Introduction
The fame of the cloud chamber is closely tied to the scientific activities of Charles Thomson Rees Wilson at the turn of the twentieth century. Over a period of almost twenty years, as a researcher at the Cavendish Laboratory in Cambridge, Wilson managed to transform a device used for the study of atmospheric clouds into an apparatus for the study of subatomic particles, in which radioactive emission was visualized as trails of condensed water vapour. Wilson's apparatus made it possible to take pictures of the emission in order to perform in-depth analysis of this phenomenon.Footnote 1 The two communications given by Wilson at the Royal Society in 1911 and 1912 provide both a full account of the apparatus and a total of nineteen pictures which constituted the first visual catalogue of particle events (Figure 1).Footnote 2
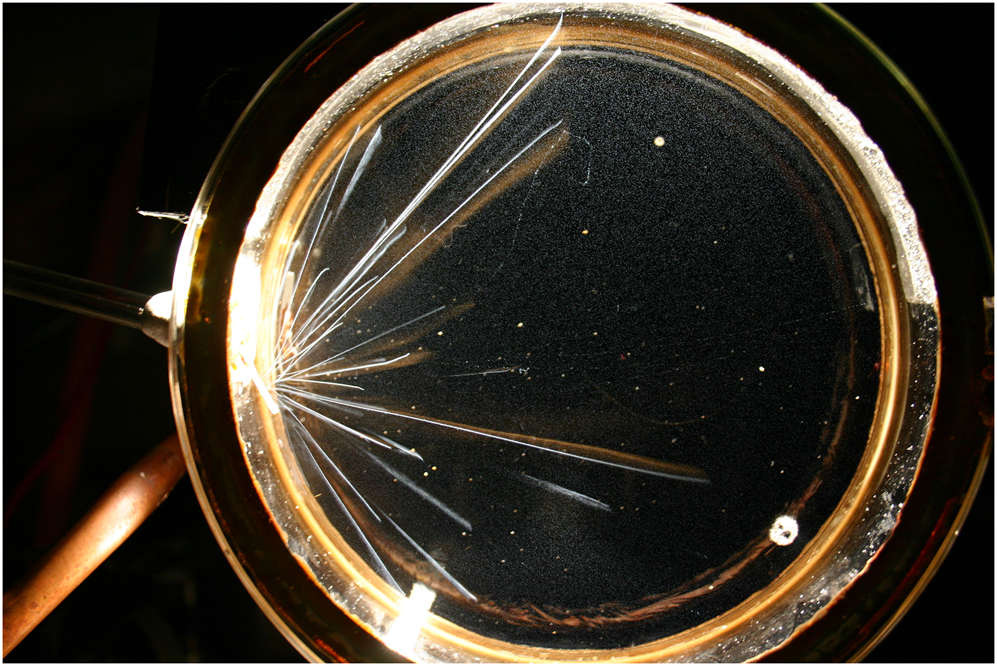
Figure 1. Tracks of beta particles from radium-226 obtained with a full-scale replica of Wilson's cloud chamber. Courtesy Wolfgang Engels.
When in 1927 Wilson was awarded the Nobel Prize, his experimental method was well known within the physics community. However, in many ways the most significant results were still to come.Footnote 3 A report written nearly twenty years later in 1946, by N.N. Das Gupta and S.K. Ghosh in the Review of Modern Physics – a journal providing in-depth essays on current research trends signed by outstanding scientists – shows how the cloud chamber method was not only being used for the study of alpha, beta and gamma rays, but since the 1930s had also been developed for the study of antimatter, artificial disintegration and uranium fission, and was still expected to produce results on new topics, such as the unstable particles discovered in cosmic radiation.Footnote 4
Understandably, the cloud chamber has been the subject of extensive studies within the history of science. With regard to twentieth-century physics, Wilson's instrument has been described as the founder of the ‘image tradition’, an experimental practice based on the search for visual evidences called ‘golden events’, distinguished from the ‘logic tradition’ which was based on statistical analysis and traceable to Geiger–Mueller counters.Footnote 5 Critique of this binary division has led historians to study the images produced by the cloud chamber in even greater depth.Footnote 6 Historians have also related the particle events visualized by Wilson's method to other relevant scientific images in other fields (anatomy, physiology, botany, palaeontology and astronomy) as part of charting the emergence of modern conceptions of objectivity and subjectivity.Footnote 7 In fact, the relationship between Wilson's experiments and ‘objectivity’ has been investigated by means of a full-scale replica of Wilson's original apparatus.Footnote 8 More recent studies offer a biographical perspective on the instrument by relating the numerous discoveries made with the cloud chamber to the technical evolution of the instrument from the end of the nineteenth century to the 1960s.Footnote 9 However, despite the richness of this scholarship, no extensive accounts of the pedagogical role played by the Wilson cloud chamber throughout its history have been provided yet. This is the goal of the present work.
