Pre-term birth before 32 weeks of gestation is a risk factor for heart failure in childhood and young adulthood.Reference Carr, Cnattingius, Granath, Ludvigsson and Edstedt Bonamy1,Reference Crump, Howell, Stroustrup, McLaughlin, Sundquist and Sundquist2 This may have its origin in developmental alterations in cardiac function and morphometry associated with pre-maturity and its effects on the developmental programming of the heart.Reference Aye, Lewandowski and Lamata3–Reference Burchert and Lewandowski5 The terminal differentiation of myocytes that occurs in late foetal life, specifically during the third trimester, dictates cardiomyocyte endowment for life as the heart loses the proliferative capacity (hyperplasia) soon after birth.Reference Bensley, Stacy, De Matteo, Harding and Black6 Pre-mature birth may limit the cell population available to support myocardial growth trajectory and therefore affect ventricular function in pre-term born infants.Reference Mohlkert, Hallberg and Broberg7
Normal ventricular function requires coordinated electrical activation and mechanical contraction. Myocardial deformation analysis has emerged as a quantitative echocardiographic technique to characterise global and regional ventricular function in neonates.Reference Breatnach, Levy, James, Franklin and El-Khuffash8 Since the left ventricle consists of longitudinal fibres in the endocardial and epicardial layers, and circumferential fibres in the mid-wall layer, left ventricular deformation can be described in terms of normal strain (longitudinal, circumferential, and radial thickening) and shear strain in the circumferential–longitudinal plane (rotational mechanics).Reference El-Khuffash, Schubert, Levy, Nestaas and de Boode9 Collectively, the regional inhomogeneity and helical arrangement of these myocardial fibres facilitate the mechanical shortening and lengthening sequences of the left ventricle walls, resulting in highly efficient global function of the normal heart.Reference Sedmera10
Torsion describes the twisting or wringing motion that occurs during the rotation of the apex and base of the left ventricle during systole and diastole. This rotational twist of myofibres orchestrates mechanical shortening and serves as an important determinant of left ventricular performance by improving ejection of blood during systole, early diastole, with the recoil produced by untwisting generating a suction force to facilitate diastolic filling. Torsion has been described from infancy to adulthood in patients born at term,Reference Notomi, Srinath and Shiota11 but there is a paucity of studies establishing longitudinal patterns of rotational mechanics in pre-mature infants.Reference James, Corcoran, Mertens, Franklin and El-Khuffash12–Reference James, Corcoran, Hayes and Franklin14 The reported differences in rotational shear strain between pre-mature infants and term neonates are likely due to both the haemodynamic changes after birth, inherent myocardial structural anisotropy, and subsequent ventricular remodelling with adaptation of the pre-mature myocardium,Reference James, Corcoran, Mertens, Franklin and El-Khuffash12,Reference Breatnach, Forman and Foran13,Reference LeGrice, Smaill, Chai, Edgar, Gavin and Hunter15 but these mechanisms remain incompletely characterised in pre-term born infants.
Our group has demonstrated that maturational patterns of global and regional left ventricular longitudinal deformation patterns in extremely pre-term infants were preserved throughout the neonatal period and were not affected by the presence of common neonatal morbidities, for example, bronchopulmonary dysplasia and/or pulmonary hypertension.Reference Levy, El-Khuffash and Patel16 Accordingly, we hypothesised that rotation mechanics would similarly be preserved in pre-term infants, but pre-maturity-associated cardiopulmonary conditions may influence the rotational physiology differently. The primary objectives of this study were to determine the maturational changes of left ventricular rotational mechanics and to establish patterns for rotation, twist and torsion in pre-term infants. Our secondary aim was to evaluate the effect of pre-maturity-associated complications on the maturational process of the rotational mechanics in pre-term infants over the first year of age.
Material and methods
Study population
In this prospective longitudinal study, 137 extremely pre-term infants (born between 23 0/7 and 28 6/7 weeks gestation) were recruited at birth and longitudinally followed until 1-year corrected age between August 2011 and November 2013 at the Washington University in St. Louis/St. Louis Children’s Hospital neonatal ICU. The infants were recruited from the Premature and Respiratory Outcomes Program (ClinicalTrials.gov identifier NCT01435187).Reference Pryhuber, Maitre and Ballard17 Infants with any suspected congenital anomalies of the airways, lungs or chest wall, CHD (except for haemodynamically insignificant ventricular or atrial septal defects), chromosomal anomalies, and neuromuscular disorders were excluded from the study. Reference values and maturational patterns of right ventricle fractional area of change,Reference James, Corcoran and Franklin18,Reference Levy, Dioneda and Holland19 deformation (strain),Reference Levy, El-Khuffash and Patel16,Reference Levy, Holland, Sekarski, Hamvas and Singh20–Reference James, Corcoran, Breatnach, Franklin, Mertens and El-Khuffash22 and pulmonary artery acceleration timeReference Levy, Patel, Choudhry, Hamvas and Singh23,Reference Patel, Breatnach and James24 from this cohort have been published, but complete rotational measures over the first year of age have not been detailed for the cohort. The institutional review board of Washington University School of Medicine approved the protocol. Written informed consent was obtained from the parents or guardians of participants.
