasites will help to determine if such moieties identified by Veríssimo et al., are indeed produced by the parasite or a result of cross-contamination with host molecules.
Behn (1997) proposed that glycans play a central role in Angiostrongylus biology considering their involvement with mechanisms of parasite adaptability to different temperatures of its two hosts, which vary from 36–38 °C in the mammal hosts and from 20–28 °C in mollusc hosts. Behn further proposed that a trehalose residue would promote such thermal tolerance, even though this carbohydrate has never been identified in the extracts of this parasite. While such role of trehalose is doubtful in the case of A. cantonensis, it is evident that other nematodes use trehalose for thermotolerance, among them the sealworm Pseudoterranova decipiens (Nematoda: Anisakidae), a parasite infecting animals in cold marine waters (Stormo et al., Reference Stormo, Praebel and Elvevoll2009).
The immunogenicity of A. cantonensis glycans, however, is better demonstrated. Such molecules boost specific anti-glycan antibodies, observed when sera of individuals infected to the Angiostrongylus were tested with the 31 kDa antigen, a complex antigen containing N-glycosylated glycoproteins (Veríssimo et al., Reference Veríssimo, Morassutti, von Itzstein, Sutov, Hartley-Tassell, McAtamney, Dell, Haslam and Graeff-Teixeira2016). Initially, Morassutti et al. (Reference Morassutti, Levert, Perelygin, da Silva, Wilkins and Graeff-Teixeira2012) demonstrated the importance of the glycan portion of the antigen by treating it with meta-periodate, which resulted in an abrogated immune recognition of the 31 kDa spots by sera from infected patients. Further, Veríssimo et al. (Reference Veríssimo, Morassutti, von Itzstein, Sutov, Hartley-Tassell, McAtamney, Dell, Haslam and Graeff-Teixeira2016) confirmed that N-glycans associated with the 31 kDa antigen were the protagonists of this immune response by treating the antigen with the N-glycosidase PNGase F, which removed the carbohydrate moieties. Additionally, heterologous expression of the 31 kDa antigen in different prokaryotic or eukaryotic systems failed to produce an immunogenic recombinant protein (Morassutti et al., Reference Morassutti, Levert, Perelygin, da Silva, Wilkins and Graeff-Teixeira2012).
Glycoconjugates in helminth parasites: phylum Platyhelminthes
Echinococcus
Echinococcus (Platyhelminthes: Cestoda: Taeniidae) is a genus of taeniid tapeworm. Two species (or species complex) are primarily responsible for human echinococcosis, E. granulosus and E. multilocularis. The species cause unilocular and alveolar echinococcosis which are characterized by the presence of cystic lesions containing the infectious protoscoleces. Infection of the intermediate host, a mammal, occurs by the ingestion of eggs that were released with the feces of the canid definitive host.
The hydatid cyst wall of E. granulosus is formed of three layers, the outer pericyst, the middle-laminated layer and the inner germinal layer. The last layer produces the abundant brood capsules which in turn produce multiple protoscoleces. The crude antigen of the hydatid cyst administered at different stages prevents DCs from maturing. The inhibitory effect of the antigen operates through inhibition of CD1a and enhancement of CD86 expression. Furthermore, crude antigen stimulates IL-4 production during antigen presentation by mature DCs, which suggests that E. granulosus stimulates Th2 pattern responses (Rigano et al., Reference Riganò, Buttari, Profumo, Ortona, Delunardo, Margutti, Mattei, Teggi, Sorice and Siracusano2007).
