Introduction
Medusahead [Taeniatherum caput-medusae (L.) Nevski] is one of the most detrimental invasive plants impacting rangeland sustainability and livestock operations (Johnson and Davies Reference Johnson and Davies2012; Mangla et al. Reference Mangla, Sheley and James2011; Nafus and Davies Reference Nafus and Davies2014); it decreases wildlife habitat and plant diversity and increases the frequency of fires (Davies Reference Davies2011). Recently, the integration of multiple control strategies has reestablished desirable plant species (Davies and Boyd Reference Davies and Boyd2018; Schantz et al. Reference Schantz, Sheley and Hardegree2019), but the cost and uncertainty of success still exists for land managers (James et al. Reference James, Gornish, DiTomaso, Davy, Doran, Becchetti, Lile, Brownsey and Laca2015). Consequently, there is a critical need to assess the underlying causes of T. caput-medusae invasion and how these causes impact traditional control strategies and to develop management strategies that decrease success uncertainty. These challenging questions can be partially addressed through a conceptual model that considers how tissue silicon (Si) concentration influences T. caput-medusae plant characteristics and the invasive processes.
High tissue Si concentration is a vital attribute of T. caput-medusae, as it forms mineral silica complexes within stems and an epidermal silica varnish on the leaves, awns, and glumes (Bovey et al. Reference Bovey, Tourneau and Erickson1961; Epstein Reference Epstein1999; Swenson et al. Reference Swenson, Le Tourneau and Erickson1964). The varnish hinders digestive processes within the rumen of herbivores (Montes-Sánchez and Villalba Reference Montes-Sánchez and Villalba2017) and contributes to a defense mechanism that facilitates aversive grazing behavior (Coskun et al. Reference Coskun, Deshmukh, Sonah, Menzies, Reynolds, Ma, Kronzucker and Bélanger2019; Hunt et al. Reference Hunt, Dean, Webster, Johnson and Ennos2008; McNaughton et al. Reference McNaughton, Tarrants, McNaughton and Davis1985). The same varnish also limits leaf litter decomposition, causing a persistent litter or thatch layer (Torell et al. Reference Torell, Erickson and Haas1961; Young Reference Young1992). Accumulated litter inhibits establishment of non–T. caput-medusae seedlings and increases fine fuels, facilitating a grass-fire positive feedback cycle (Davies and Nafus Reference Davies and Nafus2013; Kyser et al. Reference Kyser, DiTomaso, Doran, Orloff, Wilson, Lancaster, Lile and Porath2007; Young et al. Reference Young, Clements and Nader1999). Finally, high tissue Si concentrations may increase T. caput-medusae productivity directly through enhanced plant fitness and indirectly through accumulated litter, which may improve T. caput-medusae germination and root development (Evans and Young Reference Evans and Young1970). Consequently, T. caput-medusae Si concentrations are likely linked to increasing both the relative and overall abundance of the weed in plant communities, decreasing native species diversity, and reducing net primary productivity, and contribute to the creation of positive feedbacks that favor persistence of exotic species at the expense of native species (Elgersma et al. Reference Elgersma, Yu, Vor and Ehrenfeld2012; Inderjit and Cahill Reference Inderjit and Cahill2015; Suding et al. Reference Suding, Stanley Harpole, Fukami, Kulmatiski, MacDougall, Stein and van der Putten2013).
The conceptual model we propose portrays linkages between tissue Si concentrations and a self-reinforcing positive feedback cycle of T. caput-medusae invasion. These linkages may enhance a more mechanistic understanding of the invasive processes and potentially refine T. caput-medusae control and restoration effort opportunities (Figure 1). This model illustrates how tissue Si concentrations directly influence critical plant characteristics (e.g., fitness, structure, and chemical composition), which subsequently impact fundamental invasive processes (e.g., plant productivity, litter decomposition, and herbivory). However, despite the connectivity between plant characteristics and invasive processes associated with T. caput-medusae abundance, close examination of the current knowledge base suggests that considerable uncertainty exists in how control strategies impact tissue Si concentrations.
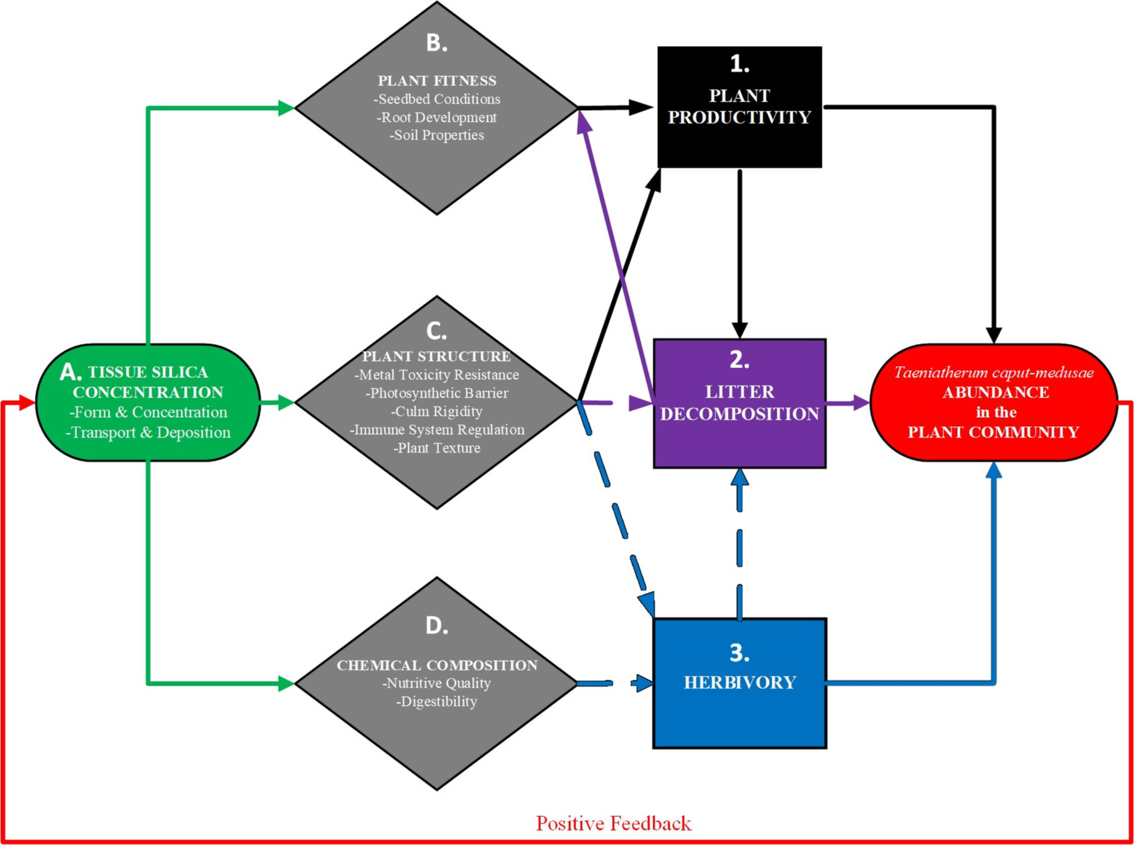
Figure 1. A model explaining the self-reinforcing positive cycle feedback of Taeniatherum caput-medusae invasion in relation to silicon (Si). Rhomboids represent plant characteristics; rectangles represent invasion processes; solid lines represent net positive effects and dashed lines net negative effects.