Patient characteristics, definitions, identification of an “uncomplicated” cohort
Demographic characteristics of infants were collected at 32 weeks post-menstrual age, 36 weeks post-menstrual age, and 1-year corrected age. Physiologic and clinical biomarkers were obtained at these time points and included heart rate, blood pressure, respiratory rate, level of supplemental oxygen, and respiratory support. The infants’ antenatal, delivery, and demographic characteristic were obtained. We used a previously published approach to define a cohort of uncomplicated pre-term infants.Reference Levy, El-Khuffash and Patel16,Reference Levy, Dioneda and Holland19,Reference Patel, Breatnach and James24 Infants with “cardiorespiratory healthiness” were identified and defined to be a part of an “uncomplicated” cohort of infants.Reference Levy, El-Khuffash and Patel16,Reference Levy, Dioneda and Holland19,Reference Patel, Breatnach and James24 This cohort did not have (1) bronchopulmonary dysplasia, defined as the need for persistent supplemental oxygen support at 36 weeks post-menstrual age,Reference Poindexter, Feng and Schmidt25 (2) patent ductus arteriosus at 32 or 36 weeks post-menstrual age, or (3) echocardiographic signs of late-onset pulmonary hypertension beyond 32 weeks post-menstrual age, defined as having estimated right ventricular systolic pressure more than 40 mmHg, a ratio of right ventricular systolic pressure to systemic systolic blood pressure >0.5, any cardiac shunt with bidirectional or right to left flow, unusual degree of right ventricular hypertrophy or dilatation, or ventricular septal wall flattening.Reference Levy, El-Khuffash and Patel16 This protocol followed the same exclusion criteria for the presence of a haemodynamically significant patent ductus arteriosus as the original study.Reference Levy, El-Khuffash and Patel16 The assessment of “cardiorespiratory healthiness” was used as a confounder to adjust for clinically significant physiologic factors in our statistical model. The study flow diagram is presented in Fig 1.

Figure 1. Study flow diagram of pre-term infants. Gestational age (GA). Post-menstrual age (PMA). Corrected age (CA). Bronchopulmonary dysplasia (BPD). Pulmonary hypertension (PH). Patent ductus arteriosus (PDA).
Echocardiography
Transthoracic echocardiograms were performed at 32 weeks post-menstrual age, 36 weeks post-menstrual age, and 1-year corrected age using a commercially available ultrasound imaging system (Vivid 7 and 9; General Electric Medical Systems, Milwaukee, Wisconsin). One designated paediatric cardiac sonographer (T.S.) experienced in acquiring images for conventional and two-dimensional speckle-tracking echocardiography analysis obtained all the echocardiographic images according to the American Society of Echocardiography guidelines.Reference Lang, Badano and Mor-Avi26 We also utilised a validated neonatal protocol to acquire the images from the decubitus position during restful period without changing the position of the infant or disturbing the haemodynamic condition to minimise heart rate and respiratory variation during the image acquisition.Reference Levy, El-Khuffash and Patel16,Reference Levy, Holland, Sekarski, Hamvas and Singh20 The timings of the echocardiograms at 32 weeks and 36 weeks post-menstrual age were selected to avoid the early postnatal period of clinical and cardiopulmonary instability and early mortality associated with extreme pre-term birth.Reference Levy, Dioneda and Holland19
Rotational mechanics