The laminated layer of E. granulosus is a mucin-rich extracellular matrix composed primarily of O-glycan core 1 (Galβ1-3GalNAc), also known as T antigen, and core 2 [Galβ1-3(GlcNAcβ1-6) GalNAc] the Tn antigen (Díaz et al., Reference Díaz, Fontana, Todeschini, Soulé, González, Casaravilla, Portela, Mohana-Borges, Mendonça-Previato, Previato and Ferreira2009). The Galβ1-3 residue of core 1 can be decorated with additional Galβ1-3 residues, generating a linear chain (Galβ1-3Galβ1-3GalNAc), and the elongation of mucin glycans with Galβ1-3 distinguishes E. multilocularis from E. granulosus (del Puerto et al., Reference Del Puerto, Rovetta, Navatta, Fontana, Lin, Moyna, Dematteis, Brehm, Koziol, Ferreira and Díaz2016). Other structures extended with Galβ1-4 are also present, although complex glycans are much less abundant than those containing a non-decorated core (Díaz et al., Reference Díaz, Fontana, Todeschini, Soulé, González, Casaravilla, Portela, Mohana-Borges, Mendonça-Previato, Previato and Ferreira2009).
Khoo et al. (Reference Khoo, Nieto, Morris and Dell1997) analysed the glycan composition of E. granulosus antigen 5 (Ag5) and found small amounts of high mannose and truncated glycan structures. Subsequent analysis of the same antigen by Paschinger et al. (Reference Paschinger, GonzalezSapienza and Wilson2012), identified a range of other N-glycans, among them a moiety containing two antennae with a core of α1,6-fucose and capped by PC. The identification of these additional glycans demonstrated that the permethylation step used by Khoo et al. (Reference Khoo, Nieto, Morris and Dell1997) impairs the detection of PC temini. Other important glycoconjugates have been identified in extracts of Echinococcus. Among these molecules, the O-glycosylated Tn antigen from E. granulosus protoscoleces has been proposed as a biomarker of hydatidoses, since high levels of this antigen are detected in sera from infected patients (Alvarez-Errico et al., Reference Alvarez Errico, Medeiros, Míguez, Casaravilla, Malgor, Carmona, Nieto and Osinaga2001). A synthetic form of Em2(11), the main glycan antigen isolated from the laminated layer of E. multilocularis hydatid cysts, showed high sensitivity and specificity when applied on diagnostic tests (Koizumi et al., Reference Koizumi, Yamano, Schweizer, Takeda, Kiuchi and Hada2011). Together these results reinforce the view that the characterization of glycan produced by different organisms is necessary to improve diagnostic specificity. The benefits of glycan analysis include, for example, our current comprehension of the cross-reactivity commonly observed in diagnostic tests using sera from E. multilocularis, E. granulosus or F. hepatica infected patients, which is now attributed to the glycan moiety, Galβ1-6Gal, attached to molecules produced by all these parasites (Yamano et al., Reference Yamano, Goto, Nakamura-Uchiyama, Nawa, Hada and Takeda2009).
Taenia
Taenia (Platyhelminthes: Cestoda: Taeniidae) is a genus of tapeworms infecting humans and some carnivores. Humans serve as definitive host of two species, T. solium and T. saginata, where intestinal infection by the adult parasite results in taeniasis. Additionally, humans can become infected by ingesting the eggs, commonly of T. solium and rarely of T. crassiceps. In this case, the host will develop cysticercosis in the tissues, specifically in the central nervous system (Hawk et al., Reference Hawk, Shahlaie, Kim and Theis2005).
Different species of Taenia express a species-specific glycan profile. N-glycans containing high mannose and truncated structures were found attached to glycoproteins secreted by T. solium (Haslam et al., Reference Haslam, Restrepo, Obregón-Henao, Teale, Morris and Dell2003), whereas T. crassiceps, produces N-glycans with fucose terminals (Lee et al., Reference Lee, Dissanayake, Panico, Morris, Dell and Haslam2005). Taenia crassiceps carbohydrates play key roles in host immune evasion, either by stimulating Th2 polarization (Gomez-Garcia et al., Reference Gómez-García, Rivera-Montoya, Rodríguez-Sosa and Terrazas2006) or by inducing myeloid-suppressor innate cells (Gomez-Garcia et al., Reference Gomez-Garcia, Lopez-Marin, Saavedra, Reyes, Rodriguez-Sosa and Terrazas2005). Several experiments using metaperiodate helped to show that only intact glycosylated antigens can induce Th2 responses and other immunomodulatory effects such as higher expression of MHC-II. DC exposure to Taenia glycans resulted in abrogated response to TLR ligands, inhibition of IL-15, IL-12 and TNF-α secretion, and downregulation of a chemokine receptor (CCR7) that impairs DC migration (Montero-Barrera et al., Reference Montero-Barrera, Valderrama-Carvajal, Terrazas, Rojas-Hernández, Ledesma-Soto, Vera-Arias, Carrasco-Yépez, Gómez-García, Martínez-Saucedo, Becerra-Díaz and Terrazas2015).