Several categories of T. caput-medusae control (i.e., mechanical, cultural, and chemical) have been applied successfully to reduce and/or suppress the plant’s abundance (Davies Reference Davies2010; Kyser et al. Reference Kyser, DiTomaso, Doran, Orloff, Wilson, Lancaster, Lile and Porath2007; Monaco et al. Reference Monaco, Osmond and Dewey2005; Stonecipher et al. Reference Stonecipher, Panter and Villalba2016). However, tissue Si concentration is an underlying cause of invasion that is often overlooked, because Si is not considered an essential element of plant nutrition (Arnon and Stout Reference Arnon and Stout1939; Epstein Reference Epstein1999). Thus, the focus of this article will be to summarize the role of tissue Si in plants, identify T. caput-medusae’s characteristics and its invasive processes in relation to tissue Si using a conceptual model (Figure 1), address how control strategies address T. caput-medusae Si, and finally, suggest research avenues that may aid in novel or improved control strategies that target T. caput-medusae–silica relationships.
Silicon and Plants
Form and Concentration
Si is the second most abundant element found within the earth’s crust (Epstein Reference Epstein1999), and the majority of plant Si exists as amorphous Si (i.e., phytoliths), which is insoluble in water. In contrast, its soluble form, orthosilicic acid, or simply silicic acid (Ma and Yamaji Reference Ma and Yamaji2006), is the form taken up by plant roots and deposited as the insoluble form, silica (Currie and Perry Reference Currie and Perry2007; Epstein Reference Epstein1994, Reference Epstein1999). Often the literature does not distinguish between the two forms of Si, particularly with T. caput-medusae. Thus, unless soluble Si is directly stated herein, it is referring to the insoluble form, silica. Tissue silica varies widely among plant species (Hodson et al. Reference Hodson, White, Mead and Broadley2005), and plants are generally classified as accumulators (1% to 10% silica on a dry matter basis) or nonaccumulators (<1%). Some monocots such as rice (Oryza sativa L.), sugarcane (Saccharum officinarum L.), and members of the Cyperaceae family (i.e., sedges) are considered hyperaccumulators, having greater than 10% silica in their tissues (Handreck and Jones Reference Handreck and Jones1967; Ma and Takahashi Reference Ma and Takahashi2002; Neumann Reference Neumann and Müller2003). Consequently, many grasses are considered accumulators, but tissue silica varies widely depending on geographic location, phenological stage, and environmental conditions (McNaughton et al. Reference McNaughton, Tarrants, McNaughton and Davis1985). For instance, tissue silica ranges between 1% and 4% in alpine tundra grasses, whereas African savanna grasses contain up to 20% (Johnston et al. Reference Johnston, Bezeau and Smoliak1968; McNaughton et al. Reference McNaughton, Tarrants, McNaughton and Davis1985). Semiarid rangeland grasses are considered intermediate accumulators, with tissue silica ranging between 3% and 9% (Shewmaker et al. Reference Shewmaker, Mayland, Rosenau and Asay1989), but T. caput-medusae stands out as a hyperaccumulator, with values ranging between 10% and 19% (Bovey et al. Reference Bovey, Tourneau and Erickson1961; Epstein Reference Epstein1999; Swenson et al. Reference Swenson, Le Tourneau and Erickson1964).
Transport and Deposition
The uptake of soluble Si varies based on species, genotype, and root structure, and its transport occurs via the transpiration stream (Ma and Yamaji Reference Ma and Yamaji2006). Transport can be active, passive, and/or rejective (Ma et al. Reference Ma, Mitani, Nagao, Konishi, Tamai, Iwashita and Yano2004; Mitani and Ma Reference Mitani and Ma2005; Takahashi et al. Reference Takahashi, Ma and Miyake1990). For example, accumulation in grasses is believed to be a passive process, wherein these plants often display tissue silica concentrations reflective of soluble soil Si concentrations (Handreck and Jones Reference Handreck and Jones1967; Ma et al. Reference Ma, Miyake, Takahashi, Datnoff, Snyder and Korndörfer2001). In contrast, some dicots exhibit a rejective transport mechanism associated with a physical barrier in roots, allowing water and nutrient passage but limiting soluble Si uptake (Jones and Handreck Reference Jones and Handreck1969). Hyperaccumulators such as rice (Feng et al. Reference Feng, Yamaji and Mitani-Ueno2011; Ma et al. Reference Ma, Yamaji, Mitani, Tamai, Konishi, Fujiwara, Katsuhara and Yano2007), barley (Hordeum vulgare L.) (Chiba et al. Reference Chiba, Mitani, Yamaji and Ma2009), maize (Zea mays L.) (Mitani et al. Reference Mitani, Yamaji and Ma2008), wheat (Triticum aestivum L.) (Casey et al. Reference Casey, Kinrade, Knight, Rains and Epstein2004; Rains et al. Reference Rains, Epstein, Zasoski and Aslam2006), and cucumber (Cucumis sativus L.) (Liang et al. Reference Liang, Si and Römheld2005) display an active transport mechanism that facilitates soluble Si uptake and deposition (Chiba et al. Reference Chiba, Mitani, Yamaji and Ma2009; Ma et al. Reference Ma, Tamai, Yamaji, Mitani, Konishi, Katsuhara, Ishiguro, Murata and Yano2006, Reference Ma, Yamaji, Mitani, Tamai, Konishi, Fujiwara, Katsuhara and Yano2007). In passive transport, soil moisture is one of the primary limiting factors of mineral uptake (Bloom et al. Reference Bloom, Chapin and Mooney1985; Hu and Schmidhalter Reference Hu and Schmidhalter2005). In semiarid environments where soil moisture is limited, grass species display low to moderate levels of tissue Si concentrations (Shewmaker et al. Reference Shewmaker, Mayland, Rosenau and Asay1989), likely due to passive transport. Taeniatherum caput-medusae has tissue silica concentrations similar to that of rice, although it is unknown whether it uses an active or passive transport mechanism. An active transport mechanism is more plausible, as concentrations of T. caput-medusae tissue silica are greater than those of other semiarid species (Bovey et al. Reference Bovey, Tourneau and Erickson1961; Swenson et al. Reference Swenson, Le Tourneau and Erickson1964).
As soluble Si is transported to various parts of a plant, it is dehydrated and polymerized into a di- or poly-silicic acid, which can be further polymerized within cellular structures to silica (Casey et al. Reference Casey, Kinrade, Knight, Rains and Epstein2004; Ma and Yamaji Reference Ma and Yamaji2006; Mitani and Ma Reference Mitani and Ma2005). Cellular constituents such as hemicellulose, callose, pectin, and lignin have been shown to provide a framework for deposition (Guerriero et al. Reference Guerriero, Hausman and Legay2016). Tissue silica can also take on various forms, depending on deposition location, cell shape, and environmental conditions (Blackman Reference Blackman1971; Li et al. Reference Li, Song and Cornelis2014). The location for silica deposition also varies greatly among plant species (Lewin and Reimann Reference Lewin and Reimann1969; Lux et al. Reference Lux, Luxová, Abe, Tanimoto, Hattori and Inanaga2003). Tissue silica bodies have been observed in all major aboveground plant parts of T. caput-medusae, including sausage-shaped bodies beneath the epidermis of culms and a varnish-like structure on the awns as silicified barbs (Swenson et al. Reference Swenson, Le Tourneau and Erickson1964).
Tissue Silicon and Taeniatherum caput-medusae Plant Characteristics
Plant Fitness
High tissue Si in T. caput-medusae may influence many factors associated with plant fitness that can be linked to plant productivity (Figure 1). For instance, tissue Si may increase root development, slow litter decomposition, alter soil conditions, and contribute to microsite conditions that favor T. caput-medusae germination. Overall, high tissue Si likely has a cascade of net positive effects on T. caput-medusae productivity and abundance within plant communities, which in turn strengthens the feedback cycle of invasion.
