Significant outcomes
-
• Cannabidiol as antidepressant.
-
• Cannabidiol presented antidepressant-like effects on the forced swimming test.
-
• Cannabidiol increased BDNF levels in the rat amygdala.
Limitations
The dose of cannabidiol that presented effects on the behaviour test was not the same that presented effect on brain-derived neurotrophic factor (BDNF) levels.
Introduction
Major depression is a serious and disabling psychiatric illness that affects approximately 17% of the population worldwide and has a significant negative impact on public health and productivity (Reference Baune, Adrian and Jacobi1,Reference Mathers and Loncar2). The current clinically used antidepressants increase the extracellular concentrations of monoamines serotonin or norepinephrine either by inhibiting their reuptake from the synapse or by blocking their degradation by inhibiting monoamine oxidase (Reference Castrén3–Reference Moreira and Guimarães5). However, the currently used antidepressant drugs show therapeutic efficacies in a maximum of 60–70% of depressive patients, thereby research has been undertaken to find alternative antidepressive treatments (Reference Souery, Amsterdam and de Montigny6,Reference Tomba, Grandi and Fava7).
The plant Cannabis sativa has been used for many centuries. It is known to have therapeutically relevant properties and 400 different identifiable chemical constituents; more than 60 of them are cannabinoids (Reference Gaoni and Mechoulam8). The two main cannabinoids are delta-9-tetrahydrocannabinol and cannabidiol. Cannabidiol is known to antagonise the anxiogenic and psychotomimetic effects of high doses of delta-9-tetrahydronannabinol (Reference Zuardi, Shirakawa, Finkelfarb and Karniol9,Reference Zuardi, Crippa, Hallak, Moreira and Guimarães10). Cannabinoids exert their effects by interaction with specific endogenous cannabinoid receptors (CB). The CB1 receptor is expressed predominantly in central nervous system, in areas that can mediate most of the effects on cognitive function, pain and short-term memory (hippocampus and cerebral cortex), motor control and coordination (basal ganglia and cerebellum), hypothermia and hyperphagia (hypothalamus) (Reference Pertwee11), and CB2 expression is restricted to immune cells, T-cells, B-cells, spleen, tonsils and activated microglial cells (Reference Herkenham, Lynn, Johnson, Melvin, de Costa and Rice12–Reference Tsou, Brown, Sanudo-Pena, Mackie and Walker14).
Cannabidiol is a drug with multiple mechanisms of action (Reference Zuardi15), including anti-inflammatory effects (Reference Costa, Colleoni and Conti16–Reference Walter, Franklin and Witting18), antioxidative and anti-cancer actions (Reference Hampson, Grimaldi, Axelrod and Wink19–Reference Mechoulam, Parker and Gallily21), neuroprotective effects (Reference Valvassori, Elias and de Souza22–Reference El-Remessy, Khalil and Matragoon24), regulation of intracellular Ca2+ levels (Reference Ryan, Drysdale, Lafourcade, Pertwee and Platt25) and it ameliorates the manifestations of diabetes (Reference Weiss, Zeira and Reich26,Reference Durst, Danenberg and Gallily27). In addition, cannabidiol is known by the action on ischaemia (Reference Carlini, Leite, Tanhauser and Berardi28), antiepileptic (Reference Izquierdo, Orsingher and Berardi29,Reference Turkanis, Cely, Olsen and Karler30) and antipsychotic actions (31–34) and anxiolytic effects (35, 36–41), these effects were observed in animal models, as well as in humans. Moreover, it has recently been suggested that the endocannabinoid system may be involved in the pathophysiology of depression (42–45) and that cannabidiol may have agonist properties at 5-HT1A receptors (Reference Joca, Padovan and Guimarães46,Reference Karege, Vaudan, Schwald, Perroud and La Harpe47), which have been related to the therapeutic effect of antidepressant drugs (Reference Shirayama, Chen, Nakagawa, Russell and Duman48).
Several studies have pointed to the role of BDNF in major depression. In fact, decreased levels of BDNF have been shown in animal models of depression and in patients with depression (Reference Nibuya, Morinobu and Duman49,Reference Siuciak, Lewis, Wiegand and Lindsay50). Conversely, administration of antidepressant treatments increases BDNF expression (Reference McArthur and Borsini51) and brain infusion of BDNF produces antidepressant-like actions in rats (Reference Siuciak, Lewis, Wiegand and Lindsay50,Reference McArthur and Borsini51).