MGL1, a lectin receptor expressed on the surface of APCs, such as mature DC and macrophages, recognizes antigens such as Lex and other motifs containing galactose residues. MGL1 is directly involved with activation of innate immunity, antigen recognition and resistance to T. crassiceps infection. Animals with deficiency of MGL1 receptors are more susceptible to cysticercosis (Montero-Barrera et al., Reference Montero-Barrera, Valderrama-Carvajal, Terrazas, Rojas-Hernández, Ledesma-Soto, Vera-Arias, Carrasco-Yépez, Gómez-García, Martínez-Saucedo, Becerra-Díaz and Terrazas2015), indicating that glycans recognized by MGL1 are important for parasite survival in the host.
Schistosoma
Species of Schistosoma (Platyhelminthes: Trematoda: Schistosomatidae) are blood flukes that cause hepato-intestinal and genitourinary diseases. Glycans from schistosomes are widely studied. In every stage of their life cycles, schistosomes synthesize various glycan motifs containing LDN or its fucosylated form, LDNF (Hokke et al., Reference Hokke, Deelder, Hoffmann and Wuhrer2007). LDN/LDNF containing glycans are commonly attached to glycoproteins and glycolipids and are considered elementary molecules, since they form a base for further modification into more complex glycans (Khoo et al., Reference Khoo, Sarda, Xu, Caulfield, McNeil, Homans, Morris and Dell1995; Wuhrer et al., Reference Wuhrer, Koeleman, Deelder and Hokke2006; Jang-Lee et al., Reference Jang-Lee, Curwen, Ashton, Tissot, Mathieson, Panico, Dell, Wilson and Haslam2007). Truncated mannose moieties also often occur, but with uncommon modifications, mainly those containing core α(1-3)-fucose and β(1-2)-xylose, similar to those found in plants and insects (van Die et al., Reference van Die, Gomord, Kooyman, van den Berg, Cummings and Vervelde1999; van Die and Cummings, Reference van Die and Cummings2006). Several glycan moieties were identified in schistosome extracts, among them the Lex, poly-Lex, LacdiNAc (LDN), LDN fucosylated (LDNF, LDN-diF and FLDN), lacto-N-fucopentaose-III (LNFP-III), N-glycans containing β(1-2)-xylose, N-glycans containing core α(1-3)-fucose, circulatory antigens (CCA and CAA) and the T and Tn types (Cummings and Nyame, Reference Cummings and Nyame1999; Kantelhardt et al., Reference Kantelhardt, Wuhrer, Dennis, Doenhoff, Bickle and Geyer2002).
Schistosome glycans are differentially expressed throughout the various life cycle stages. The Lex motif is identified throughout all schistosome developmental stages. N-glycans containing LeX and xylose, as well as complex O-glycans, rapidly disappear after cercarial transformation to schistosomula, the migratory larva, whereas LDN-motifs are predominant in adult worms (Smit et al., Reference Smit, van Diepen, Nguyen, Wuhrer, Hoffmann, Deelder and Hokke2015).
Schistosome females lay hundreds of eggs per day in their mammal hosts. The eggs, which embryonate within the host, are largely responsible for the pathogenicity and are central with respect to diagnosis. Therefore, a full understanding of the molecular composition of eggs through development, including their glycan profile, is key to creating efficient alternatives of diagnosis and perhaps of disease amelioration. Immature eggs only present short O-glycan cores, while fully developed eggs express several complex N- and O-glycans containing LeX and multi-fucosylated LDN motifs.
