Introduction
This article is the first in a series regarding new minerals with REE as species-defining cations (henceforth ‘REE minerals’) from polymineralic nodules found in the Mochalin Log deposit, Chelyabinsk Oblast, South Urals, Russia. The discoveries are the result of a focused study regarding the mineral diversity of this famous rare-earth deposit.
The Mochalin Log REE deposit:
Historical context
Due to its unique rare-earth mineralisation and rich species diversity, Mochalin Log is a famous mineral locality in the South Urals. It is located at 55°48′42″N, 60°33′46″E, in the valley of the Mochalin Log stream (‘log’ in Russian means ‘large gentle ravine’), a tributary of the Borzovka river, in the Potaniny (Potaninskie) Mountains. It is ~10 km N of Kyshtym and ~90 km NNW of Chelyabinsk and 120 km S of Ekaterinburg (Fig. 1).

Fig. 1. Geographical position of the Mochalin Log deposit.
In the 19th century, the area was mined as a placer gold deposit and the first recorded REE mineral, ‘kyshtymoparisite’ (Korovaev, Reference Korovaev1861) later recognised as a member of the bastnäsite group, was reported as early as 1861. By the beginning of the 20th century gold mining had ceased, but the unusual alluvial pebbles and nodules containing REE minerals were abundant on the mine dumps, and began to draw the attention of mineralogical researchers.
The earliest phase of research dates to the late 1920s when Mochalin Log was studied intensively by outstanding Russian mineralogist and geochemist Veniamin Arkadievich Silberminz (1887–1939) and his colleagues. They undertook a geological and petrological review of the deposit and provided detailed descriptions of the multiple dumps that contained significant nodules and numbered these dumps 1–8. The minerals bastnäsite, cerite, chevkinite, ‘lessingite’ (britholite), ‘orthite’ (allanite) and törnebohmite were identified (Silberminz, Reference Silberminz1928, Reference Silberminz1929, Reference Silberminz1930; Alimarin, Reference Alimarin1930; Knipovitch, Reference Knipovitch1930; Kuznetsov, Reference Kuznetsov1930).
From the mid 1950s to mid 1960s the Uralian mineralogist Nikolay Vasilievich Svyazhin (1927–1967) focused on the deposit. He further described the mineralogy of the nodules, noting also ‘cerianite’ and providing improved physical and optical properties alongside chemical data. Of particular note are the reported ratios of Ce/La, OH/F and the first X-ray diffraction (XRD) data (Svyazhin, Reference Svyazhin1956, Reference Svyazhin1962, Reference Svyazhin1965a,Reference Svyazhinb; Yunikov and Svyazhin, Reference Yunikov and Svyazhin1965).
At the turn of the 20th Century, one of the authors (I.V.P) focused his attention on the deposit, culminating in its attribution as type locality for two minerals, hydroxylbastnäsite-(Ce) (the ‘kyshtymoparisite’ reported by Silberminz in 1929) and törnebohmite-(La) [a new species proposed by Levinson (Reference Levinson1966) based on data from Svyazhin (Reference Svyazhin1962)]. Furthermore, several nodules were analysed with electron microprobe techniques (EMPA) and powder XRD, providing important new data on the bastnäsite group and lanthanide composition in other REE minerals (Pekov et al., Reference Pekov, Alimova, Kononkova and Kanonerov2002; Alimova et al., Reference Alimova, Pekov, Kononkova and Kanonerov2003).
The current study was initiated in 2017 to focus on mineral species diversity at the locality and build on the work of those listed above. So far, 380 nodules have been investigated and 300 have been shown to contain REE minerals. All nodules have been studied using scanning electron microscopy (SEM) and EPMA, and grains separated from ~40 nodules have been investigated by other analytical methods including, but not limited to, single-crystal XRD, powder XRD, Raman and infrared (IR) spectroscopy. Selected results of these analyses are presented herein and in the accompanying series of articles to be published.
Geological context
The Mochalin Log deposit is related to the southern contact zone of the Vishnevogorskiy alkaline (syenite–miaskite) intrusive complex, this is composed mainly of miaskites, alkaline syenites and granite–gneisses with minor amphibolites (Fig. 2). The miaskites are typically composed of microcline, nepheline, albite and biotite (mainly annite, rarely Fe-enriched phlogopite) with accessory magnetite, rutile (Nb-enriched variety, so-called ilmenorutile), fluorapatite, titanite, zircon and secondary zeolites. In the peripheral parts of the bodies, these transition into alkaline syenites composed mainly of albite and microcline with alkali pyroxene and minor biotite, quartz and titanite. The alkaline syenites in turn transition into the granite–gneisses which occupy the central part of the Mochalin Log valley. They are composed of quartz, microcline, albite (including plagioclase with composition Ab86An14) and aegirine–augite with accessory fluorapatite, titanite and zircon. The rare amphibolites are composed of approximately equal amounts of plagioclase (Ab70An30) and amphibole-group minerals with very minor quartz, titanite, fluorapatite and diopside (Silberminz, Reference Silberminz1930). The current work has incidentally identified that the amphiboles are represented by hornblende- (magnesio-hornblende, magnesio-ferri-hornblende and ferro-ferri-hornblende) and hastingsite- (hastingsite ss, magnesio-hastingsite, potassic-hastingsite and potassic-magnesio-hastingsite) series minerals.