Seedbed Conditions
Successful seedling establishment in plants is often determined by the number of safe sites provided by the soil surface (Evans and Young Reference Evans and Young1970; Harper et al. Reference Harper, Williams and Sagar1965). Litter can create a favorable microenvironment for T. caput-medusae seeds that do not arrive at soil safe sites. For instance, as a consequence of high tissue Si concentrations, T. caput-medusae litter is slow to decompose (Figure 1), contributing to years of persistent decadent plant material (Bovey et al. Reference Bovey, Tourneau and Erickson1961; Young Reference Young1992). While many plant components such as lignin (Aerts Reference Aerts1997; Laishram and Yadava Reference Laishram and Yadava1988; Stott et al. Reference Stott, Kassim, Jarrell, Martin and Haider1983), tannin-protein complexes (Palm Reference Palm1988), and high C:N and C:P ratios (Goldman et al. Reference Goldman, Caron and Dennett1987) reduce litter decomposition rates, Si is thought to be the primary contributor to slowed decay rates in T. caput-medusae (Torell et al. Reference Torell, Erickson and Haas1961; Young Reference Young1992). Evans and Young (Reference Evans and Young1970) found that T. caput-medusae litter reduces seasonal and daily temperature fluctuations and water evaporation and increases humidity, which together contribute to a more favorable seedling microenvironment. The awns of T. caput-medusae seeds also get caught within the litter, limiting seed burial and intimate contact with the soil surface (Evans and Young Reference Evans and Young1970). For other plant species, this may pose a dire problem, as they require direct contact with a moisture-supplying substrate such as the soil, but T. caput-medusae seeds absorb moisture from the litter environment (i.e., they are hygroscopic) (Young Reference Young1992). In support of the favorable, self-induced seedbed conditions, T. caput-medusae was found to produce 47 times more seedlings within its litter environment than on bare ground conditions (Evans and Young Reference Evans and Young1970). This is not to say that T. caput-medusae cannot germinate without the presence of litter, but rather the accumulation of litter enhances T. caput-medusae establishment and persistence.
Root Development
Cheatgrass (Bromus tectorum L.) and T. caput-medusae roots develop at comparable rates and can grow to depths of 100 cm (Hironaka Reference Hironaka1961). Both annual grasses primarily germinate in the autumn, continue slow root growth through the winter, and have greater root elongation into spring and summer than newly established native perennial grass species (Harris Reference Harris1977; Hironaka Reference Hironaka1961; Vasquez et al. Reference Vasquez, Sheley and Svejcar2008). Collectively, these unique root development traits enable T. caput-medusae and similar annual grasses to gain a competitive advantage over perennial plant species through greater resource acquisition (James et al. Reference James, Ziegenhagen and Aanderud2010; Vasquez et al. Reference Vasquez, Sheley and Svejcar2008; Young and Mangold Reference Young and Mangold2008).
Taeniatherum caput-medusae diverges from other invasive annual grass species when it comes to root cell size and structure. For instance, Harris (Reference Harris1977) compared the root cellular composition of T. caput-medusae, B. tectorum, and a perennial grass through photomicrographs and showed that T. caput-medusae had thicker cell walls and overall larger root diameters than B. tectorum, but lower values than the perennial grass species. It was proposed that the thicker cellular root endodermis may allow for transpiration to occur even if the surrounding soil environment is drier, particularly in the upper soil horizons. When a Si-based fertilizer was applied to a variety of plant species (e.g., sorghum [Sorghum bicolor (L.) Moench], rice, wheat, and potatoes [Solanum tuberosum L.]), root endodermis cells were more rigid and efficient in water transpiration under moisture-lacking conditions (Chen et al. Reference Chen, Yao, Cai and Chen2011; Crusciol et al. Reference Crusciol, Pulz, Lemos, Soratto and Lima2009; Gong et al. Reference Gong, Zhu, Chen, Wang and Zhang2005; Hattori et al. Reference Hattori, Inanaga, Tanimoto, Lux, Luxová and Sugimoto2003). In general, Si has been associated with increased plant tissue rigidity in several plant species (Ma et al. Reference Ma, Tamai, Yamaji, Mitani, Konishi, Katsuhara, Ishiguro, Murata and Yano2006; Namaganda et al. Reference Namaganda, Krekling and Lye2009; Raven Reference Raven1983). Despite considerable information on Si and its beneficial role in other plants, there is very limited information pertaining to T. caput-medusae roots and how Si influences root development.
Soil Properties
Taeniatherum caput-medusae can be found on a variety of soil types, but more frequently in clayey soils as opposed to coarse-textured, sandy soils (Dahl and Tisdale Reference Dahl and Tisdale1975). Because clayey soils are known for their high water-holding capacity and shrink–swell characteristics, which create large soil cracks and/or fissures when they dry, plant seedlings and their roots can experience increased desiccation as cracks develop (Young et al. Reference Young, Clements and Nader1999). However, T. caput-medusae roots are well adapted to this potential threat. For instance, if the primary root of T. caput-medusae is injured or dries out, an adventitious root emerges to replace the primary root (Young Reference Young1992). Vascular tissues have been found to be strengthened by Si accumulation and may be able to withstand the shrinkage-induced tissue stress from the drying of clayey soils (Hattori et al. Reference Hattori, Inanaga, Tanimoto, Lux, Luxová and Sugimoto2003). With high tissue Si concentrations, T. caput-medusae likely responded more favorably (i.e., greater root growth) on clayey soils and large/infrequent watering treatments compared with other invasive annual grasses such as B. tectorum or ventenata [Ventenata dubia (Leers) Coss.] (Bansal et al. Reference Bansal, James and Sheley2014).
Edaphic conditions, such as nutrient availability, may also be related to T. caput-medusae invasion. A transplant study between T. caput-medusae seeds from California and France showed that California soils produced larger plants than those from France (Blank and Sforza Reference Blank and Sforza2007). It was proposed that California soils have greater nutrient content than those from France. In a comparative growth study, nitrogen and phosphorus additions increased biomass production of B. tectorum more so than T. caput-medusae (Dakheel et al. Reference Dakheel, Radosevich and Barbour1993). In the same study, when soils were deficient in phosphorus, B. tectorum also outproduced T. caput-medusae. Similarly, B. tectorum produced more shoot mass than T. caput-medusae when grown in different nitrogen nutrient solutions (MacKown et al. Reference MacKown, Jones, Johnson, Monaco and Redinbaugh2009). Nevertheless, none of these studies reported soil or plant Si concentrations, likely due to the fact that Si is not considered an essential element of plant nutrition (Arnon and Stout Reference Arnon and Stout1939; Epstein Reference Epstein1972). Because Si has been shown to increase root and leaf elongation in hyperaccumulator plant species (i.e., sorghum and rice) (Hattori et al. Reference Hattori, Inanaga, Tanimoto, Lux, Luxová and Sugimoto2003; Hossain et al. Reference Hossain, Mori, Soga, Wakabayashi, Kamisaka, Fujii, Yamamoto and Hoson2002), it is possible that the combination of Si dissolution in clayey soils (Tubaña and Heckman Reference Tubaña, Heckman, Rodrigues and Datnoff2015) and the potentially high uptake capacity of soluble Si by T. caput-medusae may facilitate increased growth and offset the competitive superiority of B. tectorum described in the aforementioned studies.