Left ventricular global and segmental circumferential and radial strain were measured from the parasternal short-axis views using three beat clips at three levels, namely apical (distal to papillary muscles), mid (level of the papillary muscles), and basal (level of the mitral valve leaflets) levels of the left ventricle according to a previously validated image acquisition and data analysis protocols.Reference James, Corcoran, Mertens, Franklin and El-Khuffash12,Reference Breatnach, Forman and Foran13 In addition, we used a specific research protocol that individually obtained the images at the basal, mid-ventricular, and apical levels to ensure that the left ventricular cross section was as circular as possible. The clips were acquired sequentially to minimise the effect of the beat-to-beat variation. The heart rate ranged within five beats per minute between each clip. Sequential clips with heart rates greater than five beats per minute were not analysed. A frame rate to heart rate ratio between 0.7 and 0.9 frames/sec per beats per minute was utilised to optimise myocardial speckle-tracking and mechanical event timing.Reference Sanchez, Levy, Sekarski, Hamvas, Holland and Singh27 Vendor customised software (EchoPAC; General Electric Medical Systems, Waukesha, WI, USA, version 210.0.x) was used to perform offline measurements of left ventricular basal and apical rotation, twist, and torsion. Rotation was defined as the circumferential clockwise or counterclockwise movement (in degrees) of the apex and base along the long axis of the left ventricle occurring during systole. Viewed from the apex, clockwise rotation was depicted as negative and counterclockwise rotation as positive. Basal and apical rotations were plotted against time during one cardiac cycle (Fig 2). Twist was defined as the difference between peak apical and peak basal rotation using the formula twist (°) = apical rotation – basal rotation. Torsion (°/cm) was derived by indexing twist (°) to left ventricular end-diastolic length using the formula: torsion (°/cm) = twist (°)/ left ventricular length (cm). Left ventricular length, the distance between the midpoint of the mitral valve annulus and the apex, was measured in the apical four-chamber view in diastole with the mitral valve closed. This was computed using the average of three measurements. Indexing twist to left ventricular length enables comparison of twist across different left ventricular lengths.Reference James, Corcoran, Mertens, Franklin and El-Khuffash12
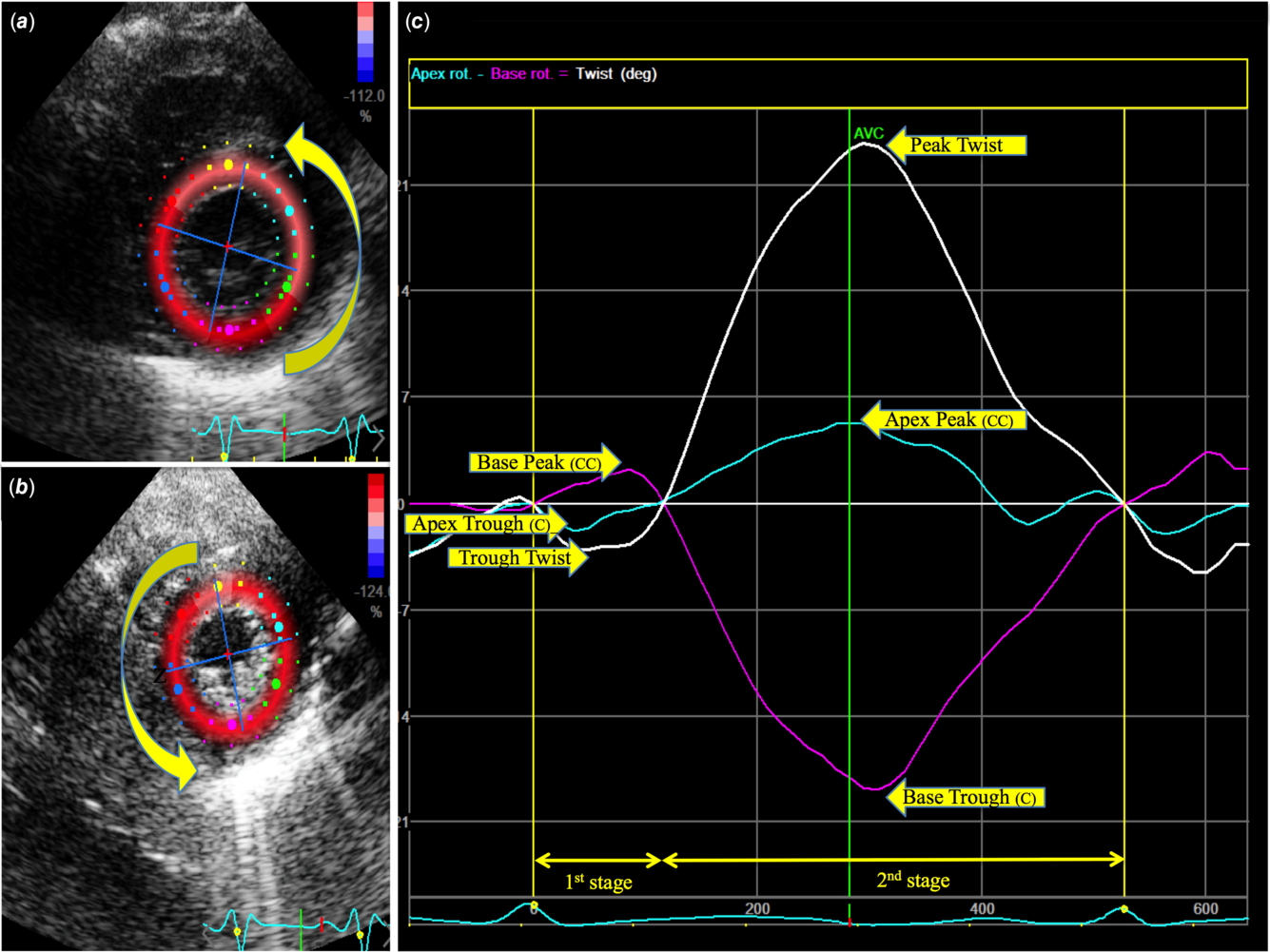
Figure 2. Rotational Strain Analysis at the (a) basal and (b) apical levels of parasternal short-axis view. Region of interest tracings of the left ventricular endocardial border are shown. (c) Basal and apical rotation are plotted against time during one cardiac cycle and determine the net twist. C, clockwise; CC, clockwise. AVC, aortic valve closure.
Feasibility and reproducibility
Intra- and interobserver variability for all rotational measures were assessed in 50% of the infants by two investigators, both of whom were blinded to the clinical and demographic data. Each observer used the same measurement protocolReference James, Corcoran, Mertens, Franklin and El-Khuffash12 and was blinded to the other’s results. Equal distributions of studies were chosen at each time point, and coefficient of variation and intraclass correlation coefficient were used to assess the reproducibility.
Statistical analysis