Fig. 2. Geological map of the Mochalin Log deposit (modified after Kuznetsov, Reference Kuznetsov1930).
The rich REE mineralisation, presented in nodules of the Mochalin Log placer deposit, has a contact metasomatic origin. Although not confirmed, it was probably formed during fenitisation of granitic pegmatites located within granite–gneisses in the exocontact zone of the alkaline intrusion of Potaniny Mts.
A detailed description of the geology and petrology of the deposit can be found elsewhere, such as Silberminz (Reference Silberminz1930), Kuznetsov (Reference Kuznetsov1930) and Svyazhin (Reference Svyazhin1956).
Mineralogical context
The great diversity of REE minerals forming nodules are the outstanding mineralogical feature of the Mochalin Log deposit. Most nodules have been collected from dumps 2, 5, 7, 8 and in a dump hereby designated ‘2bis’ that was uncovered in 2018 (Figs 2, S1). The typical nodule size is 1 to 7 cm (Fig. S2a) however, the biggest recorded was 12 cm across, weighing 2 kg (Silberminz, Reference Silberminz1930). It is still possible to find large nodules and in 2019 one of the authors (A.M.K.) found at dump 7 a nodule measuring 11 cm × 10 cm × 6 cm, weighing 1.1 kg (Fig. S2b).
Thirty-seven REE mineral species have been identified from Mochalin Log for the first time during our works, eight of which are new to science and have been approved by the Commission on New Minerals, Nomenclature and Classification (CNMNC) of the International Mineralogical Association (IMA), two of those being the focus of the latter part of this article. This brings the total of all recognised REE minerals at the deposit to 51. Alongside species identification, the frequency of species occurrence in the 300 REE-bearing nodules studied has been noted and rated; abundant (n > 100 nodule occurrences), common (n = 50–99), subordinate (n = 20–49), rare (n = 5–19) and very rare (n < 5). An overview of this data gathering exercise is presented in Table 1.
Table 1. REE minerals of the Mochalin Log deposit and their distribution (based on our data).

Notes: Distribution of minerals: ХХХХХ – abundant, ХХХХ – common, ХХХ – subordinate, ХХ – rare, Х – very rare.
Minerals for which Mochalin Log deposit is type locality are given in italic. Those of them that are discovered by us are given in bold italic with IMA numbers in square brackets.
* Formulae are given in accordance with the New IMA List of Minerals (Pasero, Reference Pasero2020).
** Minerals identified by the authors for the first time at the deposit.
$ According to our data; in the literature, however, cerite-(Ce) is mentioned as common (Silberminz, Reference Silberminz1928, Reference Silberminz1929, Reference Silberminz1930; Yunikov and Svyazhin, Reference Yunikov and Svyazhin1965).
? Mineral is mentioned in the literature but its identification remains questionable.
1 Minerals associated with ferriperboeite-(La) and perboeite-(La).
Each nodule typically contains several REE minerals, 8 to 15 distinct species is common but as many as 21 have been found. Monomineralic nodules are very rare, only four were identified composed completely of bastnäsite-(Ce), bastnäsite-(La), ferriallanite-(Ce) and perrierite-(Ce). Previously, Alimova et al. (Reference Alimova, Pekov, Kononkova and Kanonerov2003) recorded several monomineralic allanite nodules.
Associated non-REE minerals in these nodules are numerous but usually very minor by volume. The full list of these species and their distribution in the 300 REE nodules studied, using the same scale as for REE minerals, are given in Supplementary material (Table S1).
Regarding general distribution of REE in the nodules, La and Ce significantly dominate other light rare earth elements (LREE) detectable by EPMA that shows Nd > Pr > Sm. In general, nodules show very steep LREE enrichment followed by heavy rare earth element (HREE) impoverishment in chondrite-normalised REE patterns. Four ‘very rare’ (n = 1) species were identified with a REE dominance other than La or Ce, aeschynite-(Nd), aeschynite-(Y), fergusonite-(Y) and xenotime-(Y). All were found in nodules composed dominantly of the non-REE minerals albite, microcline and quartz, so it is suggested these represent a different assemblage with a paragenesis related to the common granitic pegmatites.
The gross La:Ce ratio varies widely from one nodule to another but is often close to 1:1, therefore both Ce- and La-dominant members of the same mineral series or group may occur in the same specimen, examples include, allanite, bastnäsite, ferriallanite, monazite and perrierite and the later focus of this paper, ferriperbøeite and perbøeite. It should be noted that the absence of a ‘Levinson suffix’ in the text below should be interpreted as the presence of both Ce- and La-dominant mineral species together.
A significant exception to this is fluorbritholite, which was ‘abundant’ (n > 100), yet only twice showed a slight dominance of La over Ce, but not enough to consistently characterise what would be the new mineral species ‘fluorbritholite-(La)’. Even in the nodules where all other REE minerals are La-dominant, fluorbritholite showed Ce >> La.
The REE-bearing nodules can be divided into two main types on the basis of their internal textures. Type 1 shows concentric zonation and are typically ovoid, the core is bastnäsite, an intermediate zone is perbøeite/ferriperbøeite and/or törnebohmite and/or fluorbritholite-(Ce) while the rim is allanite/ferriallanite (Fig. 3). The ovoid structures were reported previously (e.g. Silberminz, Reference Silberminz1930; Svyazhin, Reference Svyazhin1956; Alimova et al., Reference Alimova, Pekov, Kononkova and Kanonerov2003) and while these works mention identical core (bastnäsite) and rim (allanite) minerals, the middle zone is mainly reported as cerite-(Ce), which in the literature is reported as a common mineral from the locality (Silberminz, Reference Silberminz1928, Reference Silberminz1929, Reference Silberminz1930; Yunikov and Svyazhin, Reference Yunikov and Svyazhin1965). This is in direct contradiction with results presented herein, where cerite-(Ce) was found in only two nodules.
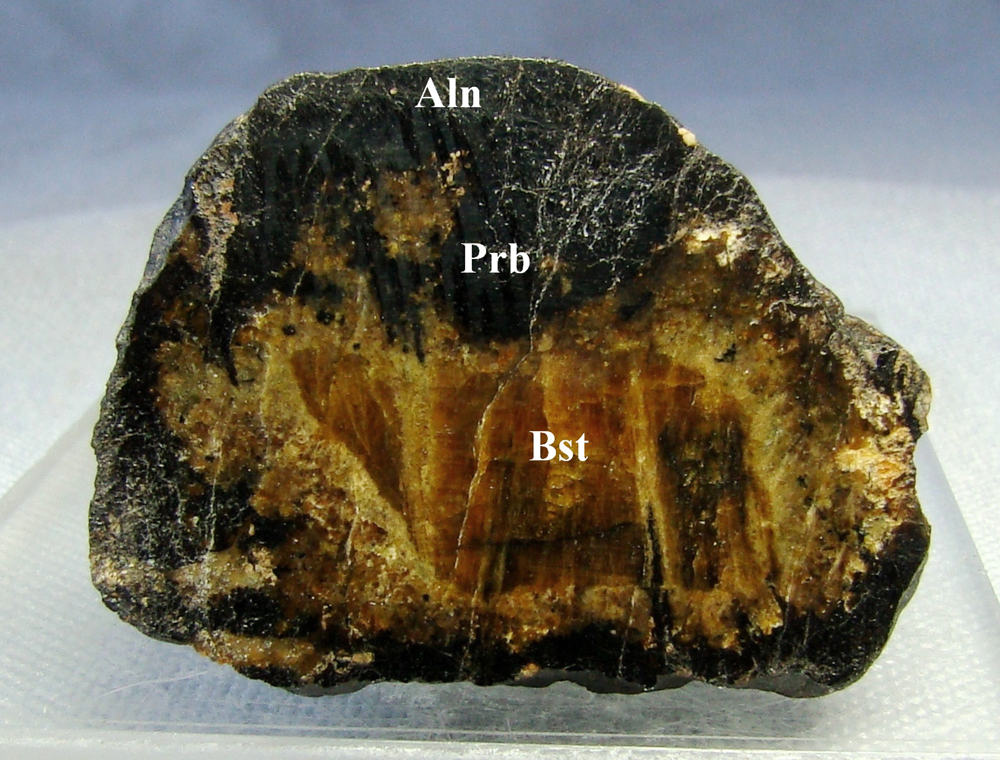
Fig. 3. Cut and polished nodule showing typical ovoidal structure: orange-yellow core of bastnäsite (Bst), black middle zone of perbøeite/ferriperbøeite (Prb) and black rim of allanite/ferriallanite (Aln). Size of the sample: 2 cm × 1.7 cm. Photo: A.M. Kuznetsov, specimen no. ML 59-2.
In type 2 nodules, REE minerals form thin and chaotic intergrowths with each other, typically composed of allanite/ferriallanite, bastnäsite, fluorbritholite-(Ce), monazite, perbøeite/ferriperbøeite, perrierite and törnebohmite (Fig. 4).

