Introduction
Tetrahedrite-group minerals are the most common sulfosalts in different kinds of hydrothermal ore deposits. They form a complex isotypic series characterised by multiple homo- and heterovalent substitutions, representing an interesting link between mineralogy and ore geochemistry (Moëlo et al., Reference Moëlo, Makovicky, Mozgova, Jambor, Cook, Pring, Paar, Nickel, Graeser, Karup-Møller, Balić-Žunić, Mumme, Vurro, Topa, Bindi, Bente and Shimizu2008; Biagioni et al., Reference Biagioni, George, Cook, Makovicky, Moëlo, Pasero, Sejkora, Stanley, Welch and Bosi2020a). The classification and nomenclature of the tetrahedrite-group minerals, in keeping with the current International Mineralogical Association (IMA) rules (Mills et al., Reference Mills, Hatert, Nickel and Ferraris2009; Bosi et al., Reference Bosi, Hatert, Hålenius, Pasero, Miyawaki and Mills2019) was published recently by Biagioni et al. (Reference Biagioni, George, Cook, Makovicky, Moëlo, Pasero, Sejkora, Stanley, Welch and Bosi2020a). The general structural formula of minerals belonging to this group can be written as M (2)A6M (1)(B4C2)Σ6X (3)D4S(1)Y12S(2)Z, where A = Cu+, Ag+, □ (vacancy) and (Ag6)4+ cluster; B = Cu+ and Ag+; C = Zn2+, Fe2+, Hg2+, Cd2+, Ni2+, Mn2+, Cu2+, Cu+ and Fe3+; D = Sb3+, As3+, Bi3+ and Te4+; Y = S2– and Se2–; and Z = S2–, Se2– and □ (Biagioni et al., Reference Biagioni, George, Cook, Makovicky, Moëlo, Pasero, Sejkora, Stanley, Welch and Bosi2020a).
Silver-rich members with 3–6 Ag atoms per formula unit (apfu) (the A constituent, now the freibergite/arsenofreibergite series) have been known for a long time. Indeed, ‘freibergite’ was first described from the Hab Acht Mine near Freiberg, Saxony, Germany by Weissenbach (Reference Weissenbach1831) and named by Kenngott (Reference Kenngott1853). Currently IMA-accepted minerals belonging to the freibergite series are argentotetrahedrite-(Fe) (Welch et al., Reference Welch, Stanley, Spratt and Mills2018), argentotetrahedrite-(Hg) (Wu et al., Reference Wu, Gu, Qu, Yang and Wang2021), argentotetrahedrite-(Zn) (Sejkora et al., Reference Sejkora, Biagioni, Števko, Raber and Roth2021), kenoargentotetrahedrite-(Fe) (the former ‘freibergite’ – Welch et al., Reference Welch, Stanley, Spratt and Mills2018; Biagioni et al., Reference Biagioni, George, Cook, Makovicky, Moëlo, Pasero, Sejkora, Stanley, Welch and Bosi2020a) and kenoargentotetrahedrite-(Zn) (Qu et al., Reference Qu, Sima, Gu, Sun, Fan, Hou, Ni, Wang, Yang and Wang2021). The arsenofreibergite series comprises fewer species, being currently represented only by argentotennantite-(Zn) (Spiridonov et al., Reference Spiridonov, Sokolova, Gapeev, Dashevskaya, Evstigneeva, Chvileva, Demidov, Balashov and Shul'ga1986; Biagioni et al., Reference Biagioni, George, Cook, Makovicky, Moëlo, Pasero, Sejkora, Stanley, Welch and Bosi2020a) and kenoargentotennantite-(Fe) (Biagioni et al., Reference Biagioni, Sejkora, Moëlo, Makovicky, Pasero and Dolnícek2020b).