Plant Structure
Tissue Si may influence many plant structural factors of T. caput-medusae, such as metal toxicity resistance, protection from ultraviolet-b (UV-B) radiation damage, increased culm rigidity, and upregulation of the innate immune system. These factors may directly decrease litter decomposition rate and herbivory, indirectly increase plant fitness and productivity, and contribute to the overall abundance of T. caput-medusae in the plant community (Figure 1).
Metal Toxicity Resistance
The accumulation of toxic metals can negatively impact plant fitness (Nagajyoti et al. Reference Nagajyoti, Lee and Sreekanth2010), and evidence suggests that tissue Si mitigates this toxicity through structural adaptations within the plant (Ma Reference Ma2004). An apoplastic root membrane was shown to regulate the translocation of metals through plant cell walls (Emamverdian et al. Reference Emamverdian, Ding, Xie and Sangari2018). Deposition of Si within the membrane was thought to decrease the porosity of the cell wall, consequently reducing the movement of toxic metals and salts into the plant (Coskun et al. Reference Coskun, Deshmukh, Sonah, Menzies, Reynolds, Ma, Kronzucker and Bélanger2019; Gong et al. Reference Gong, Randall and Flowers2006; Wu et al. Reference Wu, Shi, Zhu, Wang and Gong2013). Some toxic metals may pass through the membrane, but soluble Si can form mineral complexes to increase adsorption and reduce toxic metal movement within plants (Keller et al. Reference Keller, Rizwan, Davidian, Pokrovsky, Bovet, Chaurand and Meunier2015; Wang et al. Reference Wang, Stass and Horst2004; Ye et al. Reference Ye, Yan, Liu, Lu, Liu and Song2012). However, Adrees et al. (Reference Adrees, Ali, Rizwan, Zia-ur-Rehman, Ibrahim, Abbas, Farid, Qayyum and Irshad2015) suggested that changes in root structure were not the only mechanism of decreased toxic metal uptake. Modulation of the influx transporters by Si may also decrease toxic metal uptake. Taeniatherum caput-medusae was found to colonize waste mine sites high in toxic metals, although the aforementioned mechanisms of reduced metal toxicity were not addressed (Martínez-Ruiz et al. Reference Martínez-Ruiz, Fernández-Santos and Gómez-Gutiérrez2001; Reglero et al. Reference Reglero, Monsalve-González, Taggart and Mateo2008). Consequently, these mechanisms could reduce metal toxicity in T. caput-medusae, contributing to its invasion, but further exploration is needed.
Photosynthetic Barrier
Reductions in the atmospheric ozone layer have resulted in increased UV-B radiation reaching the earth’s surface (Madronich et al. Reference Madronich, McKenzie, Björn and Caldwell1998). Increased UV-B radiation can damage leaves and reduce the photosynthetic capacity of plants (Kakani et al. Reference Kakani, Reddy, Zhao and Sailaja2003). Supplementing rice with soluble Si reduced UV-B damage through increased leaf rigidity and the production of phenolic compounds (Li et al. Reference Li, Shi, Wang and Zhang2004; Tamai and Ma Reference Tamai and Ma2008). The presence of tissue Si within the cellular membranes also mitigated UV-B–induced reactive oxygen species, which are known to cause membrane damage, decrease enzyme activity, increase electrolyte leakage, and alter gene expression (Coskun et al. Reference Coskun, Deshmukh, Sonah, Menzies, Reynolds, Ma, Kronzucker and Bélanger2019; Shen et al. Reference Shen, Zhou, Duan, Li, Eneji and Li2010). Despite these studies, the relationship between photosynthesis and tissue Si in T. caput-medusae has not been evaluated.
Culm Rigidity
Taeniatherum caput-medusae plants can reach a height of more than 60 cm, which is impressive, given that its culms are typically thin (e.g., <1-mm diameter) and inflorescences are relatively large and “wispy” (McKell et al. Reference McKell, Robison and Major1962), possibly making them susceptible to lodging and breakage (Savant et al. Reference Savant, Snyder, Datnoff and Sparks1996). Culm integrity of T. caput-medusae is potentially enhanced due to the accumulation of silica, which increases the rigidity of the stem and leaves of certain plants (Ma et al. Reference Ma, Tamai, Yamaji, Mitani, Konishi, Katsuhara, Ishiguro, Murata and Yano2006; Namaganda et al. Reference Namaganda, Krekling and Lye2009). For instance, rice lodging was reduced with increasing tissue Si concentrations (Lee et al. Reference Lee, Kwon and Park1990; Savant et al. Reference Savant, Snyder, Datnoff and Sparks1996). However, no studies have examined the associations between culm rigidity in T. caput-medusae, tissue Si, and lodging.
Immune System Regulation
Wagner (Reference Wagner1940) was the first to suggest that tissue Si increases plant defenses against stem-boring pathogens; this is known as the mechanical barrier hypothesis. However, studies in rice (Rodrigues et al. Reference Rodrigues, Benhamou, Datnoff, Jones and Bélanger2003, Reference Rodrigues, McNally, Datnoff, Jones, Labbé, Benhamou, Menzies and Bélanger2004) and wheat (Bélanger et al. Reference Bélanger, Benhamou and Menzies2003; Rémus-Borel et al. Reference Rémus-Borel, Menzies and Bélanger2009) have shown that there is an upregulation of the innate immune system (e.g., chitinases, peroxidase, polyphenol oxidases, phytoalexins, and phenolic compounds) following predation rather than the aforementioned physical defense. Tissue Si is known to act as a modulator of these plant defenses. For instance, tissue Si–associated defenses increase the binding affinity of plant proteins within the defense signaling pathway, preventing these pathogenic enzymes from reaching their target (Datnoff et al. Reference Datnoff, Elmer and Huber2007; Fauteux et al. Reference Fauteux, Rémus-Borel, Menzies and Bélanger2005). Recent work on pathogens and stem-boring insects demonstrated that these predators release effector proteins that interfere with the defense signaling pathway (Giraldo and Valent Reference Giraldo and Valent2013; Mugford et al. Reference Mugford, Barclay, Drurey, Findlay and Hogenhout2016). Soluble Si deposition strengthens the apoplastic membrane and prevents the effector proteins from reaching their target (Holub and Cooper Reference Holub and Cooper2004; Nuernberger and Lipka Reference Nuernberger and Lipka2005). Another postulation is that tissue Si allows for the redistribution of energy stores to upregulate defense responses. For instance, Si-accumulating plants may replace energy-dependent processes involved in building structural carbohydrates for non–energy dependent ones (McNaughton et al. Reference McNaughton, Tarrants, McNaughton and Davis1985; Van Soest Reference Van Soest2006). Energy stores that would otherwise be used for growth and development of plant structural components are redistributed to the innate immune system (Coskun et al. Reference Coskun, Deshmukh, Sonah, Menzies, Reynolds, Ma, Kronzucker and Bélanger2019). Overall, the redistribution of energy stores and production of a tissue Si physical barrier allow for a stronger defense response upon predation. However, these theories on tissue Si and the upregulation of the innate immune response have not been explored in T. caput-medusae.