Thus, the main objective of this study was to evaluate behavioural and molecular effects induced by acute and chronic administration of cannabidiol, imipramine or saline (control group) in rats. The behavioural effects of both drugs were evaluated in the forced swimming test, which is a valid behavioural despair assay widely used for screening antidepressant drugs (Reference Detke, Rickels and Lucki52). The BDNF protein levels were measured using an enzyme-linked immunosorbent assay (ELISA) kit in rat prefrontal cortex, hippocampus amygdala acutely and chronically treated with cannabidiol, imipramine or saline.
Materials and methods
Animals
Male adult Wistar rats (60 days old) were obtained from Universidade do Extremo Sul Catarinense (Criciúma, SC, Brazil) breeding colony. They were housed five per cage with food and water available ad libitum and were maintained on a 12-h light/dark cycle (lights on at 7:00 h). All experimental procedures involving animals were performed in accordance with the NIH Guide for the Care and Usage of Laboratory Animals and the Brazilian Society for Neuroscience and Behavior (SBNeC) recommendations for animal care and with approval by local Ethics Committee under protocol number 94/2009.
Drugs and treatments
Cannabidiol was obtained from THC-Pharm/STI-Pharm (Frankfurt, Germany) and imipramine, the standard antidepressant, from Novartis Pharmaceutical Industry (São Paulo, Brazil). Different groups of rats (n = 15 each) were administrated intraperitoneally with saline (control group), imipramine (positive control) (30 mg/kg) or different doses of cannabidiol (15, 30 and 60 mg/kg) (Reference El-Remessy, Khalil and Matragoon24) one single time (acute treatment) or over 14 days, once a day (chronic treatment) 60 min before the test sessions. Imipramine was dissolved in saline solution and cannabidiol was suspended in polyoxythylenesorbitan monoleate (Tween 80) 2% saline. All treatments were administrated in a volume of 1 ml/kg. Rats were tested in the open field and forced swim tests following acute or chronic saline, imipramine and cannabidiol treatments.
Forced swimming test
The forced swimming test was conducted according to previous reports (53–56). The test involves two individual exposures to a cylindrical tank with water in which rats cannot touch the bottom of the tank or escape. The tank is made of transparent Plexiglas, 80 cm tall, 30 cm in diameter and filled with water (22–23°C) to a depth of 40 cm. In the acute treatment, for the first exposure, rats without drug treatment were placed in the water for 15 min (pretest session). Twenty-four hours later, rats were placed in the water again for a 5-min session (test session). Rats were treated with cannabidiol, imipramine or saline only 60-min before the second exposure to the cylindrical tank of water (test session). During the test session, some behavioural parameters were recorded in seconds, such as immobility time (i.e. no additional activity is observed other than that required to keep the rat's head above the water), climbing time, which is defined as upward-directed movements of the forepaws along the side of the swim chamber, and swimming time (i.e. movement usually horizontal throughout the swim chamber).
In the chronic treatment on the 13th day of chronic treatment, 1 h after drug treatment, rats were individually placed in the cylinder containing water for 15 min (pretest session). On the 14th day, rats received the last intraperitoneal drug treatment and after 1 h they were subjected again to the forced swimming test for a 5-min session (test session) and the immobility, climbing and swimming time of rats were recorded in seconds.
Open-field test
This apparatus consists of a brown plywood arena 45 × 60 cm surrounded by wood 50 cm high walls and containing a frontal glass wall. The floor of the open field was divided into nine rectangles (15 × 20 cm each) by black lines. Animals were gently placed on the left rear quadrant and left to explore the arena. In a separate series of experiments, naÏve rats were acutely treated with cannabidiol (15–60 mg/kg), imipramine (30 mg/kg) and saline 60 min before the exposure to the open-field apparatus. In the chronic treatment on 12th day, rats were exposed to the open-field apparatus. The number of horizontal (crossings) and vertical (rearings) activities performed by each rat during 5-min observation period were counted by an expert observer, in order to assess possible effects of drug treatment on spontaneous locomotor activity.