Baseline demographic and clinical characteristics of patients were described as mean ± standard deviations or as percentages. Continuous variables of strain imaging were tested for normality using the Kolmogorov–Smirnov test and a histogram of the data. All outcome variables with non-normal distributions were analysed in simple comparisons using Wilcoxon rank sum tests or Kruskal–Wallis one-way analysis of variance for tests with more than two independent groups. A random effects model for repeated measures, controlling for gestational age and “cardiopulmonary health”, was used to compare rotational strain values from 32 weeks post-menstrual age, 36 weeks post-menstrual age, and 1-year corrected age. A subgroup analysis was performed by stratifying the cohort into an “uncomplicated” and “complicated” cohort. A random effects model for repeated measures, controlling for gestational age, was then run for each cohort. To further analyse the change in torsion from 32 to 36 weeks post-menstrual age between the “uncomplicated” and “complicated” cohort, a two-way repeated measures analysis of variance was performed. Univariate analysis was used to determine the best predictors to enter in the model, and then backward stepwise regression was performed to assess the independent effect of gestational age, gender, total oxygen days, length of stay, and common neonatal morbidities (necrotising enterocolitis, intraventricular haemorrhage, and retinopathy of pre-maturity), while adjusting for weight at examination.Reference Patel, Breatnach and James24 Because of the lack of data regarding the relationship between rotational mechanics and pulmonary hypertension and the observational nature of this study, we used data from previous literature that investigated rotational mechanics in pre-term infants and data from our deformation studies to estimate the sample size, assuming an alpha of 0.05, where 40 subjects per group would provide 99% power to detect 20% differences in echocardiographic measures between groups.Reference James, Corcoran, Mertens, Franklin and El-Khuffash12,Reference Breatnach, Forman and Foran13,Reference Levy, El-Khuffash and Patel16 Statistical analyses were performed using SAS version 9.4 (SAS Institute Inc. Cary, NC) and SPSS version 25 (SPSS Chicago, IL).
Results
Study population
The maternal and infant clinical and demographic data have been previously described for this cohort,Reference Levy, El-Khuffash and Patel16,Reference Levy, Dioneda and Holland19,Reference Patel, Breatnach and James24 with the relevant infant data for rotational analysis characterised in Table 1. Systolic blood pressure, diastolic blood pressure, and mean arterial blood pressure increased from 32 weeks post-menstrual age to 1-year corrected age and heart rate decreased, as expected. Of the 137 eligible pre-term infants born <29 weeks of gestational age enrolled at birth, 13 died within the first month of life (sepsis n = 3; necrotising enterocolitis n = 4; severe cardiopulmonary insufficiency n = 6), five infants were transferred prior to 32 weeks post-menstrual age, and two infants withdrew from the study. There were 117 infants alive at 32 weeks post-menstrual age that received echocardiograms, of which 114 had feasible images to perform rotational analysis. At 36 weeks post-menstrual age, 117 infants had feasible images and of these infants, 80 returned for follow-up at 1-year corrected age and underwent an echocardiogram. Sixty-five infants had sufficient imaging quality necessary to obtain rotational strain measurements via two-dimensional speckle-tracking echocardiography (Fig 2).