Plant Texture
Invertebrate insects (Massey et al. Reference Massey, Ennos and Hartley2006), voles (Massey and Hartley Reference Massey and Hartley2006), rabbits (Cotterill et al. Reference Cotterill, Watkins, Brennon and Cowan2007), livestock (Massey et al. Reference Massey, Massey, Roland Ennos and Hartley2009; Shewmaker et al. Reference Shewmaker, Mayland, Rosenau and Asay1989), small granivores, and birds (Longland Reference Longland1994; Savage et al. Reference Savage, Young and Evans1969) all show decreased preference for Si-rich grasses. This avoidance may be a consequence of an undesirable oral texture. For instance, T. caput-medusae inflorescences have a large vertical awn attached to the seed, with shorter lateral spiked glumes (Miller et al. Reference Miller, Clausnitzer, Borman, Sheley and Petroff1999). Microscopic examination of T. caput-medusae awns revealed that they contain a Si varnish with barbs (Swenson et al. Reference Swenson, Le Tourneau and Erickson1964), which may contribute to the undesirable texture causing irritation to mouthparts (Massey and Hartley Reference Massey and Hartley2009). In addition, tissue Si in grasses has been associated with increased tooth wear (Baker et al. Reference Baker, Jones and Wardrop1959), gastrointestinal urolithiasis (Bailey Reference Bailey1981), and esophageal tumors (O’Neill et al. Reference O’Neill, Clarke, Hodges, Jordan, Newman, Pan, Liu, Ge, Chang and Toulson1982, Reference O’Neill, Hodges, Riddle, Jordan, Newman, Flood and Toulson1980), possibly causing further T. caput-medusae avoidance.
Disarticulation of seeds from the seed head occurs from July to October, with the majority being dropped in August (Davies Reference Davies2008), providing an opportunity for herbivory without the consequence of oral irritation. However, Si bodies are still present in the stem (Swenson et al. Reference Swenson, Le Tourneau and Erickson1964), and likely in the spiked glume after seed disarticulation, and continue to provide an abrasive texture. In contrast, other annual grasses such as B. tectorum lack these short spiked glumes, and when seed drop occurs, the plants become susceptible to herbivory (Vallentine and Stevens Reference Vallentine and Stevens1994). Overall, the texture caused by T. caput-medusae tissue Si may deter herbivory of T. caput-medusae, facilitating a self-reinforcing positive feedback cycle.
Chemical Composition
Tissue Si concentrations have a direct effect on the nutrient content and digestibility of T. caput-medusae (Figure 1). These effects negatively impact herbivory and increase litter accumulation, which both directly and indirectly increase T. caput-medusae abundance within the plant community.
Nutritive Quality
Tissue nutrient concentrations and their association to plant secondary compounds or toxins largely influence the likelihood of a plant being consumed by an herbivore (Provenza et al. Reference Provenza, Villalba and Bryant2002). Bovey et al. (Reference Bovey, Tourneau and Erickson1961) showed that T. caput-medusae contains 10.4% crude protein (CP), 2.6% fat, 26.8% crude fiber, 6.1% lignin, and 13.9% ash during the vegetative phenological stage. Subsequently, rapid maturation causes a decline in CP and fat content, while fiber, lignin, and ash concentrations increase. Other more recent studies show similar trends for the nutritional composition of T. caput-medusae over time (Montes-Sánchez and Villalba Reference Montes-Sánchez and Villalba2017; Villalba and Burritt Reference Villalba and Burritt2015). Plant ash is an indicator of tissue silica content, and silica in ash comprises values greater than 90% in rice plants (Charca et al. Reference Charca, Guzman and Flora2007; Yalçin and Sevinç Reference Yalçin and Sevinç2001). These values can be lower, depending on plant species and tissue location (Lanning et al. Reference Lanning, Hopkins and Loera1980; Lanning and Eleuterius Reference Lanning and Eleuterius1983). Taeniatherum caput-medusae ash comprises more than 70% tissue silica, whereas B. tectorum comprises 47% (Bovey et al. Reference Bovey, Tourneau and Erickson1961). In the early phenological stages, T. caput-medusae has similar nutritional value to that of other desirable grass, and livestock grazing can be used as a method of control (Bovey et al. Reference Bovey, Tourneau and Erickson1961; DiTomaso et al. Reference DiTomaso, Kyser, George, Doran and Laca2008; Hamilton et al. Reference Hamilton, Burritt and Villalba2015; Villalba and Burritt Reference Villalba and Burritt2015). Because of its rapid maturation, decline in nutritional value, and high silica concentrations, the window of palatability is limited to 2 to 4 wk (Brownsey et al. Reference Brownsey, James, Barry, Becchetti, Davy, Doran, Forero, Harper, Larsen, Larson-Praplan, Zhang and Laca2017). After this period, consumption of T. caput-medusae is reduced, and grazing pressure on more nutritious plant species may occur and be deleterious (DiTomaso and Smith Reference DiTomaso and Smith2012; Sheley et al. Reference Sheley, Bingham and Svejcar2008). Even if T. caput-medusae was palatable after seed drop, the nutritional quality of the plant is inadequate to sustain grazers. Domestic ungulates require a minimum of ~7.5% CP for body maintenance (National Research Council 2000, 2007a, 2007b), and supplementation is required for animals grazing most grasses at later phenological stages (Ganskopp and Bohnert Reference Ganskopp and Bohnert2001). Animal supplementation of nutrient-rich forages in combination with T. caput-medusae were shown to increase consumption of the troublesome grass (Hamilton et al. Reference Hamilton, Burritt and Villalba2015; Montes-Sánchez et al. Reference Montes-Sánchez, Van Miegroet and Villalba2017; Stonecipher et al. Reference Stonecipher, Panter and Villalba2016). Nevertheless, in these studies, T. caput-medusae consumption was low and would not likely discourage T. caput-medusae abundance within the plant community. Additionally, tissue Si was unaltered when fed to grazers, and thus the defense mechanism of the plant still existed, possibly limiting utilization of the chemical constituents within. Supplemental grazing of T. caput-medusae in the autumn also does not reduce the soil seedbank, as seed drop has already occurred, and may actually increase T. caput-medusae abundance in subsequent years (DiTomaso et al. Reference DiTomaso, Kyser, George, Doran and Laca2008; Lusk et al. Reference Lusk, Jones, Torell and Kell1961). These studies indicate that similar to plant texture (discussed earlier), chemical composition alone does not explain the low intake and palatability of T. caput-medusae.
Digestibility
Tissue Si within plants has been proposed as a defense mechanism against herbivory (Hunt et al. Reference Hunt, Dean, Webster, Johnson and Ennos2008), similar to those induced by plant secondary compounds or toxins (Provenza et al. Reference Provenza, Villalba and Bryant2002). An epidermal silica varnish in T. caput-medusae acts as a physical barrier limiting the degradation of the cell wall by rumen microorganisms (Hunt et al. Reference Hunt, Dean, Webster, Johnson and Ennos2008; Montes-Sánchez and Villalba Reference Montes-Sánchez and Villalba2017). This barrier prevents utilization of the organic constituents beneath such a layer (Mayland and Shewmaker Reference Mayland and Shewmaker2001; Van Soest Reference Van Soest1994; Van Soest and Jones Reference Van Soest and Jones1968). Support for this reduced digestion mechanism was shown by Montes-Sánchez and Villalba (Reference Montes-Sánchez and Villalba2017), who reported declines in digestibility of T. caput-medusae with increments in the plant’s particle size; with larger particles conserving the tissue Si barrier to a greater extent than particles of a smaller size. Although lignin content has been associated with decreased digestibility of certain plants, differences in T. caput-medusae digestion rates were not influenced by this factor, as alfalfa (Medicago sativa L.) and tall fescue (Schedonorus arundinaceus (Schreb.) Dumort., nom. cons.) hay had lignin concentrations similar to those present in T. caput-medusae. In addition, soluble Si limits enzymatic activity (Kind et al. Reference Kind, King, Pash, Roman and Schmidt1954), thus decreasing plant digestibility in the rumen (Smith et al. Reference Smith, Nelson and Boggino1971). Finally, for every percentile unit increase in tissue silica, there are between 1 and 3 percentile units of reduction in forage digestibility (Smith et al. Reference Smith, Nelson and Boggino1971; Van Soest and Jones Reference Van Soest and Jones1968). In avian species, such as chukar partridges (Alectoris graeca Meisner), B. tectorum seeds were preferred over T. caput-medusae seeds (Savage et al. Reference Savage, Young and Evans1969), likely due to undesirable high tissue Si in T. caput-medusae and a concomitant lower digestibility, but other factors such as seed size may influence ingestion.
