1. Introduction
The origin of adakitic rocks, which are characterized by SiO2≥56 wt.%, Al2O3≥15 wt.%, Sr≥400 ppm, Sr/Y≥20, Y≤18 ppm and Yb≤1.9 ppm according to the definition of Defant & Drummond (Reference Defant and Drummond1990), is a matter of continuous discussion in the geoscientific community. Originally adakitic lavas, occurring in fore-arc environments, were interpreted as typical melting products of very young (<25 Ma) subducted oceanic crust (Defant & Drummond, Reference Defant and Drummond1990, Reference Defant and Drummond1993). However, in ‘cold’ subduction zones, relatively old oceanic crust usually follows P–T paths with low geothermal gradients of c. 5–7°Ckm−1, thereby preventing melting at shallow depths (e.g. Peacock, Rushmer & Thompson, Reference Peacock, Rushmer and Thompson1994; Peacock et al. Reference Peacock, van Keken, Holloway, Hacker, Abers and Ferguson2005; Kimura, Kasahara & Takeda, Reference Kimura, Kasahara and Takeda2009). Therefore, the presence of adakitic igneous rocks in several fore-arc environments above former ‘cold’ subduction zones (e.g. Nakamura & Iwamori, Reference Nakamura and Iwamori2013) led to several alternative tectonogenetic models such as the subduction of the leading edges of newly subducted slabs (Sajona et al. Reference Sajona, Maury, Bellon, Cotton, Defant, Pubellier and Rangin1993; Yogodzinski et al. Reference Yogodzinski, Lees, Churikova, Dorendorf, Woerner and Volynets2001) or slab-window margins (Thorkelson & Breitsprecher, Reference Thorkelson and Breitsprecher2005), slab tearing (Pallares et al. Reference Pallares, Maury, Bellon, Royer, Calmus, Aguillón-Robles, Cotton, Benoit, Michaud and Bourgois2007; Calmus et al. Reference Calmus, Pallares, Maury, Aguillón-Robles, Bellon, Benoit and Michaud2011), highly oblique convergence (Yogodzinski & Kelemen, Reference Yogodzinski and Kelemen1998), and flat subduction (Gutscher et al. Reference Gutscher, Maury, Eissen and Bourdon2000) to account for the melting of old oceanic crust beneath fore-arc regions. Furthermore, due to the occurrence of adakitic igneous rocks, which are not the result of slab melting, various geodynamic models have been suggested to explain their petrogenesis (see Castillo, Reference Castillo2006, Reference Castillo2012; Ribeiro, Maury & Grégoire, Reference Ribeiro, Maury and Grégoire2016): (1) melting of mafic, fertilized mantle-derived materials underplated at the base of lower crust (e.g. Atherton & Petford, Reference Atherton and Petford1993; Guo, Wilson & Liu, Reference Guo, Wilson and Liu2007; Zhao et al. Reference Zhao, Xiong, Wang, Wyman, Bao, Bai and Qiao2008); (2) high-pressure mineral fractionation of mantle-derived wet basaltic magmas (Castillo, Janney & Solidum, Reference Castillo, Janney and Solidum1999; Garrison & Davidson, Reference Garrison and Davidson2003; MacPherson, Dreher & Thirlwall, Reference Macpherson, Dreher and Thirlwall2006; Rodriguez et al. Reference Rodríguez, Sellés, Dungan, Langmuir and Leeman2007; Petrone & Ferrari, Reference Petrone and Ferrari2008; Gao et al. Reference Gao, Klemd, Long, Xiong and Qian2009); and (3) mixing and mingling of evolved melts with mantle melts in shallower crustal storage regions, periodically refluxed by mantle melts (e.g. Castillo, Janney & Solidum, Reference Castillo, Janney and Solidum1999; Ribeiro, Maury & Grégoire, Reference Ribeiro, Maury and Grégoire2016).
Mesozoic–Cenozoic magmatic rocks are widespread in the southern Lhasa sub-terrane (Fig. 1; Schärer, Xu & Allegre, Reference Schärer, Xu and Allegre1984; Coulon et al. Reference Coulon, Maluski, Bollinger and Wang1986; Chung et al. Reference Chung, Liu, Ji, Chu, Lee, Wen, Lo, Lee, Qian and Zhang2003; Mo et al. Reference Mo, Dong, Zhao, Guo, Wang and Chen2005; Chu et al. Reference Chu, Chung, Song, Liu, O'Reilly, Pearson, Ji and Wen2006; Wen et al. Reference Wen, Chung, Song, Iizuka, Yang, Ji, Liu and Gallet2008a , Reference Wen, Liu, Chung, Chu, Ji, Zhang, Song, Lee, Yeh and Lo b ; Ji et al. Reference Ji, Wu, Chung, Li and Liu2009a , Reference Ji, Wu, Liu and Chung b , Reference Ji, Wu, Liu and Chung2012; Zhu et al. Reference Zhu, Zhao, Pan, Lee, Kang, Liao, Wang, Li, Dong and Liu2009, Reference Zhu, Zhao, Niu, Mo, Chung and Hou2011a , Reference Zhu, Wang, Zhao, Chung, Cawood, Niu, Liu, Wu and Mo2015; Mo, Reference Mo2011; Ma et al. Reference Ma, Wang, Wyman, Li, Jiang, Yang, Gou and Guo2013, Reference Ma, Wang, Jiang, Wang, Li, Wyman, Zhao, Yang, Gou and Guo2014; Qiu et al. Reference Qiu, Wang, Zhao and Yu2015). Among them, Cretaceous–Miocene (137–10 Ma) adakitic rocks have been recognized and intensively investigated (e.g. Chung et al. Reference Chung, Liu, Ji, Chu, Lee, Wen, Lo, Lee, Qian and Zhang2003; Hou et al. Reference Hou, Gao, Qu, Rui and Mo2004; Gao et al. Reference Gao, Hou, Kamber, Wei, Meng and Zhao2007, Reference Gao, Yang, Santosh, Hou, Wei and Tian2010; Guo, Wilson & Liu, Reference Guo, Wilson and Liu2007; Wen et al. Reference Wen, Chung, Song, Iizuka, Yang, Ji, Liu and Gallet2008a ; Zhu et al. Reference Zhu, Zhao, Pan, Lee, Kang, Liao, Wang, Li, Dong and Liu2009; Guan et al. Reference Guan, Zhu, Zhao, Zhang, Liu, Li, Yu, Liu and Mo2010, Reference Guan, Zhu, Zhao, Dong, Zhang, Li, Liu, Mo, Liu and Yuan2012; Zhang et al. Reference Zhang, Zhao, Santosh, Wang, Dong and Shen2010; Chen et al. Reference Chen, Xu, Zhao, Dong, Wang and Kang2011; Ji et al. Reference Ji, Wu, Liu and Chung2012; Jiang et al. Reference Jiang, Wang, Li, Wyman, Tang, Jia and Yang2012; Zheng et al. Reference Zheng, Hou, Li, Sun, Liang, Fu, Li and Huang2012; Ma et al. Reference Ma, Wang, Wyman, Li, Jiang, Yang, Gou and Guo2013, Reference Ma, Wang, Jiang, Wang, Li, Wyman, Zhao, Yang, Gou and Guo2014; Meng et al. Reference Meng, Zhao, Zhu, Mo, Guan, Huang, Dong, Zhou, DePaolo, Harrison, Zhang, Liu, Liu, Hu and Yuan2014; Xu et al. Reference Xu, Zhang, Luo, Guo and Yang2015). The Cretaceous adakitic rocks in the southern Lhasa sub-terrane are believed to be a result of partial melting of the subducted Neo-Tethyan oceanic plate or of partial melting of thickened lower crust in response to the subduction of the Neo-Tethyan oceanic plate (Wen et al. Reference Wen, Chung, Song, Iizuka, Yang, Ji, Liu and Gallet2008a ; Zhu et al. Reference Zhu, Zhao, Pan, Lee, Kang, Liao, Wang, Li, Dong and Liu2009; Guan et al. Reference Guan, Zhu, Zhao, Zhang, Liu, Li, Yu, Liu and Mo2010; Zhang et al. Reference Zhang, Zhao, Santosh, Wang, Dong and Shen2010; Jiang et al. Reference Jiang, Wang, Li, Wyman, Tang, Jia and Yang2012; Ma et al. Reference Ma, Wang, Wyman, Li, Jiang, Yang, Gou and Guo2013; Xu et al. Reference Xu, Zhang, Luo, Guo and Yang2015). The Cenozoic adakitic rocks were suggested to have formed by partial melting of thickened lower mafic crust, subducted oceanic crust or metasomatized mantle during the India–Asia collision (Chung et al. Reference Chung, Liu, Ji, Chu, Lee, Wen, Lo, Lee, Qian and Zhang2003; Hou et al. Reference Hou, Gao, Qu, Rui and Mo2004; Gao et al. Reference Gao, Hou, Kamber, Wei, Meng and Zhao2007, Reference Gao, Yang, Santosh, Hou, Wei and Tian2010; Guo, Wilson & Liu, Reference Guo, Wilson and Liu2007; Guan et al. Reference Guan, Zhu, Zhao, Dong, Zhang, Li, Liu, Mo, Liu and Yuan2012; Ji et al. Reference Ji, Wu, Liu and Chung2012; Ma et al. Reference Ma, Wang, Jiang, Wang, Li, Wyman, Zhao, Yang, Gou and Guo2014; Meng et al. Reference Meng, Zhao, Zhu, Mo, Guan, Huang, Dong, Zhou, DePaolo, Harrison, Zhang, Liu, Liu, Hu and Yuan2014). Typically, the Cenozoic adakitic rocks were thought to indicate the timing of the crustal thickening of the Tibetan Plateau as well as the subduction of the Indian continent beneath the Lhasa terrane (Chung et al. Reference Chung, Liu, Ji, Chu, Lee, Wen, Lo, Lee, Qian and Zhang2003; Hou et al. Reference Hou, Gao, Qu, Rui and Mo2004; Guo, Wilson & Liu, Reference Guo, Wilson and Liu2007; Guan et al. Reference Guan, Zhu, Zhao, Dong, Zhang, Li, Liu, Mo, Liu and Yuan2012; Ji et al. Reference Ji, Wu, Liu and Chung2012; Ma et al. Reference Ma, Wang, Jiang, Wang, Li, Wyman, Zhao, Yang, Gou and Guo2014; Meng et al. Reference Meng, Zhao, Zhu, Mo, Guan, Huang, Dong, Zhou, DePaolo, Harrison, Zhang, Liu, Liu, Hu and Yuan2014). In contrast, the presence of Early Jurassic adakitic rocks is poorly documented in the southern Lhasa sub-terrane.