Mature eggs secrete a complex set of proteins that may interact with the host immune system in order to help them to reach the intestine (Chuah et al., Reference Chuah, Jones, Burke, Owen, Anthony, McManus, Ramm and Gobert2013). The importance of the immune response generated by eggs was confirmed by evaluating HIV positive patients co-infected with either S. mansoni or S. haematobium. In these patients, the egg excretion from the host, number of eggs per gram of feces, is significantly impaired due to the depleted immune response (N'Zoukoudi-N'Doundou et al., Reference N'Zoukoudi-N'Doundou, Dirat, Akouala, Penchenier, Makuwa and Rey1995; Karanja et al., Reference Karanja, Colley, Nahlen, Ouma and Secor1997; Mwanakasale et al., Reference Mwanakasale, Vounatsou, Sukwa, Ziba, Ernest and Tanner2003).
Soluble egg antigen (SEA), a crude extract of eggs used as a proxy for excreted/secreted components, is widely used for studies exploring diagnosis, immunomodulation and pathogenesis of schistosomiasis (Caldas et al., Reference Caldas, Campi-Azevedo, Oliveira, Silveira, Oliveira and Gazzinelli2008). SEA contains, among other proteins the glycoproteins, IL-4-inducing principle (IPSE/ α-1) and the T2 ribonuclease Omega-1 glycoproteins. Both glycoproteins contain N- and O-glycan able to stimulate and modulate the host immune system (Abdulla et al., Reference Abdulla, Lim, McKerrow and Caffrey2011; Ferguson et al., Reference Ferguson, Newland, Gibbs, Tourlomousis, Fernandes dos Santos, Patel, Hall, Walczak, Schramm, Haas, Dunne, Cooke and Zaccone2015). Glycoproteins from SEA often present terminals that are multi-fucosylated with α(1-3) and α(1-6)-linked fucose, N-glycans containing xylose, LDN, LDNF and Lex (Khoo et al., Reference Khoo, Nieto, Morris and Dell1997; Jacobs et al., Reference Jacobs, Deelder and Van Marck1999; Nyame et al., Reference Nyame, Yoshino and Cummings2002). When SEA components interact with DCs, different effects are observed: (1) a Th2 type response, caused by increased the expression of co-stimulatory molecules and cytokines; (2) a Th1 response, caused by upregulated IL-12 production; or (3) a suppression of immune inflammatory events through TRL ligand-induction that stimulates DC maturation or activation (Kane et al., Reference Kane, Cervi and Sun2004; Reference Kane, Jung and Pearce2008; Kariuki et al., Reference Kariuki, Farah, Wilson and Coulson2008; Everts et al., Reference Everts, Hussaarts, Driessen, Meevissen, Schramm, van der Ham, van der Hoeven, Scholzen, Burgdorf, Mohrs, Pearce, Hokke, Haas, Smits and Yazdanbakhsh2009). In addition, glycoconjugates containing LNFP, a component of SEA, alone can drive a Th2 response, by inducing DCs in a TLR4-dependent manner (Thomas et al., Reference Thomas, Carter, Atochina, Da'Dara, Piskorska, McGuire and Harn2003). Interestingly, experimental immunization of mice with Schistosoma eggs (Kariuki et al., Reference Kariuki, Farah, Wilson and Coulson2008) or derived glycoconjugates generated a non-protective immune response, making some argue that the eggs-derived glycans are actually diverting the host immune response and therefore would not be useful for vaccine formulation (Eberl et al., Reference Eberl, Langermans, Vervenne, Nyame, Cummings, Thomas, Coulson and Wilson2001).
