Introduction
Food webs are models of trophic interactions among species, usually simplified into networks of species and energy links between them (Thompson et al., Reference Thompson, Brose, Dunne, Hall, Hladyz, Kitching, Martinez, Rantala, Romanuk, Stouffer and Tylianakis2012). The trophic dynamic theory supports the understanding of food web structure and species interactions that ultimately shape modern marine ecosystem ecology, conservation and management (Treblico et al., Reference Treblico, Baum, Salomon and Dulvy2013). Therefore, the analysis of diet to identify the role of each predator species on prey resource sharing relationships within the fish community is of great value (Svanbäck et al., Reference Svanbäck, Quevedo, Olsson and Eklöv2015).
The coexistence of ecologically similar fishes in high abundance in an ecosystem is possible because of the development of strategies that allow not only spatial and/or temporal separation of the species (Azevedo et al., Reference Azevedo, Araújo, Cruz-Filho, Gomes and Pessanha1999) but also ecological variations in the use of available niches and/or partitioning of the available resources (Herder & Freyhof, Reference Herder and Freyhof2006; Sandlund et al., Reference Sandlund, Museth, Næsje, Rognerud, Saksgard, Hesthagen and Borgstrøm2010). On the other hand, the functional or morphological homology of sympatric species can potentially increase competition (Wootton, Reference Wootton1990). If the food resources are limited, this competition can result in exclusion of the less adapted species. Nevertheless, according to the principles of competitive exclusion, interspecific competition also favours trophic niche diversification or resource partitioning across species (Schluter, Reference Schluter1996; Svanbäck & Bolnick, Reference Svanbäck, Bolnick, Jorgensen and Fath2008).
The isotopic niche concept proposed by Newsome et al. (Reference Newsome, Martínez del Rio, Bearhop and Phillips2007), a refinement of Hutchinson's idea (Hutchinson, Reference Hutchinson1957, Reference Hutchinson1978), suggests the use of isotopic tools to assess the ecological characteristics of organisms that ecologists aim to investigate, such as coexistence and resource sharing. Stable isotopic signatures of carbon (C) and nitrogen (N) have been widely used in this sense (Jackson et al., Reference Jackson, Inger, Parnell and Bearhop2011; Yasue et al., Reference Yasue, Doiuchi and Takasuka2014; Gallagher et al., Reference Gallagher, Shiffman, Byrnes, Hammerschlag-Peyer and Hammerschlag2017; Jensen et al., Reference Jensen, Kiljunen, Knudsen and Amundsen2017; Rader et al., Reference Rader, Newsome, Sabat, Chesser, Dillon and Rio2017), as the values measured in the consumer's tissues are closely related to the values from its diet. Stable nitrogen isotope ratios in consumers are typically enriched in the heavier isotope (15N) by 2–4‰ per trophic level (Minagawa & Wada, Reference Minagawa and Wada1984; Peterson & Fry, Reference Peterson and Fry1987), making δ15N values useful for the definition of trophic positions of consumers (Post, Reference Post2002). In contrast, the fractionation of carbon isotopes (13C) is lower (0–1‰) and is typically used to define energy sources (De Niro & Epstein, Reference De Niro and Epstein1978).
The determination of total mercury (THg) in organisms is a complementary tool for isotopic analysis that is widely used in trophic ecology investigations (Eagles-Smith et al., Reference Eagles-Smith, Suchanek, Colwell and Anderson2008; Fry & Chumchal, Reference Fry and Chumchal2012; Pouilly et al., Reference Pouilly, Rejas, Pérez, Duprey, Molina, Hubas and Guimarães2013). This tool has been used in ecological studies as a good indicator of the trophic level of organisms (Fry & Chumchal, Reference Fry and Chumchal2012), but it can also be used to identify spatiotemporal patterns of fish bioaccumulation (Buckman et al., Reference Buckman, Taylor, Broadley, Hocking, Balcom, Mason, Nislow and Chen2017; Liu et al., Reference Liu, Liu, Yuan, Liu and Lam2017) and biomagnification processes (Cresson et al., Reference Cresson, Bouchoucha, Miralles, Elleboode, Mahé, Marusczak, Thebault and Cossa2015; Chouvelon et al., Reference Chouvelon, Cresson, Bouchoucha, Brach-Papa, Bustamante, Crochet, Marco-Miralles, Thomas and Knoery2018). Tropical estuaries are known as source of anthropogenic Hg for fish feeding in coastal areas (Le Croizier et al., Reference Le Croizier, Schaal, Point, Le Loc'h, Machu, Fall, Munaron, Boyé, Walter, Laë and De Morais2019). In estuarine and coastal environments, it is estimated that 90% of the river-derived Hg is buried in sediments on ocean margins (Chester, Reference Chester and Chester1990), highlighting the contribution of estuaries to the transport of Hg to coastal marine waters and, consequently, to the associated food webs.
In Brazil, the Ariidae family is represented by demersal marine catfishes usually found in coastal and estuarine ecosystems (Marceniuk & Menezes, Reference Marceniuk and Menezes2007; Schmidt et al., Reference Schmidt, Martins, Reigada and Dias2008; Denadai et al., Reference Denadai, Bessa, Santos, Fernandez, Santos, Feijó, Arcuri and Turra2012; Pereyra et al., Reference Pereyra, Mont'Alverne and Garcia2016). Along the coast of São Paulo and Rio de Janeiro, their spatial distribution in estuarine systems is governed by salinity (Bizerril, Reference Bizerril1999; Denadai et al., Reference Denadai, Bessa, Santos, Fernandez, Santos, Feijó, Arcuri and Turra2012). They usually have wide trophic niches that, in general, overlap with each other's (Bruton, Reference Bruton1996; Denadai et al., Reference Denadai, Bessa, Santos, Fernandez, Santos, Feijó, Arcuri and Turra2012) as many other sympatric fish species (Svanbäck et al., Reference Svanbäck, Quevedo, Olsson and Eklöv2015; Cachera et al., Reference Cachera, Ernande, Villanueva and Lefebvre2017), and have been classified as generalist opportunistic omnivorous predators, with crustaceans, fishes, molluscs, annelids, detritus and algae commonly observed as the main groups of food items (Chaves & Vendel, Reference Chaves and Vendel1996; Denadai et al., Reference Denadai, Bessa, Santos, Fernandez, Santos, Feijó, Arcuri and Turra2012; Pinheiro-Sousa et al., Reference Pinheiro-Sousa, Silva, Pioski, Rocha, Carvalho-Neta and Almeida2015; Tavares & Di Beneditto, Reference Tavares and Di Beneditto2017; Di Beneditto et al., Reference Di Beneditto, Tavares and Monteiro2018; Di Beneditto & Tavares, Reference Di Beneditto and Tavares2019).