BDNF analysis
Immediately after the forced swimming test saline, imipramine and cannabidiol-treated rats were killed and the skulls were removed and prefrontal cortex, hippocampus and amygdala were dissected and stored at −70 °C for biochemical analysis. BDNF levels in prefrontal cortex, hippocampus and amygdala were measured by anti-BDNF sandwich-ELISA, according to the manufacturer's instructions (Chemicon, Temecula, CA, USA). Briefly, the rat prefrontal cortex, hippocampus and amygdala were homogenised in phosphate buffer solution with 1 mM phenylmethylsulfonyl fluoride and 1 mM ethylene glycol tetraacetic acid. Microtiter plates (96-well flat bottom) were coated for 24 h with the samples diluted 1:2 in sample diluents and standard curve ranged from 7.8 to 500 pg/ml of BNDF. The plates were then washed four times with sample diluent and a monoclonal anti-BNDF rabbit antibody diluted 1:1000 in sample diluent was added to each well and incubated for 3 h at room temperature. After washing, a peroxidase conjugated anti-rabbit antibody (diluted 1:1000) was added to each well and incubated at room temperature for 1 h. After addition of streptavidin enzyme, substrate and stop solution, the amount of BDNF was determined by absorbance in 450 nm. The standard curve shows a direct relationship between optical density and BDNF concentration. Total protein was measured by Lowry's method using bovine serum albumin as a standard, as previously described by Lowry and et al. (Reference Patel, Cravatt and Hillard57).
Statistical analysis
All data are presented as mean ± SEM. Differences among experimental groups in the forced swimming and open-field tests and in the assessment of BDNF levels were determined by one-way analysis of variance (ANOVA), followed by Tukey post hoc test when ANOVA was significant; p-values <0.05 were considered to be statistical significant.
Results
The administration of the standard antidepressant imipramine at the dose of 30 mg/kg and cannabidiol at the dose of 30 mg/kg reduced the immobility time of rats, compared with saline group in both acute (Fig. 1a; F (4–47) = 4.54; p = 0.003) and chronic (Fig. 1b; F (4–38) = 3.7, p = 0.01) treatments, and imipramine, but not cannabidiol increased both climbing (Fig. 1a; F (4–43) = 2.5, p = 0.5 and Fig. 1b; F (4–37) = 2.89, p = 0.03) and swimming (Fig. 1a; F (4–46) = 5.5, p = 0.001 and Fig. 1b; F (4–38) = 5.7, p = 0.001) times. Cannabidiol at the dose of 30 mg/kg increased the swimming time, but not climbing time of rats in both acute and chronic treatments (Fig. 1a; p = 0.001 and Fig. 1b; p = 0.001).

Fig. 1. Effects of acute (a) and chronic (b) administration of cannabidiol (15, 30 and 60 mg/kg), imipramine (30 mg/kg) and saline on the immobility time of rats subjected to the forced swimming test. Bars represent means ± SEM of 15 rats. *p < 0.05 versus saline according to ANOVA followed by Tukey post hoc test.
In the open-field test, acute and chronic treatments with imipramine and cannabidiol at all doses did not modify the number of crossings (acute: F (4–35) = 0.15, p = 0.96; chronic: F (4–45) = 0.59, p = 0.66) and rearings (acute: F (4–35) = 0.76, p = 0.55; chronic: F (4–45) = 0.44, p = 0.77), compared with saline-treated rats (Table 1).
Table 1 Effects of acute and chronic treatments with cannabidiol (15, 30 and 60 mg/kg), imipramine (30 mg/kg) and saline on rat behaviour in the open-field task
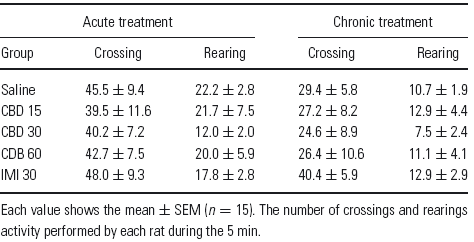
Each value shows the mean ± SEM (n = 15). The number of crossings and rearings activity performed by each rat during the 5 min.
Figure 2 illustrates the effects of acute and chronic treatments with imipramine (30 mg/kg), cannabidiol (15, 30 and 60 mg/kg) and saline in BDNF protein levels in prefrontal cortex, hippocampus and amygdala. In the acute treatment both imipramine and cannabidiol did not alter BDNF protein levels in prefrontal cortex (Fig. 2a; F (4–32) = 0.45, p = 0.76), hippocampus (Fig. 2a; F (4–33) = 0.98, p = 0.42) or amygdala (Fig. 2a; F (4–34) = 0.33, p = 0.85). In the chronic treatment, there was an increase in BDNF protein levels in amygdala in rats treated with imipramine at the dose of 30 mg/kg and cannabidiol at the dose of 15 mg/kg, compared with saline group (Fig. 2b; F (4–22) = 5.38, p = 0.004). In prefrontal cortex (Fig. 2b; F (4–33) = 0.51, p = 0.72) and in hippocampus (Fig. 2b; F (4–28) = 0.42, p = 0.78) chronic treatment with imipramine and cannabidiol did not alter BDNF protein levels.