Figure 1. (a) Tectonic framework of south Tibet, showing major tectonic subdivisions (modified from Zhu et al. Reference Zhu, Zhao, Niu, Mo, Chung and Hou2011a ). (b) Geological map of the Gangdese belt (modified from Mo et al. Reference Mo, Dong, Zhao, Yu, Shen, Zhu, Ke, Zhou, Wang and Wang2013), showing the distributions and ages of Late Triassic–Early Jurassic intrusive rocks (age data are from Chu et al. Reference Chu, Chung, Song, Liu, O'Reilly, Pearson, Ji and Wen2006; Liu et al. Reference Liu, Jiang, Jian, Ye, Wu and Hu2006; Qu, Xin & Xu, Reference Qu, Xin and Xu2007; Zhang et al. Reference Zhang, Xu, Guo, Zong, Cai and Yuan2007a , Reference Zhang, Xu, Guo, Zong, Cai and Yuan b ; Ji et al. Reference Ji, Wu, Chung, Li and Liu2009a , Reference Ji, Wu, Liu and Chung b ; Yang et al. Reference Yang, Hou, Jiang, Zhang and Song2011; Zhu et al. Reference Zhu, Zhao, Niu, Mo, Chung and Hou2011a ; Dong & Zhang, Reference Dong and Zhang2013; Guo et al. Reference Guo, Liu, Liu, Cawood, Wang and Liu2013; Qiu et al. Reference Qiu, Wang, Zhao and Yu2015; Meng et al. Reference Meng, Dong, Cong, Xu and Cao2016a , Reference Meng, Xu, Santosh, Ma, Chen, Guo and Liu b ; Shui et al. Reference Shui, He, Zhang and Lu2016; Ma et al. Reference Ma, Meng, Xu and Liu2017). Abbreviations: JSSZ = Jinshajiang Suture Zone; BNSZ = Bangong–Nujiang Suture Zone; SNMZ = Shiquan River–Nam Tso Mélange Zone; LMF = Luobadui–Milashan Fault; IYZSZ = Indus–Yarlung Zangbo Suture Zone.
Furthermore, a long-standing controversy exists as to whether the Late Triassic–Early Jurassic igneous rocks are the products of partial melting of underplated mafic lower crust or of ancient continental crust (Chu et al. Reference Chu, Chung, Song, Liu, O'Reilly, Pearson, Ji and Wen2006; Zhang et al. Reference Zhang, Xu, Guo, Zong, Cai and Yuan2007a , Reference Zhang, Xu, Guo, Zong, Cai and Yuan b ; Yang et al. Reference Yang, Hou, Jiang, Zhang and Song2011; Zhu et al. Reference Zhu, Zhao, Niu, Mo, Chung and Hou2011a ; Dong & Zhang, Reference Dong and Zhang2013; Guo et al. Reference Guo, Liu, Liu, Cawood, Wang and Liu2013; Meng et al. Reference Meng, Dong, Cong, Xu and Cao2016a , Reference Meng, Xu, Santosh, Ma, Chen, Guo and Liu b ; Shui et al. Reference Shui, He, Zhang and Lu2016; Ma et al. Reference Ma, Meng, Xu and Liu2017). Therefore, the study of the largely neglected Early Jurassic adakitic rocks will provide important information on their petrogenesis and tectonic setting in the southern Lhasa sub-terrane.
In this paper, we present petrological, geochemical, zircon U–Pb and Hf isotopic data of the Early Jurassic adakitic rocks and associated mafic enclaves from the Jiacha area in order to constrain their petrogenesis and discuss the Early Jurassic tectonic evolution of the southern Lhasa sub-terrane.
2. Geological background and petrography
The Lhasa terrane is bounded by the Bangong–Nujiang suture zone to the north and the Indus–Yarlung Tsangpo suture zone to the south, representing Meso- and Neo-Tethys ocean relics, respectively (Fig. 1a; Yin & Harrison, Reference Yin and Harrison2000). It is generally divided into southern, central and northern sub-terranes, which are separated by the Luobadui–Milashan Fault (LMF) and the Shiquanhe–Nam Tso Mélange Zone (SNMZ) (Fig. 1a; Zhu et al. Reference Zhu, Zhao, Niu, Mo, Chung and Hou2011a ). The Bangong–Nujiang ocean (Meso-Tethys) is believed to have subducted southward beneath the Lhasa terrane during the Permian to the Early Cretaceous (e.g. Pan et al. Reference Pan, Ding, Yao and Wang2004; Qiu et al. Reference Qiu, Zhou, Deng, Li, Xiao and Cai2004; Zhu et al. Reference Zhu, Zhao, Niu, Mo, Chung and Hou2011a ). However, Kapp et al. (Reference Kapp, Murphy, Yin, Harrison, Ding and Guo2003, Reference Kapp, DeCelles, Gehrels, Heizler and Ding2007) suggested a northward subduction beneath the Qiangtang terrane in the Late Jurassic.
The southern Lhasa sub-terrane mainly consists of juvenile crust with Precambrian basement slivers (e.g. Mo, Reference Mo2011; Ji et al. Reference Ji, Wu, Chung, Li and Liu2009a ; Zhu et al. Reference Zhu, Zhao, Niu, Mo, Chung and Hou2011a , Reference Zhu, Zhao, Niu, Dilek and Mo b ). This sub-terrane comprises the Gangdese belt, which extends ~2500 km from east to west (Fig. 1b; Mo et al. Reference Mo, Dong, Zhao, Guo, Wang and Chen2005; Chu et al. Reference Chu, Chung, Song, Liu, O'Reilly, Pearson, Ji and Wen2006; Ji et al. Reference Ji, Wu, Chung, Li and Liu2009a , Reference Ji, Wu, Liu and Chung b ; Mo, Reference Mo2011). It consists of large-scale intrusive complexes and widespread volcanic rocks, which were mainly generated between 220 Ma and 13 Ma (Fig. 1a; Yin & Harrison, Reference Yin and Harrison2000; Chung et al. Reference Chung, Liu, Ji, Chu, Lee, Wen, Lo, Lee, Qian and Zhang2003; Mo et al. Reference Mo, Dong, Zhao, Guo, Wang and Chen2005, Reference Mo2011; Chu et al. Reference Chu, Chung, Song, Liu, O'Reilly, Pearson, Ji and Wen2006; Wen et al. Reference Wen, Chung, Song, Iizuka, Yang, Ji, Liu and Gallet2008a , Reference Wen, Liu, Chung, Chu, Ji, Zhang, Song, Lee, Yeh and Lo b ; Zhu et al. Reference Zhu, Pan, Chung, Liao, Wang and Li2008, Reference Zhu, Zhao, Pan, Lee, Kang, Liao, Wang, Li, Dong and Liu2009, Reference Zhu, Zhao, Niu, Mo, Chung and Hou2011a ; Ji et al. Reference Ji, Wu, Chung, Li and Liu2009a , Reference Ji, Wu, Liu and Chung b , Reference Ji, Wu, Liu and Chung2012; Kang et al. Reference Kang, Xu, Wilde, Feng, Chen, Wang, Fu and Pan2014; Meng et al. Reference Meng, Dong, Cong, Xu and Cao2016a , Reference Meng, Xu, Santosh, Ma, Chen, Guo and Liu b ). These igneous rocks show two age peaks of ~109–80 Ma and ~65–41 Ma (Ji et al. Reference Ji, Wu, Chung, Li and Liu2009a , Reference Ji, Wu, Liu and Chung b ; Zhu et al. Reference Zhu, Zhao, Niu, Mo, Chung and Hou2011a ). The Late Triassic –Early Jurassic intrusions (215–182 Ma) mainly consist of intermediate-silicic rocks (Chu et al. Reference Chu, Chung, Song, Liu, O'Reilly, Pearson, Ji and Wen2006; Liu et al. Reference Liu, Jiang, Jian, Ye, Wu and Hu2006; Qu, Xin & Xu, Reference Qu, Xin and Xu2007; Zhang et al. Reference Zhang, Xu, Guo, Zong, Cai and Yuan2007a , Reference Zhang, Xu, Guo, Zong, Cai and Yuan b ; Ji et al. Reference Ji, Wu, Chung, Li and Liu2009a , Reference Ji, Wu, Liu and Chung b ; Zhu et al. Reference Zhu, Zhao, Niu, Mo, Chung and Hou2011a ; Dong & Zhang, Reference Dong and Zhang2013; Guo et al. Reference Guo, Liu, Liu, Cawood, Wang and Liu2013; ; Meng et al. Reference Meng, Dong, Cong, Xu and Cao2016a , Reference Meng, Xu, Santosh, Ma, Chen, Guo and Liu b ; Ma et al. Reference Ma, Meng, Xu and Liu2017), with coeval volcanic rocks of the Sangri Group (Kang et al. Reference Kang, Xu, Wilde, Feng, Chen, Wang, Fu and Pan2014) and the Yeba Formation (Zhu et al. Reference Zhu, Pan, Chung, Liao, Wang and Li2008; Wei, Reference Wei2014).