The amount of information generated by stomach assessments by scientists all over Brazil report the high importance of fishes and invertebrates (mainly crabs) as prey items of ariids (Chaves & Vendel, Reference Chaves and Vendel1996; Denadai et al., Reference Denadai, Bessa, Santos, Fernandez, Santos, Feijó, Arcuri and Turra2012; Tavares & Di Beneditto, Reference Tavares and Di Beneditto2017). To date, the trophic ecology of marine catfishes has been intensively studied over the last two decades (Chaves & Vendel, Reference Chaves and Vendel1996; Bizerril, Reference Bizerril1999; Denadai et al., Reference Denadai, Bessa, Santos, Fernandez, Santos, Feijó, Arcuri and Turra2012; Pinheiro-Sousa et al., Reference Pinheiro-Sousa, Silva, Pioski, Rocha, Carvalho-Neta and Almeida2015; Tavares & Di Beneditto, Reference Tavares and Di Beneditto2017; Di Beneditto et al., Reference Di Beneditto, Tavares and Monteiro2018; Di Beneditto & Tavares, Reference Di Beneditto and Tavares2019). However, few studies off the Brazilian coast have focused on isotopic tracers (Giarrizzo et al., Reference Giarrizzo, Schwamborn and Saint-Paul2011; Claudino et al., Reference Claudino, Pessanha, Araújo and Garcia2015; Pereyra et al., Reference Pereyra, Mont'Alverne and Garcia2016; Di Beneditto et al., Reference Di Beneditto, Tavares and Monteiro2018), and no data are available regarding stable isotopes coupled with Hg analyses in marine catfishes.
The present study used isotopic tracers of carbon (13C) and nitrogen (15N) coupled with mercury (Hg) to understand the trophic relationships among three marine catfishes Aspistor luniscutis (Valenciennes, 1840), Bagre bagre (Linnaeus, 1766) and Genidens genidens (Cuvier, 1829) in south-eastern Brazil, leading to an improvement in the knowledge of their trophic dynamics in Brazilian shallow waters. The questions to be answered are as follows: (1) Do the isotopic niches of these catfish species overlap? (2) Are there any spatial and/or temporal patterns in habitat usage among these sympatric marine catfishes? and (3) Do these catfish species compete for the same resource items?
Materials and methods
Study site and sampling
Samples were collected off Manguinhos Beach (21°29′S 41°01′W), District of São Francisco do Itabapoana, at ~10 m depth (Figure 1). This area is under the influence of the Itabapoana (north) and Paraíba do Sul (south) rivers. The shelf is naturally depleted of rock substratum or other hard substrates, and it is covered by extensive sandy beaches with variable amounts of mud and calcareous nodules, such as rhodolites (Zalmon et al., Reference Zalmon, Novelli, Gomes and Faria2002).

Fig. 1. Study area and sampling location (●) off Manguinhos Beach, northern Rio de Janeiro state, south-eastern Brazil.
The marine catfishes Aspistor luniscutis, Bagre bagre and Genidens genidens and their potential prey groups were collected every three months between April 2010 and January 2011. The captured ariid specimens were classified as adults from 180 mm total length for both sexes of A. luniscutis (Froese & Pauly, Reference Froese and Pauly2019), from 159 and 212 mm total length for females and males, respectively, of B. bagre (Véras & Almeida, Reference Véras and Almeida2016), and from 55 and 85 mm total length for females and males, respectively, of G. genidens (Mazzoni et al., Reference Mazzoni, Petito and Miranda2000).
Fishes were sampled at 13 randomized sampling points with bottom gillnets (n = 78), measuring 25 m in length and 3 m in height and mesh sizes of 20 mm (n = 18), 30 mm (n = 42) and 40 mm (n = 18), measured between adjacent nodes, submerged for 24 h.
Potential food resources identified based on previous stomach content studies of ariids (Chaves & Vendel, Reference Chaves and Vendel1996; Denadai et al., Reference Denadai, Bessa, Santos, Fernandez, Santos, Feijó, Arcuri and Turra2012; Tavares & Di Beneditto, Reference Tavares and Di Beneditto2017; Di Beneditto & Tavares, Reference Di Beneditto and Tavares2019) off the south-eastern Brazilian coast were also sampled in their natural habitat in the same sampling area. Corers (15 cm diameter, 20 cm height) were used to collect sediment and invertebrates. Phytoplankton and zooplankton were captured by surface trawl nets (30 cm mouth, 1.10 m length and of 20 and 70 μm mesh sizes, respectively) to sample local pelagic trophic end members.
All megafauna (fishes and invertebrates) collected were identified to the lowest taxonomic level, counted, measured (total length) and weighed (total body mass). Dorsal muscle tissues of fish, mantles of cephalopods and pieces of soft tissues (avoiding gastric tracts) of crustaceans as well as total organic matter in the sediment (SOM) and phytoplankton and zooplankton were dried and homogenized for stable isotope and THg analysis. All laboratory analyses were performed at the Laboratório de Ciências Ambientais from Universidade Estadual do Norte Fluminense Darcy Ribeiro.