The Ag-rich members of the tetrahedrite group, however, are known from many occurrences worldwide and at some deposits represent the main Ag ore, extremely Ag-rich members, with Ag > 8 apfu (~45 wt.% Ag; as both the A and B constituents), are distinctly rarer. The first documented find is probably from the Kutná Hora deposit (Czech Republic) where Kvaček et al. (Reference Kvaček, Novák and Drábek1975) described ‘tetrahedrite’ with ~8 Ag apfu (and with Fe and Sb dominant as C and D constituents, respectively). Later, Paar et al. (Reference Paar, Chen and Günthe1978) found ‘freibergite’ (Zn–Sb) with 8.6–10.5 Ag apfu in material from the “Knappenstube” mine, Hochtor, Salzburg (Austria). The Fe- and As-dominant member with 8.8 Ag apfu was reported from the Manson Lode deposit, Kelantan, Malaysia by Gan (Reference Gan1980). Ixer and Stanley (Reference Ixer and Stanley1983) analysed a Zn- and As-dominant member occurring as inclusion in galena from the Sark's Hope mine, Sark, Channel Islands, with ~8 Ag apfu. In the 1990s, further findings of extremely Ag-rich members of the tetrahedrite group were reported from China and Russia. Li and Wang (Reference Li and Wang1990) described ‘freibergite’ (Fe–Sb dominant) from the Dachang ore field, Guangxi (China), with 9.0–9.6 Ag apfu and with a = 10.950 Å. Zhdanov et al. (Reference Zhdanov, Amuzinskiy and Andrianov1992) described Ag-rich ‘tetrahedrite’ (Fe–Sb dominant) from the deposit of Khachakchan, Verkhoyan-Kolyma region, Russia, with Ag content ranging between 10.2 and 10.7 apfu and a unit-cell parameter a = 10.92 Å. Later, Samusikov and Gamyanin (Reference Samusikov and Gamyanin1994) presented an almost Ag-pure sample (52 wt.% Ag, with only 0.7 wt.% Cu; a = 10.90 Å) from Yakutia, Russia and named it ‘tarynite’ from the locality. However, no official proposal was submitted to the then ‘Commission on New Minerals and Mineral Names’ of the IMA by these authors. Foit and Ulbricht (Reference Foit and Ulbricht2001) documented ‘tetrahedrite’ samples (Hg and Sb dominant) from the O'Keefe pit, Steens-Pueblo, Oregon, USA with 9.3 Ag apfu. The Zn and As dominant ‘argentotennantite’ with 9.1 Ag apfu was described by Koch and Heider (Reference Koch and Heider2018) from the Frische Lutter mine, Bad Lauterberg, Harz, Germany. The first IMA-approved member of tetrahedrite group with Ag > 8 apfu was rozhdestvenskayaite-(Zn), Ag6(Ag4Zn2)Sb4S13, described by Welch et al. (Reference Welch, Stanley, Spratt and Mills2018) from the Moctezuma mine, Sonora, Mexico.
During a systematic investigation of tetrahedrite-group minerals from the Czech Republic, a sample characterised by an extremely Ag-rich composition and with As > Sb was identified. This new mineral and its name were approved by the Commission on New Minerals, Nomenclature and Classification (CNMNC) of the IMA (IMA2020-061, Sejkora et al., Reference Sejkora, Biagioni, Vrtiška and Moëlo2020). Zvěstovite-(Zn) is named after the type locality, Zvĕstov, Czech Republic; the suffix indicates the dominance of Zn as the C constituent, according to the approved nomenclature of the tetrahedrite group (Biagioni et al., Reference Biagioni, George, Cook, Makovicky, Moëlo, Pasero, Sejkora, Stanley, Welch and Bosi2020a). The holotype material (polished section) is deposited in the mineralogical collection of the Department of Mineralogy and Petrology of the National Museum, Prague, Czech Republic (catalogue number P1P 50/2020). The crystal used for the single-crystal X-ray diffraction study is stored in the mineralogical collection of the Museo di Storia Naturale of the Università di Pisa, Via Roma 79, Calci (PI), under catalogue number 19921.
Occurrence and mineral description
Occurrence
One hand-sized specimen of zvěstovite-(Zn) was collected at the mine dump in the southern part of the abandoned small deposit of Zvěstov (Stříbrnice), which is located 1200 m NNE from the village of Zvěstov. This locality is 10 km SW of Vlašim, central Bohemia region, Czech Republic. The GPS coordinates of the occurrence of zvěstovite-(Zn) are 49°38′39.069″N, 14°47′51.993″E. The Zvěstov deposit is represented by an irregular hydrothermal vein system ~1300 m long, with thickness ~20–50 cm and a vertical extent (verified by prospect borehole) of ~60 m (Nouza, Reference Nouza1988). There are no written records about historical mining at this deposit (Velebil, Reference Velebil2004). A fragment of a ceramic mug found in the studied mine dump was dated at the turn of the 15th and 16th Centuries (Velebil et al., Reference Velebil, Macek and Soumar2016). The exploration was last carried out here in the years 1956–1957 (Nouza, Reference Nouza1988). The quartz + baryte veins of the Zvěstov deposit host grains of galena, sphalerite, chalcopyrite, pyrite, arsenopyrite and minerals of the tetrahedrite group (Nouza, Reference Nouza1988; Velebil et al., Reference Velebil, Macek and Soumar2016). This deposit is one of the small ore occurrences connected with the Blanice Graben (Fig. 1), which represents an ~200 km long NNE–SSW trending crustal-scale brittle tectonic zone in the Moldanubian Unit with minimal sinistral movement of ~17 km (Zachariáš and Hübst, Reference Zachariáš and Hübst2012). It hosts low-fineness Au mineralisation (Roudný, Dobrá Voda) and younger Ag–Pb–Zn mineralisations (e.g. Ratibořské Hory – Stará Vožice, Rudolfov or Stříbrná Skalice).