Traditional Control Strategies
Commonly used T. caput-medusae control strategies can be categorized into mechanical, cultural, and chemical. Each addresses certain aspects of the Si positive feedback cycle (Figure 1). Integrating multiple strategies is often more successful than using one strategy by itself (Davies Reference Davies2010; Davies and Sheley Reference Davies and Sheley2011; Kyser et al. Reference Kyser, DiTomaso, Doran, Orloff, Wilson, Lancaster, Lile and Porath2007; Monaco et al. Reference Monaco, Osmond and Dewey2005). This section will address individual strategies and their roles in the Si positive feedback cycle, potentially giving insight into why integrated control is often more successful.
Mechanical Control
Mowing
A window of opportunity for mowing T. caput-medusae was proposed, which was ~35 d from late vegetative to early reproductive phenological stage (Brownsey et al. Reference Brownsey, James, Barry, Becchetti, Davy, Doran, Forero, Harper, Larsen, Larson-Praplan, Zhang and Laca2017). The same study estimated that mowing before seed head emergence decreased T. caput-medusae seed production by ~50%. However, early spring mowing may miss younger plants and allow recovery of damaged plants, enabling T. caput-medusae to produce seeds and only temporarily reduce plant productivity. The optimal timing of mowing and control should be tailored to occur when the majority of plants are in the boot phenological stage, thus limiting seed production and additional late-maturing plants. Plant Si has been shown to increase plant tissue rigidity (Ma et al. Reference Ma, Tamai, Yamaji, Mitani, Konishi, Katsuhara, Ishiguro, Murata and Yano2006; Namaganda et al. Reference Namaganda, Krekling and Lye2009; Raven Reference Raven1983) and potentially decrease microbial degradation, increasing litter accumulation (Torell et al. Reference Torell, Erickson and Haas1961; Young Reference Young1992). Mowing mulches the standing biomass and may artificially accelerate litter decomposition, but it may also create favorable microsites for T. caput-medusae seedlings (Evans and Young Reference Evans and Young1970). Taeniatherum caput-medusae seeds remain viable for up to 3 yr (Nelson and Wilson Reference Nelson and Wilson1969); thus, repeated mowing treatments must be applied, which may lead to deleterious impacts on native plant species (Davies et al. Reference Davies, Bates and Nafus2012). The small window of opportunity for mowing may also be impractical for repeated application over large-scale infestations. Finally, T. caput-medusae infestations often occur on landscapes that are inaccessible to machinery due to slope and terrain, as well as being on uneven, rocky soils that can damage equipment (Young Reference Young1992). Overall, mowing may partially address the plant fitness and structural characteristics of T. caput-medusae within the Si positive feedback cycle (Figure 1), but it does not address the chemical composition of the plant.
Tillage
Tilling can break up litter, incorporating it into the soil, and create favorable seedbed conditions for revegetation efforts (Kaltenecker Reference Kaltenecker1997). In addition, tilling can damage T. caput-medusae roots, bury germinable seeds, and reduce T. caput-medusae cover by ~50% (Kyser et al. Reference Kyser, DiTomaso, Doran, Orloff, Wilson, Lancaster, Lile and Porath2007). These impacts on T. caput-medusae address both plant fitness and structure in the Si positive feedback cycle (Figure 1). It has been shown that T. caput-medusae seeds emerge poorly at depths greater than 5 cm (Young et al. Reference Young, Evans and Eckert1969). However, tillage may also increase erosion potential, facilitate soil moisture and organic matter losses, and have deleterious effects on native vegetation (Nafus and Davies Reference Nafus and Davies2014; Pierson et al. Reference Pierson, Blackburn and Van Vactor2007). Soil disturbance has been shown to favor T. caput-medusae invasion through increased standing biomass and seed production in the subsequent year (Miller Reference Miller1996). As with mowing, tillage may not be feasible, due to topographic features and surface obstacles that could damage equipment. Finally, tillage may negatively impact fragile biological crusts and interrupt nutrient cycling, all while providing a disturbance regime that favors additional undesirable exotic plant species (Kaltenecker Reference Kaltenecker1997; Locke and Bryson Reference Locke and Bryson1997; Young Reference Young1992).
Cultural Control
Prescribed Fire
Prescribed fire can consume standing T. caput-medusae biomass, seeds, and litter (Kyser et al. Reference Kyser, Doran, McDougald, Orloff, Vargas, Wilson and DiTomaso2008), while potentially interrupting aspects of the Si positive feedback cycle (Figure 1). Fire may also provide an opportunity for revegetation and additional treatments (Davies Reference Davies2010; Davies et al. Reference Davies, Boyd, Johnson, Nafus and Madsen2015). Taeniatherum caput-medusae matures 2 to 4 wk later than other annual grasses, including B. tectorum (Dahl and Tisdale Reference Dahl and Tisdale1975; Hironaka Reference Hironaka1961; Young Reference Young1992), and retains its seeds within inflorescences until August (Davies Reference Davies2008). Thus, timing a prescribed fire to coincide with viable seed retention can destroy these seeds. Coordinating the ideal timing and achieving suitable conditions for a prescribed fire may be difficult in the Intermountain Region of the western United States due to low air temperatures in the autumn/winter and insufficient amounts of combustible material in the spring and early summer (Kyser et al. Reference Kyser, Doran, McDougald, Orloff, Vargas, Wilson and DiTomaso2008). Delaying prescribed fires to late summer or early autumn raises the risk of escape due to the contribution of other community vegetation to the overall fuel load. After seed drop, fires must reach temperatures greater than 250 C to consume seeds located on the soil surface (DiTomaso et al. Reference DiTomaso, Kyser and Hastings1999; Sweet et al. Reference Sweet, Kyser and DiTomaso2008). Slow-burning, high-temperature fires may result in unintended damage to desirable plant species, increasing the dominance of fire-adapted invasive annual grasses (Billings Reference Billings1994; Whisenant Reference Whisenant1990), possibly perpetuating the grass-fire feedback cycle (D’Antonio and Vitousek Reference D’Antonio and Vitousek1992). Uncontrolled fires have been shown to create opportunities for exotic plant establishment and contribute to air pollution and atmospheric CO2 that impact the climate (Campbell and Cahill Reference Campbell and Cahill1996; D’Antonio and Vitousek Reference D’Antonio and Vitousek1992; Peters and Bunting Reference Peters and Bunting1994; Young Reference Young1992). Fire also alters soil infiltration rates, soil porosity, soil hydraulic conductivity, and soil water storage capacity (Neary et al. Reference Neary, Klopatek, DeBano and Ffolliott1999). Despite these difficulties and risks, under optimal timing and conditions, prescribed fire has been shown to successfully reduce T. caput-medusae populations in the California annual grasslands (Kyser et al. Reference Kyser, Doran, McDougald, Orloff, Vargas, Wilson and DiTomaso2008; Meyer and Schiffman Reference Meyer and Schiffman1999).
