Introduction
Approaching a precise identification of the Kimmeridgian-Tithonian boundary in Mexico based on ammonite biostratigraphy has been a difficult task due to the occurrence of widespread endemism, unfavorable facies, and/or the scarcity of precise, bed-by-bed sampling programs (Villaseñor et al., Reference Villaseñor, Olóriz and González-Arreola2000, Reference Villaseñor, Olóriz, López Palomino and López-Caballero2012, and references therein). Mexican ammonites traditionally related to the Kimmeridgian-Tithonian boundary were first interpreted as Waagenia Neumayr, Reference Neumayr1878 and later as Hybonoticeras. Their occurrence was variably related to those belonging to genus Mazapilites Burckhardt (Reference Burckhardt1919 in 1919–1921). No precise biostratigraphy has been reported for these two genera since pioneer works, after more than one hundred years of research, and only three publications have approached fine biostratigraphy, phenotypic response to paleoenvironmental forcing, and paleobiogeographic dynamics for Hybonoticeras based on bed-by-bed sampling (Olóriz et al., Reference Olóriz, Lara, De La Mora, Villaseñor and González-Arreola1993, Reference Olóriz, Villaseñor and González-Arreola2000; Olóriz and Villaseñor, Reference Olóriz and Villaseñor1999). Updated data and interpretations about these two genera will improve the potential for correlation involving the Kimmeridgian-Tithonian boundary, which is one of the topics receiving special attention by the International Subcomission on Jurassic Stratigraphy. Moreover, they will provide support for future research focused on species-level definitions and intraspecies structure, as well as on paleobiogeographic interpretations in Mexican-Caribbean areas. Given the long history of contributions including data about Mexican records of Hybonoticeras and Mazapilites, a revision of relevant, previous works is obligatory.
Ammonites currently included in the genera Mazapilites and Hybonoticeras have been known in Mexico since the first quarter of the past century. Among the types housed in the Colección Paleontológica Nacional, Maria del Carmen Perrilliat (Instituto de Geología, UNAM, Mexico City), there are six specimens and fragments of Waagenia (= Hybonoticeras) belonging to Burckhardt’s collections that have been revised and reillustrated; a seventh type specimen is lost. Also housed there, and analyzed, is a plaster cast of the type of Waagenia parrasensis Imlay, Reference Imlay1939. Three plaster casts of Hybonoticeras sp. gr. H. beckeri (Neumayr, Reference Neumayr1873) studied by Zell et al. (Reference Zell, Beckmann and Stinnesbeck2014), housed in the Colección del Museo del Desierto (Saltillo, Coahuila) were also analyzed. All of these records refer to macroconchiate specimens of the genus Hybonoticeras. Also found in the Colección Paleontológica Nacional, Maria del Carmen Perrilliat, and re-examined are 18 specimens and fragments of Mazapilites from the Burckhardt (Reference Burckhardt1906, 1919–Reference Burckhardt1921) collections, two Mazapilites from the Peña-Muñoz (Reference Peña-Muñoz1964) collection, one from the Verma and Westermann (Reference Verma and Westermann1973) collection, and one from the authors’ collection (Olóriz et al., Reference Olóriz, Villaseñor, González-Arreola and Westermann1999); the syntypes of Pulchellia mexicana Aguilera in Del Castillo and Aguilera, Reference Del Castillo and Aguilera1895 (= Mazapilites mexicanus) are lost, but a plastotype from the Smithsonian Institution’s National Museum of Natural History was available for analysis. An additional specimen of Mazapilites was analyzed from the Villaseñor et al. (Reference Villaseñor, González-León, Lawton and Aberhan2005) collection, which is housed at the Estación Regional del Noreste del Instituto de Geología, UNAM (Hermosillo, Sonora).