Fig. 4. Cut and polished section of nodule showing black ferriperbøeite-(La) (Fpb) intergrown with black allanite/ferriallanite (Aln), yellow and brown bastnäsite (Bst) and greenish to yellow-greenish törnebohmite (Tnb). Size of the nodule: 2.2 cm × 1.3 cm. Photo: A.M. Kuznetsov, specimen no. ML 56-2.
Mochalin Log shows a similarity to the Bastnäs-type deposits at Riddarhyttan, Västmanland, Sweden (Öhman et al., Reference Öhman, Nysten and Langhof2004; Holtstam and Andersson, Reference Holtstam and Andersson2007) and Jamestown, Colorado, USA (Allaz et al., Reference Allaz, Raschke, Persson and Stern2015), but has much greater REE mineral species diversity; it should be noted that all these occurrences differ in their attributed origin.
Ferriperbøeite-(La) and perbøeite-(La)
Ferriperbøeite-(La) [Russian Cyrillic: феррипербёит-(La)], ideally (CaLa3)(Fe3+Al2Fe2+)[Si2O7][SiO4]3O(OH)2, and perbøeite-(La) [Russian Cyrillic: пербёит-(La)], ideally (CaLa3)(Al3Fe2+)[Si2O7][SiO4]3O(OH)2 are named in accordance with the gatelite supergroup nomenclature scheme (Bonazzi et al., Reference Bonazzi, Holtstam and Bindi2019) as analogues of ferriperbøeite-(Ce) (Bindi et al., Reference Bindi, Holtstam, Fantappiè, Andersson and Bonazzi2018) and perbøeite-(Ce) (Bonazzi et al., Reference Bonazzi, Lepore, Bindi, Chopin, Husdal and Medenbach2014), respectively, with La as the dominant REE.
Both new minerals and their names have been approved by the IMA-CNMNC as IMA2018-106 [ferriperbøeite-(La), Kasatkin et al., Reference Kasatkin, Pekov, Zubkova, Chukanov, Škoda, Polekhovsky, Belakovskiy, Agakhanov, Kuznetsov and Pushcharovsky2018] and IMA2018-116 [perbøeite-(La), Kasatkin et al., Reference Kasatkin, Pekov, Zubkova, Chukanov, Škoda, Polekhovsky, Belakovskiy, Agakhanov, Kuznetsov and Pushcharovsky2019]. The holotype specimens are deposited in the systematic collection of the Fersman Mineralogical Museum of the Russian Academy of Sciences, Moscow with the catalogue numbers 96276 [ferriperbøeite-(La)] and 96484 [perbøeite-(La)].
Occurrence and general appearance
Nodules containing both new minerals were first found in 1980s by local collectors at dump no. 2 (Fig. S1a) and preserved in the private collection of one of the authors (A.M.K.). In 2017 the specimens were cut, polished and given to the senior author (A.V.K.) for routine EMPA. This revealed two, then potentially new, REE minerals and started the characterisation and systematic study of the REE species diversity at Mochalin Log.
Ferriperbøeite-(La) and perbøeite-(La) occur in the polymineralic nodules composed mainly of bastnäsite, allanite/ferriallanite and törnebohmite (Figs 3–7). Each new mineral forms isolated anhedral grains, up to 0.3 mm for ferriperbøeite-(La) and 0.5 mm for perbøeite-(La), and granular aggregates, up to 1.5 mm × 0.5 mm for ferriperbøeite-(La) and 3 mm × 1 mm for perbøeite-(La). The nodules often have a concentrically zoned, ovoid structure with a core of bastnäsite, an intermediate zone of ferriperbøeite-(La)/perbøeite-(La) and a rim of allanite/ferriallanite (Figs 3, 7). The new minerals are typically intimately intergrown with, or overgrown by törnebohmite and when aggregated contain inclusions of allanite/ferriallanite.