The glycan motif DF-LDN-DF so far has been found only in S. mansoni and S. japonicum and, therefore, became a promising diagnostic target (Khoo et al., Reference Khoo, Nieto, Morris and Dell1997; Peterson et al., Reference Peterson, Hokke, Deelder and Yoshino2009). This motif is recognized by the monoclonal antibody 114-4D12, which was then used to identify DF-LDN-DF in eggs extract, and also used in a sandwich ELISA to capture the glycan in blood and urine samples (Robijn et al., Reference Robijn, Koeleman, Hokke and Deelder2007). Other fucosylated antigens isolated from cercariae were shown to elicit specific IgM and IgG antibodies that recognize structures such as LDN, LDNF, Lex, F-LDN and LDN-diF, which are fucosylated antigens considered potential diagnostic targets (Nyame et al., Reference Nyame, Lewis, Doughty, Correa-Oliveira and Cummings2003; Vermeer et al., Reference Vermeer, van Dam, Halkes, Kamerling, Vliegenthart, Hokke and Deelder2003).
Since the 1980s, Schistosoma gut-secreted glycosylated antigens, known as circulating cathodic antigen (CCA) and circulating anodic antigen (CAA), have been proposed as diagnostic targets for schistosomiasis (Deelder et al., Reference Deelder, Kornelis, Van Marck, Eveleigh and Van Egmond1980). Glycan analysis of CCA revealed an exclusive O-glycosylation moiety formed by LeX repeating units linked to the protein. Because of the presence of LeX in human circulating neutrophils, it was suggested that the antigenic CCA poly-LeX might be involved in immunomodulation of granulocytes during schistosome infection (van Dam et al., Reference Van Dam, Bergwerff, Thomas-Oates, Rotmans, Kamerling, Vliegenthart and Deelder1994). In 2004, Van Dam and collaborators reported on a Reagent Strip Test they had developed. The strip containing labelled monoclonal antibody specific for detection of Schistosoma CCA in the urine. The authors used the strips to evaluate a group of infected school children in Tanzania and the results were compared with direct egg counts in feces by Kato-Katz. Determination of CCA and CAA levels in sera and CCA in urine by ELISA showed sufficient sensitivity and specificity to be applied in the field as a non-invasive and rapid test (van Dam et al., Reference van Dam, Wichers, Ferreira, Ghati, van Amerongen and Deelder2004). More recent studies have shown that the sensitivity of the CCA assay ranges from 65 to 100%, largely depending on the intensity of infection of the population evaluated (Stothard et al., Reference Stothard, Kabatereine, Tukahebwa, Kazibwe, Rollinson, Mathieson, Webster and Fenwick2006; Colley et al., Reference Colley, Binder, Campbell, King, Tchuem Tchuenté, N'Goran, Erko, Karanja, Kabatereine, van Lieshout and Rathbun2013). The commercially available point-of-care urine dipstick test POC-CCA is now receiving great attention for field studies (Corstjens et al., Reference Corstjens, De Dood, Kornelis, Fat, Wilson, Kariuki, Nyakundi, Loverde, Abrams, Tanke, Van Lieshout, Deelder and Van Dam2014; Casacuberta et al., Reference Casacuberta, Kinunghi, Vennervald and Olsen2016; Lindholz et al., Reference Lindholz, Favero, Verissimo, Candido, de Souza, Dos Santos, Morassutti, Bittencourt, Jones, St Pierre and Graeff-Teixeira2018) and thought to possibly replace Kato-Katz in epidemiological studies.
Fasciola species
Fasciola hepatica and F. gigantica (Platyhelminthes: Trematoda; Fasciolidae), the liver fluke of ruminants and humans, are listed among the neglected tropical diseases. Fascioliasis has a major impact on livestock production. With respect to human health, fascioliasis is now estimated to infect over 1 million people, with 17 million people are at risk of infection (Ravida et al., Reference Ravidà, Aldridge, Driessen, Heus, Hokke and O'Neill2016).