To this purpose, this paper will draw on extant scholarship on the relationship between scientific instruments and pedagogy and will aim to extend their methodological significance. Scholars have shown how many nineteenth-century instruments had a second, didactical career once their research role was over.Footnote 10 These studies now need to be extended to twentieth-century instruments, particularly in the light of the fact that many instruments have not been properly preserved in museums or collections.Footnote 11 It is vital to remember that instruments and equipment matter, not just because of the part they play in creating knowledge, but also because they were and are central to the teaching of the experimental sciences in a variety of educational systems.Footnote 12
In relation to this, it is important to note also that many historical studies on science pedagogy have focused on the role of textbooks. Starting from a critical approach to Thomas Kuhn's view on textbooks as repositories of accepted paradigms, significant attention has been devoted to the role that pedagogy plays in defining and helping to shape new directions in research.Footnote 13 Particularly relevant to the present study is the notion that textbooks encapsulate and organize an inherited body of knowledge, while at the same time, and sometimes at moments of turmoil, integrating that knowledge with emerging theories. This intrinsic tension characteristic of textbooks is relevant to retracing the cloud chamber pedagogical history: when used as complementary sources to the instrument, textbooks made it possible to situate the technical evolution within the broader development of a rapidly changing physics curriculum over the first half of the twentieth century.
The first part of this paper is organized around three case studies focused on different moments of the cloud chamber's pedagogical history and marked by milestone publications in the field: the period before the publication of Wilson's catalogue of cloud chamber pictures (1911–12), the period between this publication and the appearance of the first cloud chamber atlas (1940), and the period between the publication of the first and the second atlases (1940–52).Footnote 14 The first case study focuses on the work of W.N. Shaw in Cambridge and the physics textbook written by J.H. Poynting and J.J. Thomson, and it will show how the cloud chamber was already in use as a demonstrative device for meteorology or gas condensation before the Wilson prototype of 1911. The second case study, dealing with the work of Robert Pohl at the University of Göttingen during the 1930s, shows how the instrument became a major explanatory tool in physics, particularly with regard to conceptual work in atomic physics and quantum mechanics. In the third case study, the paper will examine how physics pedagogy began to reorganize itself around particle physics, the consolidation of which was symbolized by the publication of the atlases and supported by the overhaul of Wilson's cloud chamber by the Officine Galileo company in Florence. The paper will go on to discuss the didactical uses to which the cloud chamber was put after it had been largely retired from front-line research, particularly in the context of large-scale educational projects during the Cold War (for example, the Physical Science Study Committee in the United States). In this final section, the focus on Wilson's instrument illuminates the way in which historical experiments were redesigned in the light of the new teaching methods and paradigms. The implications of these studies for the history and historiography of physics pedagogy are discussed in the conclusion.
A matter of clouds and fog: condensation nuclei and the birth of the cloud chamber
Pedagogical debates are involved from the very beginning of the cloud chamber story. Scholars have argued that the cloud chamber should be seen as the material embodiment of two different approaches, an analytic approach to the study of matter pursued at the Cavendish Laboratory and the mimetic approach to nature developed in England in the late nineteenth century.Footnote 15 According to this view, Wilson managed to transform a device conceived by John Aitken to replicate the formation of clouds into an instrument to probe an artificial phenomenon – the formation of droplets on ions and the visualization of particle tracks. However, an intermediary tradition already present at the Cavendish Laboratory – the meteorological studies carried out by William Napier Shaw – also appears to have played a central role for Wilson's elaboration.Footnote 16
University lecturer and demonstrator William Napier Shaw (together with Richard Glazebrook) was a pioneer in developing laboratory classes for ever-growing numbers of students. They codified their practices in the textbook Practical Physics, which went through four editions between 1885 and 1893 and quickly became Britain's most important laboratory teaching text.Footnote 17 However, it was not the book, but a lecture given by Shaw to the Royal Meteorological Society in March 1895, that first put Wilson in contact with Shaw's pedagogical activity and ideas. A week after the lecture, Wilson described in his notebook the core idea conveyed by Shaw in his lecture – that is, ‘above the clouds the air will be supersaturated but devoid of nuclei’.Footnote 18 In May of the same year, in a one-page paper, Wilson explored this notion with experiments showing that the presence of dust particles is not a necessary condition for the formation of clouds. Moreover, he indicated the ratio between the initial and final volume of the vapour at which the condensation, upon an adiabatic expansion, can be observed: 1.258 at 16.7 °C.Footnote 19
At the time of these results – which didn't permit the visualization of particle tracks but clarified the nature of the condensation nuclei – the cloud chamber was already considered an interesting instrument by J.J. Thomson, then director of the Cavendish Laboratory. According to Thomson's view, electric ions would provide good candidates as condensation nuclei, once the dust particles had been removed. According to this line of thought, when electric ions were removed from the chamber, the condensation of the vapour should not take place at all. This observation was reported by Thomson in an 1898 paper, after using a cloud chamber crossed by Röntgen rays (which would create ions) kept under a strong electric field (which would remove the ions themselves):
If the ions produced by the Röntgen rays act as nuclei for the drops, then, since these ions can be withdrawn from the gas by applying to it a strong electric field, it follows that a cloud ought not to be formed when the air which is expanded is exposed to a strong electric field while the rays are passing through it. This was found to be the case, and the experiment is a striking one.Footnote 20
This observation became the final step of a teaching discourse developed in Poynting and Thomson's textbook around the cloud chamber.