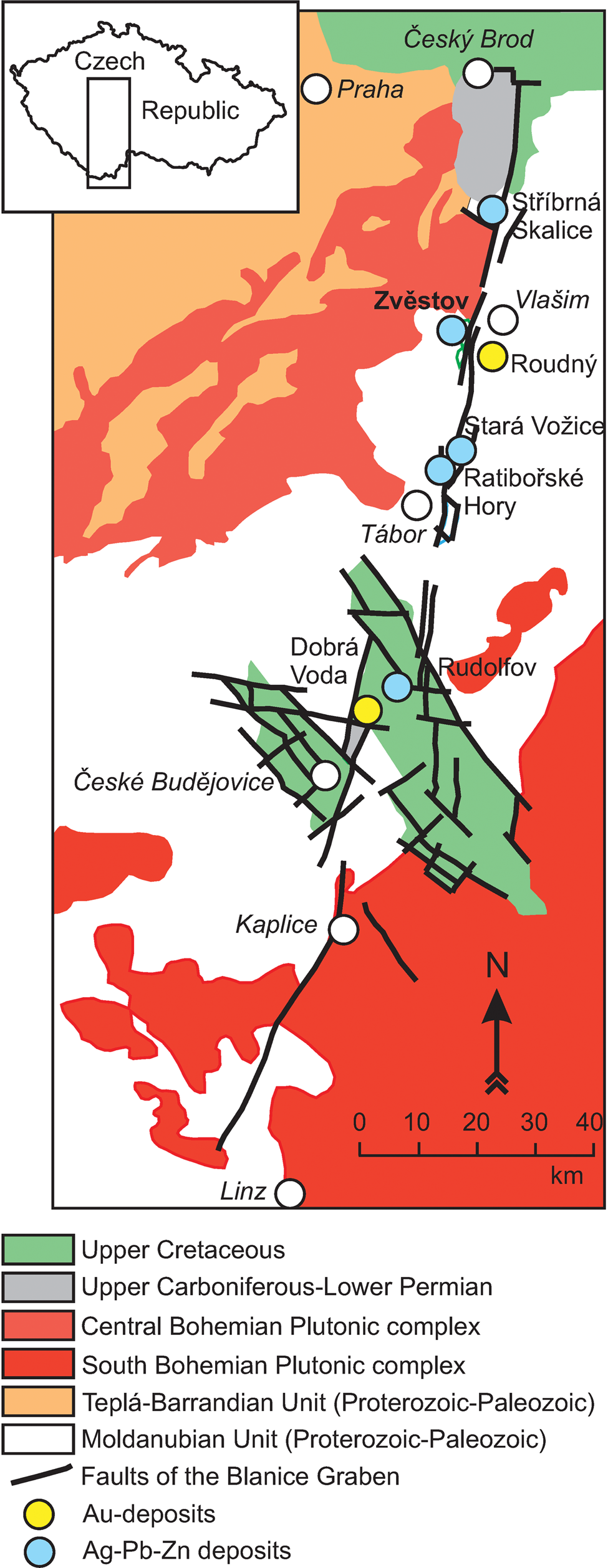
Fig. 1. Simplified geological map of the Blanice Graben and surrounding units with location of Au and Ag–Pb–Zn hydrothermal vein-type deposits (modified from Zachariáš and Hübst, Reference Zachariáš and Hübst2012).
The holotype specimen of zvĕstovite-(Zn) is a fragment of a quartz + baryte vein with sulfide disseminations [tennantite-(Zn), tetrahedrite-(Zn), argentotennantite-(Zn) and acanthite], as well as clasts of the host rocks (gneiss); it is partly supergenically altered, originating azurite and malachite.
Physical and optical properties
Zvěstovite-(Zn) forms relic anhedral grains rimmed by acanthite (Fig. 2), up to 100 μm in size. The mineral is grey in colour and is opaque in transmitted light; it has a metallic lustre. No cleavage was observed; it is brittle with a conchoidal fracture. The calculated density (Z = 2) for the empirical formula is 5.16 g.cm–3. Mohs hardness is assumed at 3½–4 by analogy with other members of the tetrahedrite group. In reflected light, zvěstovite-(Zn) is grey with a greenish tint, without bireflectance, pleochroism and anisotropy. Internal reflections are ubiquitous and deep red in colour. Reflectance spectra were measured in air with a TIDAS MSP400 spectrophotometer attached to a Leica microscope (50× objective) using a WTiC (Zeiss no. 370) standard, with a square sample measurement field of ca. 7 μm × 7 μm. The results from the 400–700 nm range are given in Table 1 and plotted in Fig. 3 in comparison with published data (Welch et al., Reference Welch, Stanley, Spratt and Mills2018) for rozhdestvenskayaite-(Zn), argentotetrahedrite-(Fe) and kenoargentotetrahedrite-(Fe) (originally ‘freibergite’).

Fig. 2. Zvĕstovite-(Zn), as relict grains rimmed by acanthite; (a) reflected light photo, partly crossed polarisers; (b) back-scattered electron photo, where the area of extracted fragment for single-crystal X-ray diffraction study is outlined in red. Holotype material (catalogue number P1P 50/2020).