Stable isotope analysis – δ13C and δ15N
All samples were stored in clean transparent plastic bags in iceboxes and then transported to the laboratory where they were kept frozen (−18°C) in dry sterile vials prior to analysis. Freeze-dried samples were ground with a mortar and pestle to a homogeneous fine powder. Approximately 0.5–1 mg of animal and phytoplankton tissues and 10 mg of sediment were used in the analysis.
For the elemental composition of carbon and nitrogen as well for δ13C and δ15N of sediment, ~10 mg was weighed in silver capsules, followed by acidification through the addition of HCl (2 M) to remove inorganic carbon (Kennedy et al., Reference Kennedy, Kennedy and Papadimitriou2005; Brodie et al., Reference Brodie, Leng, Casford, Kendrick, Lloyd, Yongqiang and Bird2011).
The elemental and isotopic composition of all samples were determined using a Flash 2000 Elemental Analyzer with a CONFLO IV interface coupled to a Delta V Advantage isotope ratio mass spectrometer (Thermo Scientific, Germany) in Laboratório de Ciências Ambientais. Samples were analysed using analytical blanks and urea analytical standards (IVA Analysentechnik-330802174; CH4N2O Mw = 60, C = 20%, N = 46%) using certified isotopic compositions (δ13C = −39.89‰ and δ15N = −0.73‰). For biota samples, analytical control was performed for every 10 samples using certified isotopic standards (Elemental Microanalysis Protein Standard OAS: 46.5 ± 0.78% for C; 13.32 ± 0.40% for N; −26.98 ± 0.13‰ for δ13C; + 5.94 ± 0.08‰ for δ15N). The accuracy of the sediment analysis verified using the Elemental Microanalysis Low Organic Soil Standard (1.52 ± 0.02% for C; 0.13 ± 0.02% for N; −27.46 ± 0.11‰ for δ13C; + 6.70 ± 0.15‰ for δ15N).
Carbon and nitrogen contents were expressed as per cent elements (%) and the detection limits were 0.05% and 0.02%, respectively. Carbon and nitrogen isotope ratios were expressed in δ notation as ‰ relative to Pee Dee Belemnite (PDB) and atmospheric nitrogen, respectively, and were calculated using the following equation:

where δ = δ13C or δ15N and R = δ13C:δ12C or δ15N:δ14N. Analytical reproducibility was based on triplicates for every 10 samples: ± 0.3‰ for δ15N and ± 0.2‰ for δ13C. There was no prior lipid extraction from the fish muscle samples, but the C/N ratios were lower than 3.5, indicating a low lipid level that did not compromise the carbon isotope results and their interpretation (Post et al., Reference Post, Layman, Arrington, Takimoto, Quattrochi and Montaña2007).
Total mercury analysis – THg
Dry muscle samples were digested according to Bastos et al. (Reference Bastos, Malm, Pfeiffer and Cleary1998). Briefly, the digestion of tissue samples was performed in a digestion block (60°C, 4 h) with a mixture of 3 ml HNO3:H2SO4 (1:1) and 1 ml of concentrated H2O2 and allowed to stand overnight. The addition of 5 ml of 5% KnMnO4 and subsequent 30 min of heating were followed by titration with hydroxylamine hydrochloride (NH3OHCl + NaCl 12%). THg was determined with a QuickTrace M-7500 CETAC (CV-AAS). The detection limit was 0.25 ng g−1. The analytical control of the method was determined and monitored using replicate analysis, blank solutions, and certified reference material from the National Research Council-Canada (DORM-2 dogfish Squalus acanthias muscle sample). The analytical coefficient of variation between replicates was less than 10%, and the recovery of THg was in agreement with certified values (higher than 90%). Our results are expressed in ng g−1 dry weight (dw).
Data treatment and analysis
The differences between species regarding total length and δ15N were assessed via one-way parametric ANOVA followed by Tukey's HSD post hoc test. For total mass, δ13C, C/N and THg, normality (Shapiro–Wilk test) and homoscedasticity (Levene test) were not achieved, and therefore non-parametric analysis of variance (Kruskal–Wallis) was applied followed by Dunn's post hoc test. Linear regressions were performed to check for possible relationships between variables (total length and δ15N, total mass and δ15N, total length and log10THg and total mass and log10THg). Correlation between δ15N and log10THg was also used with the organisms that compose the food web. Results were considered significant at P < 0.05.
Isotopic niche breadth was quantified for each marine catfish using the standard ellipse area corrected for small sample sizes (SEAc), calculated with the Stable Isotope Bayesian Ellipses tool in R (SIBER) (Jackson et al., Reference Jackson, Inger, Parnell and Bearhop2011). To evaluate the relative contribution of the different prey, Xiphopenaeus kroyeri (Heller, 1862), Callinectes sp., Doryteuthis sanpaulensis (Brakoniecki, 1984), Porichthys porosissimus (Cuvier, 1829) and Sciaenidae, that represent a pool of species composed by Isopisthus parvipinnis (Cuvier, 1830), Paralonchurus brasiliensis (Steindachner, 1875) and Stellifer rastrifer (Jordan, 1889), were used in the Bayesian stable isotope mixing model in the software package SIAR (Stable Isotope Analysis in R) (Parnell & Jackson, Reference Parnell and Jackson2013), which allows the inclusion of isotopic signatures and fractionation together with the uncertainty of these values within the model. To reduce the uncertainty in the interpretation of the mixing model, the potential prey were reduced/grouped to a total of five sources, similarly to the previous study of Di Beneditto et al. (Reference Di Beneditto, Tavares and Monteiro2018).