Both Poynting and Thomson did their initial training at Owens College (today the University of Manchester) and specialized at the University of Cambridge, where the two met in 1876. Poynting was one of the second generation of the Maxwellians formed at the Cavendish under Maxwell's direct supervision, and he encouraged Thomson to learn more about the Treatise on Electricity and Magnetism, as well as Maxwell's earlier papers.Footnote 21 Poynting and Thomson's collaboration resulted in a multi-volume undergraduate physics textbook, which covered a vast range of topics and was in print for more than fifty years.Footnote 22 According to the authors, their book was meant ‘for the use of students who lay most stress on the study of the experimental part of Physics, and who have not yet reached the stage at which the reading of advanced treatises on special subjects is desirable’.Footnote 23
Within Poynting and Thomson's didactical presentation of physics, the cloud chamber is used to convey notions of meteorology, condensation and analytical matter theory within a more general thermodynamic framework. In the third volume, for example, dedicated to the subject of ‘Heat’, three chapters out of twenty are dedicated to the topic of state changes. In this context, the cloud chamber is used to discuss condensation of water vapour and to draw attention to the sources of condensation, the ‘condensation nuclei’. Since the authors were writing in the period in between Wilson's early research on condensation and his final work in 1911–12, the volume refers only to the first of Wilson's papers (1895, 1896 and 1899).Footnote 24 Within a dedicated section, condensation is introduced as a surface phenomenon occurring on nuclei of dust or suspended matter other than air or vapour, first discovered by Coulier and later investigated by Aitken. Before any further elaboration, an experiment carried out with a cloud-chamber-like apparatus is described (Figure 2).

Figure 2. Cloud chamber apparatus for obtaining dust-free space in John Henry Poynting and Joseph John Thomson, A Textbook of Physics: Heat, 2nd edn, London: C. Griffin, 1906, p. 168.
Without recourse to quantitative observation, the authors refer to a cloud-chamber-like apparatus as a means of obtaining a qualitative understanding of the relation between the number of dust particles, the dimensions of the drops and the density of the fog. Starting with a base situation in which dust particles contained in regular air are present in the spherical glass bulb whose inner surface is wet with water, the connection with the vacuum made on the bottle-like glass is opened (t 2) to induce the first expansion. With a high number of dust particles available, the drops are small, and the fog is dense. The opening of the connection on the left (t 1) allows new air (purified by the cotton wool filter) to enter the apparatus and to get it ready for a successive expansion. Proceeding with successive expansions, the dust particles become fewer and fewer in number so that the vapour condenses on fewer nuclei. Drops will be larger and the fog less dense, until the point drops are visible ‘like those in a Scotch mist’.Footnote 25 The quantitative part of the section starts with Aitken's method for counting the particles of dust, his apparatus of 1888 and his examinations of the numbers of dust particles in different settings: higher in dry town air than in the country, higher at lower altitudes than on mountains.Footnote 26 With reference to Aitken's work in 1890 the authors report the absolute numbers measured in specific localities: Kingairloch, on the shores of Loch Linnhe, where the number of dust particles ranged from 205 to 4,000 per cubic centimetre; at the top of Ben Nevis, 335 to 473 per cubic centimetre. In London and Paris the number counted a hundred thousand.Footnote 27 Aitken's results are then extended to the data collected around the world, which showed that the number of dust particles at sea level can vary from 200 per cubic centimetre in the Indian Ocean to 4,000 in the Atlantic.
Wilson's work is discussed directly in the analysis of this procedure, where the possibility of condensing vapour in the absence of dust particles (and the hypothesis on the new types of condensation nuclei and their dimensions) is considered. Some elements of Wilson's work on the conditions for ‘condensation without dust’ are then examined by pointing out that condensation takes place according to three regimes bounded by two values of the expansion ratio: no condensation in dust-free air below 1.25, distinct raindrops between 1.25 and 1.37, dense fog above 1.37.Footnote 28 According to the authors, Wilson's observations show that, when the air is completely empty of dust, condensation starts to take place around other condensation nuclei, and these ‘new’ condensation nuclei grow (in number) and diminish (in dimensions) as soon as the regimes are crossed. However, no hypothesis on ions or electrons is explicitly made. Poynting and Thomson limit themselves to the suggestion that one can hardly suppose that the nuclei are of foreign matter, meaning that, in this condition, condensation cannot be accounted for by the accidental entry of dust particles. Wilson's observations that the effect of condensation was increased when ultraviolet (and Röntgen and uranium) rays crossed the ‘dust-free’ chamber are used as the closing remarks for the section.Footnote 29
In their account, starting from a meteorological perspective with the understanding of the different types of air and fog, Poynting and Thomson move to the physics of condensation established by Wilson's experiments and elaborate on the different size of drops and number of condensation nuclei through the different regimes of expansion. The exposition does two things – integrating Wilson's latest results with a solid foundation in meteorological studies, and using the cloud chamber as a means of extending and illustrating Shaw's account of the subject.
Magnifying and detailing: three-metre-long tracks for observing the electron
The second case study of this paper is drawn from the ‘golden age’ of the cloud chamber, when it was applied to the study of a wide range of physical topics. However, this period didn't start immediately on the publication of Wilson's 1911 and 1912 papers. Despite the enthusiasm with which Wilson's 1912 pictures were received, the technical method took some time to be appreciated and appropriated by the physics community. For about ten years, Wilson's pictures were the main (if not the only) visual material through which physicists ‘projected’ their understanding of atomic processes. In 1914, for example, Ernest Rutherford saw them as convincing evidence of the correctness of his view that large deflections do occasionally occur as a result of an encounter with a single atom, while in 1915 Kovarick and McKeehan in America found that Wilson's pictures confirmed their own results from electrical counting methods.Footnote 30 But from the 1920s, this changed as practical research with the cloud chamber method began in Germany, the United States, India and South Africa. Over the course of a few years, discoveries such as the first observation of the transmutation of nitrogen, the identification of the ‘Compton effect’ in 1925 and the discovery of the positron in 1932 all heralded the onset of the instrument's ‘golden age’.