Table 1. Demographic and clinical characteristics of pre-mature infants at 32 weeks post-menstrual age, 36 weeks post-menstrual age, and 1-year corrected age.

Data expressed as mean ± standard deviation or as number (percentage). p-value significance determined by ANOVA with p < 0.05 or Chi-square statistic
Bronchopulmonary dysplasia was diagnosed in 69 infants (59%) at 36 weeks post-menstrual age. There were 25 infants (21.4%) with a patent ductus arteriosus at 32 weeks post-menstrual age, 14 infants (12.0%) with a patent ductus arteriosus at 36 weeks post-menstrual age and none at 1-year corrected age. Seventeen infants (14.5%) had echocardiographic evidence of pulmonary hypertension at 32 and 36 weeks post-menstrual age. Additional clinical data and presence of bronchopulmonary dysplasia, patent ductus arteriosus, and/or pulmonary hypertension are presented in Supplemental Table 3.
Patterns of rotational strain in all pre-term infants
Longitudinal patterns of rotational strain measures of entire cohort pre-term infants are listed in Table 2. Left ventricular torsion significantly decreased (p < 0.001) from 32 weeks post-menstrual age (6.13 ± 2.72) to 36 weeks post-menstrual age (5.13 ± 2.04) and to 1-year corrected age (4.08 ± 2.02) after controlling for gestational age, bronchopulmonary dysplasia, patent ductus arteriosus, and pulmonary hypertension. Basal rotation was characterised by an initial counterclockwise rotation that increased over time (p = 0.0024) but had a predominantly clockwise component with an overall increase in magnitude (p = 0.0021). Apical rotation was characterised by an initial clockwise rotation that increased over time (p = 0.029), but had a predominant counterclockwise component that decreased in magnitude (p = 0.207).
Table 2. Patterns of rotational mechanics in pre-mature infants over the first year of age.

Data expressed as mean ± standard deviation
ANOVA, analysis of variance; PMA = post-menstrual age, CA = corrected age
There is no difference in LV length between the uncomplicated and complicated groups at 32 weeks PMA, 36 weeks PMA, or 1-year CA
Confounding cardiopulmonary factors
Comparisons were made between healthy uncomplicated pre-term infants and infants classified with cardiorespiratory disease, the “complicated” group. We also accounted for the effect of antenatal and postnatal administration of steroids, caffeine, and diuretic use. Rotational strain measurements for each cohort mirrored the trends as those seen in the entire cohort (Table 2). In both cohorts, the initial counterclockwise followed by clockwise basal rotation increased in magnitude by 1-year corrected age. For left ventricular apical rotation, the initial clockwise rotation increased in magnitude by 1-year corrected age. At 1-year corrected age, apical counterclockwise rotation in uncomplicated infants increased in magnitude (p = 0.007), while the counterclockwise rotation in the complicated cohort decreased in magnitude (p = 0.368).
Torsion decreased from 32 weeks post-menstrual age to 1-year corrected age in both groups (Fig 3). A subgroup analysis that compared torsion over time revealed that the decrease in torsion from 32 to 36 weeks post-menstrual age differed significantly (p = 0.019) between the “uncomplicated” (5.37 ± 2.35 °/cm to 5.32 ± 2.14°/cm, p < 0.001) and “complicated” infants (6.62 ± 2.85 °/cm to 5.07 ± 2.02 °/cm, p < 0.001) (Fig 3). There was no significant difference in the change in torsion over time from 36 weeks post-menstrual age to 1-year corrected age when comparing the “uncomplicated” (5.32 ± 2.14 °/cm to 4.13 ± 1.99 °/cm, p < 0.001) to “complicated” (5.07 ± 2.02°/cm to 4.12 ± 2.04°/cm, p < 0.001) pre-term infants. Torsion for both of these cohorts was relatively the same (4.13°/cm versus 4.12°/cm) at 1-year corrected age.