The trophic enrichment factors (TEFs) are key parameters in isotopic mixing models, representing the isotopic differences between consumers' tissues and their prey items after they reached equilibrium (Parnell et al., Reference Parnell, Inger, Bearhop and Jackson2010). In the absence of species-specific TEF values from controlled diet experiments, these values can be obtained in meta-analyses for phylogenetically related species, considering the same tissue (Newsome et al., Reference Newsome, Martínez del Rio, Bearhop and Phillips2007). In this sense, we calculated TEF15N and TEF13C based on equations from a meta-analysis of isotopic studies that considered muscle of fish species (Caut et al., Reference Caut, Angulo and Courchamp2009) (Δ15N = −0.281 δ15N + 5.879 and Δ13C = −0.248 δ13C−3.4770). The calculated values for our data were + 1.8 ± 0.2‰ for TEF15N and + 0.5 ± 0.2‰ for TEF13C, similar to those of marine catfishes of the same region (Di Beneditto et al., Reference Di Beneditto, Tavares and Monteiro2018).
The isotopic niche metrics that relate the characteristics of the isotopic space filled by each marine catfish species in this study were calculated and consisted of the following: NR (maximum δ15N range – larger range suggests more trophic levels and greater degree of trophic diversity); CR (maximum δ13C range – increased range suggests higher diversity of basal sources); TA (total area of isotopic niche – representing the total amount of niche space occupied); SEAc (corrected standard ellipses area for small sample size – isotopic niche width); CD (mean Euclidean distance from the centroid – higher distances suggests high average degree of trophic diversity within the food web); NND (mean Euclidean distance to the nearest neighbour – similar trophic ecology will exhibit small NND); and SDNND (standard deviation of NND – low values suggests more even distribution of trophic niche) (Layman et al., Reference Layman, Arrington, Montana and Post2007).
One-way ANOVAs were also used to evaluate differences and interactions between species considering CD and MNND, because they involve comparisons of means. The statistic SDNND, being a standard deviation, was compared between groups by an F-ratio test. The P values were interpreted as strengths of evidence towards null hypotheses rather than on the dichotomic scale of significance testing (Hurlbert & Lombardi, Reference Hurlbert and Lombardi2009).
All statistics and models were fitted using R version 3.5.2 (2018–12–20, ‘Eggshell Igloo’) for Linux in the RStudio software (Version 1.1.436) (R Core Team, 2018).
Results
The potential basal sources and prey groups are presented in Table 1. The lowest values of δ13C and δ15N were observed for particulate organic matter in sediment (SOM) and phytoplankton, respectively, while the highest values were found for Sciaenidae and Doryteuthis sanpaulensis, respectively. THg concentrations were lowest for phytoplankton and highest for Callinectes sp.
Table 1. Potential basal sources and prey group size range (mm), mean ± standard deviation (SD) of δ13C (‰), δ15N (‰) and THg (ng g−1 dw) sampled in northern Rio de Janeiro state, south-eastern Brazil

a For fish species, the size range is standard length; for X. kroyeri, the size range is total length; for Callinectes sp., the size range is carapace width.
b Composite sample.
c One single specimen.
Overall, 65 muscle samples were analysed for δ13C, δ15N and THg representing the three ariid species in the present study. For Aspistor luniscutis, Bagre bagre and Genidens genidens similar δ13C values (Kruskal–Wallis, χ2 = 4.545, P > 0.05) and C/N ratios (Kruskal–Wallis, χ2 = 4.029, P > 0.05) were observed (Table 2). The species A. luniscutis is the smallest (total length – ANOVA, F = 26.962, P < 0.001) and lightest (mass – Kruskal–Wallis, χ2 = 17.610, P < 0.001) catfish. Nitrogen isotope signatures (δ15N) were similar for A. luniscutis and B. bagre, while a lower value (δ15N – ANOVA, F = 18.089, P < 0.001) was found for G. genidens. Total mercury (THg) was significantly different (Kruskal–Wallis, χ 2 = 21.892, P < 0.001) for all three species, with the highest concentrations in G. genidens and lowest concentrations in B. bagre.
Table 2. Number of individuals (n), mean ± standard deviation (SD) of total length (mm), total mass (kg), δ13C (‰), δ15N (‰), C/N (%) and THg (ng g−1 dw) of the marine catfishes Aspistor luniscutis, Bagre bagre and Genidens genidens in northern Rio de Janeiro state, south-eastern Brazil

Letters indicate significant differences (P < 0.05).
The δ13C and δ15N values of the invertebrates Doryteuthis sanpaulensis, Callinectes sp., Xiphopenaeus kroyeri and of the bony fishes Porichthys porosissimus and Sciaenidae, all prey items for marine catfishes, ranged from −18.00 ± 0.80‰ for P. porosissimus to −15.77 ± 1.44‰ for Sciaenidae and 10.60 ± 0.60‰ for P. porosissimus to 13.25 ± 0.55‰ for D. sanpaulensis, respectively. The δ13C of organic matter (OM) basal sources (SOM and phytoplankton) and zooplankton ranged from −20.67 ± 0.93‰ for SOM to −17.68 ± 0.75‰ for zooplankton while δ15N values ranged from 7.05‰ for phytoplankton to 9.03 ± 2.66‰ for SOM (Figure 2).

Fig. 2. Relationship between δ13C and δ15N considering Aspistor luniscutis (Al), Bagre bagre (Bb), Genidens genidens (Gg), Doryteuthis sanpaulensis (Ds), Xiphopenaeus kroyeri (Xk), Callinectes sp. (Csp), Sciaenidae (Sc), Porichthys porosissimus (Pp), zooplankton (Zoo), phytoplankton (phyto) and organic matter in sediment (SOM) in northern Rio de Janeiro state, south-eastern Brazil. The range of isotopic ratios measured for the potential SOM and phytoplankton (through zooplankton) sources is represented by small hatched squares. Hatched zones represent the zone influenced by the OM sources.
The δ15N was significantly correlated with total length and total mass only for B. bagre (Figure 3A, B). THg also showed correlations, but with total length, for both B. bagre and G. genidens, and with total mass, for B. bagre only (Figure 3C, D). No correlations between variables were observed for A. luniscutis.