During this interwar period, large-scale experimental demonstrations were conducted by Robert Wichard Pohl, a professor of experimental physics at the University of Göttingen in Germany. Pohl worked according to a specific teaching paradigm, where any explanation had to start from ‘facts’ and not ‘theories’. Lectures and demonstrations were central elements of academic physics teaching in Germany in the early twentieth century. Carefully prepared and staged and heavily relying on the individual abilities of the demonstrator/professor, they were considered to be not just a teaching technique, but also a form of art. Within this framework, Pohl became famous for his approach, which was based on the epistemology of simplicity demonstrated through a set of specially designed instruments (for example, the rotating chair to show angular momentum and centrifugal forces), as well as a novel series of physics textbooks. By establishing a collaboration with instrument-making companies (such as Spindler & Hoyer and Leybold Nachfolger), Pohl's system became a highly influential rigorous modular standardized system in Germany.Footnote 31
Particularly illustrative of Pohl's approach was a cloud chamber capable of projecting three-metre-long tracks on an auditorium's screen in front of four hundred students. The instrument was made of a circular chamber twenty centimetres in diameter made of glass which stood in a vertical position and where the condensation of the vapour was induced by means of a membrane (‘pressure-driven mechanism’). Furthermore, being entirely crossed by light, the chamber was placed between two lenses and transformed into the ‘diapositive’ of a modern projector. The apparatus, connected to a vacuum pump, could be activated every ten seconds and produced three-metre-long tracks projected on the auditorium's screen.Footnote 32
Notably, the technical adjustments made by Pohl to magnify and project the tracks on a screen were based on the same technical strategy (‘the pressure-driven mechanism’) used by Cambridge physicists to pursue one of their research goals – identifying the positron in cosmic rays. By the end of the 1920s, cosmic-ray researchers had started to revise the 1911 original version of the cloud chamber to allow for more and more sophisticated observations. The more significant adaptation in this respect was the ‘Geiger-controlled cloud chamber’ developed in 1932 by Patrick Blackett and Giuseppe Occhialini in Cambridge, where the chamber stands in a vertical position while two Geiger counters placed above and below the chamber signal the passage of a cosmic ray particle and trigger the light flash and the camera. This was, in fact, a method in which the cosmic particles are led ‘to take their own photograph’, as the two authors defined it in a letter to Nature.Footnote 33
Although the Geiger-controlled system improved the efficiency of taking the photograph, it didn't solve the problems of tracks being formed through a process of condensation induced by the falling of the heavy piston. The movement of the piston was easily subjected to mechanical friction, which could slow the fall and compromise the quality of visualization, especially when the chamber stood in a vertical position (to offer the cosmic rays the cylinder's entire volume) and consequently the piston moved in a horizontal direction. That was the reason why, in 1933, Wilson replaced the piston with a plastic membrane and induced the vapour's condensation by reducing the pressure within the chamber instead of increasing its volume (‘pressure-driven mechanism’).Footnote 34 The removal of mechanical friction was the last step in the tuning of the method, which remained unaltered throughout the whole ‘golden age’.
In 1935 Wilson described a cloud chamber of radial form standing in a vertical position working according to a pressure-driven mechanism, whose shape was particularly suitable for inserting the chamber within the two poles of a magnet.Footnote 35 This ‘second’ Wilson instrument is almost identical to the one that – according to to Pohl's assistant Rudolf Hilsch in 1939 – had been in pedagogical use for the past five years at the University of Göttingen.Footnote 36
Robert Pohl, who, together with Max Born and James Franck, had been one of the most influential figures in physics at Göttingen University and would go on to be a leader in solid-state physics, remained in his position at the University of Göttingen throughout the period of the Third Reich.Footnote 37 Immediately after the war, required by the military forces to justify his position, he claimed that his role as consultant to the Luftwaffe's research leadership had allowed him to keep science alive in Göttingen, continuing his didactical projects and the writing of his pedagogical textbooks while maintaining his distance from political events.Footnote 38 He tried to manage a similar distance from the politically motivated debate over the Anschaulichkeit which began with Philipp Lenard's attacks on Albert Einstein. As is well known, at the eighty-sixth meeting of the German Society of Scientists and Physicians held in Bad Nauheim, on 23 September 1920, Lenard argued that the principle of relativity had destroyed an intuitively vivid – anschaulich – picture of nature which should be rooted in experimental observations and not descend from theoretical principles.Footnote 39 Einstein's robust response – that what is considered anschaulich changes over time, and that physics should be considered more conceptual than anschaulich – did not end the debate, which was motivated by the racist ideology that was to become deutsche Physik.