Fig. 5. Ferriperbøeite-(La) (Fpb) associated with bastnäsite-(Ce) (Bst), törnebohmite-(La) (Tnb) and radekškodaite-(La) (Rsk). Dark-grey inclusions in ferriperbøeite-(La) are allanite-(La)/ferriallanite-(La). Black grains are quartz. In the right upper corner there is a trace from the grain extracted for the structural investigation. Polished section. SEM (back-scattered electron) image, scale bar 500 μm, specimen no. ML 56-2.
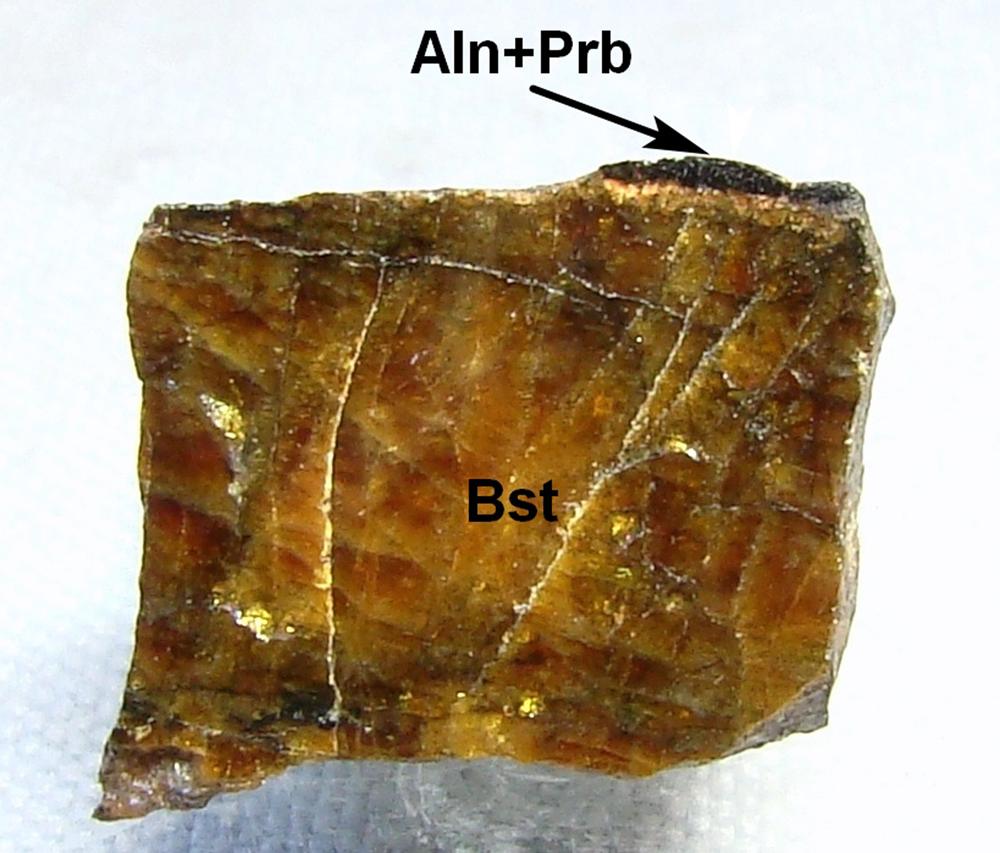
Fig. 6. Cut and polished section of nodule (fragment) showing thin black crust of perbøeite-(La) (Prb) together with allanite-(La)/ferriallanite-(La) (Aln) (see Fig. 7) on orange-yellow bastnäsite (Bst). Size of the sample: 1.5 cm × 1 cm. Photo: A.M. Kuznetsov, specimen no. ML 24-2.

Fig. 7. Section of a crust (see Fig. 6) mainly consisting of perbøeite-(La) (Prb) which is ‘sandwiched’ between a bastnäsite-(Ce) core (Bst) and allanite-(La)/ferriallanite-(La) rim (Aln). Dark-grey inclusions in perbøeite-(La) are allanite-(La)/ferriallanite-(La), black grains are quartz. Polished section. SEM (back-scattered electron) image, scale bar 500 μm, specimen no. ML 24-2.
Ferriperbøeite-(La) and perbøeite-(La) should be considered ‘common’ minerals at Mochalin Log (Table 1), during this study, ferriperbøeite-(La) was found without perbøeite-(La) in 42 nodules, perbøeite-(La) was found without ferriperbøeite-(La) in 39 and the two minerals were found together in another 40; most of these nodules were from dumps nos. 2, 2bis and 7.
Ferriperbøeite-(La) and perbøeite-(La) occur in an assemblage rich in REE minerals. Apart from the texturally dominant species mentioned above, associated minerals also include 25 other REE minerals, two non-REE minerals that are in this case REE-bearing (huttonite and epidote), and 19 other non-REE minerals. All of these are marked with 1 symbol in Tables 1 and S1.
Physical properties and optical data
Both new minerals are brownish-black, translucent in thin fragments with brown streak and vitreous lustre. They are non-fluorescent under ultraviolet light. Both are brittle, cleavage is good on {100} and imperfect on {001}, parting is not observed and fracture is uneven (observed under the scanning electron microscope). Their Mohs’ hardness is ~6, based on scratch tests. Their density could not be measured due to lack of pure material in suitable grains, so density values were calculated as 4.510 g cm–3 for ferriperbøeite-(La) and 4.483 g cm–3 for perbøeite-(La) using the empirical formulae and the unit-cell parameters from single-crystal XRD data.
Both minerals are optically biaxial (+). Ferriperbøeite-(La) has α = 1.788(5), β = 1.790(5), γ = 1.810(5) (589 nm), 2Vmeas = 40(10)° and 2Vcalc = 35°. Dispersion of optical axes was not observed. The mineral is weakly pleochroic from nearly colourless in thin grains or pale-brownish in thicker ones (X) to greenish-brown (Y and Z). The absorption scheme is Z > Y > X. Perbøeite-(La) has α = 1.778(8), β = 1.783(8), γ = 1.805(8) (589 nm), 2Vmeas = 40(10)° and 2Vcalc = 51.5°. Dispersion of optical axes is noticeable, inclined. Perbøeite-(La) is strongly pleochroic: X = colourless, Y = medium-dark brown, Z = nearly colourless or brownish. The absorption scheme is Y > Z ~ X. the optical orientation could not be determined for either mineral due to the anhedral shape of their grains.
In reflected light, both minerals are dark grey, anisotropy is weak for ferriperbøeite-(La) and very weak for perbøeite-(La). Bireflectance is very weak, ΔR = 0.5% for ferriperbøeite-(La) and 0.3% (589 nm) for perbøeite-(La). The reflectance values, (R max/R min) for both minerals are given in Table 2, and were measured in air using a MSF-21 microspectrophotometer (LOMO company, St. Petersburg, Russia) with a monochromator slit width of 0.4 mm, beam diameter of 0.1 mm and SiC (474251, No. 545, Germany) used as a standard.
Table 2. Reflectance data (R, %) of ferriperbøeite-(La) [1] and perbøeite-(La) [2].