Fig. 3. Relationships between: (A) δ15N (‰) and total length (mm), (B) δ15N (‰) and total mass (kg), (C) log-transformed total mercury (THg) and total length (mm) and (D) log-transformed total mercury (THg) and total mass (kg) of the three marine catfishes Aspistor luniscutis (Al), Bagre bagre (Bb) and Genidens genidens (Gg) in northern Rio de Janeiro state, south-eastern Brazil. The results from the linear models (lines + 95% confidence interval grey polygons) are plotted on observed log-transformed data. The r and P-values of the Pearson correlation as well as the equations of the lines (derived from linear model output) are indicated. Bold values indicate significant (P < 0.05) relationships.
The relationship between δ15N and THg was highly significant. The slope was 0.34, indicating biomagnification of Hg over the increase in δ15N in this marine food web (Figure 4).
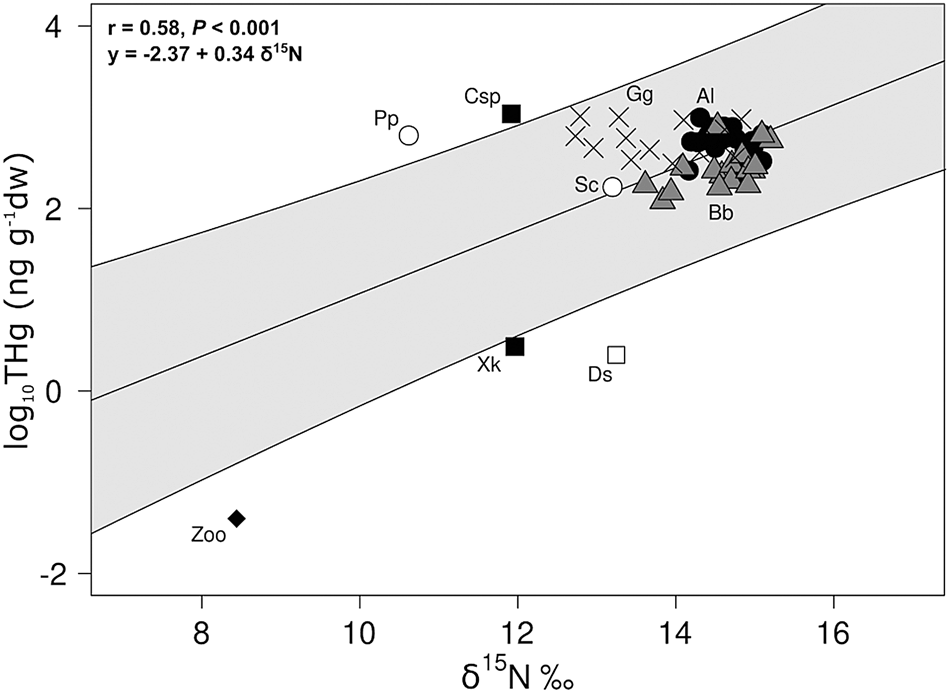
Fig. 4. Relationships between δ15N (‰) and log-transformed THg considering Aspistor luniscutis (Al), Bagre bagre (Bb), Genidens genidens (Gg), Doryteuthis sanpaulensis (Ds), Xiphopenaeus kroyeri (Xk), Callinectes sp. (Csp), Sciaenidae (Sc), Porichthys porosissimus (Pp) and zooplankton (Zoo) in northern Rio de Janeiro state, south-eastern Brazil. The results from the linear model (lines + 95% confidence interval) are plotted on observed log-transformed data. The r and P-values of the Pearson correlation as well as the equation of the line (derived from linear model output) are indicated. Bold values indicate significant (P < 0.05) relationships.
The isotopic niche area represented by SEAc revealed a broader trophic niche for G. genidens (1.88‰2) than for A. luniscutis (0.55‰2) and B. bagre (0.47‰2) (Figure 5). The greatest isotopic niche overlap was observed for the ellipses of B. bagre on A. luniscutis, followed by A. luniscutis overlapping the ellipse of B. bagre, covering an area of 0.29‰2. The lowest SIBER ellipse overlaps were observed for G. genidens on A. luniscutis and B. bagre, with overlapping areas of 0.21 and 0.15‰2, respectively (Figure 5, Table 3).

Fig. 5. The standard ellipse areas corrected for small sample size (SEAc) for the three marine catfishes Aspistor luniscutis, Bagre bagre and Genidens genidens in northern Rio de Janeiro state, south-eastern Brazil.
Table 3. Overlapping SEAc (%) between the three marine catfishes Aspistor luniscutis, Bagre bagre and Genidens genidens in northern Rio de Janeiro state, south-eastern Brazil

Table 4 presents the quantitative metrics to estimate the isotopic niche breadth for the three marine catfishes. The highest values of food web length (NR), variability of food resources (CR), total occupied niche area (TA) and small sample size corrected standard ellipse area (SEAc) were observed for G. genidens. Additionally, trophic diversity within the demersal food web (CD – ANOVA, F = 7.515, P = 0.001), trophic redundancy (MNND – ANOVA, F = 6.842, P = 0.001) and evenness (SDNND – F-ratio test, P < 0.001) were higher for G. genidens than for A. luniscutis and B. bagre. Similar to G. genidens, the species A. luniscutis also presented elevated variability of food resources compared with B. bagre.
Table 4. Isotopic niche metrics of the marine catfishes Aspistor luniscutis, Bagre bagre and Genidens genidens in northern Rio de Janeiro state, south-eastern Brazil

Letters indicate significant differences (P < 0.05).
The SIAR analysis revealed similar relative contributions of all prey items in A. luniscutis and B. bagre, highlighting higher assimilations of Sciaenidae fishes and D. sanpaulensis (73 and 70%, respectively) compared with G. genidens, which presented no preference for any prey (Figure 6).