Grazing
Livestock grazing has been shown to be one of the preferred methods of T. caput-medusae control due to its low cost and practicality (Hamilton et al. Reference Hamilton, Burritt and Villalba2015; James et al. Reference James, Gornish, DiTomaso, Davy, Doran, Becchetti, Lile, Brownsey and Laca2015; Johnson et al. Reference Johnson, Davies, Schreder and Chamberlain2011), positive effects on nutrient cycling (Davies et al. Reference Davies, Bates, Svejcar and Boyd2010; Hobbs Reference Hobbs1996), and limited disturbance compared with other control methods (e.g., mowing, tillage, and fire). For instance, livestock grazing removes standing vegetation and litter, and the deposition of animal wastes (i.e., feces and urine), which are high in nitrogen (N), can directly influence soil N mineralization (Davies et al. Reference Davies, Bates, Svejcar and Boyd2010; Hobbs Reference Hobbs1996). However, livestock consumption of T. caput-medusae has a narrow time frame of palatability before seed head emergence; livestock tend to avoid the plant after this time (DiTomaso et al. Reference DiTomaso, Kyser, George, Doran and Laca2008; Young Reference Young1992). Livestock avoidance may be due to high tissue Si concentration, which presents an abrasive oral texture (McNaughton et al. Reference McNaughton, Tarrants, McNaughton and Davis1985) and limits rumen digestibility (Montes-Sánchez and Villalba Reference Montes-Sánchez and Villalba2017). The plant’s structure and chemical composition may directly and indirectly increase T. caput-medusae abundance in the plant community (Figure 1) through reduced livestock consumption and repeated defoliation of other, more palatable grasses (Belsky and Gelbard Reference Belsky and Gelbard2000; Heady Reference Heady1961; Hunt et al. Reference Hunt, Dean, Webster, Johnson and Ennos2008; Mueggler Reference Mueggler1972). Grazing before seed head emergence has been shown to overcome these obstacles, but the window of opportunity is narrow (Brownsey et al. Reference Brownsey, James, Barry, Becchetti, Davy, Doran, Forero, Harper, Larsen, Larson-Praplan, Zhang and Laca2017) and high stocking rates when T. caput-medusae is most palatable may not be feasible (DiTomaso et al. Reference DiTomaso, Kyser, George, Doran and Laca2008). Grazing has been shown to be successful in the California annual grasslands when proper timing and stocking rates are considered (DiTomaso et al. Reference DiTomaso, Kyser, George, Doran and Laca2008; Lusk et al. Reference Lusk, Jones, Torell and Kell1961). In contrast, year-round grazing and early spring grazing saw increases in T. caput-medusae abundance (DiTomaso et al. Reference DiTomaso, Kyser, George, Doran and Laca2008; Harrison et al. Reference Harrison, Inouye and Safford2003). Overall, grazing can be used as a method of control to address the Si positive feedback cycle (Figure 1) if high-intensity grazing is timed to occur when T. caput-medusae is in the boot phenological stage.
Chemical Control
Herbicides are the primary form of chemical control of T. caput-medusae and have the potential to reduce plant fitness, alter plant structural components, and increase nutritive quality, consequently interrupting the Si positive feedback cycle (Figure 1). There are many herbicides labeled for the control of T. caput-medusae, including PRE, POST, broad-spectrum, grass-selective, and growth-regulator herbicides. Imazapic and rimsulfuron are two PRE herbicides that have been used to control T. caput-medusae (Davies Reference Davies2010; Davies and Sheley Reference Davies and Sheley2011; Kyser et al. Reference Kyser, DiTomaso, Doran, Orloff, Wilson, Lancaster, Lile and Porath2007, Reference Kyser, Peterson, Davy and DiTomaso2012b; Monaco et al. Reference Monaco, Osmond and Dewey2005; Sheley et al. Reference Sheley, Carpinelli and Morghan2007), but they are highly variable in their efficacy (Kyser et al. Reference Kyser, DiTomaso, Doran, Orloff, Wilson, Lancaster, Lile and Porath2007, Reference Kyser, Peterson, Davy and DiTomaso2012b). This may in part be due to the accumulated litter inhibiting penetration of the herbicide to the soil surface (Kyser et al. Reference Kyser, DiTomaso, Doran, Orloff, Wilson, Lancaster, Lile and Porath2007) or rapid degradation in warmer soils (Kyser et al. Reference Kyser, Peterson, Davy and DiTomaso2012b). A new herbicide, indazaflam, has been shown to effectively control annual grasses through its PRE residual capacity (Sebastian et al. Reference Sebastian, Nissen and De Souza Rodrigues2016a, Reference Sebastian, Sebastian, Nissen and Beck2016b, Reference Sebastian, Nissen, Sebastian and Beck2017a, Reference Sebastian, Nissen, Sebastian, Meiman and Beck2017b), but it is not yet labeled for areas grazed by livestock. This poses a problem, as most areas heavily infested with T. caput-medusae are also used for livestock production. Aminopyralid, a PRE growth-regulator herbicide, was shown to suppress T. caput-medusae seed production >95% in a greenhouse setting (Rinella et al. Reference Rinella, Bellows and Roth2014), with similar success in the field (Rinella et al. Reference Rinella, Davy, Kyser, Mashiri, Bellows, James and Peterson2018). Glyphosate, a broad-spectrum POST herbicide, has been used at low rates to decrease T. caput-medusae abundance by ~95% with limited damage to shrubs and other native vegetation in big sagebrush (Artemisia tridentata Nutt.)–scrub ecosystems (Kyser et al. Reference Kyser, Creech, Zhang and DiTomaso2012a). Glyphosate application before seed set may also temporarily decrease plant fitness by reducing seed production and viability and arresting root development (Figure 1). However, multiple applications are needed, as glyphosate is a foliar contact herbicide and does not directly influence the soil seedbank (Kyser et al. Reference Kyser, Creech, Zhang and DiTomaso2012a). Even with the potential of T. caput-medusae herbicide control, there are risks associated with herbicide application. For instance, spray drift due to wind, volatilization, and different herbicide formulations may cause nonuniform application and injury to non-target species (DiTomaso Reference DiTomaso1997). Repetitive herbicide application selects for herbicide-tolerant plants and may decrease desirable plant species within the population (DiTomaso Reference DiTomaso1997). Chemical application is often temporary, with multiple applications needed, but has shown potential for T. caput-medusae control and interrupting the Si positive feedback cycle.
Research Needs
Although we know orthosilicic acid is the soluble form taken up by many plants (Ma and Yamaji Reference Ma and Yamaji2006) and deposited as amorphous mineral silica in T. caput-medusae tissues (Bovey et al. Reference Bovey, Tourneau and Erickson1961; Epstein Reference Epstein1999; Swenson et al. Reference Swenson, Le Tourneau and Erickson1964), critical knowledge gaps exist. In particular, our understanding of how Si is transported through the soil to the root of T. caput-medusae, how roots respond to available Si, what mechanisms within the root are responsible for uptake, and how the Si is transported through the plant are largely unknown. In addition, addressing ways to constrain tissue Si deposition within T. caput-medusae may be key to interrupting the positive feedback cycle of invasion and facilitate control of T. caput-medusae. Consequently, there are critical research needs in relation to plant Si and T. caput-medusae that may lead to alternative methods of control and more successful management strategies.