Fig. 3. Reflectance curve for zvěstovite-(Zn) compared with published data (Welch et al., Reference Welch, Stanley, Spratt and Mills2018) for rozhdestvenskayaite-(Zn), argentotetrahedrite-(Fe) and kenoargentotetrahedrite-(Fe).
Table 1. Reflectance values (%) for zvěstovite-(Zn).

The reference wavelengths required by the Commission on Ore Mineralogy (COM) are given in bold.
Chemical composition
Chemical analyses were performed using a Cameca SX100 electron microprobe (National Museum, Prague) operating in wavelength-dispersive mode (25 kV, 20 nA and 1 μm wide beam). The following standards and X-ray lines were used to minimise line overlaps: Ag (AgLα), Au (AuMα), Bi (BiMβ), CdTe (CdLα), Co (CoKα), chalcopyrite (CuKα, SKα), pyrite (FeKα), HgTe (HgMα), Ni (NiKα), NiAs (AsLα), PbS (PbMα), PbSe (SeLα), PbTe (TeLα), Sb2S3 (SbLα), TlBrI (TlLα) and ZnS (ZnKα). Peak counting times were 20 s for all elements, and 10 s for each background. Gold, Bi, Co, Hg, Ni, Pb, Se, Te and Tl were found to be below the detection limits (0.02–0.05 wt.%). Raw intensities were converted to the concentrations of elements using the automatic ‘PAP’ (Pouchou and Pichoir, Reference Pouchou and Pichoir1985) matrix-correction procedure.
Analytical data for the zvěstovite-(Zn) grain used for single-crystal study are given in Table 2. On the basis of ΣMe = 16 apfu, the empirical chemical formula is Ag6.27[(Ag3.90Cu0.38)Σ4.28(Zn1.60Fe0.09Cd0.03)Σ1.72](As2.26Sb1.48)Σ3.74S12.50. The ideal formula is Ag6(Ag4Zn2)As4S13, which requires Ag 56.01, Zn 6.79, As 15.56 and S 21.64, a total of 100.00 wt.%. Representative analyses for all zvěstovite-(Zn) grains in the holotype sample are given in Table 3. The grains studied show distinct AgCu–1 substitution (Fig. 4) with Ag and Cu contents 8.23–10.51 apfu and 0.10–2.00 apfu, respectively. The dominant Me 2+ is Zn (1.55–1.85 apfu) accompanied by minor Fe (0.07–0.15 apfu) and Cd (0.02–0.05 apfu). Arsenic (2.05–2.37 apfu) always prevails over Sb (1.35–1.79 apfu). The determined contents of S are in the range 12.32–12.99 apfu, values of S > 12.50 apfu were found for 9 of the 14 point analyses (Table 3).

Fig. 4. Relation between Ag vs. Cu contents (apfu) in the zvěstovite and rozhdestvenskayaite series. Dashed line indicates the ideal Cu + Ag = 10 apfu. Zvěstovite-(Zn): [1] holotype sample – grain used for crystal structure study; [2] holotype sample – other grains; [3] Bad Lauterberg (Koch and Heider, Reference Koch and Heider2018). Rozhdestvenskayaite-(Zn): [4] Moctezuma mine (Welch et al., Reference Welch, Stanley, Spratt and Mills2018); [5] Moctezuma mine (Sejkora, unpublished data), [6] Knappenstube (Paar et al., Reference Paar, Chen and Günthe1978). Not approved ‘rozhdestvenskayaite-(Fe)’: [7] Khachakchan (Zhdanov et al., Reference Zhdanov, Amuzinskiy and Andrianov1992); [8] Dachang (Li and Wang, Reference Li and Wang1990). Not approved ‘rozhdestvenskayaite-(Hg)’: [9] O'Keefe pit, Steens-Pueblo (Foit and Ulbricht, Reference Foit and Ulbricht2001).
Table 2. Chemical data (wt.%) for zvěstovite-(Zn) crystal used for the single-crystal X-ray study (n = 4) and chemical formula (apfu) based on ΣMe = 16 apfu.

S.D. – standard deviation
Table 3. Representative analyses (wt.%) for zvěstovite-(Zn) from Zvěstov.*

* [1–4] crystal used for the single-crystal study; [5–14] other grains in holotype sample; coefficients of the empirical formula were calculated on the basis 16 Me apfu.
X-ray diffraction data
Powder X-ray diffraction data could not be collected, due to the paucity of available material. Consequently, powder X-ray diffraction data, given in Table 4, were calculated using the software PowderCell 2.3 (Kraus and Nolze, Reference Kraus and Nolze1996) on the basis of the structural model discussed below.