The studied Early Jurassic quartz diorite and tonalite rocks were collected in the Jiacha area in the eastern part of the Gangdese belt (Fig. 2). Middle Carboniferous, Late Cretaceous and Palaeogene granitoids are also exposed in this area (Fig. 2; Ji et al. Reference Ji, Wu, Liu and Chung2009b , Reference Ji, Wu, Liu and Chung2012; Dong & Zhang, Reference Dong and Zhang2013). The quartz diorite has an exposure area of c. 60 km2 and is distinctly elongated in an approximately E–W direction (Fig. 2). The subspherical to irregular mafic enclaves (5–35 cm in diameter) are randomly distributed in the quartz diorite (Fig. 3a, b). They have sharp to gradational contacts with the host quartz diorite. Plagioclase phenocrysts cross-cut the interface between the mafic enclaves and the host quartz diorite (Fig. 3b). The tonalites were collected from three different intrusive bodies, which cross-cut the quartz diorite in places (Figs 2, 3c, d). A summary of the sample locations and the petrographical features is given in Table 1.

Figure 2. Geological map of the Jiacha area, eastern Gangdese belt.

Figure 3. Field photos of the Jiacha Early Jurassic adakitic intrusions. (a) The quartz diorite contains elongated mafic enclaves. (b) Ellipsoidal, spindle-shaped mafic enclaves in the host quartz diorite. Note that some crystals cross-cut the boundary between host rock and enclave. Plagioclase phenocrysts are indicated with red arrows. (c) The tonalite intrudes the quartz diorite. (d) The tonalite outcrop lies in the Xiangmucun area, east of Jiacha.
Table 1. Ages, lithologies and major mineral assemblages of the Jiacha Early Jurassic adakitic rocks.

Abbreviations: Amp =amphibole; Bi = biotite; Kfs = K-feldspar; Pl = plagioclase; Qtz = quartz.
The quartz diorite, which displays a weak mylonitic foliation in places, is medium-grained and mainly consists of plagioclase (55–60 vol.%), amphibole (10–15 vol.%), quartz (~15 vol.%), biotite (~10 vol.%), minor K-feldspar (~2 vol.%) and accessory magnetite, titanite, apatite and zircon (Fig. 4a). Andesitic plagioclase is 1.0–3.0 mm in length (An=32–50; Supplementary Table S1, available at https://doi.org/10.1017/S0016756817000577) (Fig. 4a). The euhedral to subhedral amphibole is commonly interstitial, sometimes poikilitic, enclosing plagioclase and oxides, and partly altered to chlorite and epidote (Fig. 4a). The mafic enclaves contain 2–3 mm long plagioclase phenocrysts (An=39–68) in a matrix of fine- to medium-grained plagioclase (40–55 vol.%), amphibole (20–30 vol.%), biotite (~5 vol.%) and quartz (~5 vol.%) with minor K-feldspar and needle-like apatite (Fig. 4b, c).

Figure 4. Photomicrographs of the Jiacha Early Jurassic adakitic intrusions. (a) The quartz diorite consists mainly of plagioclase, amphibole and quartz. (b) The mafic enclave of quartz diorite consists of plagioclase phenocrysts and fine-grained plagioclase, amphibole and biotite. (c) Amphibole from mafic enclave contains plagioclase, quartz and biotite inclusions; also showing the needle-like apatite. (d) The tonalite mainly consists of plagioclase and quartz (GD15-L08-1). (e) The tonalite mainly consists of plagioclase, quartz, biotite, amphibole and minor K-feldspar (GD14-50-1). (f) The tonalite from Xiangmucun mainly consists of plagioclase, quartz, minor K-feldspar, biotite and some amphibole (GD14-102-1). (a, b, d, e, f) Crossed polarized light. (c) Plane polarized light. Abbreviations: Amp = amphibole; Pl = plagioclase; Bi = biotite; Ap = apatite; Qtz = quartz.
The small tonalite body intruding the quartz diorite (Fig. 2) is fine-grained and mainly consists of plagioclase (~60 vol.%), quartz (~30 vol.%), biotite (~3%) and minor K-feldspar (Fig. 4d). Plagioclase exhibits typical oscillatory zoning (Fig. 4d). Samples GD14-50-1 and GD14-50-2 are collected from a tonalite body near Sangri, to the west of the Jiacha area (Figs 1, 2). They are medium-grained, weakly mylonitized and mainly consist of plagioclase (55–60 vol.%), quartz (25–30 vol.%), biotite (~5 vol.%), amphibole (~5 vol.%) and minor K-feldspar (Fig. 4e). The tonalite body near Xiangmucun is medium-grained and consists of plagioclase (An = 30–50; 50–60 vol.%), quartz (20–25 vol.%), biotite (3–10 vol.%) and minor amphibole and K-feldspar, with accessory zircon and apatite (Figs 2, 3d, 4f).
3. Analytical methods
The chemical composition of plagioclase was determined using a JEOL JXA 8800R microprobe with a 20 kV accelerating voltage and a 20 nA beam current at the Institute of Mineral Resources, Chinese Academy of Geological Sciences (CAGS), Beijing. The representative microprobe analytical results are listed in Supplementary Table S1.
Zircon grains were extracted using standard density and magnetic separation techniques. Cathodoluminescence (CL) images of analysed zircon grains were obtained using an FEI NOVA NanoSEM 450 scanning electron microscope equipped with a Matan Mono CL4 cathodoluminescence system at the Institute of Geology, CAGS. Zircon U–Pb dating was carried out using a laser ablation inductively coupled plasma mass spectrometer (LA-ICP-MS; Perkin Elmer Elan DRC II) equipped with a Microlas system (GeoLas 200 M, 193 nm ArFexcimer laser) at the Key Laboratory of Crust–Mantle Materials and Environments of CAS at the University of Science and Technology of China.
Zircon 91500 and SRM610 were used as external standards for the U–Pb isotope ratios and the trace element analysis, respectively. Details of the instrument parameters and analysis procedures are given in Liu et al. (Reference Liu, Gao, Diwu, Yuan and Hu2007). The spot diameter of the laser ablation pits is 32 μm. Details of the instrument parameters and analysis procedures are given in Liu et al. (Reference Liu, Gao, Diwu, Yuan and Hu2007) and Gu et al. (Reference Gu, Xiao, Santosh, Li, Yang, Pack and Hou2013). The LaDating (version 1.5) software was used for processing the U/Pb data. The quantitative calibration for the Pb isotope dating was performed by ComPbcorr#3_18 (Andersen, Reference Andersen2002). The age calculations and concordia diagrams (data-point error ellipses are 68.3% conf.) were performed using Isoplot/Ex_ver3 (Ludwig, Reference Ludwig2003).