While Pohl accepted the primacy of experiments over theories, his concept of Anschaulichkeit resonated beyond the ordinary understanding of deutsche Physik, for two reasons at least. The first lies in his educational focus and his belief that an impressive scientific demonstration (like the projection of particle tracks across an auditorium) was vital for enhancing student understanding. In this regard, in 1974, Pohl's former doctoral student and assistant Heinz Pick recalled another dramatic demonstration performed in the Göttingen auditorium, the visualization of the diffraction patterns of crystals (today known as ‘Laue diagrams’). Pick's account of the demonstration focused on the underlying philosophy, where ‘to show’ something meant to make it possible for the audience to see and judge phenomena with their own eyes as they occurred in real time. Pictures simply weren't good enough.Footnote 40
The second thing which characterized Pohl's Anschaulichkeit was the belief that theoretical concepts must emerge from empirical experiment, and that theorizing was an activity to be undertaken only with caution. However, it is also clear from the textbooks that Pohl's dictum that ‘theories come and go, but facts remain’ should not be understood as an exclusion of theoretical elements from his teaching. Classical experimental topics such as radioactivity and cosmic rays, as well as highly theoretical concepts such as particle–wave dualism, were not avoided, but actively introduced on an experimental basis in Pohl's textbooks. As will be shown in the next section, Pohl's complex interrelation of theorical and experimental elements is similar to that shown by other scientific figures of the time, like Patrick Blackett, and can be illustrated by two examples.
The corpuscular view of matter: observing electrons and ions
In Pohl's account of radioactivity provided in Chapter 10 of the volume on electricity, the ionic theory hinted at by Poynting and Thomson is developed in detail.Footnote 41 Starting from a generic radioactive emission, Pohl shows how three different components (alpha, beta and gamma) can be separated and detected with electrical methods. The experiment consisted in placing a radium source covered with a two-millimetre aluminum foil at two metres distance from an ionization chamber with a 0.1 millimetre hole as a unique point of access. Nonetheless, Pohl points out that an electroscope placed inside the chamber itself would signal the presence of some radiation and argues that only gamma rays can travel such a distance. The detection of beta rays proceeds in a similar manner. In this case, when the radium source approaches to within twenty centimetres the current circulates, indicating that another kind of ray is present, travelling a shorter distance through air. The cloud chamber is then introduced to resolve the rays in single particles. Here a direct comparison between alpha particles (ions) and electrons is carried out starting from the unmistakable signature which they leave in the chamber and following the line of reasoning developed by Wilson in his 1912 paper: tracks of the alpha particles are straight, continual and thick, and they can be observed emitted directly from the source. Electrons can be observed as a secondary product: once X-rays pass through the chamber volume, the tracks of the electrons emitted by the atoms of the chamber become visible as irregular, curved and ornate twines. The difference in thickness is due to the difference of mass, which for the electron is 7,200 times smaller than that of the alpha particles.
The corpuscular view of light: the ‘quanta’ of energy
The corpuscular view of light as ‘photons’ is discussed by Pohl in Chapter 13 of his textbook on optics, dedicated to the wave–particle dualism.Footnote 42 After a brief historical introduction focusing on the corpuscular view of Newton, Pohl stresses the importance of the hypothesis introduced by Planck on the minimum quanta of energy. This explanation of the existence of light quanta – photons – starts from a fundamental observation performable with the cloud chamber which leads to the abandonment of the wave-like hypothesis. When the chamber is crossed by X-rays emitted from a Röntgen lamp and provoking the emission of electrons from the atoms, one can calculate that the electrons’ energy varies between 104 and 105 eV. Moving from the experimental to the theoretical level, Pohl remarks that, should this energy be emitted by the Röntgen lamp as a uniform distribution in space, it would take months for the electrons of the atoms to accumulate such an amount of energy (Pohl discussed the point by taking into account the power of a normal Röntgen lamp, the dimensions of the molecules and the volume of the irradiated chamber). Then he remarks how, in order to explain these and other phenomena, a localized distribution of energy (the corpuscular view) must be considered.
The notion is then examined through an experiment carried out by Walter Bothe and Hans Geiger in 1924. This experiment confirmed the Compton scattering observed of 1922 and so Einstein's hypothesis of light quanta. An X-ray radiation is emitted from the centre in the left and right direction of a cloud chamber containing argon. At the opposite wall two counters are ready to signal receiving the radiation. As the radiation propagates, its interaction with the atoms of the chamber becomes visible. Pohl's analysis draws students’ attention to the two ‘experimental facts’ which lead to the photon's introduction: as the experiment proceeds and the radiation is emitted, the two counters never coincide. This fact demonstrates that the emission doesn't follow wave-light behavior, it is not isotropic, but it does have a preferential direction.Footnote 43 Preferential direction in emission is only one step toward the identification of the photon, so Pohl turns to the observation of the tracks which form during the propagation: they are not a continuous line, but have a granular structure and are made by dots. Moreover, their vividness decreases while they extend from the centre to one of the sides. The interaction is then discrete, and the loss of vividness testifies the exchange of energy between the electron of the gas and the photons of the radiation.
Pohl's interweaving of experimental and theoretical information, and his approach of combining pedagogy with cutting-edge research, were maintained throughout the successive editions of his textbooks. New discoveries, such as the pair productions of electrons and positrons, were integrated and discussed with extant knowledge. A few years later, the capacity to link physics pedagogy to milestone discoveries would be even more enhanced by the two atlases that emerged from the cloud chamber research community.