Fig. 2. Effects of acute (a) and chronic (b) administration of cannabidiol (15, 30 and 60 mg/kg), imipramine (30 mg/kg) and saline on the BDNF levels in rat prefrontal cortex, hippocampus and amygdala. Bars represent means ± SEM of 10 rats. * p < 0.05 versus saline according to ANOVA followed by Tukey post hoc test.
Discussion
It is known that cannabidiol has a wide spectrum of pharmacological actions (Reference Sumariwalla, Gallily, Tchilibon, Fride, Mechoulam and Feldmann17). In this study, we showed that acute and chronic administration with cannabidiol at the dose of 30 mg/kg reduced immobility time and increased swimming time of rats, without affecting locomotor activity.
Other studies have suggested that there are functional interactions between the endogenous cannabinoid system and stress circuitry (Reference Zanelati, Biojone, Moreira, Guimarães and Joca43,Reference Martin, Ledent, Parmentier, Maldonado and Valverde58), emotional regulation and depression (Reference Russo, Burbett, Hall and Parker44,Reference Carlson, Wang and Alger59) and synaptic transmission in the hippocampus (Reference Fortunato, Réus and Kirsch60). In addition, chronic, non-habituating stress resulted in a decrease in functional endogenous cannabinoid signalling within the hippocampus, and the stress-reduced impairment in reversal learning can be reversed by exogenous activation of CB1 receptors (Reference Anderson45), suggesting that pharmacological modulation of endogenous cannabinoid signalling could represent a novel approach to the treatment of cognitive deficits that accompany a variety of anxiety-related neuropsychiatric disorders. Moreover, cannabidiol at the dose of 30 mg/kg and imipramine at the dose of 30 mg/kg reduced immobility time in the forced swimming test, without changing exploratory behaviour in mice (Reference Anderson45). Furthermore, delta-9-tetrahydrocannabinol and cannabidiol, but not cannabigerol and cannabinol, exhibited a significant antidepressant-like action in mice (Reference Hill, Patel and Carrier42), suggesting these effects may contribute to the overall mood-elevating properties of cannabis.
In this study, we also showed that acute and chronic treatments with imipramine at the dose of 30 mg/kg reduced immobility time and increased both swimming and climbing time in rats, without affecting locomotor activity. Several other studies from our laboratory also have showed that a single injection or chronic administration of imipramine (30 mg/kg) decreased the immobility and increased swimming and climbing times of rats on the forced swimming test, without modifying the locomotor activity (54,55,61,62).
The forced swimming test is a current model and gauges an animal's ‘depression-related' responses to acute or chronic inescapable stress (Reference Aan het Rot, Mathew and Charney63). In addition, Detke et al. (Reference Detke, Rickels and Lucki52) reported that despite the anti-immobility effect antidepressant drugs that enhance noradrenergic neurotransmission increase climbing behaviour, whereas the enhancement of serotonergic neurotransmission increases swimming time in the rat forced swimming test. Our findings indicate that cannabidiol consistently reduced immobility time and significantly increased swimming time, and antidepressant effects observed by cannabidiol may be by serotonergic and noradrenergic neurotransmission actions. In fact, cannabidiol may have properties at 5-HT1A receptors (Reference Anderson45–Reference Karege, Vaudan, Schwald, Perroud and La Harpe47), which have been related to the therapeutic effect of antidepressant drugs (Reference Karege, Vaudan, Schwald, Perroud and La Harpe47). Nevertheless, there are relationships between the serotonin and BDNF (Reference Martinowich and Lu64,Reference Nestler, Barrot, DiLeone, Eisch, Gold and Monteggia65). For example, BDNF stimulates the transcription of genes involved in serotonin function and activation of serotonin receptors by serotonin stimulates expression of the BDNF factor gene. During brain development this cyclic process promotes outgrowth, synapse formation and survival of serotonin neurons and the eventual innervation of multiple brain regions (Reference Duman and Monteggia66).