Fig. 6. Results of SIAR (Stable Isotope Analysis in R) showing 95% (dark grey), 75% (intermediate grey) and 25% (light grey) credibility intervals of prey items contributions to the diet of the catfishes Aspistor luniscutis, Bagre bagre and Genidens genidens. Xk, Xiphopenaeus kroyeri; Csp, Callinectes sp.; Ds, Doryteuthis sanpaulensis; Pp, Porichthys porosissimus; and Sc, Sciaenidae. Numbers above credibility intervals are the percentage of contribution of each prey to the respective predator.
Discussion
This study revealed the extent of sharing and segregating food resources of the three sympatric marine catfish species Aspistor luniscutis, Bagre bagre and Genidens genidens based on comparisons of food assimilation using SIAR, SIBER, isotopic niche breadth metrics and THg concentrations. Diet overlaps observed between the three marine catfish species were explained by proportions in the assimilation of Sciaenidae fishes (Isopisthus parvipinnis, Paralonchurus brasiliensis and Stellifer rastrifer), Doryteuthis sanpaulensis, Xiphopenaeus kroyeri and Callinectes sp.
The present study corroborated using a stable isotope approach to classify marine catfish trophic guilds as omnivorous, as previously determined by stomach contents and stable isotope studies (Chaves & Vendel, Reference Chaves and Vendel1996; Denadai et al., Reference Denadai, Bessa, Santos, Fernandez, Santos, Feijó, Arcuri and Turra2012; Tavares & Di Beneditto, Reference Tavares and Di Beneditto2017; Di Beneditto et al., Reference Di Beneditto, Tavares and Monteiro2018; Di Beneditto & Tavares, Reference Di Beneditto and Tavares2019). In south-eastern Brazil, Denadai et al. (Reference Denadai, Bessa, Santos, Fernandez, Santos, Feijó, Arcuri and Turra2012) analysed stomach contents and intestine remains in juveniles, sub-adults and adults, concluding that marine catfishes have wide trophic plasticity during their lifetimes. Juveniles feed mainly on soft and small food items, such as algae and molluscs; adults forage on harder and larger items, such as crustaceans, molluscs and fishes; and sub-adults feed on both prey types.
The SIBER analysis revealed overlaps between the isotopic niches of A. luniscutis, B. bagre and G. genidens. The greater overlaps (>53%) between the isotopic niches of A. luniscutis and B. bagre indicate that these two species rely on similar prey proportions of assimilation, specifically on Sciaenidae and D. sanpaulensis, as observed by SIAR analysis. The species A. luniscutis and B. bagre presented lower trophic diversity within the demersal food web (CD) and higher trophic redundancy (MNND) and evenness (SDNND) when compared with G. genidens, reinforcing its more restricted assimilation of prey resources. Based on isotopic niche metrics, G. genidens showed the broadest niche breadth among the catfishes, as evidenced by the highest isotopic niche metrics observed (NR, CR, TA, SEAc, CD, MNND, SDNND). Similar to what other authors found through stomach content analysis (Denadai et al., Reference Denadai, Bessa, Santos, Fernandez, Santos, Feijó, Arcuri and Turra2012), the isotopic data suggest that diet of G. genidens is the most diversified, based on the higher proportion of assimilation of Porichthys porosissimus and Callinectes sp. in the Paraíba do Sul river (PSR) estuary compared with A. luniscutis and B. bagre.
The higher trophic plasticity of G. genidens and the similar isotopic niches of A. luniscutis and B. bagre may be a result of three situations, individually or combined: (1) the high abundance of shrimps, crabs and neritic squids in the study area; (2) different spatial habitat usage by catfishes in the estuarine system; and (3) foraging of other prey items to avoid competition.
Situation 1 can be sustained, as lolignid squids (Doryteuthis plei and D. sanpaulensis) have a strong association with the sea bottom and are considered abundant in the inner shelf from 10 to 50 m (Robin et al., Reference Robin, Roberts, Zeidberg, Bloor, Rodriguez, Briceño, Downey, Mascaró, Navarro, Guerra, Hofmeister, Barcellos, Lourenço, Roper, Moltschaniwskyj, Green, Mather and Vidal2014; Costa et al., Reference Costa, Mincarone, Braga, Martins, Lavrado, Haimovici and Falcão2015). A short distance south (100 km), in the Cabo Frio upwelling region, Soares et al. (Reference Soares, Muto, Lopez, Clauzet and Valiela2014) found similar δ15N values (higher than for other invertebrates of the present study) of loliginid species (D. plei – 12.5‰ and D. sanpaulensis – 11.0‰) compared with our results (13.3‰). Shrimps (Xiphopenaeus kroyeri) and crabs (Callinectes sp.) are considered to be as abundant as squids on the northern coast off Rio de Janeiro (Di Beneditto et al., Reference Di Beneditto, Souza, Tudesco and Klôh2010; Santos & Menegon, Reference Santos and Menegon2010; Fernandes et al., Reference Fernandes, Keunecke and Di Beneditto2014). Thus, the local abundance of prey is high to consumers. Nevertheless, the mean δ15N values of decapods (Callinectes sp. and X. kroyeri) and D. sanpaulensis are higher than that of P. porosissimus (Table 2), reflecting the nitrogen isotope values of the consumers. In this sense, it is noteworthy that the δ15N values of the catfishes represented differences in their prey isotopic values rather than in their trophic levels.
Denadai et al. (Reference Denadai, Bessa, Santos, Fernandez, Santos, Feijó, Arcuri and Turra2012) observed a slight overlap between A. luniscutis and G. genidens because of crustacean fragments and fish scales in both diets and associated the small overlap (37.6%) with differences in spatial usage of the estuary. However, situation 2 may not be plausible. Pereyra et al. (Reference Pereyra, Mont'Alverne and Garcia2016) found that G. genidens adults consumed freshwater-derived carbon sources, and Bizerril (Reference Bizerril1999) also observed spatial differences governed by salinity in the habitat usage of marine catfishes, allowing their coexistence. The stable isotopes of the present study revealed no variation in habitat usage. The δ13C results were similar among the three ariids, suggesting not only the absence of distinctions in basal OM assimilated sources but also similarity in habitat usage (Bouillon et al., Reference Bouillon, Connolly, Gillikin, Wolanski and McLusky2011). Nevertheless, our δ13C values are in accordance with the previous study of Di Beneditto et al. (Reference Di Beneditto, Tavares and Monteiro2018), reinforcing that marine catfishes are characteristically widespread in the coastal waters influenced by the PSR.