Recognizing tracks and scrutinizing processes: the atlases of the cloud chamber
The first atlas, published in Germany in 1940, was a compendium of the most relevant pictures associated with the cloud chamber, such as the transmutation of nitrogen observed by Blackett in 1925 and the first track of the positron obtained by Anderson in 1932.Footnote 44 The second volume, published in 1952, was dedicated to cosmic-ray research and reported the first picture of a cosmic ray taken at sea level (Leningrad, 1929) and the highest-energy ray ever seen, taken at an altitude of 3,027 metres (Echo Lake, 1949).
The fact that the first atlas was published in Germany at the beginning of the Second World War may seem strange in light of the fact that it drew very heavily on the work of British physicists. But this reveals the strength of the scientific network which had formed around cloud chamber studies in the interwar period. Through this network of people and laboratories, a variety of methodological strategies had circulated, including the coincidence method, introduced in 1927 by Walther Bothe in Germany, and developed further in 1929 by Bothe, Werner Kolhörster and the Italian physicist Bruno Rossi. This last innovation involved including Geiger counters in the coincidence system in order to make it suitable for studying the penetrating charged particles of cosmic rays. The coincidence method was then applied to the cloud chamber by Patrick Blackett in Cambridge and Rossi's student Giuseppe Occhialini for the previously mentioned positron studies. German studies on the cloud chambers continued to develop systematically in the 1930s alongside Bothe's interest in cosmic rays. Thanks to Bothe's assistant Maier-Leibnitz, Bothe's research group, which included Wolfgang Gentner, set up a cloud chamber research programme at the Institute of Physics in Heidelberg.
Both the atlases were conceived with a particular pedagogical aim: by carefully scrutinizing the thickness, curvature and length of the tracks and applying the conservation laws of physics, young researchers were to learn how to distinguish the ‘already known’ from potential new discoveries. The pedagogical value of the atlases is made explicit by P.M.S. Blackett, who received the 1948 Nobel Prize in physics for the development of the cloud chamber method. In the preface to the second volume, Blackett states explicitly that the atlas
must surely help us to make clear that this world of subatomic events is one which can be easily visualized and understood without the aid of complicated mathematics or mastery of deep theories. If one asks why these complicated events happen, one may be led into the subtle intricacies and uncertainties of modern fundamental theoretical physics, but if the experimenter contents himself with asking how they happen, then these pictures, and the attached commentaries, are an ideal guide to the world of elementary particles.Footnote 45
One may note an echo of Robert Pohl and his ‘epistemology of simplicity’ here in Blackett's preface. Still, just as Pohl's textbook revealed a complex interrelation of experimental and theoretical elements, so Blackett's attitude toward theories wasn't as simplistic as it might seem. In 1948, Blackett was just at the beginning of his study of the Earth's magnetism, an endeavour which demonstrated his willingness, and his capacity, to pursue bold theoretical hypotheses.Footnote 46 While at Cambridge and after the establishment of Bohr's institute in Copenhagen in 1920, Blackett had seen theoretical physics beginning to break down the walls of the fortress of experimental physics – aka the Cavendish Laboratory directed by Rutherford. Theoretical work gained legitimation thanks to Gamow's mathematical theory of the atomic nucleus based on wave mechanics, and steadily began to increase its importance and identity as a research field.Footnote 47 In 1933, however, Blackett had drawn attention to the fact that the increasing abstraction of physical theories was making it more and more difficult for the experimentalist to follow developments in theoretical physics. This fact had remarkable consequences: ‘Today’, he concluded, ‘an experimenter cannot always be expected to understand fully the theoretical implications of his work, and must hand over half his job, and that the most fascinating to his intellect, to the mathematical physicist’.Footnote 48
Among the physicists who certainly took Blackett's perspective and considered the atlases a central pedagogical tool for physics teaching was Carlo Ballario, at the time professor of experimental physics at the University of Bologna. In fact, Ballario was asked by the Florentine scientific instrument company Officine Galileo to design a revision of the Wilson cloud chamber specifically for the school market, and it is this instrument which is the focus of this paper's third case study.Footnote 49 What makes this apparatus particularly meaningful is that, on the one hand, as with all the other pedagogical apparatus for introducing school students to particle physics, it was simple to operate. On the other hand, unlike any other didactical apparatus, it was extremely precise and high-performing. It could show the students the atomic processes as they were portrayed in the atlases.
To achieve this double goal – simplicity and precision – the revision of the Wilson instrument carried out by Ballario relies on the heavy use of electronics as a means of simplifying the use of the device: one might say that the instrument reduces the whole technical evolution of the Wilson method to the pressing of a button and the turning of a knob (Figure 3). The chamber is crossed by an electric field to remove the dust particles and ensure that the vapour condenses only on the ions created by the radioactive source placed within, as with Wilson and Thomson in the 1896 experiments. The expansion of the vapour takes place according to the pressure-driven mechanics introduced by Wilson in 1933; the volume of the chamber is circular and surrounded by circular magnets to deviate charged particles, as done by cosmic-ray physicists since the 1930s. As with Wilson's prototype of 1912, the expansion is connected to a camera and a flash of light. When the expansion takes place, a magnetic field is switched on inside the chamber in order to deviate charged particles according to their sign. Thanks to electronics, all this is done by the press of a single button. The second element is a knob, the turning of which controls the expansion of vapour and allows the operator to tune the instrument to atmospheric conditions.