Figure 3. Left ventricular (LV) torsion from 32 weeks post-menstrual age (PMA), 36 weeks PMA, and at 1-year corrected age (CA) in “uncomplicated” versus “complicated” pre-term infants. Uncomplicated infants neither have bronchopulmonary dysplasia, a patent ductus arteriosus, nor echocardiographic signs of late pulmonary hypertension at 32 or 36 PMA. * p < 0.01 between groups. A subgroup analysis revealed that the decrease in LV torsion from 32 to 36 weeks PMA differed significantly (p = 0.019) between the “uncomplicated” (5.37 ± 2.35 °/cm to 5.32 ± 2.14°/cm, p < 0.001) and “complicated” infants (6.62 ± 2.85 °/cm to 5.07 ± 2.02 °/cm, p < 0.001). There was no significant difference in the change in torsion over time from 36 weeks PMA to 1 year CA when comparing the “uncomplicated” (5.32 ± 2.14 °/cm to 4.13 ± 1.99 °/cm, p < 0.001) to “complicated” (5.07 ± 2.02°/cm to 4.12 ± 2.04 °/cm, p < 0.001) pre-term infants. At 1-year CA, there is no difference in torsion between groups.
Feasibility and reproducibility
At 32 and 36 weeks post-menstrual age, the measurements were feasible in 95% of the obtained images. At 1-year corrected age, the measurements were feasible in 81% of the obtained images. At all times points with the acquired images, there was high degree of intraobserver agreement for all measurement (coefficient of variation = 5.3%, intraclass correlation coefficient = 0.93 [95% CI, 0.92–0.99]) and interobserver agreement (coefficient of variation = 4.9%, intraclass correlation coefficient = 0.92 [95% CI, 0.88–0.98]).
Discussion
In this prospective longitudinal study of extremely pre-mature infants, normative patterns of rotational strain and the impact of common cardiopulmonary disease were assessed using two-dimensional speckle-tracking echocardiography from 32 weeks post-menstrual age to 1-year corrected age. This study reveals that left ventricular torsion decreases over the first year of age in all pre-term infants, even after controlling for gestational age (p < 0.0001). Interestingly, neonates with pre-maturity-related cardiorespiratory complications demonstrated a more prominent decrease in torsion from 32 to 36 weeks post-menstrual age with recovery by 1-year corrected age. The developmental rotational patterns suggest that the pre-term myocardium in healthy uncomplicated infants physiologically adapts due to the balanced relationship of the basal and apical fibre contribution to torsion. Pre-term infants with cardiopulmonary disease exhibit differences in apical counterclockwise rotation, but overall demonstrate adaptive changes to different haemodynamic loading conditions during the late neonatal period with recovery by 1-year corrected age.
Rotational strain patterns in pre-term infants
Rotational mechanics are complex in the neonatal heart with previous works delineating maturational patterns of rotational mechanics from birth to 36 weeks post-menstrual age and accounting for the impact of some of the pre-maturity-related confounding factors.Reference James, Corcoran, Mertens, Franklin and El-Khuffash12–Reference James, Corcoran, Hayes and Franklin14 Breatnach et al.Reference Breatnach, Forman and Foran13 found that at 36 weeks post-menstrual age, pre-term infants with bronchopulmonary dysplasia had higher left ventricular torsion compared to infants without bronchopulmonary dysplasia, secondary to a higher apical rotation. This observation was also seen in our cohort at 32 weeks post-menstrual age but not exhibited at 36 weeks post-menstrual age or 1-year corrected age. James at al.Reference James, Corcoran, Mertens, Franklin and El-Khuffash12 demonstrated no differences in left ventricular torsion between infants with haemodynamics significant patent ductus arteriosus over the first week of age. While a significant patent ductus arteriosus will increase pulmonary venous return to the left atrium and could affect maximal torsion, previous studies in pre-term infants, children, and adults have found almost no consequences of increased preload on rotational mechanics.Reference Kaku, Takeuchi and Tsang28,Reference Moon, Ingels, Daughters, Stinson, Hansen and Miller29
Unlike other studies, this study not only accounted for the presence of bronchopulmonary dysplasia and patent ductus arteriosus but also assessed the impact of pulmonary hypertension on left ventricular mechanics. Compared with well-documented changes in right ventricular strain mechanicsReference Levy, El-Khuffash and Patel16 and measures of pulmonary haemodynamicsReference Patel, Breatnach and James24 in pre-term infants with echocardiographic evidence of pulmonary hypertension, previous literature reports relatively no change in left ventricular longitudinal strain between asymptomatic pre-term infants and those with pulmonary hypertension.Reference Levy, El-Khuffash and Patel16 Similarly, it has been well documented that left ventricular longitudinal strain remains relatively preserved through 1-year