Based on new material collected bed-by-bed and the analysis of existing material and references in the literature, the aims of the present research are: (1) to provide the first conclusive evidence of lower Tithonian macroconchiate Hybonoticeras from Mexico, with identification of a new species, and evaluation of its value for precise biostratigraphy and intercontinental correlation; (2) to provide a preliminary re-evaluation of the genus Mazapilites based on the analysis of type material to explore its precise biostratigraphic meaning and present status at the species level; (3) to revise reported occurrences of Hybonoticeras and Mazapilites in the Mexico-Caribbean area, approaching an updated interpretation at the species level; (4) to report new material collected bed-by-bed that makes it possible to illustrate for the first time the co-occurrence of Hybonoticeras and Mazapilites in Mexico; and (5) to revisit previous reports of co-occurrences of these two genera from the Mexico-Caribbean area.
Geographic range, stratigraphy, and general paleoenvironmental setting
The present geographic distribution of Hybonoticeras records covers a wide area across north-central Mexico (i.e., north-central San Luis Potosí, northern Zacatecas, eastern Durango, and southern Coahuila) and northeastern Mexico (i.e., eastern Chihuahua, southeastern Nuevo León, and southeastern and northeastern Tamaulipas). The geographic distribution of Mazapilites is larger, including northwestern and southeastern Mexico. Both genera also occur in western Cuba (Fig. 1.1). The paleobiogeographic ranges of H. hybonotum Oppel, Reference Oppel1863 and closely related species, as well as of members of the genus Mazapilites, are shown in Figure 2.1.