Zircon Lu–Hf isotopic analyses were carried out in situ using a Neptune Plus multi-collector inductively coupled plasma mass spectrometer (MC-ICP-MS) in combination with a Geolas 2005 excimer ArF laser ablation system at the State Key Laboratory of Geological Processes and Mineral Resources, China University of Geosciences (Wuhan). The energy density of the laser ablation was 5.3 J cm−2 and the ablation spot was 44 μm in diameter. Zircons 91500, GJ-1, Mud Tank and Temora were analysed as reference standards. Detailed operating conditions for the laser ablation system and the MC-ICP-MS instrument and analytical procedures are similar to those described by Hu et al. (Reference Hu, Liu, Gao, Liu, Zhang, Tong, Lin, Zong, Li, Chen, Zhou and Yang2012). Off-line selection and integration of analysis signals, and mass bias calibrations were performed using ICPMSDataCal (Liu et al. Reference Liu, Gao, Hu, Gao, Zong and Wang2010). The measured 176Lu/177Hf ratios and the 176Lu decay constant of 1.867×10−11a−1 (Söderlund et al. Reference Söderlund, Patchett, Vervoort and Isachsen2004) were used to calculate initial 176Hf/177Hf ratios. The Chondritic values of 176Lu/177Hf=0.0336 and 176Hf/177Hf=0.282785 reported by Bouvier, Vervoort & Patchett, (Reference Bouvier, Vervoort and Patchett2008) were used to calculate the εHf values. The depleted mantle Hf model ages (T DM) were calculated using the measured 176Lu/177Hf ratios based on the assumption that the depleted mantle reservoir has a linear isotopic growth from 176Hf/177Hf=0.279718 at 4.55 Ma to 0.283250 at present, with 176Lu/177Hf=0.0384 (Griffin et al. Reference Griffin, Pearson, Belousova, Jackson, van Achterbergh, O'Reilly and Shee2000). Two-stage model ages (T DM2) were calculated by assuming that the parental magma was produced from an average continental crust (176Lu/177Hf=0.015) (Griffin et al. Reference Griffin, Wang, Jackson, Pearson, O'Reilly, Xu and Zhou2002).
The whole-rock major- and trace-element compositions were analysed at the National Research Centre for Geoanalysis, CAGS. The whole-rock major elements were performed using X-ray fluorescence (XRF, PW4400) with an analytical uncertainty of <2%. Trace-element abundances were measured by inductively coupled plasma mass spectrometry (ICP-MS, PE300D), which gives a precision better than 10% for most of the elements analysed.
4. Results
4.a. Whole-rock major and trace elements
Whole-rock major and trace elements of the Jiacha Early Jurassic igneous rocks are listed in Supplementary Table S2. All samples are sub-alkaline in the total alkali–silica (TAS) diagram (Fig. 5a). The quartz diorite samples have variable SiO2 (58–63 wt.%) and high Al2O3 (>16%), MgO (2.63–3.46 wt.%), Na2O/K2O (>2) and Mg# (48–54) values. They are metaluminous with A/CNK [molar Al2O3/(CaO+Na2O+K2O)] ratios of 0.87–0.93 and plot in the medium-K calc-alkaline series field on the K2O vs SiO2 diagram (Fig. 5b, c). In the PRIMA-normalized spidergram (Fig. 6a) these rocks show a strong enrichment of large-ion lithophile elements (LILEs) and pronounced negative Nb, Ta and Ti anomalies. Furthermore, they display slightly negative Eu anomalies (Eu/Eu*=0.83–0.92) and have inclined chondrite-normalized rare earth element (REE) patterns (Fig. 6b), while (La/Yb)N ranges from 3.90 to 7.93. In addition, they have relatively low heavy rare earth element (HREE) (Yb=1.09–1.74 ppm) and Y concentrations (10.4–15.9 ppm), as well as high Sr contents (412–504 ppm) and Sr/Y ratios (29.2–45.1). Therefore these rocks have an adakitic character according to the definition of Defant & Drummond (Reference Defant and Drummond1990, Reference Defant and Drummond1993), which is also revealed by using the Sr/Y vs Y discrimination diagram (Fig. 5d).

Figure 5. (a) Total alkalis vs silica diagram (after Middlemost, Reference Middlemost1994); (b) K2O vs SiO2 diagram (after Rollinson, Reference Rollinson1993); (c) A/NK vs A/CNK diagram (after Maniar & Piccoli, Reference Maniar and Piccoli1989); (d) Sr/Y vs Y discrimination diagram showing data for adakites and island-arc andesite–dacite–rhyolite rocks (after Defant & Drummond, Reference Defant and Drummond1990, Reference Defant and Drummond1993). Data sources: Late Cretaceous–Miocene (83–10 Ma) lower crust-derived adakites in the Lhasa terrane (Chung et al. Reference Chung, Liu, Ji, Chu, Lee, Wen, Lo, Lee, Qian and Zhang2003; Hou et al. Reference Hou, Gao, Qu, Rui and Mo2004; Gao et al. Reference Gao, Hou, Kamber, Wei, Meng and Zhao2007, Reference Gao, Yang, Santosh, Hou, Wei and Tian2010; Guo, Wilson & Liu, Reference Guo, Wilson and Liu2007; Wen et al. Reference Wen, Chung, Song, Iizuka, Yang, Ji, Liu and Gallet2008a ; Guan et al. Reference Guan, Zhu, Zhao, Dong, Zhang, Li, Liu, Mo, Liu and Yuan2012; Ma et al. Reference Ma, Wang, Jiang, Wang, Li, Wyman, Zhao, Yang, Gou and Guo2014; Meng et al. Reference Meng, Zhao, Zhu, Mo, Guan, Huang, Dong, Zhou, DePaolo, Harrison, Zhang, Liu, Liu, Hu and Yuan2014). Cretaceous (137–86 Ma) subducted oceanic crust-derived adakites in the Lhasa terrane (Zhu et al. Reference Zhu, Zhao, Pan, Lee, Kang, Liao, Wang, Li, Dong and Liu2009; Guan et al. Reference Guan, Zhu, Zhao, Zhang, Liu, Li, Yu, Liu and Mo2010; Zhang et al. Reference Zhang, Zhao, Santosh, Wang, Dong and Shen2010; Jiang et al. Reference Jiang, Wang, Li, Wyman, Tang, Jia and Yang2012; Ma et al. Reference Ma, Wang, Wyman, Li, Jiang, Yang, Gou and Guo2013).

Figure 6. Spider diagrams (a, c) (Sun & McDonough, Reference Sun, McDonough, Saunders and Norry1989) and chondrite-normalized REE pattern (b, d) for the Jiacha Early Jurassic adakitic intrusions (Taylor & McLennan, Reference Taylor and McLennan1985). Adakite fields are shown for comparison. Data sources: Late Cretaceous–Miocene (83–10 Ma) lower crust-derived adakites in the Lhasa terrane (Gao et al. Reference Gao, Hou, Kamber, Wei, Meng and Zhao2007; Wen et al. Reference Wen, Chung, Song, Iizuka, Yang, Ji, Liu and Gallet2008a ; Guan et al. Reference Guan, Zhu, Zhao, Dong, Zhang, Li, Liu, Mo, Liu and Yuan2012; Zheng et al. Reference Zheng, Hou, Li, Sun, Liang, Fu, Li and Huang2012; Ma et al. Reference Ma, Wang, Jiang, Wang, Li, Wyman, Zhao, Yang, Gou and Guo2014; Meng et al. Reference Meng, Zhao, Zhu, Mo, Guan, Huang, Dong, Zhou, DePaolo, Harrison, Zhang, Liu, Liu, Hu and Yuan2014); Cretaceous (137–86 Ma) subducted oceanic crust-derived adakites in the Lhasa terrane (Zhu et al. Reference Zhu, Zhao, Pan, Lee, Kang, Liao, Wang, Li, Dong and Liu2009; Zhang et al. Reference Zhang, Zhao, Santosh, Wang, Dong and Shen2010; Jiang et al. Reference Jiang, Wang, Li, Wyman, Tang, Jia and Yang2012; Ma et al. Reference Ma, Wang, Wyman, Li, Jiang, Yang, Gou and Guo2013).
The mafic enclaves in the quartz diorites have SiO2 contents of 52–53 wt.%, MgO contents of 4.82–5.72 wt.% and an Mg# of 51–58. In the PRIMA-normalized spidergram (Fig. 6a), they exhibit a relative enrichment in LILEs (e.g. Rb, K and Pb). Their REE patterns are mildly fractionated with (La/Yb)N of 3.3–3.7 and slightly negative Eu anomalies (Eu/Eu*=0.81–0.90) in the chondrite-normalized spidergram (Fig. 6b). The REE concentrations are rather high (Yb=2.56–2.62 ppm and Y=21.5–28.0 ppm) compared to those of the host quartz diorites, and plot in the island-arc field on the Sr/Y vs Y discrimination diagram (Fig. 5d).
The tonalite samples have high SiO2 (64–70 wt.%) and total alkali (K2O+Na2O=5.04–6.07 wt.%) concentrations and low MgO (0.93–2.25 wt.%) and P2O5 (0.07–0.15 wt.%) contents compared to those of the quartz diorite samples. According to the K2O vs SiO2 diagram, the samples belong to the medium-K series (Fig. 5b) and are metaluminous to slightly peraluminous, with A/CNK ratios of 0.93–1.08 (Fig. 5c). The samples show strong enrichments of LILEs and pronounced negative Nb–Ta–Ti anomalies in the PRIMA-normalized spidergrams (Fig. 6c). They further display fractionated REE patterns with negative to positive Eu anomalies (Eu/Eu*=0.84–1.43) in the chondrite-normalized spidergram (Fig. 6d). The Sr contents (363–530 ppm), the Sr/Y ratios (27.3–105) as well as the Y (4.46–13.3 ppm) and Yb (95–1.39 ppm) contents are in accordance with the adakite definition as also revealed by the Sr/Y vs Y discrimination diagram (Fig. 5d).