Initial analyses of the F. hepatica glycan repertoire revealed structures containing Tn antigen, mannose and glucose residues, Gal(β1-6)Gal-terminating glycolipids and truncated N-glycans, followed by N-glycans containing oligomannose and some that are core-fucosylated (Freire et al., Reference Freire, Casaravilla, Carmona and Osinaga2003; Wuhrer et al., Reference Wuhrer, Grimm, Dennis, Idris and Geyer2004; Georgieva et al., Reference Georgieva, Georgieva, Mizinska and Stoitsova2012; Garcia-Campos et al., Reference Garcia-Campos, Ravidà, Nguyen, Cwiklinski, Dalton, Hokke, O'Neill and Mulcahy2016). These glycans were identified mainly in the miracidium, on the tegument of newly excysted juvenile, and on the surface of the oral and ventral suckers and the gut of adult flukes. Ravidà et al. (Reference Ravidà, Aldridge, Driessen, Heus, Hokke and O'Neill2016) showed that tegument of Fasciola contains complex N-glycans, some of which are phosphorylated. F. hepatica is well known for inducing a strong Th2/Treg response in its hosts. Glycans might contribute to this bias, since many, including phosphorylated oligosaccharides, associate with proteins in the tegument, and may interact with lectin receptors in APCs cells. Indeed, it was demonstrated that oligomannose motifs of Fasciola specifically interact with C-type lectin receptors resulting in increased IL-4 and IL-10 production and suppression of IFN-γ (Rodríguez et al., Reference Rodríguez, Noya, Cervi, Chiribao, Brossard, Chiale, Carmona, Giacomini and Freire2015).
Discussion
The glycan profile expressed by different parasites dictates the way these organisms interact with their hosts. Several studies have demonstrated that glycans, as opposed to the protein backbone to which they attach, are often the main stimulating agent of the host's immune system during parasitism. Indeed, glycan molecules have been implicated in the antibody response during malaria, amoebiasis, trypanosomiasis, leishmaniasis, schistosomiasis, filariasis, angiostrongyliasis, cysticercosis and hydatidosis (Norden and Strand, Reference Norden and Strand1985; Cummings and Nyame, Reference Cummings and Nyame1996; Reference Cummings and Nyame1999; Eberl et al., Reference Eberl, Langermans, Vervenne, Nyame, Cummings, Thomas, Coulson and Wilson2001; Hokke and Deelder, Reference Hokke and Deelder2001; Morassutti et al., Reference Morassutti, Levert, Perelygin, da Silva, Wilkins and Graeff-Teixeira2012; Montero-Barrera et al., Reference Montero-Barrera, Valderrama-Carvajal, Terrazas, Rojas-Hernández, Ledesma-Soto, Vera-Arias, Carrasco-Yépez, Gómez-García, Martínez-Saucedo, Becerra-Díaz and Terrazas2015; del Puerto et al., Reference Del Puerto, Rovetta, Navatta, Fontana, Lin, Moyna, Dematteis, Brehm, Koziol, Ferreira and Díaz2016; Veríssimo et al., Reference Veríssimo, Morassutti, von Itzstein, Sutov, Hartley-Tassell, McAtamney, Dell, Haslam and Graeff-Teixeira2016).
The literature related to glycans derived from parasites so far reflects the potential application of glycans for the development of immunological tests or vaccines, and also the necessity to improve the production of recombinant antigenic glycoproteins. The expression systems used most often are peptide-based, built around prokaryotic plasmids cloned into prokaryotes that are incapable of producing eukaryotic glycan modifications (Dell et al., Reference Dell, Haslam, Morris and Khoo1999). Furthermore, the general similarities among glycans produced by different parasites, and their hosts, represent an even bigger challenge for the development and application of such molecules in specific diagnosis (van Die and Cummings, Reference van Die and Cummings2010). Any immunological reagent based on glycans have to take this into consideration and must be validated extensively to ensure that it is not a cross-reactive antigen.
In past years, deep exploration of glycan molecules has resulted in advances in the development of diagnosis, vaccines and drugs. Important diseases, such as neurocysticercosis, now have an alternative and better diagnosis as a result of glycan research (Nunes et al., Reference Nunes Dda, Gonzaga, Ribeiro Vda, da Cunha and Costa-Cruz2013). In this review, we discussed the involvement of glycans in important processes of recognition and interactions with hosts, which potentially can allow wider comprehension of the host–parasite relationship and important aspects of infection, including the preference for certain hosts. In this last aspect, there is evidence that glycans can be important determinants of host-specificity. For example, the pattern of glycosylation of the glycoproteins present in the haemolymph of different strains of Biomphalaria glabrata, the intermediate host of S. mansoni, revealed that those strains expressing higher amounts of fucosylated glycan moieties have a higher susceptibility to S. mansoni infection (Lehr et al., Reference Lehr, Frank, Natsuka, Geyer, Beuerlein, Doenhoff, Hase and Geyer2010). Further understanding of these interactions, may result in a successful strategy for control of parasite through transmission blocking.