The Soil
The normal range of soluble Si in soil is between 0.6 to 1.0 mM but can be higher or lower depending on soil weathering processes (Epstein Reference Epstein1994). When soluble Si concentrations exceed 2 mM, the Si polymerizes into a gel and becomes unavailable for plant uptake (Ma et al. Reference Ma, Miyake, Takahashi, Datnoff, Snyder and Korndörfer2001). Rice and an Equisetum species began to wilt and leaves became necrotic when grown in deficient soluble Si conditions (Chen and Lewin Reference Chen and Lewin1969; Yoshida et al. Reference Yoshida, Ohnishi and Kitagishi1962). Reduced growth and production yields were also observed in Gramineae species when grown in similar deficient conditions (Vlamis and Williams Reference Vlamis and Williams1967). Thus, soil concentrations of soluble Si play a fundamental role in plant uptake and fitness for these species.
Understanding the role of soil soluble Si in T. caput-medusae–invaded landscapes could help in improving and/or developing better control strategies. Similar to the aforementioned Si hyperaccumulators, Si-deficient soils may reduce T. caput-medusae fitness (Figure 1). Nevertheless, it is unknown how low soil values of soluble Si impact T. caput-medusae. If the soil soluble Si deficiency can reduce T. caput-medusae fitness, novel studies could develop control strategies that decrease available soil Si. Because soluble Si polymerizes at concentrations greater than 2 mM (Ma et al. Reference Ma, Miyake, Takahashi, Datnoff, Snyder and Korndörfer2001), future studies could help develop strategies that target reductions or increases in soil soluble Si concentrations, limiting uptake and consequently decreasing T. caput-medusae fitness.
The Root
Fitness of rice plants has been associated with the ability to take up soluble Si through the root (Takahashi et al. Reference Takahashi, Ma and Miyake1990) and uptake regulation by the Ls1 gene (Ma et al. Reference Ma, Mitani, Nagao, Konishi, Tamai, Iwashita and Yano2004). Modification of the active transport mechanisms in rice has led to increased plant production and disease resistance (Ma et al. Reference Ma, Tamai, Yamaji, Mitani, Konishi, Katsuhara, Ishiguro, Murata and Yano2006). If increased plant fitness can be achieved by identifying the uptake mechanism of soluble Si in rice, it is reasonable to conclude that identifying the transport mechanism in T. caput-medusae might enable modifications to be made to reduce T. caput-medusae fitness. Nevertheless, the practicality of applying these modifications to T. caput-medusae in highly variable ecological system will require considerable research before implementation.
Chemical application, rather than genetic modification, may provide a more viable means of interrupting the uptake mechanism. For instance, in a laboratory setting, the active transporter in rice was inhibited through the use of metabolic inhibitors such as NaCN and 2,4-dinitrophenol at low temperatures (Ma et al. Reference Ma, Mitani, Nagao, Konishi, Tamai, Iwashita and Yano2004). Although these chemicals would be hazardous if applied in a landscape-type setting, this research sets the stage for exploring environmentally friendly chemicals that could have similar beneficial results. While previous methods of restricting Si uptake rely on an active transport mechanism, if a passive transport mechanism exists, the addition of specific soil minerals may provide a form of competitive adsorption, reducing soluble Si uptake into the plant. However, identifying the uptake mechanism is critical before exploring soluble Si inhibition techniques.
When compared with roots of other grasses, the roots of T. caput-medusae were found to have thicker cell walls and overall larger root diameters than an annual grass, but values were lower than those of a perennial grass species (Harris Reference Harris1977). A thicker root endodermis may allow for transpiration to occur even if the surrounding soil environment is drier, particularly in the upper soil horizons; however (Harris Reference Harris1977), root Si concentrations were not reported. Evaluating root Si concentrations may give insight into why T. caput-medusae is superior in acquiring soil resources and tolerating soil environmental fluctuations (e.g., drought, temperature extremes). If root tissue Si plays a role in T. caput-medusae fitness, opportunities for research become available for discovering how deposition of soluble Si occurs in roots. This may lead to control strategies that manipulate soluble Si deposition processes within roots, decrease T. caput-medusae fitness, and reduce invasive superiority. Overall, little is known about T. caput-medusae roots in relation to Si uptake and tissue silica concentrations; thus, further examination is needed.
The Shoot
Little information is available about soluble Si transport and deposition as amorphous silica within tissues. Manipulation and reduction of Si deposition would be key to increased herbivore preference and digestibility and would make the plant texture less abrasive for herbivores. For instance, sublethal doses of a glyphosate-containing herbicide with a surfactant were shown to reduce tissue silica concentrations and increase tillering in quackgrass [Elymus repens (L.) Gould] (Coupland and Caseley Reference Coupland and Caseley1975). Similarly, low rates of glyphosate increased the nutritional quality of annual ryegrass (Lolium ridigum Gaudin) (Armstrong et al. Reference Armstrong, Simpson, Pearce and Radojevic1992; Gatford et al. Reference Gatford, Simpson, Siever-Kelly, Leury, Dove and Ciavarella1999) and increased cattle preference for glyphosate-treated fescue pastures (Kisseberth et al. Reference Kisseberth, Buck, Mansfield and Manuel1986). However, tissue Si concentrations were not reported in these last three studies. Understanding how glyphosate alters the soluble Si deposition process and increases nutritional composition may give insight into other chemical treatments with similar results.
Aspects of plant structure (e.g., toxicity resistance, photosynthetic barrier, culm rigidity, immune system response) are possibly linked to T. caput-medusae tissue Si, but these aspects need further study. For instance, toxic metal accumulation in plants may affect plant productivity (Adrees et al. Reference Adrees, Ali, Rizwan, Zia-ur-Rehman, Ibrahim, Abbas, Farid, Qayyum and Irshad2015; Nagajyoti et al. Reference Nagajyoti, Lee and Sreekanth2010), increased UV-B radiation could damage leaves and reduce the photosynthetic capacity of plants (Kakani et al. Reference Kakani, Reddy, Zhao and Sailaja2003), low tissue Si can increase susceptibility of culms to breakage in rice (Lee et al. Reference Lee, Kwon and Park1990; Savant et al. Reference Savant, Snyder, Datnoff and Sparks1996), and a weak immune system response to predation has detrimental consequences to fitness (Holub and Cooper Reference Holub and Cooper2004; Nuernberger and Lipka Reference Nuernberger and Lipka2005), whereas tissue Si mitigates these constraints. These principles and processes are known for crops; however, they are unexplored for T. caput-medusae. Elucidation of the influence of Si on T. caput-medusae fitness could lead to more efficient and/or novel control strategies for T. caput-medusae, interrupting its invasive superiority.
Conclusion
Taeniatherum caput-medusae is a superior invasive annual grass overtaking rangeland ecosystems and leading to drastic changes in ecosystem services. While control strategies exist for invasive annual grasses, these strategies do not take into consideration the role of high tissue Si in T. caput-medusae invasion. High tissue concentration of Si in T. caput-medusae creates a self-reinforcing feedback cycle that contributes to its invasive success and difficulty of control. The proposed conceptual model in this article summarizes T. caput-medusae characteristics associated with Si content (e.g., plant fitness, structure, and chemical composition) and the invasive processes associated with these characteristics (e.g., plant productivity, litter decomposition, and herbivory). These characteristics and processes help to identify critical research needs that can be used to develop improved and/or novel management practices of control, strengthening current strategies. Interrupting the Si positive feedback cycle of T. caput-medusae invasion should be considered when researching and developing future management strategies.
Acknowledgments
This research was funded by a Western SARE grant (SW15-003) in collaboration with Utah State University Department of Wildland Resources and USDA Agriculture Research Service, Logan, UT. No conflicts of interest have been declared.