4.b. Zircon U–Pb geochronology
To constrain the timing of the magmatic crystallization events, six samples were selected for LA-ICP-MS zircon U–Pb dating. The results are summarized in Table 1 and the data are given in Supplementary Table S3. Zircon grains from the quartz diorite and tonalite samples are euhedral, with crystal lengths of 100–250 μm. They exhibit oscillatory zoning in CL images (Fig. 7a–d), which are typical of magmatic zircon (Hoskin & Schaltegger, Reference Hoskin and Schaltegger2003). Zircon grains from the mafic enclaves are relatively small (50–100 μm in length) and display broad oscillatory zoning in the CL images (Fig. 7e, f).

Figure 7. CL images of zircon grains of the Jiacha Early Jurassic adakitic intrusions. Solid and dashed circles indicate the locations of U–Pb dating and Hf isotope analyses, respectively.
Twenty-two analyses on 22 zircon grains from quartz diorite sample GD14-89-1 yield a weighted mean 206Pb/238U age of 198±4 Ma (MSWD=1.5). A further 32 analyses were undertaken on 32 zircon grains from another quartz diorite sample GD14-93-1, which tightly plot on the concordia (Fig. 6b) and give a weighted mean 206Pb/238U age of 199±4 Ma (MSWD=2.0) (Fig. 8a, b). All analyses show variable Th (41–253 ppm) and U (95–365 ppm) contents with Th/U ratios of 0.37–0.86 that are typical for magmatic zircon (Supplementary Table S3).

Figure 8. U–Pb concordia diagrams of the Jiacha Early Jurassic adakitic intrusions.
Twenty-seven spots on 17 zircon grains yield a weighted mean 206Pb/238U age of 198±3 Ma (MSWD=0.8) for the mafic enclave sample GD14-90-3, and 18 spot analyses on 18 zircon grains from sample GD15-L09-2 give a weighted mean 206Pb/238U age of 196±3 Ma (MSWD=2.4), both of which are, within error, identical and are interpreted to represent the crystallization age of the mafic enclave (Fig. 8c, d). All analyses have Th=40–376 ppm and U=76–356 ppm with high Th/U ratios of 0.47–1.06 in accordance with magmatic zircon (Supplementary Table S3).
Seventeen U–Pb spots on 17 zircon grains from tonalite sample GD15-L08-1 and 14 U-Pb spots on 14 zircon grains from tonalite sample GD14-50-1 yield weighted mean 206Pb/238U ages of 198±2 Ma (MSWD=2.6) and 179±2 Ma (MSWD=1.2), respectively (Fig. 8e, f), which are interpreted as the crystallization ages of the tonalites. All analyses have variable Th (38–587 ppm) and U (82–562 ppm) contents and high Th/U ratios of 0.42–1.04 in accordance with magmatic zircon (Supplementary Table S3).
A previous study showed that the Xiangmucun tonalite body has a crystallization age of 184±4 Ma (Table 1; Shui et al. Reference Shui, He, Zhang and Lu2016). Therefore, the adakitic quartz diorites and tonalites from the Jiacha area of the southern Lhasa sub-terrane were formed in the Early Jurassic (202–179 Ma).
4.c. Zircon Lu–Hf isotope compositions
Zircon grains from two quartz diorite samples (GD14-89-1 and GD14-93-1), two tonalite samples (GD15-L08-1 and GD14-50-1) from different intrusive bodies (Fig. 2) and one mafic enclave sample (GD14-90-3) were analysed for their Lu–Hf isotope compositions. All zircon Hf isotopic spot analyses were performed on the same zircon grains, which were used for the U–Pb dating. The results are given in Supplementary Table S4 and illustrated in Figures 9 and 10.

Figure 9. Histograms of zircon ε Hf(t) values of the Jiacha Early Jurassic adakitic intrusions. The data of samples GD14-99-2 and GD14-102-1 are from Shui et al. (Reference Shui, He, Zhang and Lu2016).

Figure 10. Plot of zircon ε Hf(t) values vs U–Pb ages of the Jiacha Early Jurassic adakitic intrusions. Data sources: Granitoids in southern Lhasa terrane (Zhang et al. Reference Zhang, Xu, Guo, Zong, Cai and Yuan2007a ; Ji et al. Reference Ji, Wu, Chung, Li and Liu2009a , Reference Ji, Wu, Liu and Chung b ; Yang et al. Reference Yang, Hou, Jiang, Zhang and Song2011; Zhu et al. Reference Zhu, Zhao, Niu, Mo, Chung and Hou2011a ; Dong & Zhang, Reference Dong and Zhang2013; Guo et al. Reference Guo, Liu, Liu, Cawood, Wang and Liu2013; Meng et al. Reference Meng, Dong, Cong, Xu and Cao2016a ); mafic rocks in southern Lhasa terrane (Meng et al. Reference Meng, Xu, Santosh, Ma, Chen, Guo and Liu2016b ). The new continental crust (NC) evolutionary line is defined by isotopic growth from 176Hf/177Hf=0.279703 at 4.55 Ga to 0.283145 at present, with 176Lu/177Hf= 0.0375 (Dhuime, Hawkesworth & Cawood, Reference Dhuime, Hawkesworth and Cawood2011).
Fifteen Hf isotopic spot analyses on zircon grains from the quartz diorite sample GD14-89-1 yield initial 176Hf/177Hf ratios ranging from 0.282976 to 0.283039, with corresponding ε Hf(t) values of 11.1–13.4 (Figs 9a, 10) and two-stage Hf model ages of 503–359 Ma. Fifteen Hf isotopic spot analyses on the zircon grains from the other sample (GD14-93-1) yield initial 176Hf/177Hf ratios of 0.282846–0.282955, with relatively low ε Hf(t) values of 6.6–10.5 (Figs 9b, 10) and high two-stage Hf model ages ranging from 798 to 548 Ma.
Fifteen Hf isotopic spot analyses on zircon grains from the mafic enclave sample (GD14-90-3) yield a narrow range of ε Hf(t) values of 10.0–13.0 with corresponding two-stage Hf crustal model ages of 577–385 Ma (Figs 9c, 10).
Fourteen Hf isotopic analyses on zircon grains from the tonalite sample GD15-L08-1 show initial 176Hf/177Hf ratios of 0.282867–0.282952 and corresponding ε Hf(t) values of 7.3–10.3, while two-stage Hf model ages range from 750 to 555 Ma (Figs 9d, 10). Fifteen Hf isotopic analyses on zircon grains from the tonalite sample GD14-50-1 show a narrow ε Hf(t) value range of 3.5–5.5 and two-stage Hf crustal model ages of 977–850 Ma (Figs 9e, 10).
Shui et al. (Reference Shui, He, Zhang and Lu2016) obtained Hf isotopic compositions for two samples (GD14-99-2 and GD14-102-1) from the Xiangmucun tonalite in a previous study. One sample GD14-99-2 has zircon ε Hf(t) values of 8.0–9.8 and two-stage Hf model age of 695–575 Ma (Figs 9f, 10), while the other sample GD14-102-1 yields similar zircon ε Hf(t) values of 7.9–9.3 and two-stage Hf model ages of 700–610 Ma (Figs 9g, 10).
5. Discussion
5.a. Magma mixing process: the mafic enclaves in the quartz diorite
Mafic enclaves are quite common in granitoids and can provide important information on the origin of the host rocks and related geodynamic processes (e.g. Vernon, Reference Vernon1984; Chappell, White & Wyborn, Reference Chappell, White and Wyborn1987; Barbarin, Reference Barbarin2005; Mo et al. Reference Mo, Dong, Zhao, Guo, Wang and Chen2005; Yang et al. Reference Yang, Wu, Wilde, Xie, Yang and Liu2007; Gao et al. Reference Gao, Klemd, Long, Xiong and Qian2009; Shellnutt, Jahn & Dostal, Reference Shellnutt, Jahn and Dostal2010; Wang et al. Reference Wang, Li, Jia, Wyman, Tang, Li, Ma, Yang, Jiang and Gou2012; Meng et al. Reference Meng, Zhao, Zhu, Mo, Guan, Huang, Dong, Zhou, DePaolo, Harrison, Zhang, Liu, Liu, Hu and Yuan2014; Lu et al. Reference Lu, He, Zhang, Shui and Yan2016). Several possibilities were proposed for the formation of the enclaves, including xenoliths from the country rock (e.g. Xu et al. Reference Xu, Gao, Wang, Wang and Liu2006), cumulates of early-formed co-genetic crystals (e.g. Gao et al. Reference Gao, Klemd, Long, Xiong and Qian2009; Shellnutt, Jahn & Dostal, Reference Shellnutt, Jahn and Dostal2010; Niu et al. Reference Niu, Zhao, Zhu and Mo2013; Chen et al. Reference Chen, Niu, Sun, Zhang, Li, Guo and Sun2015), refractory and residual phase assemblages of the sources of the granitoids (e.g. Chappell, White & Wyborn, Reference Chappell, White and Wyborn1987), and products of magma mixing and mingling (Vernon, Reference Vernon1984; Barbarin, Reference Barbarin2005; Mo et al. Reference Mo, Dong, Zhao, Guo, Wang and Chen2005; Yang et al. Reference Yang, Wu, Wilde, Xie, Yang and Liu2007; Meng et al. Reference Meng, Zhao, Zhu, Mo, Guan, Huang, Dong, Zhou, DePaolo, Harrison, Zhang, Liu, Liu, Hu and Yuan2014; Lu et al. Reference Lu, He, Zhang, Shui and Yan2016). In the last model, the mafic enclaves are believed to have formed by a mafic magma intruding a felsic magma chamber, where they mixed and mingled (Vernon, Reference Vernon1984; Barbarin, Reference Barbarin2005; Yang et al. Reference Yang, Wu, Wilde, Xie, Yang and Liu2007).