In this review, we also highlighted how parasitic glycans are being considered in the context of vaccine development. Currently, carbohydrate-based vaccines are thought to be a decisive strategy to improve vaccination, which is reinforced by the success of vaccines based on capsular polysaccharides to combat different bacterial infections (Roggelin et al., Reference Roggelin, Vinnemeier, Fischer-Herr, Johnson-Weaver, Rolling, Burchard, Staats and Cramer2015; Gala et al., Reference Gala, D'Souza and Zughaier2016). The detection and characterization of glycans or glycoconjugates are becoming more feasible since new tools and resources are constantly emerging, including genomes and transcriptomes of various parasites as well as array-based techniques. These tools open up the possibility of predicting not only a specific glycan but the whole potential glycan metabolism of an organism, from monosaccharides transporters and nucleotide sugar biosynthesis pathways to probable glycan chain extensions of N- and O-glycans. Although still limited, since only enzymes with human homologues can be predicted, such determinations followed by more functional analyses of enzymes can lead to implementation of new recombinant systems to produce specific glycans (Prasanphanich et al., Reference Prasanphanich, Luyai, Song, Heimburg-Molinaro, Mandalasi, Mickum, Smith, Nyame and Cummings2014), and to the development of new drugs and vaccines (Mickum et al., Reference Mickum, Prasanphanich, Heimburg-Molinaro, Leon and Cummings2014).
In an example of the applicability of genomic prediction, Peterson et al. (Reference Peterson, Anderson and Yoshino2013) used a genome-wide homology-based bioinformatics approach to identify α3- and α6-fucosyltransferases (FucTs) genes that contribute to the production of fucosylated glycans in S. mansoni revealing information regarding the genomic organization, genetic variation and stage expression of these enzymes. Moreover, difficulties with the isolation and/or recombinant production of specific glycans can now be overcome using strategies of chemical synthesis of these molecules, which for instance have revolutionized the development of vaccines as mentioned for T. spiralis, Echinococcus, Schistosoma and P. falciparum. The last of these may result in the first glycan-based vaccine to combat a parasitic infection. Altogether, these data demonstrate the wide application of discoveries in glycobiology and inspire further research in this field.
Conclusions
Parasites are covered by or secrete distinct glycoconjugates that have been experimentally shown to help with the parasite's survival and propagation. The improvement of methods related to glycan analysis has opened a completely new field to be exploited. In recent years, the glycan profiles of several parasites have been characterized, giving us knowledge of the range of carbohydrates produced by different organisms. In fact, these molecules were shown to vary from complex to simple structures, and their expression might change with parasite genus, species and even strains and developmental stage, highlighting the numerous applications these molecules could have in strategies to control parasitic infections.
However, the general similarities among glycans produced by different parasites, and their hosts, represent a challenge for the development of specific diagnoses, as these molecules seem to haunt immunological tests through cross-reactivity that lead to false positive reactions. Forthcoming investigations and development of more advanced technologies for glycan analyses should permit us to deal with the cross-reactivity problem and uncover the machinery behind glycan biosynthesis and allow large scale production of them to fight against a wide range of diseases including parasitic infections.
Author ORCIDs
Alessandra Loureiro Morassutti, 0000-0002-8142-1055
Financial support
This work was supported by the The Brazilian National Council for Scientific and Technological Development (CNPq) (A.L.M grant number: 406149/2016-0 and C.G.T. 307005/2014-3) and the National Health and Medical Research Council of Australia (to MKJ).
Conflicts of interest
None.