BDNF-mediated signalling is involved in neuroplastic responses to stress and antidepressants (Reference Aan het Rot, Mathew and Charney63,Reference Nestler, Barrot, DiLeone, Eisch, Gold and Monteggia65). In this data, we investigated the effects of acute and chronic administration of imipramine and cannabidiol in BDNF protein levels in the rat prefrontal cortex, hippocampus and amygdala. These brain areas are implicated in major depression; in addition, the hippocampus has connections with amygdala and prefrontal cortex (Reference Larsen, Hay-Schmidt, Ronn and Mikkelsen67). Our study showed that chronic but not acute treatment increased the BDNF protein levels after administration of imipramine at the dose of 30 mg/kg and cannabidiol at the dose of 15 mg/kg only in the amygadal, suggesting that imipramine and cannabidiol effects are dependent on dose, brain region and treatment regime. In fact, Zanelati et al. (Reference Anderson45) showed that both imipramine (30 mg/kg) and cannabidiol (30 mg/kg) did not change hippocampal BDNF levels. Previous studies of our group also showed that acute and chronic administration of imipramine decreased the immobility time of rats in forced swimming test, but did not alter BDNF protein levels in the hippocampus (54,55,61,62); in these studies we did not evaluate the effects of imipramine in amygdala.
Recently, Larsen et al. (Reference Kovaru, Pav, Kovaru, Raboch and Fiserova68) detailed temporal profiles of the effects of three antidepressants with different pharmacological profiles on the expression of BDNF mRNA and showed a significant increase in BDNF mRNA expression in the granular cell layer after 7 days of treatment with venlafaxine, and after 14 days of treatment with imipramine, but not after 1 day of treatment and a modest decrease in BDNF mRNA expression was observed in the CA3 region after chronic treatment with imipramine. These results indicated that the change in BDNF levels is dependent on treatment time and the region of the hippocampus.
In another study from our group, which investigated the effects of cannabidiol in an animal model of mania induced by d-amphetamine, our group showed that cannabidiol did not have any effect against d-amphetamine-induced hyperactivity, but cannabidiol at the dose of 30 mg/kg reversed the d-amphetamine-induced damage and increased BDNF expression; in addition, cannabidiol (30 or 60 mg/kg) prevented the d-amphetamine-induced formation of carbonyl group in prefrontal cortex (Reference El-Remessy, Khalil and Matragoon24), suggesting these effects vary depending on the brain regions evaluated and doses of cannabidiol administrated.
It is well known that in the hippocampus, as well as prefrontal cortex, increased BDNF results in antidepressant responses (Reference Yu and Chen69), the fact that no differences in these areas reported in this study may be related to differences in the neural circuitry. In fact, stress seems to exert opposite effects in amygdala and hippocampus, for example, stress increases spine synapse formation in amygdala (Reference Vyas, Mitra, Shankaranarayana Rao and Chattarji70), but decreases in hippocampus (Reference Pawlak, Rao, Melchor, Chattarji, McEwen and Strickland71). In addition, in the hippocampus and prefrontal cortex BDNF inhibits depressive symptoms, whereas in the amygdala it facilitates depressive-like symptoms (Reference Yu and Chen69). However, acute social stress or repeated restraint exposure reduced amygdala BDNF (Reference Pizarro, Lumley and Medina72,Reference Fanous and Hammer73), suggesting positive effects of both imipramine and cannabidiol in this study.
In conclusion, given the central role of the amygdala in the modulation of emotional responses (Reference Murray74), the effects of cannabidiol on antidepressant-like behaviour may attribute to changes in amygdalar neuroplasticity or could be because of the combinations of cannabidiol with other effects, e.g. inflammatory effects (Reference Walter, Franklin and Witting18–Reference Jacobsson, RongÄrd, Stridh, Tiger and Fowler20), antioxidative action (Reference Mechoulam, Parker and Gallily21,Reference El-Remessy, Khalil and Matragoon24) and neuroprotective effect (Reference El-Remessy, Khalil and Matragoon24,Reference Ryan, Drysdale, Lafourcade, Pertwee and Platt25), which are involved in major depression (Reference Lucca, Comim and Valvassori75,Reference Lucca, Comim and Valvassori76).
Acknowledgements
This study was supported in part by grants from CNPq-Brazil (J. Q., F. K., J. A. C., A. W. Z. and J. E. H.), FAPESP-Brazil (J. A. C., A. W. Z. and J. E. H.), FAPESC-Brazil (J. Q.), Instituto Cérebro e Mente-Brazil (J. Q. and F. K.) and UNESC-Brazil (J. Q.). J. Q., F. K., J. A. C. and A. W. Z. are recipients of CNPq (Brazil) Productivity Fellowships. G. Z. R. is holder of a CAPES studentship. This study was also sponsored by THC-Pharm (Frankfurt, Germany) and STI-Pharm (UK) who kindly provided cannabidiol.