Here, oval or irregular-shaped mafic enclaves are abundant in the host quartz diorite (Fig. 3a–c) and both types have, within error, identical U–Pb zircon magmatic crystallization ages (Fig. 8a–d). The mafic enclaves contain euhedral acicular apatites enclosed within the larger plagioclase grains (Fig. 4c), corresponding with a typical rapid quenching texture (Sparks & Marshall, Reference Sparks and Marshall1986). Some plagioclase phenocrysts in the mafic enclaves seem to be transferred from the host magma (Figs 3b, 4a, b), which is thought to be due to the low rheological contrast between the two magmas that allows crystal transfer from a more felsic host magma into a more mafic magma (Waight, Maas & Nicholls, Reference Waight, Maas and Nicholls2000; Perugini et al. Reference Perugini, Poli, Christofides and Eleftheriadis2003). Amphiboles in such mafic enclaves are commonly characterized by inclusions of quartz, biotite, Fe–Ti oxides and needle-like apatite, also indicating magma mixing (Foley et al. Reference Foley, Pearson, Rushmer, Turner and Adam2013; Lu et al. Reference Lu, He, Zhang, Shui and Yan2016) (Fig. 4b, c). Therefore, the mafic enclaves in the Jiacha quartz diorites are thought to represent globules of mafic magmas that were injected and mixed with the colder, partially crystallized host magma (e.g. Wang et al. Reference Wang, Li, Jia, Wyman, Tang, Li, Ma, Yang, Jiang and Gou2012).
The mafic enclaves are medium-K calc-alkaline rocks that are relatively enriched in LILE and depleted in HFSE with negative Nb, Ta and Ti anomalies (Figs 5a, b, 6b). The depleted Hf isotopic values (ε Hf(t)=10.0–13.0) of the mafic enclaves are similar to those of contemporaneous gabbroic rocks (215 Ma) in the southern Lhasa sub-terrane (Fig. 10), which were interpreted to have formed in the metasomatized mantle wedge (Meng et al. Reference Meng, Xu, Santosh, Ma, Chen, Guo and Liu2016b ). The mafic enclaves exhibit Nb/Ta ratios ranging from 15.1 to 20.2 that are similar to or slightly higher than those (15.5–17.4) of the primitive and depleted mantle and chondrite (Barth et al. Reference Barth, McDonough and Rudnick2000). This indicates that the primitive magma of the mafic enclaves originated from metasomatized mantle peridotites.
5.b. Adakitic quartz diorites: partial melting of subducted oceanic crust
As noted above, the Jiacha quartz diorite has adakitic affinities, e.g. high Al2O3 (16.60–18.22 wt.%), Sr (412–504 ppm), Sr/Y (29.2–45.1), low Y (10.4–15.9 ppm) and Yb (1.09–1.74 ppm) and slightly negative Eu anomalies (Figs 5d, 6a, b). Adakitic rocks may be generated by a variety of mechanisms (see above). We propose that the studied Jiacha adakitic quartz diorite probably formed by partial melting of subducting oceanic crust based on the following reasons: (1) The Jiacha adakitic quartz diorite has low K2O/Na2O (0.28–0.35) and high CaO/Al2O3 (0.33–0.39) values, which are similar to those of adakitic rocks generated by partial melting of subducted oceanic crust (with K2O/Na2O<0.71 and CaO/Al2O3>0.2), including the Cretaceous adakites from the southern Lhasa sub-terrane (Fig. 5b, c; Stern & Kilian, Reference Stern and Kilian1996; Li et al. Reference Li, Zhu, Wang, Zhao, Zhang, Liu, Chang, Lu, Dai and Zheng2016). Furthermore, the Jiacha adakitic quartz diorite samples mostly plot in the field of adakitic rocks derived from melting of subducting oceanic crust in the Ni, Cr, Mg# and MgO discrimination diagrams (Fig. 11 a–e). The high MgO and Mg# values may indicate the interaction of a slab-derived adakitic melt and a mantle-derived magma which is also supported by the presence of the mafic enclaves (Sen & Dunn, Reference Sen and Dunn1994; Rapp et al. Reference Rapp, Shimizu, Norman and Applegate1999). As shown in the SiO2 vs Mg# diagram (Fig. 11b), mantle AFC modelling (DePaolo, Reference DePaolo1981) indicates that the Jiacha adakitic melts most likely mixed with a small amount (<10%) of mantle-derived magma (Hofmann, Reference Hofmann1988); (2) The HREE and Y contents, and the Sr/Y, (La/Yb)N and (Dy/Yb)N ratios of the quartz diorite are generally consistent with those of the Cretaceous adakites derived from subducted oceanic crust in the southern Lhasa sub-terrane (Fig. 6a, b). Their relatively low (La/Yb)N ratios may also have resulted from interaction with a mantle-derived magma. Furthermore, their Th and Th/La values are similar to those of the Cretaceous slab-derived adakites in the southern Lhasa sub-terrane (Fig. 11f; Zhu et al. Reference Zhu, Zhao, Pan, Lee, Kang, Liao, Wang, Li, Dong and Liu2009; Zhang et al. Reference Zhang, Zhao, Santosh, Wang, Dong and Shen2010; Jiang et al. Reference Jiang, Wang, Li, Wyman, Tang, Jia and Yang2012; Ma et al. Reference Ma, Wang, Wyman, Li, Jiang, Yang, Gou and Guo2013); and (3) The ε Hf(t) values of the Jiacha adakitic quartz diorite display positive zircon ε Hf(t) values (6.6–13.4) (Fig. 9), which are similar to those of Indian Ocean MORB (Chauvel & Blichert-Toft, Reference Chauvel and Blichert-Toft2001). Furthermore at least half of the values plot near the ‘new continental crust’ evolutionary line (Dhuime, Hawkesworth & Cawood, Reference Dhuime, Hawkesworth and Cawood2011) (Fig. 10), implying significant involvement of newly formed juvenile crustal material.