Data for wavelengths recommended by the IMA Commission on ore microscopy (COM) are marked in boldtype.
Neither mineral reacted with cold hydrochloric or nitric acid.
Raman spectroscopy
The Raman spectra (Figs 8 and 9) of ferriperbøeite-(La) and perbøeite-(La) were obtained from polished sections using a Horiba Labram HR Evolution spectrometer. This dispersive, edge-filter-based system is equipped with an Olympus BX 41 optical microscope, a diffraction grating with 600 grooves per millimetre, and a Peltier-cooled, Si-based charge-coupled device (CCD) detector. After careful tests with different lasers (473, 532 and 633 nm), the 633 nm He–Ne laser with the beam power of 10 mW at the sample surface was selected for acquisition to minimise analytical artefacts. The Raman signal was collected in the range of 100–4000 cm–1 with a 100× objective and the system being operated in confocal mode, beam diameter was ~1 μm and the lateral resolution ~2 μm. No visual damage of the analysed surface was observed at these conditions after the excitation. Wavenumber calibration was done using the Rayleigh line and low-pressure Ne-discharge lamp emissions. The wavenumber accuracy was ~0.5 cm–1, and the spectral resolution was ~2 cm–1. Band fitting was done after appropriate background correction, assuming combined Lorentzian-Gaussian band shapes using the Voight function (PeakFit, Jandel Scientific Software).

Fig. 8. Raman spectra of perbøeite-(La) (a) and ferriperbøeite-(La) (b) in the 100–1250 cm–1 region. The measured spectra are shown by dots. The curves matched to dots are a result of spectral fit as a sum of individual Voigt peaks shown below the curves.

Fig. 9. Raman spectra of perbøeite-(La) (a) and ferriperbøeite-(La) (b) in the 2900–3600 cm–1 region. The measured spectra are shown by dots. The curves matched to dots are a result of spectral fit as a sum of individual Voigt peaks shown below the curves.
The spectra of both minerals are very similar. Bands in the 3200–3350 cm–1 area correspond to O–H stretching vibrations of the O10–H and O11–H groups. The assignment of each band to a particular group is difficult, as the angle ∠(D–Н⋅⋅⋅A) and the distance D⋅⋅⋅A contribute with opposite signs to the difference in the energies of hydrogen bonds formed by these groups (see structural data below). It is to be noted that correlations between wavenumbers of O–H stretching vibrations and lengths of hydrogen bonds (Libowitzky, Reference Libowitzky1999) cannot be applied to compare situations with significantly different D–Н⋅⋅⋅A angles. The ill-defined shoulder at 3437 cm–1 in the Raman spectrum of perbøeite-(La) could be due to adsorbed water or (taking into account minor amounts of Fe in the M2 site) O–H-stretching vibrations of the Fe⋅⋅⋅O–Н⋅⋅⋅O fragments. Bands and shoulders in the 1000–1100 cm–1 range correspond to stretching vibrations of the Si–O–Si fragments in Si2O7 groups, those in the 850–1000 cm–1 region, to stretching vibrations of apical Si–O bonds, and those in the 660–700 cm–1 area to Al–O⋅⋅⋅H bending vibrations. Bands in the 300–630 cm–1 range are due to mixed modes and overlapping bands of Al–O and Fe3+–O stretching vibrations, as well as bending vibrations of silicate groups, while those with frequencies lower than 300 cm–1 correspond to lattice modes involving REE–O-, Ca–O- and Fe2+–O-stretching vibrations and librational vibrations of silicate groups. The Raman spectra of ferriperbøeite-(La) and perbøeite-(La) are similar to that of västmanlandite-(Ce) (Holtstam et al., Reference Holtstam, Kolitsch and Andersson2005) in the range of 300–1100 cm–1 but significantly differ from the latter in the regions of O–H stretching vibrations (above 3200 cm–1) and stretching vibrations involving REE and divalent cations forming low-force-strength bonds (below 300 cm–1).
The bands at 971, 692 and 413 cm–1 in the Raman spectrum of ferriperbøeite-(La) and those at 969, 690 and 415 cm–1 in the Raman spectrum of perbøeite-(La) are close to strong bands in the Raman spectrum of allanite-(Ce) observed at 972, 689 and 421 cm–1 (Andò and Garzanti, Reference Andò and Garzanti2014; Čopjaková et al., Reference Čopjaková, Škoda, Vašinová Galiová, Novák and Cempírek2015) and can be assigned tentatively to the epidote-type module (see below).
Infrared spectroscopy
Due to a lack of sizeable pure material, an IR absorption spectrum of perbøeite-(La) could not be obtained, and a spectrum for ferriperbøeite-(La) (Fig. 10) was obtained only after subtracting contributions from ferriallanite-(La) using a pure ferriallanite-(La) standard from the same assemblage as a comparator. Hand-picked fragments, ultimately composed of both ferriallanite-(La) and ferriperbøeite-(La) were ground in an agate mortar and pestle, mixed with anhydrous KBr, pelletised, and analysed using an ALPHA FTIR spectrometer (Bruker Optics) in the range of wavenumbers from 360 to 3800 cm–1, with a spectral resolution of 4 cm–1. The IR spectrum of a pure KBr disk was used as a reference.