Although the focus of the present study was on trophic ecology rather than habitat usage variations, the THg concentrations observed in the present study coupled with previous knowledge of ariids suggesting different spatial usage in estuaries (Azevedo et al., Reference Azevedo, Araújo, Cruz-Filho, Gomes and Pessanha1999; Bizerril, Reference Bizerril1999; Schmidt et al., Reference Schmidt, Martins, Reigada and Dias2008; Denadai et al., Reference Denadai, Bessa, Santos, Fernandez, Santos, Feijó, Arcuri and Turra2012), can lead to misunderstandings about their spatial dynamics in the estuarine habitat. Regarding THg, a decrease in its concentration in fishes along the river–ocean gradient is expected (Liu et al., Reference Liu, Liu, Yuan, Liu and Lam2017). As adult catfishes usually live in adjacent coastal waters influenced by estuaries (Mishima & Tanji, Reference Mishima and Tanji1983; Denadai et al., Reference Denadai, Bessa, Santos, Fernandez, Santos, Feijó, Arcuri and Turra2012) and only seek riverine waters during the spawning period (Schmidt et al., Reference Schmidt, Martins, Reigada and Dias2008), the differences in THg concentrations between marine catfishes observed in the present study seem to be related to biological factors, not to spatial segregation.
Previous studies developed along this area support the Hg assimilation related to predator–prey interactions (Carvalho et al., Reference Carvalho, Di Beneditto, Souza, Ramos and Rezende2008; Kehrig et al., Reference Kehrig, Fernandes, Malm, Seixas, Di Beneditto and Souza2009; Di Beneditto et al., Reference Di Beneditto, Bittar, Camargo, Rezende and Kehrig2012), suggesting that the past practices of gold-mining and the use of mercurial fungicides on sugarcane plantations in the PSR drainage basin are the main input sources of Hg in this fluvial ecosystem, which is largely exported to the adjacent marine areas, coupled with Hg atmospheric deposition (Lacerda et al., Reference Lacerda, Carvalho, Rezende and Pfeiffer1993; Lacerda, Reference Lacerda1996; Araujo et al., Reference Araujo, Hintelmann, Dimock, Almeida and Rezende2017; Azevedo et al., Reference Azevedo, Pestana, Rocha, Meneguelli-Souza, Lima, Almeida, Bastos and Souza2018). Reinfelder et al. (Reference Reinfelder, Fisher, Luoma, Nichols and Wang1998) indicated that the assimilation of dissolved Hg in the water column is an important route for bioaccumulation by aquatic organisms with small body sizes and greater surface areas. The input of Hg in food webs through phytoplankton and the successive increase in metal concentrations to the next trophic levels (Pickhardt et al., Reference Pickhardt, Folt, Chen, Klaue and Blum2002) were already observed in coastal areas influenced by PSR in other fish species (Di Beneditto et al., Reference Di Beneditto, Bittar, Camargo, Rezende and Kehrig2012; Kehrig et al., Reference Kehrig, Seixas, Malm, Di Beneditto and Rezende2013).
The reasons for the elevated mean THg values observed in larger ariid individuals seem to be three-fold: first, it is related to the bioaccumulation of THg during the catfish lifespan, evidenced by the significant correlations (P < 0.05) between THg and total length and mass. The accumulation might occur in ariids in the adjacent marine waters of the PSR estuary in accordance with the findings of other authors that suggest that larger and/or older fish have higher THg concentrations due to longer exposure and the difficulty of eliminating mercury, as THg has a very slow excretion rate due to the metal's high affinity for thiol groups that constitute the protein fraction of the muscles (Nakao et al., Reference Nakao, Seoka, Tsukamasa, Kawasaki and Ando2007). Second, the elevated assimilation of species closely related to the bottom sediment, that provides higher Hg bioavailability than the water column (Muto et al., Reference Muto, Soares, Sarkis, Hortellani, Petti and Corbisier2014; Le Croizier et al., Reference Le Croizier, Schaal, Point, Le Loc'h, Machu, Fall, Munaron, Boyé, Walter, Laë and De Morais2019), by G. genidens (Callinectes sp. – highest THg in prey and P. porosissimus – highest THg in prey fishes) and A. luniscutis (Sciaenidae fish) resulted in the higher THg than in B. bagre. Finally, the seasonality of the PSR discharge (input of Hg to the ocean) may also be an influencing factor. As observed by Rocha et al. (Reference Rocha, Franco, Gatts and Zalmon2015), at times of higher PSR discharge, G. genidens and A. luniscutis were more abundant, thus contributing to higher mean THg values for both species compared with B. bagre. At times of lower PSR discharge, B. bagre is more abundant; thus as suggested by authors worldwide and for the PSR estuary, during low river discharge the main source of Hg is the direct deposition of the contaminant from the atmosphere to surface waters, resulting in higher concentrations of THg in fish due to the rapid methylation and absorption by biota, thus becoming more bioavailable (Harris et al., Reference Harris, Rudd, Amyot, Babiarz, Beaty, Blanchfield, Bodaly, Branfireun, Gilmour, Graydon, Heyes, Hintelmann, Hurley, Kelly, Krabbenhoft, Lindberg, Mason, Paterson, Podemski, Robinson, Sandilands, Southworth, Louis and Tate2007; Mason et al., Reference Mason, Choi, Fitzgerald, Hammerschmidt, Lambrog, Soerensen and Sunderland EM2012; Araujo et al., Reference Araujo, Hintelmann, Dimock, Almeida and Rezende2017; Azevedo et al., Reference Azevedo, Pestana, Rocha, Meneguelli-Souza, Lima, Almeida, Bastos and Souza2018).