Table 4. Calculated powder X-ray diffraction data for zvĕstovite-(Zn).*

* Intensity and d hkl were calculated using the software PowderCell2.3 (Kraus and Nolze, Reference Kraus and Nolze1996) on the basis of the structural data given in Tables 5 and 6. Only reflections with I rel. ≥ 0.5 are listed. The five strongest reflections are given in bold.
Table 5. Summary of data collection conditions and refinement parameters for zvěstovite-(Zn).

* w = 1/[σ2(F o2)+(0.0827P)2+30.0580P], where P = (F o2+2F c2)/3
** Flack (Reference Flack1983)
Table 6. Atoms, atom coordinates and or equivalent isotropic displacement parameters (Å2) for zvĕstovite-(Zn).

Wyck – Wyckoff position; s.o.f. – site occupation factor
A short prismatic fragment of zvěstovite-(Zn), 50 μm × 40 μm × 30 μm, extracted from the polished section analysed using electron microprobe (Fig. 2), was mounted on a glass fibre and examined with a Bruker Smart Breeze diffractometer equipped with an air-cooled CCD detector (Dipartimento di Scienze della Terra, Università di Pisa), and graphite-monochromatised MoKα radiation. The detector-to-crystal distance was 50 mm. Data were collected using ω scan mode, in 0.5° slices, with an exposure time of 45 s per frame. Owing to the small crystal size and the weakness of the observed reflections, intensity data collection was possible up to 2θ = ~47°. From the total of 2367 reflections, 199 were independent and 193 classified as unique observed with I > 4σ(I). The data were corrected for Lorentz and polarisation factors and absorption using the software package Apex3 (Bruker AXS Inc., 2016).
The crystal structure of zvĕstovite-(Zn) was refined using Shelxl-2018 (Sheldrick, Reference Sheldrick2015) starting from the atomic coordinates of rozhdestvenskayaite-(Zn) (Welch et al., Reference Welch, Stanley, Spratt and Mills2018). The occurrence of a racemic twin was modelled [twin ratio 0.4(2)]. The following neutral scattering curves, taken from the International Tables for Crystallography (Wilson, Reference Wilson1992) were used: Ag vs. vacancy at M(2); Ag vs. Zn at M(1); As vs. Sb at X(3); and S at S(1) and S(2) sites. An isotropic model converged to R 1 = 0.09, thus confirming the correctness of the structural model. Site occupancies at the split M(2a) and M(2b) positions were found close to a full Ag occupancy at M(2); consequently, in the latest stages of the refinement, the sum of the site occupancies at the two split sites was constrained to full occupancy. The anisotropic structural model converged to R 1 = 0.0508 for 193 reflections with F o > 4σ(F o) and 27 refined parameters. Details of data collection and refinement are given in Table 5. Fractional atomic coordinates and equivalent isotropic displacement parameters are reported in Table 6. Table 7 reports selected bond distances. Finally, Table 8 gives the bond-valence sums (BVS) obtained using the bond-valence parameters of Brese and O'Keeffe (Reference Brese and O'Keeffe1991). Anisotropic displacement parameters are reported in the crystallographic information file, which has been deposited with the Principal Editor of Mineralogical Magazine and is available as Supplementary material (see below).
Table 7. Selected bond distances (in Å) for zvĕstovite-(Zn).

Table 8. Weighted bond-valence sums (in valence units) in zvĕstovite-(Zn).

Crystal structure of zvĕstovite-(Zn)
The crystal structure of zvĕstovite-(Zn) agrees with the general features of the members of the tetrahedrite group. The M(2) site was found to be split into two sub-positions, labelled M(2a) and M(2b) (Fig. 5), similar to those reported by Makovicky et al. (Reference Makovicky, Karanović, Poleti and Balić-Žunić2005) in Cu-excess unsubstituted tennantite, and by Welch et al. (Reference Welch, Stanley, Spratt and Mills2018) in rozhdestvenskayaite-(Zn). Both positions are triangularly coordinated. The observed distances agree with the Ag-pure nature of this position, in agreement with figure 5 of Welch et al. (Reference Welch, Stanley, Spratt and Mills2018). The BVS calculated at these two sub-positions, assuming the full occupancy by Ag, results in a good agreement for M(2b) [BVS = 0.97 valence units, vu], whereas the BVS at M(2a) shows an important overbonding [BVS = 1.62 vu]. Indeed, whereas the mean <M(2b)–S> distance is 2.57 Å, the <M(2a)–S> is 2.39 Å, too short with respect to ideal <Ag–S> in three-fold coordination, 2.56 Å. This short average distance is mainly related to the short M(2a)–S(2) distance. This behaviour was observed by Johnson et al. (Reference Johnson, Craig and Rimstidt1988) who highlight that three-fold Ag is distinctly aspherical, with the increase of the M(2)–S(1) distance, related to the increasing Ag content in tetrahedrite-group minerals, being significantly larger than that observed for the M(2)–S(2) bond. Indeed, Welch et al. (Reference Welch, Stanley, Spratt and Mills2018) reported a similar short M(2a)–S(2) distance (2.284 Å) in rozhdestvenskayaite-(Zn). M(2a) and M(2b) show an elongate displacement ellipsoid having their elongation axes oriented approximately along a line connecting two M(2b) positions with the M(2a) site located between them. The distances M(2a)–M(2b) and M(2b)–M(2b) are 1.00(5) and 1.97(11) Å, to be compared with the corresponding Cu2A–Cu2B and Cu2B–Cu2B distances of 1.08(2) and 2.00(3) Å reported by Makovicky et al. (Reference Makovicky, Karanović, Poleti and Balić-Žunić2005) for Cu-excess tennantite. Welch et al. (Reference Welch, Stanley, Spratt and Mills2018) proposed a split-site model for rozhdestvenskayaite-(Zn) using isotropic displacement parameters for the two split positions labelled A1 and A2, and they found distances A1–A2 and A2–A2 of 0.789(5) and 1.545(9) Å, respectively.