Fig. 10. Infrared absorption spectrum of ferriperbøeite-(La).
Due to a rather strong scattering in the high-frequency region, bands of O–H-stretching vibrations are indistinct and could be determined only approximately (as a broad absorption band at ~3130 cm–1 and a shoulder at ~3270 cm–1). The assignment of other IR absorption bands is as follows. Bands in 1000–1100 cm–1 area correspond to stretching vibrations of Si–O–Si fragments in Si2O7 groups, those in the 830–1000 cm–1 region, to stretching vibrations of apical Si–O bonds and, finally, those in the range of 360–630 cm–1, to mixed modes and overlapping bands of Al–O and Fe3+–O stretching vibrations, as well as bending vibrations of silicate groups.
Unlike allanite-group minerals, where the strongest band of Si–O-stretching vibrations in IR spectra is observed between 915 and 940 cm–1 and belongs to the Si2O7 groups (Chukanov, Reference Chukanov2014; Chukanov and Chervonnyi, Reference Chukanov and Chervonnyi2016), the main IR absorption band of ferriperbøeite-(La) has a maximum at 870 cm–1 which corresponds to vibrations of SiO4 groups.
Chemical composition
Chemical data for both new minerals were obtained using a Cameca SX-100 electron microprobe (wavelength-dispersive spectroscopy mode, acceleration voltage of 15 kV, a beam current of 20 nA, and a 3 μm beam diameter). The spectral interference of FKα and CeMζ were corrected manually using empirically determined correction factors. H2O was not determined directly due to the scarcity of pure material and was calculated by stoichiometry on the basis of O20(OH,F)2 taking into account that bond-valence sums for the sites O(10) and O(11) are close to 1.0 (see below). Both crystal structure and Raman spectroscopy data confirm the presence of OH groups and the absence of carbonate and borate groups in both new species.
Despite the fact that ferriperbøeite-(La) and perbøeite-(La) are ‘common’ at the deposit, attempts to determine the valence state of iron using Mössbauer spectroscopy were unsuccessful due to lack of pure material: small but abundant inclusions of allanite/ferriallanite in both new minerals significantly affected the reproducibility of the data. For this reason, the Fe3+:Fe2+ ratio reported herein is based on the bond-valence calculations and charge-balance requirements.
Chemical data are presented in Table 3, any other element with atomic numbers higher than that of carbon, not listed in Table 3, were below detection limits.
Table 3. Average chemical composition of ferriperbøeite-(La) and perbøeite-(La) (wt.%).

S.D. = standard deviation
* Apportioned from total Fe measured as FeO content of 7.82 wt.% for ferriperbøeite-(La) and 6.75 wt.% for perbøeite-(La) in accordance with bond-valence sums for the cationic sites and charge-balance requirement for the empirical formulae.
** For total iron calculated as FeO.
$ Calculated by stoichiometry.
‘–’ = content of a constituent is below the detection limit.
The empirical formulae calculated on the basis of O20(OH,F)2 are: ferriperbøeite-(La), (Ca0.95La1.58Ce1.30Nd0.10Pr0.06)Σ3.99(Al2.27Fe3+0.72Fe2+0.46Mg0.40Mn0.16Ti0.03)Σ4.04Si4.96O20[(OH)1.94F0.06] and perbøeite-(La), (Ca0.94Th0.01La1.49Ce1.34Nd0.14Pr0.07)Σ3.99(Al2.42Fe3+0.52Fe2+0.51Mg0.38Mn0.14Ti0.03)Σ4.00Si4.99O20[(OH)1.87F0.13].
The idealised formulae are as follows. Ferriperbøeite-(La): (CaLa3)(Fe3+Al2Fe2+)[Si2O7][SiO4]3O(OH)2 which requires CaO 5.03, FeO 6.43, La2O3 43.76, Fe2O3 7.15, Al2O3 9.14, SiO2 26.88, H2O 1.61, total 100 wt.%. Perbøeite-(La): (CaLa3)(Al3Fe2+)[Si2O7][SiO4]3O(OH)2 which requires CaO 5.14, FeO 6.58, La2O3 44.93, Al2O3 14.07, SiO2 27.62, H2O 1.66, total 100 wt.%.
X-ray crystallography
Powder XRD data for both minerals were collected with a Rigaku R-AXIS Rapid II single-crystal diffractometer equipped with cylindrical image plate detector (radius 127.4 mm) using Debye-Scherrer geometry, CoKα radiation (rotating anode with VariMAX microfocus optics), 40 kV, 15 mA and an exposure of 15 min. Angular resolution of the detector is 0.045°2θ (pixel size 0.1 mm). The data were integrated using the software package osc2tab (Britvin et al., Reference Britvin, Dolivo-Dobrovolsky and Krzhizhanovskaya2017). The powder XRD data for both minerals are given in Supplementary material (Table S2 and Table S3, see below). Both minerals are monoclinic, with unit-cell parameters calculated from the powder data, a = 8.951(5), b = 5.737(2), c = 17.65(1) Å, β = 115.97(4)° and V = 815(1) Å3 for ferriperbøeite-(La) and a = 8.964(8), b = 5.738(2), c = 17.69(2) Å, β = 116.04(5)° and V = 817(1) Å3 for perbøeite-(La).
Single-crystal X-ray studies of both new minerals were carried out using an Xcalibur S diffractometer equipped with a CCD detector. A full sphere of three-dimensional data was collected. Data reduction was performed using CrysAlisPro Version 1.171.37.35 (Agilent Technologies, 2014). The data were corrected for Lorentz factor and polarisation effect.
The crystal structure of ferriperbøeite-(La) was solved by direct methods and refined with the use of the SHELX-97 software package (Sheldrick, Reference Sheldrick2008) to R = 0.0355 for 2532 unique reflections with I > 2σ(I). The crystal structure of perbøeite-(La) was refined to R = 0.0752 for 2040 unique reflections with I > 2σ(I) on the basis of atomic coordinates of ferriperbøeite-(La). The H atoms of the OH groups of both minerals were located from the difference-Fourier synthesis and their positions were restricted to keep O–H distances of 0.85(1) Å and U iso (H) = 1.2 U eq (O).
The crystal dimensions, data collection information and structure refinement details for ferriperbøeite-(La) and perbøeite-(La) are given in Table 4, atomic coordinates, thermal displacement parameters of atoms and site occupancies in Table 5 and selected interatomic distances in Table 6. Hydrogen bond lengths are in Table 7 and bond-valence calculations are given in Supplementary material (Table S4).
Table 4. Crystal data, data collection information and structure refinement details for ferriperbøeite-(La) and perbøeite-(La).
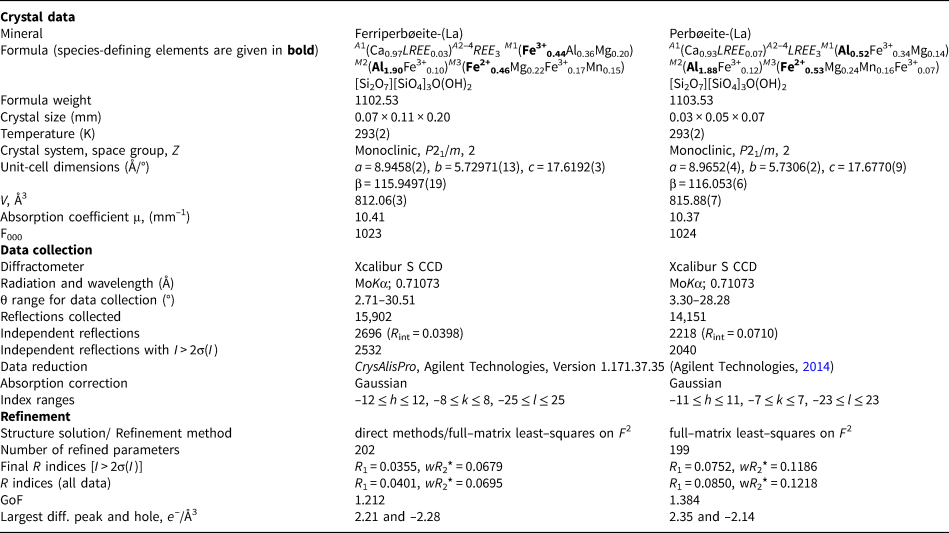
*w = 1/[σ2(Fo 2) + (0.0186P)2 + 5.3816P]; P = ([max of (0 or Fo 2)] + 2Fc 2)/3 for ferriperbøeite-(La).
w = 1/[σ2(Fo 2) + (0.0240P)2 + 16.4905P]; P = ([max of (0 or Fo 2)] + 2Fc 2)/3 for perbøeite-(La).
Table 5. Coordinates, equivalent displacement parameters (U eq, in Å2) and anisotropic displacement parameters of atoms (excepting H) and site occupancy factors (s.o.f.) for ferriperbøeite-(La) and perbøeite-(La).