Figure 3. Wilson cloud chamber according to Ballario and produced by Officine Galileo in 1948. The circular illumination surrounding the observation chamber is visible. Copyright © Fondazione Scienza e Tecnica, Florence.
Ballario's revision of the Wilson instrument tried to put students and teachers on a par with laboratory researchers when it came to observing the details of particle processes, aiming for the highest fidelity it was possible to obtain without the need for individual expertise. But at the same time, the sophistication makes the instrument delicate and possibly difficult to handle. This is probably the reason why, even though it was produced and distributed between 1959 and 1962, it was relatively quickly taken off the market. In a 1964 catalogue of the company, the Ballario teaching cloud chamber – described as a ‘Wilson chamber’ – appears just after another teaching model, one where a condensation in tracks is obtained by pressing a lever, and which seems much easier to use.Footnote 50
But in order to understand the pedagogical principles which lay behind the construction of this specific instrument, particularly interesting are three documents on the instrument conserved among the Ballario papers and belonging to the correspondence between the physicist and the instrument company.Footnote 51 Undated and incomplete (two of them are missing important figures), they can, however, be related to a request for explanatory material required by the company for marketing purposes. The first document contains an extremely detailed description of the different parts of the apparatus, on which the first part of this section is based. The second document provides a technical account of the instrument based on a historically oriented division: as a cosmic-ray physicist, Ballario distinguishes a Wilson cloud chamber ‘for radioactivity’ in which expansions occur at regular intervals of time from a ‘Wilson chamber for cosmic rays’ which are controlled by the Geiger counters and would expand only in the case of the passage of a cosmic-ray particle. The third document, however, is a didactic illustration of the use of the instrument. Ballario describes the way in which his goal behind the development of the instrument was to bring into the classroom ‘the daily effort that experimental physics endures every day to provide evidences to the great theoretical frameworks’. He details the technical procedure for maximizing both the performance of the instrument and its capacity to display bright tracks of the phenomena. Then he lists a series of processes reported in the atlas of 1940 as possible outcomes of the experiments: the scattering of alpha particles with helium (p. 51, 6b) and hydrogen (p. 51, 7b), the passage of relativistic particles through two centimetres of copper (p. 99, 48b), the production of secondary electrons by relativistic particles in the gas (p. 105, 53b), and thin particle showers (p. 120, 68c).Footnote 52
In this way, it is possible to see how the publication of the atlases and the emergence of particle physics as a distinct research sector had an impact on the teaching of physics. In fact, a few years later, this area of physics would take a stable place in physics curricula and textbooks. However, the observation of more and more sophisticated phenomena would not be the main channel through which such concepts would be explained to students.
The last section of this paper will deal with the period in which the cloud chamber became ‘history’; that is, the period when it no longer played an active role in research practice. The discovery of unstable particles in 1947 proved that ideas both about cosmic rays as particle sources and about the cloud chamber as detector should be revised: while unstable cosmic radiation was to be replaced by reliable particle accelerators, the bubble chamber became a much better candidate for the study of the nuclear phenomenology.Footnote 53 Wilson's idea of the condensation of vapor around the path of particles was then replaced by Donald Glaser's observation that a liquid could boil around the same path, both visualizing particle tracks and offering, at the same time, a higher probability of nuclear reactions.
From a specialized instrument to a school and mass instrument
The immense changes in science which followed the end of the Second World War and the escalation of the Cold War also had profound implications for scientific education and the associated market of scientific instruments. Escalations of big scientific events (like the launching of the USSR satellite sputnik in 1957) galvanized the US government into launching an unprecedented programme to reform pre-college science education by involving top scientists.Footnote 54 New educational programmes – such as the Physical Science Study Committee inaugurated at MIT in 1956 – were expected to review introductory physics education and to design, implement and monitor improvements based around the production of new textbooks, instructional movies and classroom laboratory material. Accordingly, new didactical equipment was also designed to support the reformed curriculum. As previous historians have noted, by the late twentieth century, physics teaching in high schools and colleges had hardly anything to do with the phenomenological and descriptive science of the nineteenth and early twentieth centuries.Footnote 55
The new trend also saw a diffusion of restyled historical instruments, such as the cloud chamber. They were designed and marketed on a grand scale by instrument companies and not produced as limited (if not unique) exemplars, as in the cases discussed above.Footnote 56 In order to reach a wide range of users among teachers and students, the new apparatuses were based on the simplest cloud chamber principles, thought to be entirely known and no longer the object of research: the volume-driven mechanism introduced by Wilson in 1911 and the diffusion mechanism introduced by Alexander Langsdorf in 1939.Footnote 57 The new instruments look quite different from the sophisticated apparatus of the first half of the century, conceived by university professors and often operable only by themselves or by their inner research circle (Figure 4). Still, as this section will show, the simplification of devices did not necessarily mean a simplification of teaching.

Figure 4. Wilson cloud chamber produced by Leybold since 1959.