Fig. 5. Coordination of the two split sites M(2a) and M(2b) (a and b, respectively).
The tetrahedrally coordinated M(1) site (Fig. 6) has a bond distance of 2.497(6) Å, in agreement with the M(1)–S(1) distance reported for rozhdestvenskayaite-(Zn), i.e. 2.496(2) Å (Welch et al., Reference Welch, Stanley, Spratt and Mills2018). On the basis of the electron microprobe analysis, the site population (Ag0.65Zn0.27Cu0.06Fe0.02) can be proposed. The bond-valence sum at this site is 1.44 vu, in agreement with the theoretical value (1.31 vu, assuming all Cu as monovalent and Fe as Fe3+).

Fig. 6. Coordination of the M(1) and X(3) sites.
The X(3) site (Fig. 6) has bond distance of 2.322(8) Å and it shows a mixed (As0.60Sb0.40) site occupancy, in agreement with chemical data. The bond-valence sum is 3.21 vu. Taking into account the bond-valence approach, a better site occupation factor at X(3) would be (As0.70Sb0.30), corresponding to (As2.80Sb1.20) apfu, with an As/(As + Sb) slightly higher than that determined through electron microprobe (0.70 against 0.60).
All the geometrical features of the studied samples agree with its isotypic relationships with rozhdestvenskayaite-(Zn) (Welch et al., Reference Welch, Stanley, Spratt and Mills2018). In addition, the volume of the S(2)(Ag6) octahedron, calculated using the M(2a) position, is ~15.3 Å3, agreeing with the volume observed in rozhdestvenskayaite-(Zn), i.e. 15.9 Å3 (Welch et al., Reference Welch, Stanley, Spratt and Mills2018). The refinement of the S(2) site as fully occupied results in a high U eq value, 0.086(13) Å2, similar to that reported by Welch et al. (Reference Welch, Stanley, Spratt and Mills2018), i.e. 0.095(5) Å2. The occurrence of a minor vacancy at S(2) may be possible, but the occurrence of Ag–Ag bonds as reported in kenoargentotetrahedrite-(Fe) was not observed. In addition, the high U eq value, coupled with the short M(2a)–S(2), could be the result of the dynamic disorder probably affecting both the positions of the M(2) and S(2) atoms. Indeed, an ordered distribution of S, with more reasonable Me–S distances, may be achieved through the splitting of S(2) in positions displaced from the (0,0,0) position.
Discussion
Recalculation of chemical formulae of Ag-rich tetrahedrites
There are several different approaches to recalculate the chemical formula of the minerals belonging to the tetrahedrite group.
(1) Normalisation on the basis ΣS(+Se) = 13 apfu, which prevails in the older literature especially. However, there is clear crystallographic evidence of the possible occurrence of vacancies at the S(2) site (e.g. Rozhdestvenskaya et al., Reference Rozhdestvenskaya, Zayakina and Samusikov1993; Welch et al., Reference Welch, Stanley, Spratt and Mills2018; Škácha et al., Reference Škácha, Sejkora, Plášil and Makovicky2020). Moreover, it is recognised that the S content of tetrahedrite-group minerals determined by electron microprobe can be substantially affected by analytical uncertainty, as seen in many published analyses (e.g. Repstock et al., Reference Repstock, Voudouris, Zeug, Melfos, Zhai, Li, Kartal and Matuszczak2016; Wang et al., Reference Wang, Zhang, Guo, Pi and Yang2018). Thus, this recalculation basis is clearly unsatisfactory as it assumes a fixed (S + Se) content, which in reality can be highly variable (Welch et al., Reference Welch, Stanley, Spratt and Mills2018).
(2) Normalisation on the basis of ΣMe = 16 apfu. This recalculation assumes that no vacancies occur at M(2), M(1) and X(3). However, vacancies are known there, especially in the case of goldfieldite (Makovicky and Karup-Møller, Reference Makovicky and Karup-Møller2017; Biagioni et al., Reference Biagioni, George, Cook, Makovicky, Moëlo, Pasero, Sejkora, Stanley, Welch and Bosi2020a) or pošepnýite (Škácha et al., Reference Škácha, Sejkora, Plášil and Makovicky2020). Consequently, this normalisation can be used in several cases, but it should be avoided for Te-rich members of the tetrahedrite group.