* Fixed during the refinement.
For ferriperbøeite-(La): M(1) Al vs. Fe was refined (e ref 18.46), for M(3) Fe vs. Mg was refined (e ref 22.78). Thus, based on chemical data, cation-anion distances, charge-balance requirements and e ref, M(1) site was assumed to be occupied by (Fe3+0.44Al0.36Mg0.20) possibly with minor Ti admixture; M(3) octahedron – by (Fe2+0.46Mg0.22Fe3+0.17Mn0.15).
For perbøeite-(La): M(1) Al vs. Fe was refined (e ref 17.24), for M(3) Fe vs. Mg was refined (e ref 22.47). Thus, based on chemical data, cation-anion distances, charge-balance requirements and e ref, the M(1) site was assumed to be occupied by (Al0.52Fe3+0.34Mg0.14), possibly with minor Ti admixture, and M(3) by (Fe2+0.53Mg0.24Mn0.16Fe3+0.07).
** U iso.
Table 6. Selected interatomic distances (Å) and angles (°) in the structure of ferriperbøeite-(La) and perbøeite-(La).

Table 7. Hydrogen-bond lengths (d in Å) and angles (in °) for ferriperbøeite-(La) and perbøeite-(La).

D – donor; A – acceptor.
The crystallographic information files for both minerals have been deposited with the Principal Editor of Mineralogical Magazine and are available as Supplementary material (see below).
Crystal structure
Ferriperbøeite-(La) and perbøeite-(La) are isostructural (Fig. 11). They are two new members of the recently IMA-approved gatelite supergroup (Bonazzi et al., Reference Bonazzi, Holtstam and Bindi2019) which includes minerals with the general formula A 4M 4(Si2O7)(SiO4)3(O,F)(OH)2. The A sites are occupied by cations in large cavities: Ca (A1) and REE +/– Na (A2, A3 and A4). The M sites are octahedrally coordinated and occupied by di- and trivalent cations, i.e. Al, Fe2+, Fe3+, Mg, Mn2+ and Mn3+. Lower in the hierarchy, the gatelite supergroup is divided into three mineral groups. Ferriperbøeite-(La) and perbøeite-(La) should be included into the gatelite group along with gatelite-(Ce) (CaCe3)(Al3Mg)[Si2O7][SiO4]3O(OH)2 (Bonazzi et al., Reference Bonazzi, Bindi and Parodi2003), perbøeite-(Ce) (CaCe3)(Al3Fe2+)[Si2O7](SiO4)3O(OH)2 (Bonazzi et al., Reference Bonazzi, Lepore, Bindi, Chopin, Husdal and Medenbach2014) and ferriperbøeite-(Ce) (CaCe3)(Fe3+Al2Fe2+)[Si2O7][SiO4]3O(OH)2 (Bindi et al., Reference Bindi, Holtstam, Fantappiè, Andersson and Bonazzi2018). This group includes members with formulae that can be derived from gatelite-(Ce) solely by homovalent substitutions. The key cation and anion sites for this group are: A1 = A2+; A2, A3 and A4 = A3+; M1 = M3+; M2 = M3+; M3 = M2+; O4 = O2-; O10 and O11 = (OH)– (Bonazzi et al., Reference Bonazzi, Holtstam and Bindi2019). Thus, the species-defining cations for ferriperbøeite-(La) are Ca (A1), La (A2–4), Fe3+ (M1), Al (M2), and Fe2+ (M3); for perbøeite-(La) these are Ca (A1), La (A2–4), Al (M1), Al (M2) and Fe2+ (M3) [Table 4]. For comparison of all five members of the gatelite group see Table 8.