Biomagnification was also indicated by the significant correlations of THg and δ15N. Since diet is the main source of THg assimilation in fishes (Hall et al., Reference Hall, Bodaly, Fudge, Rudd and Rosenberg1997), it might be occurring in this food web, as already observed for other fish species influenced by the PSR plume in the region (Bisi et al., Reference Bisi, Lepoint, Azevedo, Dorneles, Flach, Das, Malm and Lailson-Brito2012; Di Beneditto et al., Reference Di Beneditto, Bittar, Camargo, Rezende and Kehrig2012; Kehrig et al., Reference Kehrig, Seixas, Malm, Di Beneditto and Rezende2013) and worldwide (Al-Reasi et al., Reference Al-Reasi, Ababneh and Lean2007; Liu et al., Reference Liu, Liu, Yuan, Liu and Lam2017; Chouvelon et al., Reference Chouvelon, Cresson, Bouchoucha, Brach-Papa, Bustamante, Crochet, Marco-Miralles, Thomas and Knoery2018). In the present study, the slope of the linear regression between THg and δ15N (0.34), used as measurement of biomagnification across food webs (Kidd et al., Reference Kidd, Hesslein, Fudge and Hallard1995), was higher than those observed by Al-Reasi et al. (Reference Al-Reasi, Ababneh and Lean2007) in Gulf of Oman (0.07), Muto et al. (Reference Muto, Soares, Sarkis, Hortellani, Petti and Corbisier2014) in the Santos continental shelf (0.13), and Di Beneditto et al. (Reference Di Beneditto, Bittar, Camargo, Rezende and Kehrig2012) (0.25) and Kehrig et al. (Reference Kehrig, Seixas, Malm, Di Beneditto and Rezende2013) (0.21) in the same region as our study. Although biomagnification studies in tropical marine ecosystems are limited, this range (0.07–0.34), already observed by Lavoie et al. (Reference Lavoie, Jardine, Chumchal, Kidd and Campbell2013) in tropical marine food webs, reflects the different composition and vertical position in the water column (higher Hg bioavailability in the bottom sediment for benthic/demersal organisms) of the tropical food webs and/or differences in the growth rate of organisms (Muto et al., Reference Muto, Soares, Sarkis, Hortellani, Petti and Corbisier2014; Le Croizier et al., Reference Le Croizier, Schaal, Point, Le Loc'h, Machu, Fall, Munaron, Boyé, Walter, Laë and De Morais2019). It is already known that the biomagnification of Hg can vary greatly between marine ecosystems (Cossa et al., Reference Cossa, Harmelin-Vivien, Mellon-Duval, Loizeau, Averty, Crochet, Chou and Cadiou2012), or even between environments of the same ecosystem (Cresson et al., Reference Cresson, Bouchoucha, Miralles, Elleboode, Mahé, Marusczak, Thebault and Cossa2015).
Competitive exclusion (situation 3) seems not to regulate the preference of Sciaenidae fishes and D. sanpaulensis by A. luniscutis and B. bagre. As mentioned above, Rocha et al. (Reference Rocha, Franco, Gatts and Zalmon2015) observed A. luniscutis and G. genidens in the region mainly during the rainy season (higher discharge of PSR – October to April), while B. bagre was observed during the dry season (lower discharge of PSR – May to September). In this sense, the elevated level of partitioning of resources between A. luniscutis and B. bagre, reflected by high isotopic niche overlaps, seems to exclude none of the species. Thus, based on the high abundance of prey coupled with the different seasonal abundances in the region of the catfishes, it seems they are not competing for the same resources, at the same time, in the same place, leading us to conclude that there is low evidence of interspecific resource competition.
In conclusion, we found that G. genidens has a broader trophic niche breadth, is considered generalist and is the most opportunistic marine catfish, due to assimilation of organisms in similar proportions and with lower δ15N. The prey preference for Sciaenidae fishes and D. sanpaulensis was observed for A. luniscutis and B. bagre, resulting in the highest isotopic niche overlap and δ15N signatures, answering the first question of the present study. The coexistence of these three sympatric marine catfishes is probably regulated by different temporal patterns in the coastal area influenced by the PSR plume (question 2) and the high abundance of prey items, leading to resource partitioning with no evidence of competition among the species (question 3). The THg highlighted the different proportions of assimilation of prey as well as the seasonality in the habitat usage by the species. Bioaccumulation of Hg was observed for B. bagre and G. genidens and Hg biomagnification also occurred in their food web. The understanding of the trophic dynamics of sympatric fishes enhances the knowledge of ecological and environmental forces driving their coexistence. More studies incorporating multiple tools are important for the advancement of knowledge of the trophic dynamics, life cycles, habitat usage and environmental role of marine catfishes in the continent–ocean interface.
Acknowledgements
We are grateful to DSc. Bruno P. Masi and MSc. Thiago P. Rangel for diving and geoprocessing, respectively. The authors thank the American Journal Experts (AJE) for the English revision of the manuscript. Finally, we would like to express our thanks to Dr Vlad Laptikhovsky and the anonymous reviewers for their comments and suggestions on the document.
Financial support
This research was supported by Fundação de Amparo à Pesquisa do Rio de Janeiro – FAPERJ (Rezende CE, grant number E-26/110.437/2010) and by Conselho Nacional de Desenvolvimento Científico e Tecnológico – CNPq (INCT Material Transfer at the Continent-Ocean Interface, Rezende CER, grant number 573.601/08-9; Zalmon IR, grant number 470997/2010-9). Likewise, this research study was financed in part by the Coordenação de Aperfeiçoamento de Pessoal de Nível Superior – Brasil (CAPES) – Finance Code 001.