(3) Normalisation on the basis of (As + Sb+ Bi + Te) = 4 apfu. Previous studies (e.g. Johnson et al., Reference Johnson, Craig and Rimstidt1986) revealed negligible variations with respect to the ideal number of D atoms that occur.
(4) Normalisation on the basis of all 29 apfu for the general formula. This recalculation could mask some S deficit (e.g. only 12 apfu S instead 13 in the case of kenoargentotetrahedrite-(Fe); Biagioni et al., Reference Biagioni, George, Cook, Makovicky, Moëlo, Pasero, Sejkora, Stanley, Welch and Bosi2020a) or vacancies at M(2) and M(1) sites (Biagioni et al., Reference Biagioni, George, Cook, Makovicky, Moëlo, Pasero, Sejkora, Stanley, Welch and Bosi2020a; Škácha et al., Reference Škácha, Sejkora, Plášil and Makovicky2020).
Silver-rich members of the tetrahedrite group can show variable S contents, related to the possible occurrence of a S vacancy at S(2) and presence of (Ag6)4+ clusters. Consequently, chemical formulae of these compounds should be normalised on the basis of normalisation procedures (2) or (3). For zvěstovite-(Zn) the normalisation on the basis of ΣMe = 16 apfu gives the formula (Ag10.17Cu0.38Zn1.60Fe0.09Cd0.03)Σ12.27(As2.26Sb1.48)Σ3.74S12.50. On the basis of the crystal structure refinement the following crystal-chemical formula can be written: Ag6.27(Ag3.90Cu0.38Zn1.60Fe0.09Cd0.03)Σ6.00(As2.26Sb1.48)Σ3.74S12.50. The alternative empirical formula, based on As + Sb = 4 apfu, is (Ag10.90Cu0.41Zn1.71Fe0.09Cd0.03)Σ13.14(As2.42Sb1.58)Σ4.00S13.39. On the basis of the crystal structure, the following crystal-chemical formula can be written as Ag7.14(Ag3.76Cu0.41Zn1.71Fe0.09Cd0.03)Σ6.00(As2.42Sb1.58)Σ4.00S13.39. In both cases, the Ev(%) value, where Ev(%) = [Σ(val+) − Σ(val−)] × 100 / Σ(val−), assuming all Cu as Cu+ and Fe as Fe3+, is +0.7(8), ranges between +0.1 and +1.9.
Ag excess in zvěstovite-(Zn)
The observed Ag(+Cu) excess in zvěstovite-(Zn) has to be discussed. The occurrence of a Cu excess is known in tennantite (e.g. Makovicky et al., Reference Makovicky, Karanović, Poleti and Balić-Žunić2005); moreover, Maske and Skinner (Reference Maske and Skinner1971) reported a compositional field between Cu12.3As4S13 and Cu13.7As4S13 for synthetic tennantite. Possibly, a similar mechanism could also act in Ag-bearing members of the tetrahedrite group, although no previous data are available. The excess of Ag (0.27 apfu in the formula normalised on ΣMe = 16 apfu, and 1.14 apfu in the formula normalised on the sum of Sb and As), would correspond to a value of electrons per site (assuming a six-fold multiplicity of the site in a formula unit) ranging between ~2 and ~9 electrons. No additional maxima in the difference-Fourier maps, suggesting the occurrence of possible additional Ag sites having a low occupancy, were detected.
Another possibility is that the Ag excess could be related to a deficiency in the sum of D cations. One could propose that the observed excess of Ag (0.27 apfu) and the (As + Sb) and S deficit (0.26 and 0.50 apfu, respectively) occurring in zvěstovite-(Zn) may be explained through the substitution (As/Sb)3+ + S2– = Ag+ + □. The site scattering refined at the X(3) site is 40.25 electrons; assuming that the Ag excess occurs at the X(3) site, the calculated site scattering, with the population (As0.56Sb0.37Ag0.07), would be 40.64 electrons, in agreement with the observed value. However, assuming a full occupancy at the X(3) site by As and Sb only, the calculated site scattering would be 40.11 electrons. Moreover, the average <X(3)–S> distance suggests a higher As/(As + Sb) atomic ratio, compatible with the occurrence of higher amounts of smaller cations; on the contrary, the presence of Ag should probably increase the bond length. However, taking into account the relatively low quality of the structural refinement, the very minor Ag substitution, and the absence of any hints suggesting detectable S vacancies, there is no proof supporting this substitution in zvěstovite-(Zn).