Fig. 11. The crystal structure of ferriperbøeite-(La)/perbøeite-(La). SiO4 tetrahedra are red. H atoms of OH groups are shown as small blue circles. Alternation of (001) epidote-type slabs (E) and (${\bar 1}$02) törnebohmite-type slabs (T) is shown. The unit cell is outlined.
Table 8. Comparative data for the members of the gatelite group.

All the minerals of the gatelite supergoup are considered as iso-topological ET type polysomes within a polysomatic series which has epidote-type (E) and törnebohmite-type (T) structures as end-members. Their structure can be described as a regular alternating 1:1 stacking of slabs of (E) and (T) structures (Bonazzi et al., Reference Bonazzi, Lepore, Bindi, Chopin, Husdal and Medenbach2014, Reference Bonazzi, Holtstam and Bindi2019). The E modules are (001) slabs with the general composition A 2M1M2M3[Si2O7][SiO4]X(OH), where X = O2– and/or F– and the T modules are (${\bar 1}$02) slabs with the composition [REE 2Al(SiO4)2(OH)] (Fig. 11). In ferriperbøeite-(La) and perbøeite-(La), the T module is the same and corresponds to a törnebohmite-(La) composition, but the E module is different and has a ferriallanite-(La) composition in ferriperbøeite-(La) and an allanite-(La) composition in perbøeite-(La).
The crystal structure of ferriperbøeite-(La) and perbøeite-(La) consists of chains of edge-sharing octahedra running along the b axis: single chains of the M(2)-centred octahedra and branched chains with the M(1)-centred octahedra in the central part and the M(3)-centred octahedra attached to them from both sides. The chains are linked via isolated [SiO4] tetrahedra and disilicate groups [Si2O7]. The A(1–4) sites occur in large cavities. The angle Si(1)–O(9)–Si(2) in the disilicate group for both species is similar [148.7(4)° in ferriperbøeite-(La) and 148.3(8)° in perbøeite-(La)] and lies between the values reported for allanite- and epidote-group minerals: e.g. in ferriallanite-(La) this angle is equal to 143.61(12)° (Kolitsch et al., Reference Kolitsch, Mills, Miyawaki and Blass2012) and in epidote to 154.59(9)° (Gatta et al., Reference Gatta, Meven and Bromiley2010).
The cation distribution (see below) was determined on the basis of chemical data, cation–anion distances, charge-balance requirements and the refined number of electrons (e ref) values.
Despite La3+ being the dominant REE in ferriperbøeite-(La) and perbøeite-(La), the Ce scattering curve was used during the structure refinement of both minerals because of the significant content of Ce and only minor amounts of heavier Nd, Pr and, in the case of perbøeite-(La), very minor Th. According to refinement of the site occupation factors, the A(2–4) sites are occupied by LREE cations and the A(1) site is Ca-dominant with only a minor REE admixture: the refined site populations are Ca0.967(3)LREE 0.033(3) in ferriperbøeite-(La) and Ca0.934(7)LREE 0.066(7) in perbøeite-(La) Thus, the total composition of the A sites is [LREE 3.03Ca0.97] in ferriperbøeite-(La) and [LREE 3.07Ca0.93] in perbøeite-(La), which is in a good agreement with electron microprobe data (Table 3).
There are three octahedrally coordinated M sites, M(1–3). For the M(1) and M(2) sites, Al vs. Fe was refined, and for the M(3) site, Fe vs. Mg was refined. The M(2) site is Al-dominant in both new species with the refined population Al0.953(6)Fe0.047(6) in ferriperbøeite-(La) and Al0.936(13)Fe0.064(13) in perbøeite-(La). This site centres the smallest octahedron with the mean M(2)–O distance in ferriperbøeite-(La)/perbøeite-(La) being 1.912/1.913 Å, respectively.
The M(1)-centred octahedron is larger, with the mean M(1)–O distance in ferriperbøeite-(La)/perbøeite-(La) of 1.975/1.973 Å. The e ref in the M(1) site is 18.46 in ferriperbøeite-(La) and only 17.24 in perbøeite-(La). This is the main difference between the two minerals. In ferriperbøeite-(La) this site is determined to be occupied predominantly by Fe3+ with subordinate Al and Mg cations in the atomic ratio Fe3+:Al:Mg = 0.44:0.36:0.20, while in perbøeite-(La) it is determined to be occupied predominantly by Al with subordinate Fe3+and Mg cations in the atomic ratio Al:Fe3+:Mg = 0.52:0.34:0.14. A very minor Ti admixture is also determined to occur at the M(1) site of both minerals.
The largest M(3) octahedron with the mean M(3)–O distance of 2.136/2.14 Å in ferriperbøeite-(La)/perbøeite-(La), respectively, is predominately occupied by Fe2+ with subordinate Mg, Mn and Fe3+. According to electron microprobe data and refined numbers of electrons [e ref = 22.78 in ferriperbøeite-(La) and 22.47 in perbøeite-(La)], we fixed the ratio Fe2+:Mg:Fe3+:Mn = 0.46:0.22:0.17:0.15 in ferriperbøeite-(La) and Fe2+:Mg:Mn:Fe3+ = 0.53:0.24:0.16:0.07 in perbøeite-(La).
During the refinement, disorder was found for the O(15) site which deviates from the m plane just as it does in other members of the gatelite supergroup (see below). Bond-valence calculations (Table S4) confirm the above conclusions regarding cation distribution across different sites.
Acknowledgements
We are grateful to the Associate Editor Mike Rumsey for his thorough revision that substantially improved the manuscript. Henrik Friis and an anonymous reviewer, Structures Editor Peter Leverett and Principal Editor Stuart Mills are acknowledged for valuable comments. This study was supported by the Russian Foundation for Basic Research, grant no. 18-05-00332 (in part of XRD structure studies and crystal chemistry). This work was partly performed in accordance with the state task, state registration no. ААА-А19-119092390076-7 for N.V.C. The technical support by the SPbSU X-Ray Diffraction Resource Center in the powder XRD study is acknowledged.
Supplementary material
To view supplementary material for this article, please visit: https://doi.org/10.1180/mgm.2020.42.