corrected age in asymptomatic pre-term infants and pre-term infants with bronchopulmonary dysplasia.Reference James, Corcoran and Jain21,Reference James, Corcoran, Breatnach, Franklin, Mertens and El-Khuffash22,Reference Schubert, Müller, Abdul-Khaliq and Norman30–Reference Helfer, Schmitz, Bührer and Czernik35 In contrast, we found that left ventricular torsion was higher at 32 and 36 weeks post-menstrual age, and the rate of decline was more pronounced in pre-term infants with bronchopulmonary dysplasia, patent ductus arteriosus, and/or pulmonary hypertension compared to asymptomatic pre-term infants, although there was a recovery by 1-year corrected age. This observed difference may reflect that left ventricular longitudinal strain contributes less to overall left ventricular function and may not pick up subtle changes to haemodynamic alterations. Circumferential–longitudinal shear strain patterns (rotational strain) appear to be more sensitive to effects of disease compared to left ventricular longitudinal strain, due to its larger contribution to cardiac output. The left ventricular circumferential fibres occupy approximately 60% of the ventricular wall thickness, compared to 20% by the left ventricular longitudinal fibres, and provide the main driving force of the left ventricle by reducing ventricular diameter.Reference Greenbaum, Ho, Gibson, Becker and Anderson36–Reference Bussmann, Smith and Cappelleri38
We observed a significant difference in the change in torsion from 32 to 36 weeks post-menstrual age in “complicated” infants (Fig 3), when compared to their “uncomplicated” counterparts. However, beyond 36 weeks post-menstrual age, the myocardium of these “complicated” pre-term infants appears to normalise to their “uncomplicated” pre-term counterparts. These data may reflect myocardial adaptation specific to a transient postnatal insult on the pre-term myocardium that has the potential to persist or repair.Reference Aye, Lewandowski and Lamata3 Further studies are needed to discern whether this is truly a recovery of rotational strain and torsion that persists into adulthood or whether future insults to their myocardium may unmask maladaptive differences secondary to their exposure to pre-maturity-related complications.Reference Greenbaum, Ho, Gibson, Becker and Anderson36
As the first study to explore rotational values at 1-year corrected age in pre-term infants, we report a median value of 4.08 ± 2.02 in asymptomatic pre-term infants at 1-year corrected age. In term infants at 1 year of age, torsion varies widely from 1 to 6.Reference Notomi, Srinath and Shiota11,Reference Streeter, Spotnitz, Patel, Ross and Sonnenblick37–Reference Leeson and Lewandowski39 In term infants, apical twist increases over the first year of age and is the major contributor to torsion.Reference Streeter, Spotnitz, Patel, Ross and Sonnenblick37 In pre-term infants, there is an increasing predominance of basal clockwise rotation, while the apical myofibres had a decreasing predominance of counterclockwise rotation (Table 2). This difference can likely be explained by the recognition that torsional mechanism of left ventricular deformation is greatest towards the apex, as the right-handed helix in the subendocardium and the left-handed helix in the subepicardium converge towards the apex and by the observations that electrical excitation of cardiac motion begins in the base and travels to the apex.Reference Al-Naami40
We suspect that pre-term birth and adaptations to postnatal insults may explain the decreasing torsion over the first year of age in the pre-term population. Left ventricular torsion likely decreases in pre-term infants in response to increased systemic afterload,Reference Zhang, Zhou, Pu, Zou and Tan41 a phenomenon that was observed in this study and others.Reference James, Corcoran, Mertens, Franklin and El-Khuffash12,Reference Breatnach, Forman and Foran13 Diminished cardiomyocyte endowment, early loss of myocyte proliferative capacity from pre-mature birth,Reference Bensley, Moore, De Matteo, Harding and Black4 and decreased myocyte to extracellular matrix ratioReference Burchert and Lewandowski5 in the myocardium of pre-mature infants may also explain the decline in torsion in the face of increased afterload over time. Several researchers hypothesise that the pre-mature myocardium adapts to significant haemodynamic changes and undergoes ventricular remodelling and accelerated subendocardial fibrosis with alteration of rotational strain measures, even mimicking that of the elderly populationReference Breatnach, Levy, James, Franklin and El-Khuffash8,Reference James, Corcoran, Mertens, Franklin and El-Khuffash12,Reference LeGrice, Smaill, Chai, Edgar, Gavin and Hunter15,Reference Bussmann, Smith and Cappelleri38 Further research is needed to explore whether the pre-term myocardium, with its unique myoarchitecture, exhibits increased stiffness despite adaptive compensatory mechanisms.