As zvěstovite-(Zn) could be an ionic conductor, the analytical shift observed with respect to the ideal stoichiometry could be due to the migration of some Ag at the surface of the polished section under the electron beam. Considering that some Ag-excess was observed in other Ag-rich members of the tetrahedrite group, more data are required to solve this issue. Previous authors (e.g. Lind and Makovicky, Reference Lind and Makovicky1982; Makovicky and Karup-Møller, Reference Makovicky and Karup-Møller1994) discussed the analytical problems arising during electron microprobe analysis of tetrahedrite-group minerals. In particular, they stressed the analytical shifts related to the choice of different standards as well as the apparent Cu migration under the electron beam, leading to a shift of all Cu-enriched tetrahedrites towards the minimum Cu content.
Zvĕstovite-(Zn) in the framework of the tetrahedrite group
Zvěstovite-(Zn) has isotypic relations with its Sb-analogue, rozhdestvenskayaite-(Zn) (Welch et al., Reference Welch, Stanley, Spratt and Mills2018). Both minerals are currently unassigned members of the tetrahedrite group. However, as detailed above, several occurrences of potential new minerals suggest that, in addition to the potential rozhdestvenskayaite series already proposed in Biagioni et al. (Reference Biagioni, George, Cook, Makovicky, Moëlo, Pasero, Sejkora, Stanley, Welch and Bosi2020a), the zvěstovite series, characterised by the chemical constituents A = Ag, B = Ag, and D = As, is likely to be created.
A comparison between selected data of zvěstovite-(Zn) and valid or potential members of the not-yet formally existing rozhdestvenskayaite series is shown in Table 9. Although zvěstovite-(Zn) does not correspond to any valid or invalid unnamed mineral (Smith and Nickel, Reference Smith and Nickel2007), tetrahedrite-group minerals having chemical compositions corresponding to that of zvěstovite-(Zn) were reported previously from the Sark's Hope mine, Sark, Channel Islands, by Ixer and Stanley (Reference Ixer and Stanley1983), and from the Frische Lutter mine, near Bad Lauterberg, Harz, Germany, by Koch and Heider (Reference Koch and Heider2018). A potential Fe-analogue, ‘zvěstovite-(Fe)’, was described by Gan (Reference Gan1980) from the Manson Lode deposit, North Kentalan, Malaysia.
Table 9. Comparison of members of the zvěstovite and rozhdestvenskayaite series.

Note: ‘rozhdestvenskayaite-(Fe)’ is not currently an approved member of this series.
Stability of Ag-rich members of the tetrahedrite group
The two Ag-pure members of the tetrahedrite group can be considered as mixtures of ZnS with two simple Ag sulfosalts, i.e. ideal zvěstovite-(Zn) as Ag6(Ag4Zn2)As4S13 = 2ZnS + 3Ag3AsS3 + AgAsS2 and ideal rozhdestvenskayaite-(Zn) as Ag6(Ag4Zn2)Sb4S13 = 2ZnS + 3Ag3SbS3 + AgSbS2.
In Ag-rich sulfide ores, such simple Ag sulfosalts are very common and associated frequently with sphalerite, which would favour the formation of these two Ag-pure members of the tetrahedrite group, contrary to the observations. Their rarity may be due to crystal-chemical constraints related to Ag coordination with S. These two tetrahedrite-group minerals have major IVAg+ together with IIIAg+, while simple Ag sulfosalts have major IIIAg+ and IIAg+. Thus, contrary to Cu+, it appears more difficult to stabilise Ag+ in tetrahedral coordination relative to triangular or linear coordination, and it is possible that such a stabilisation is subordinated to low-T conditions.
Conclusion
Zvĕstovite-(Zn) is a new Ag-rich member of the tetrahedrite group, isotypic with rozhdestvenskayaite-(Zn), and the first mineral of a potential new series (i.e. the zvěstovite series). Its crystal chemical study improves the knowledge of this important group of sulfosalts and provides further data for a better understanding of Ag-rich tetrahedrites. Indeed, only accurate structural investigations on samples characterised by different chemical compositions will allow us to achieve a full understanding of the relations between Ag and S contents in these complex chalcogenides, which can pose such severe difficulties in their correct classification.
Acknowledgements
The authors thank Zdeněk Dolníček (National Museum, Praha) for his help with the analytical work and Dalibor Velebil (National Museum, Praha) for useful information about the history and geology of the Zvěstov occurrence. The helpful comments of an anonymous reviewer, Emil Makovicky, Peter Leverett, Associate Editor František Laufek and Principal Editor Stuart Mills are greatly appreciated. The study was supported financially by the Ministry of Culture of the Czech Republic (long-term project DKRVO 2019-2023/1.II.c; National Museum, 00023272) for JS and LV, and by the Ministero dell'Istruzione, Università e Ricerca (project PRIN 2017 “TEOREM – deciphering geological processes using Terrestrial and Extraterrestrial ORE Minerals”, prot. 2017AK8C32) for CB.
Supplementary material
To view supplementary material for this article, please visit https://doi.org/10.1180/mgm.2021.57