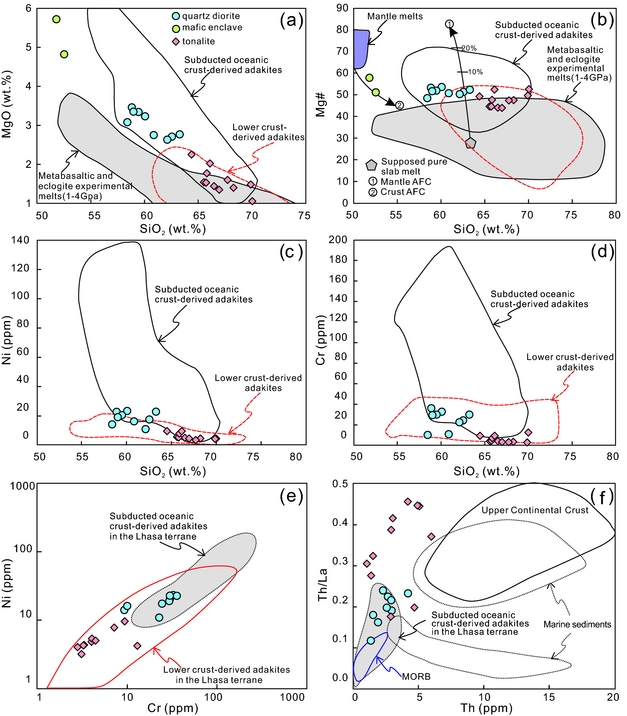
Figure 11. Discrimination diagrams for the Jiacha Early Jurassic adakitic rocks. (a) MgO vs SiO2 diagram (after Huang et al. Reference Huang, Xu, Lan, Yang and Luo2009, and references therein). Data for metabasalt and eclogite experimental melts (1–4 GPa) are from Rapp et al. (Reference Rapp, Shimizu, Norman and Applegate1999) and references therein; (b) Mg# vs SiO2 diagram (after Wang et al. Reference Wang, Li, Jia, Wyman, Tang, Li, Ma, Yang, Jiang and Gou2012, and references therein). Mantle AFC curves are after Rapp et al. (Reference Rapp, Shimizu, Norman and Applegate1999) (curve 1); the proportion of assimilated peridotite is also shown. The crustal AFC curve is after Stern & Kilian (Reference Stern and Kilian1996) (curve 2); (c) Ni vs SiO2 diagram (after Huang et al. Reference Huang, Xu, Lan, Yang and Luo2009, and references therein); (d) Cr vs SiO2 diagram (after Huang et al. Reference Huang, Xu, Lan, Yang and Luo2009, and references therein); (e) Ni vs Cr diagram. Data sources: Late Cretaceous–Miocene (83–10 Ma) lower crust-derived adakites in the Lhasa terrane (Chung et al. Reference Chung, Liu, Ji, Chu, Lee, Wen, Lo, Lee, Qian and Zhang2003; Hou et al. Reference Hou, Gao, Qu, Rui and Mo2004; Gao et al. Reference Gao, Hou, Kamber, Wei, Meng and Zhao2007, Reference Gao, Yang, Santosh, Hou, Wei and Tian2010; Guo, Wilson & Liu, Reference Guo, Wilson and Liu2007; Wen et al. Reference Wen, Chung, Song, Iizuka, Yang, Ji, Liu and Gallet2008a ; Guan et al. Reference Guan, Zhu, Zhao, Dong, Zhang, Li, Liu, Mo, Liu and Yuan2012; Ma et al. Reference Ma, Wang, Jiang, Wang, Li, Wyman, Zhao, Yang, Gou and Guo2014; Meng et al. Reference Meng, Zhao, Zhu, Mo, Guan, Huang, Dong, Zhou, DePaolo, Harrison, Zhang, Liu, Liu, Hu and Yuan2014). Cretaceous (137–86 Ma) subducted oceanic crust-derived adakites in the Lhasa terrane (Zhu et al. Reference Zhu, Zhao, Pan, Lee, Kang, Liao, Wang, Li, Dong and Liu2009; Guan et al. Reference Guan, Zhu, Zhao, Zhang, Liu, Li, Yu, Liu and Mo2010; Zhang et al. Reference Zhang, Zhao, Santosh, Wang, Dong and Shen2010; Jiang et al. Reference Jiang, Wang, Li, Wyman, Tang, Jia and Yang2012; Ma et al. Reference Ma, Wang, Wyman, Li, Jiang, Yang, Gou and Guo2013); (f) Th/La vs Th diagram. The data for upper continental crust are from Plank (Reference Plank2005) and references therein. The data for marine sediments are from Plank & Langmuir (Reference Plank and Langmuir1998) and for MORB are from Niu & Batiza (Reference Niu and Batiza1997).
In summary, the Jiacha adakitic quartz diorite was probably derived by partial melting of subducting oceanic crust. The slab-derived melts were mixed with mantle-derived magma during ascent, which increased their MgO and compatible elements contents. It is noteworthy that sample GD14-93-01 shows lower ε Hf(t) values (6.6–10.5) than the other quartz diorite sample (ε Hf(t)=11.1–13.4) (Figs 9, 10). This indicates that crustal-derived magma may also have contributed to the generation of the adakitic quartz diorites, which is also consistent with their low Ni and Cr contents relative to the typical slab-derived melts (Fig. 11c, d) and is similar to a modified slab-derived magma modified by crustal components (Kelemen, Hart & Bernstein, Reference Kelemen, Hart and Bernstein1998).
5.c. Adakitic tonalites: partial melting of thickened lower crust
Although the adakitic quartz diorite and tonalites coexist in the southern Lhasa sub-terrane, there is a disparity in their geochemical characters, indicating different magma sources (Figs 5, 6, 11). The adakitic tonalites have high SiO2, K2O and low A/NK ratios, MgO (0.93–2.25 wt.%) and Mg# (44–53) values are similar to those of experimentally derived metabasaltic and eclogitic melts at high pressures of 1.0–4.0 GPa and adakitic rocks derived from thickened lower crust (Fig. 5b, c; Fig. 11a, b). Melting experiments have revealed that pristine melts of basaltic rocks yield low MgO and Mg# values (e.g. Rapp & Watson, Reference Rapp and Watson1995), which are similar to those of adakitic rocks derived from partial melting of thickened lower crust (Castillo, Reference Castillo2012). In the Ni vs Cr and Cr vs SiO2 diagrams (Fig. 11c–e), all tonalite samples plot in the field of Late Cretaceous–Miocene adakitic rocks derived by partial melting of thickened lower crust from the southern Lhasa sub-terrane, which is in accordance with the very low concentrations of compatible elements (Cr=2.71–13.0 ppm, Ni=3.12–9.60 ppm; Fig. 11e). Therefore, we suggest that the Jiacha adakitic tonalites were probably derived by partial melting of thickened lower crust.
The concave-upward Dy–Ho–Er–Tm-depleted patterns (Fig. 6d) observed in the Jiacha adakitic tonalites indicate that they were probably formed by partial melting of basaltic lower crust under variable water fugacities, generating a garnet-bearing and amphibole-rich residue (Petford & Atherton, Reference Petford and Atherton1996; Guan et al. Reference Guan, Zhu, Zhao, Dong, Zhang, Li, Liu, Mo, Liu and Yuan2012; Shui et al. Reference Shui, He, Zhang and Lu2016). However, garnet is strongly enriched in HREE, while amphibole is relatively enriched in MREE (Green, Reference Green1994). The Jiacha adakitic tonalite samples show stronger depletions of MREE than HREE, suggesting that amphibole is the major residual phase rather than garnet.
The adakitic tonalites have lower ε Hf(t) (3.5–10.3) values relative to those of the adakitic quartz diorite (Fig. 9). All samples plot in the evolutionary zone of the Neoproterozoic crust below the ‘new continental crust’ evolutionary line (Dhuime, Hawkesworth & Cawood, Reference Dhuime, Hawkesworth and Cawood2011) (Fig. 10), implying that the Jiacha adakitic tonalites were probably formed by reworking of Neoproterozoic mafic lower crust (Figs 9, 10). However, the tonalites from the different bodies have inconsistent Hf isotopic compositions, with some high ε Hf(t) values close to those of the quartz diorites (Figs 9, 10), indicating that the Neoproterozoic mafic lower crust cannot have acted alone as their single melt source but rather represent mixing products of highly differentiated crustal and mantle-derived melts. Therefore, the heterogeneous zircon Hf isotopic compositions of the adakitic quartz diorites and tonalites of the southern Lhasa sub-terrane indicate extensive melt interaction involving slab-, mantle- and ancient crustal-derived melts. The Jiacha adakitic tonalites were probably derived by partial melting of Neoproterozoic mafic lower crust in the stability field of garnet and amphibole with variable contributions of mantle- or slab-derived magma.