The restyling of such instruments took place in a general revision of the teaching methods and conceptions and, more specifically, in an educational redesign of the major experiments, in order to explicate and emphasize critical details necessary for student understanding. In one of the many educational movies produced within the American Physical Science Study Committee (PSSC), the cloud chamber is used in this respect within a reconstruction of the Rutherford gold foil experiment. This celebrated experiment, which put forward the foundational argument for the existence of the atomic nucleus, was performed by means of an alpha-particle gun, gold foil and a scintillation screen. By observing the deflections of the alpha particles as they passed through the foil and released their energy on the screen, Rutherford and collaborators could identify the existence of a very small region where the positive charge would have been concentrated. The ‘restyled’ version proposed by the PSSC exploited a cloud chamber which, posed between the gold foil and the screen itself, made it possible to ‘directly see’ the deflection of particles from the horizontal direction of propagation.
Historical pictures which had been published in the atlases also became a pedagogical subject at school. In the Project Physics Course (PPC), active from 1962 in the United States, the historical analysis of Blackett's pictures of the transmutation of nitrogen taken in 1925 and published in the 1940 atlas is developed into a full explanation.Footnote 58 Following the line of Blackett's original argument, where four tracks in the picture mean ‘disintegration’ while three mean ‘integration’, the attention of students is drawn to the specific characteristics of the tracks that enable the identification of an alpha particle, a proton and an ‘unknown’ nucleus in which the nitrogen has transmuted. These considerations are then formalized into a mathematical relation – an equation, expressing the balance between some quantities before and after the calculation. The knowledge of these quantities – atomic number, mass number and nuclear charge for three of the four entities involved – for the nitrogen, the alpha particle and the proton – allows the student to identify the nucleus in which the nitrogen transmuted: the third track on the picture is identified as an isotope of the oxygen with atomic number eight and mass number seventeen. A cartoon-style diagram in the margin of the textbook illustrates the entities tracked by the cloud chamber as a small and a big ball transforming into a big and small ball travelling in different directions.
A compendium on the teaching applications of the Wilson instrument published in Germany in 1976, besides giving practical advice on the construction of artisanal cloud chambers, presents a collection of very detailed pictures of alpha particles, protons, neutrons, electrons and Röntgen rays, and by means of forty-one guided exercises discloses the entire range of possibilities which are opened up for the teaching of particle physics by this collection.Footnote 59 Analogous with Das Gupta and Ghosh's 1946 Review of Modern Physics report, the 1976 publication indicates the extent to which the pedagogic capacities of Wilson's method have been explored over the course of the whole century. Nowadays, it is not difficult to find cloud chamber pictures in contemporary textbooks in an opening discussion of quantum mechanics, accompanied by comments – as with Pohl – on the dual nature of radiation. Despite Blackett's warning, they are really used to introduce students to ‘the subtle intricacies and uncertainties of modern fundamental theoretical physics’.
Conclusions
This history of the pedagogical use of the Wilson cloud chamber has uncovered a dynamic relation between the research and pedagogical usage of the instrument, from the early 1900s to the 1960s. This dynamic has been investigated between the three main terms of the material culture of the cloud chamber: the apparatus, the cloud chamber pictures and pedagogical textbooks.
As far as the apparatus is concerned, this paper has shown that the technical development of the Wilson method as particle detector coincided with a series of didactical experiments and that for an extended period of time the two domains interacted with each other. From the apparatus for clouds used by Thomson and Poynting – which could hardly fall into one distinct category – and the standardized and simplified devices marketed by Leybold – easily to label as ‘didactical’ – Pohl's and Ballario's teaching revisions of the Wilson instrument contained technical knowledge which competed with, if it did not advance, developments in research. In this regard, the present paper has suggested that the overlaps between traditionally separated historiographical categories such as research and pedagogy may not be sustainable. Instead, perhaps the interaction between the two should be looked at as a historiographical category in itself.
As for cloud chamber pictures, the paper has shown how this visual source displayed a longevity which was independent of the apparatus that produced it, whether in research or in didactical laboratory practice. It is not unusual to find Wilson's or the atlases’ cloud chamber pictures in modern school and university textbooks, sometimes symbolic of that chapter in physics history, but sometimes also still used as an explanatory tool. The persistence of successful graphical representation in twentieth-century physics has been investigated in relation to Feynman and Minkowski diagrams.Footnote 60 The cloud chamber pictures share many features with these visual tools, a certain ‘ease of acquisition’ and the need for careful management of physical interpretation. The present paper has shown how possible reasons for the longevity and persistence of cloud chamber pictures in the teaching context lie in their versatility when used for teaching purposes. If Blackett and the Cambridge physicists looked at the cloud chamber pictures to test new, evolving theories (as it was for the positron in 1932 when cloud chamber pictures were determinant for confirming Dirac's theories on antimatter), the scientists–teachers looked at particle tracks as an opportunity for crafting new explanations. The effect of the condensation of vapour in tracks – the special capacity of the Wilson instrument – has been exploited very much beyond its research scope and used for introducing the ion hypothesis, discussing the electric charge of the electron and justifying the photon hypothesis, as well as nuclear particles and processes. This capacity for extracting new meanings from the same material emerges as a peculiar activity of the pedagogical labour performed with the sole goal of making cognitive models meaningful to students.
Acknowledgments
The research has been supported by the Alexander von Humboldt Foundation within the Research Fellowship for Postdoctoral Researchers programme and has been carried out at the Europa-Universität in Flensburg, Germany. My grateful thanks to Peter Heering, Paolo Brenni, Richard Staley, Wolfgang Engels and Roland Wittje for the precious discussions on the subject. Special thanks to the BJHS editorial team and the two anonymous referees for their knowledgeable advice and indispensable observations.