Clinical implications
These findings may have important clinical consequences in young adults, children, and infants born pre-term. Identifying key mechanistic underpinnings of altered cardiac performance in pre-term-born offspring is an important area of investigation in perinatal origins of adult disease.Reference Kim, Yoon and Lee42 Adults born prematurely have been found to have significantly reduced rotational mechanics, including apical and basal peak systolic rotation rate, and net twist, via cardiac MRI.Reference Buckberg, Hoffman, Mahajan, Saleh and Coghlan43 Recent evidence has now shown that maladaptive pre-term cardiac performance seen in childhood and early adolescence may be first present during a critical window of postnatal development from birth to the first year of age.Reference Aye, Lewandowski and Lamata3,Reference Levy, Patel, Choudhry, Hamvas and Singh23,Reference Patel, Breatnach and James24 Extremely pre-term infants, particularly those who must adapt to significant cardiopulmonary stressors, may have a unique cardiac phenotype that predisposes them to major cardiovascular comorbidities.Reference Burchert and Lewandowski5 Future studies are needed to longitudinally describe changes in rotational mechanics into adulthood and to ultimately optimise long-term cardiovascular risk reduction in pre-term-born offspring.
Limitations
The strengths of this study need to be interpreted within the framework of its limitations. Although this study is the first prospective longitudinal study to track rotational mechanics beyond the neonatal period in pre-term infants, it lacked a comparison term control population to properly characterise the differences and make strong associations. Future studies are needed to compare term and pre-term cohorts to assess long-term effects of pre-maturity on left ventricular rotational mechanics. There is potential selection bias given that 80 of the 117 enrolled infants alive at 32 weeks post-menstrual age returned for follow-up at 1-year corrected age and only 65 of those infants had feasible two-dimensional speckle-tracking echocardiographic images. The studied numbers were small for each group, which precluded us from determining reference values for each gestational age. We chose to compare the asymptomatic group to infants with bronchopulmonary dysplasia, pulmonary hypertension, and/or patent ductus arteriosus because of sample size limitations to provide a sufficiently powered comparison of torsion of neonates with and without patent ductus arteriosus or pulmonary hypertension alone. However, similar to previous power calculations for determining reference values for longitudinal strainReference Levy, El-Khuffash and Patel16,Reference Dong, Hees, Huang, Buffer, Weiss and Shapiro44–Reference Lewandowski, Augustine and Lamata46 and pulmonary artery acceleration time in pre-term infants from birth to 1-year corrected age,Reference Patel, Breatnach and James24 we were able to generate normal maturational patterns and compare trends between uncomplicated infants and infants with bronchopulmonary dysplasia, pulmonary hypertension, and/or patent ductus arteriosus. Although the gold standard modalities for evaluation of left ventricular twist and torsion are three-dimensional speckle-tracking echocardiography or cardiac MRI, the use of two-dimensional speckle-tracking echocardiography, a relatively angle-independent and widely available tool at the bedside, has been validated and demonstrated to be both feasible and reproducible.Reference El-Khuffash, Schubert, Levy, Nestaas and de Boode9
Conclusion
This prospective study establishes reference patterns for rotational mechanics in pre-term infants and tracks their maturational changes over the first year of age. Torsion decreases from 32 weeks post-menstrual age to 1-year corrected age. Pre-term infants that face significant cardiopulmonary stressors have a more pronounced decrease in torsion from 32 to 36 weeks post-menstrual age, but by 1-year corrected age exhibit the same left ventricular torsion as healthy pre-term infants. Rotational strain analysis may aid in characterising myocardial adaptive responses in cardiac function in this population.
Supplementary material
To view supplementary material for this article, please visit https://doi.org/10.1017/S1047951120001912
Acknowledgements
None.
Financial support
This study was supported by grant from the Premature and Respiratory Outcomes Program NCT01435187, (National Institutes of Health grants U01 HL1014650 and U01 HL101794).
Conflicts of interest
None.
Ethical standards
The authors assert that all procedures contributing to this work comply with the ethical standards of the relevant national guidelines on human experimentation of the Belmont Report and with the Helsinki Declaration of 1975, as revised in 2008, and have been approved by the institutional committee of Washington University in St. Louis. Written informed consents were obtained from parents/guardians of study subjects.