5.d. Tectonic implications for the Bangong–Nujiang Ocean (Meso-Tethys) subduction
Recently, voluminous Late Triassic–Early Jurassic intrusive rocks (226–150 Ma) including mafic rocks and normal calc-alkaline granitoids were discovered in the southern Lhasa sub-terrane (e.g. Chu et al. Reference Chu, Chung, Song, Liu, O'Reilly, Pearson, Ji and Wen2006; Qu, Xin & Xu, Reference Qu, Xin and Xu2007; Zhang et al. Reference Zhang, Xu, Guo, Zong, Cai and Yuan2007a ; Ji et al. Reference Ji, Wu, Chung, Li and Liu2009a , Reference Ji, Wu, Liu and Chung b ; Yang et al. Reference Yang, Hou, Jiang, Zhang and Song2011; Zhu et al. Reference Zhu, Zhao, Niu, Mo, Chung and Hou2011a ; Dong & Zhang, Reference Dong and Zhang2013; Guo et al. Reference Guo, Liu, Liu, Cawood, Wang and Liu2013; Qiu et al. Reference Qiu, Wang, Zhao and Yu2015; Meng et al. Reference Meng, Dong, Cong, Xu and Cao2016a , Reference Meng, Xu, Santosh, Ma, Chen, Guo and Liu b ; Shui et al. Reference Shui, He, Zhang and Lu2016; Ma et al. Reference Ma, Meng, Xu and Liu2017) (Fig. 1b). The mafic rocks were interpreted to be products of partial melting of a heterogeneous mantle source (Kang et al. Reference Kang, Xu, Wilde, Feng, Chen, Wang, Fu and Pan2014; Meng et al. Reference Meng, Xu, Santosh, Ma, Chen, Guo and Liu2016b ). Normally calc-alkaline granitoids are metaluminous to peraluminous, with concave-upward MREE-depleted REE patterns, and their magmas are thought to have dominantly formed by partial melting of juvenile lower crust with variable ancient continental crustal material contributions (Chu et al. Reference Chu, Chung, Song, Liu, O'Reilly, Pearson, Ji and Wen2006; Zhang et al. Reference Zhang, Xu, Guo, Zong, Cai and Yuan2007a ; Ji et al. Reference Ji, Wu, Chung, Li and Liu2009a , Reference Ji, Wu, Liu and Chung b ; Zhu et al. Reference Zhu, Zhao, Niu, Mo, Chung and Hou2011a ; Guo et al. Reference Guo, Liu, Liu, Cawood, Wang and Liu2013; Meng et al. Reference Meng, Dong, Cong, Xu and Cao2016a ). These Late Triassic–Early Jurassic magmatic rocks are interpreted to have formed in an arc tectonic setting (Zhang et al. Reference Zhang, Xu, Guo, Zong, Cai and Yuan2007a ; Zhu et al. Reference Zhu, Pan, Chung, Liao, Wang and Li2008; Ji et al. Reference Ji, Wu, Chung, Li and Liu2009a , Reference Ji, Wu, Liu and Chung b ; Kang et al. Reference Kang, Xu, Wilde, Feng, Chen, Wang, Fu and Pan2014; Meng et al. Reference Meng, Dong, Cong, Xu and Cao2016a , Reference Meng, Xu, Santosh, Ma, Chen, Guo and Liu b ; Wang et al. Reference Wang, Ding, Zhang, Kapp, Pullen and Yue2016). However, two contrasting tectonic models have mainly been proposed for the formation of the Late Triassic–Early Jurassic arc setting: (1) northward subduction of the Neo-Tethyan oceanic plate (e.g. Chu et al. Reference Chu, Chung, Song, Liu, O'Reilly, Pearson, Ji and Wen2006; Zhang et al. Reference Zhang, Xu, Guo, Zong, Cai and Yuan2007a , Reference Zhang, Xu, Guo, Zong, Cai and Yuan b ; Zhu et al. Reference Zhu, Pan, Chung, Liao, Wang and Li2008; Guo et al. Reference Guo, Liu, Liu, Cawood, Wang and Liu2013; Kang et al. Reference Kang, Xu, Wilde, Feng, Chen, Wang, Fu and Pan2014; Meng et al. Reference Meng, Dong, Cong, Xu and Cao2016a , Reference Meng, Xu, Santosh, Ma, Chen, Guo and Liu b ; Wang et al. 2016; Ma et al. Reference Ma, Meng, Xu and Liu2017) or (2) southward subduction of the Bangong–Nujiang (Meso-Tethys) oceanic plate (e.g. Zhu et al. Reference Zhu, Zhao, Niu, Mo, Chung and Hou2011a ; Song et al. Reference Song, Liu, Zhu, Wang, Zhang, Zhang and Zhao2014; Wei, Reference Wei2014; however, see Kapp et al. Reference Kapp, Murphy, Yin, Harrison, Ding and Guo2003, Reference Kapp, DeCelles, Gehrels, Heizler and Ding2007 for a different view). The separation of the Lhasa Terrane from northern Australia, resulting from the Neo-Tethyan back-arc spreading, began during mid- to late Triassic times (Metcalfe, Reference Metcalfe1996, Reference Metcalfe, Buffetaut, Cuny, Loeuff and Suteethorn2009, Reference Metcalfe2011; Zhu et al. Reference Zhu, Zhao, Niu, Mo, Chung and Hou2011a , Reference Zhu, Zhao, Niu, Dilek and Mo b ). Furthermore most of the well-studied ophiolites in the Yarlung Zangpo suture zone are 130–120 Ma, suggesting that the initial subduction of the Neo-Tethyan oceanic plate beneath the southern margin of the Lhasa block started in the Cretaceous (at c. 130–120 Ma; Zhu et al. Reference Zhu, Zhao, Niu, Mo, Chung and Hou2011a ; Dai et al. Reference Dai, Wang, Polat, Santosh, Li and Ge2013; Zhong et al. Reference Zhong, Dai, Wang, Li and Wei2016). Zhu et al. Reference Zhu, Zhao, Niu, Mo, Chung and Hou(2011a) argued that the Late Triassic–Early Jurassic magmatism of the Lhasa terrane has resulted from the southward Bangong–Nujiang oceanic plate subduction beneath the Lhasa terrane based on a synthesis of Mesozoic–Early Palaeogene magmatic rocks across southern Tibet. The southward-directed Bangong–Nujiang (Meso-Tethys) oceanic plate subduction may have been triggered by the Lhasa–northern Australia collision (Sengör et al. Reference Sengör, Altlner, Cin, Ustaiimer, Hsti and Audley-Charles1988; Zhu et al. Reference Zhu, Zhao, Niu, Mo, Chung and Hou2011a , Reference Zhu, Zhao, Niu, Dilek and Mo b ). Therefore, the Late Triassic–Early Jurassic magmatic rocks in the Lhasa terranes are believed to be related to the southward subduction of the Bangong–Nujiang oceanic plate.
In general, the generation of adakitic magmas from subducting oceanic crust >25 Ma requires an additional heat supply (Defant & Drummond, Reference Defant and Drummond1990; Castillio, Reference Castillo2006, Reference Castillo2012). According to experimental results and thermal modelling, partial melting of subducted oceanic crust only occurs in hot subduction zone settings under a restricted set of circumstances (800–1000°C at depths of 70–80 km: Peacock, Rushmer & Thompson, Reference Peacock, Rushmer and Thompson1994; Sen & Dunn, Reference Sen and Dunn1994; van Keken et al. Reference van Keken, Hacker, Syracuse and Abers2011). Thus, a simple normal-angle (cold) subduction model cannot easily explain shallow slab-melting (such as the studied Early Jurassic quartz diorite in the southern Lhasa sub-terrane). This is because at shallow depths of normal subduction zones (i.e. beneath the fore-arc wedge) the dominant fluid-producing process is dehydration instead of melting of the subducted oceanic crust (for an extensive review see Klemd, Reference Klemd, Harlov and Austrheim2013). Here we suggest that asthenospheric upwelling during the Neo-Tethys back-arc spreading – probably a result of slab rollback of the subducted Meso-Tethys oceanic crust – provided the high thermal regime necessary for partial melting of the oceanic crust and was thus responsible for the formation of the Jiacha Early Jurassic adakitic rocks (Fig. 12) (Zhu et al. Reference Zhu, Zhao, Niu, Mo, Chung and Hou2011a ; Song et al. Reference Song, Liu, Zhu, Wang, Zhang, Zhang and Zhao2014; Wei, Reference Wei2014). The slab rollback is thought to have triggered the invasion of deep asthenosphere into the mantle wedge and the development of an extensional environment in the overlying lithosphere mantle. The high thermal anomaly resulted in the partial melting of the subducted oceanic crust, forming the adakitic quartz diorites. Synchronously, mantle-derived basaltic magmas injected the adakitic quartz diorite to form mafic enclaves. Furthermore, the basaltic magma generated by partial melting of the metasomatized mantle wedge underplated the lower crust, resulting in the thickening and reworking of the ancient crust of the southern Lhasa sub-terrane. Subsequently the adakitic tonalites were produced by partial melting of the thickened lower crust as a result of continued basaltic magma underplating (Fig. 12).

Figure 12. Conceptual diagram illustrating the tectonic and magma genesis of the Lhasa terrane in the Early Jurassic. Slab rollback of the subducted Bangong–Nujiang oceanic plate resulted in hot asthenospheric mantle upwelling and decompression melting as well as initiation of back-arc basin (Neo-Tethys). The asthenospheric upwelling was responsible for high-temperature conditions over a wide area in the fore-arc wedge. The unusually high-temperature conditions in the mantle wedge caused the partial melting of the subducted slab, responsible for formation of the Jiacha adakitic quartz diorites. Synchronously, mantle-derived basaltic magmas were injected into the Jiacha adakitic quartz diorites to form mafic enclaves. Furthermore, the basaltic magma generated by partial melting of the metasomatized mantle wedge underplated the lower crust, resulting in the thickening and reworking of the ancient crust of the southern Lhasa sub-terrane. The adakitic tonalites were produced by partial melting of the thickened lower crust as a result of continued basaltic magma underplating.
6. Conclusions
-
(1) LA-ICP-MS zircon U–Pb dating suggests emplacement ages of 199–179 Ma for newly discovered Early Jurassic adakitic rocks from the Jiacha area in the southern Lhasa sub-terrane.
-
(2) The adakitic quartz diorites are products of partial melting of the subducted Bangong–Nujiang (Meso-Tethys) oceanic crust, while the adakitic tonalites were derived from Neoproterozoic lower crust.
-
(3) The formation of the Early Jurassic adakitic rocks and Neo-Tethys back-arc spreading probably resulted from slab rollback of the subducted Bangong–Nujiang oceanic plate.
Acknowledgements
This study was supported by National Natural Science Foundation of China (41230205); the Chinese Geological Survey Project (DD20160022); and a scholarship from the China Scholarship Council (Z.Y. H). We thank two anonymous referees for constructive comments on the manuscript, and Dr Chad Deering for editorial handling.
Supplementary material
To view supplementary material for this article, please visit https://doi.org/10.1017/S0016756817000577