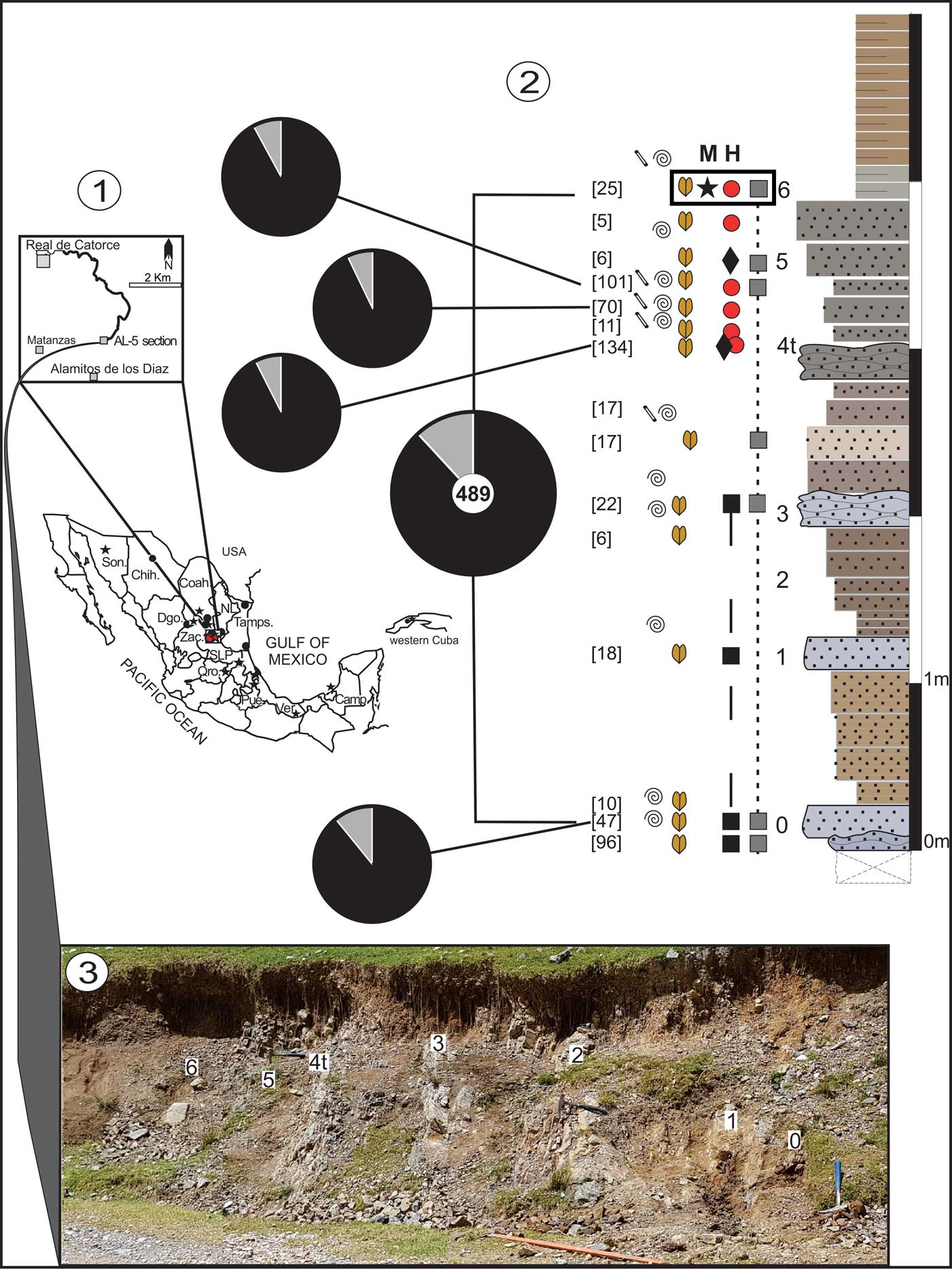
Figure 1 Geographic location, lithologic log, and outcrop view: (1) geographic distribution of Hybonoticeras (dots) and Mazapilites (stars) in Mexico and western Cuba; inset shows new records in San Luis Potosí State; (2) lithologic log of section AL-5 (levels 0–6) with records of ammonites (spirals), genus Hybonoticeras (H, large filled circles), H. authariformis n. sp. [M] (diamonds), genus Mazapilites (M, stars), haploceratines (black squares), perisphinctines (gray squares), bivalves (bilobate symbols), and serpulids (cylinders); faunal spectra (pie diagrams) shown for the complete section and characteristic beds, with numbers in brackets indicating the total fauna for each bed, and black and gray colors for benthics and ammonites, respectively; rectangle indicates the precise horizon of the combined record of Hybonoticeras and Mazapilites; (3) outcrop view of the studied section AL-5 located near Alamitos de los Díaz, known in the geological literature as Rancho Los Alamitos, 23o39'1.6''N, 100o50'54.7''W. Mexican states: Camp. = Campeche Shelf; Chih. = Chihuahua; Coah. = Coahuila; Dgo. = Durango; NL = Nuevo León; Pue. = Puebla; Qro. = Queretaro; SLP = San Luís Potosí; Son. = Sonora; Tamps. = Tamaulipas; Ver. = Veracruz; Zac. = Zacatecas.

Figure 2 Paleogeographic maps: (1) global paleogeography for 152 Ma (earliest Tithonian; Gradstein et al., Reference Gradstein, Ogg, Schmitz and Ogg2012) adapted from Scotese (Reference Scotese2001); for improved resolution of the Antarctic regions, see Golonka (Reference Golonka2007, figs. 25, 28); black symbols indicate records of macroconchiate Hybonoticeras hybonotum (plus signs); closely related, inconclusively known forms with scarce, local records (squares); microconchs belonging to the group of H. mundulum, which first appear in uppermost Kimmeridgian horizons (circles); poorly known records of early Tithonian Hybonoticeras microconchs (triangle); and Mazapilites (stars); updated to complement citations in the Systematic paleontology chapter; (2) synthetized paleogeography for the Mexican mainland and GoM during Tithonian times, based on the publications cited in Table 1 and interpretations by the authors; areas under influence of magmatic activity related to intraplate and plate boundary dynamics, seepage, effusive-extrusive flows, and volcanoclastics included (asterisks); note the combined representation of alternative locations of eastern Panthalassa volcanic arcs forcing subduction-accretion complexes in western Mexico (i.e., Guerrero-arc terrane complexes in distal and proximal settings), and assumed maximal extension of the ‘Nevadan Orogeny building’ (UaWMArC) at the expense of the subducting Mezcalera Plate and diminishing Arperos Ocean; major marine environments shown in increasing tone darkness seaward from coastal inner shelf areas for increasing depth of slightly deeper to moderate deep waters from mid-to-outer shelves, and then deeper, oceanic waters related to oceanic crust domains with assumed horst-graben seafloor topography and restricted deep-water circulation, at least for the GoM and the proto-Caribbean seaway; emerged lands (blackish). AL = Alamitos, 5 sections; Ap = Aldama platform; Ch = Chortís Block, only central and eastern Chortís terranes; Co = Coahuila Island/Peninsula; cp = Campeche platform; GoM = Gulf of Mexico; L = Laurentia; M[Y] = Maya Block, Yucatán; P[F] = Panthalassa Ocean and Farallon Plate; P[M] = Panthalassa Ocean and Mezcalera Plate; p-C = proto-Caribbean seaway; Ta = Tamaulipas archipelago; UaWMArC = Uplifted-Active-West-Mexico-Magmatic-Arc-Complexes. See text for further explanation.
The revised material was retrieved from the La Caja and La Casita formations, which include the Kimmeridgian to the lowermost Berriasian epicontinental, distal-to-proximal mixed carbonate-siliciclastic deposits in north-central and northern Mexico, respectively (Olóriz et al., Reference Olóriz, Villaseñor and González-Arreola2003), and from the Cucurpe Formation, representing Late Jurassic proximal, mainly siliciclastic deposition under volcanic influence from intra-arc to backarc contexts in northwestern Mexico (Mauel et al., Reference Mauel, Peryam, Lawton, Amato and González-León2005, Reference Mauel, Lawton, González-León, Iriondo and Amato2011). Other reports of Mazapilites without illustrations have been published from the following formations: La Casita Formation (see above); Las Trancas Formation (carbonate and siliciclastic deposition in basinal to shallow nearshore and estuarine environments, under the influence of backarc vulcanism in the Mesa Central Basin and southern areas of the eastern Sierra Madre; Kimmeridgian–Valanginian?; López-Ramos, Reference López-Ramos1985; PEMEX, 1988; López-Palomino and Piña-Arce, Reference López-Palomino and Piña-Arce2007); Tamán Formation (organic matter-rich, mixed carbonate-fine, siliciclastic deposition on a wide-shelf system across eastern Mexico; upper Kimmeridgian–lower Tithonian, Cantú-Chapa, Reference Cantú-Chapa1971, Reference Cantú-Chapa1984; Tamán or Tamán-like deposition is assumed for a proximal fringe offshore, at least in the Tampico-Tuxpan area); Pimienta Formation (mainly silty to muddy-limy and organic-rich deposition on a wide, irregular shelf under volcanic influence across eastern Mexico; uppermost Kimmeridgian–Tithonian, Cantú-Chapa, Reference Cantú-Chapa1971; lower but not lowermost Tithonian to upper Tithonian, Cantú-Chapa, Reference Cantú-Chapa1984; Tithonian–Valanginian?, López-Palomino and Piña-Arce, Reference López-Palomino and Piña-Arce2007; Pimienta or Pimienta-like to more shaley deposition is postulated for a proximal fringe offshore, at least in the Tampico-Tuxpan area); Chinameca Formation (organic matter-rich carbonates and fine clastics, shelf deposits, across southeastern Mexico, on land and offshore; Kimmeridgian to Lower Cretaceous, Burckhardt, Reference Burckhardt1930, Cantú-Chapa, Reference Cantú-Chapa2006; Kimmeridgian–Barremian, Sáenz-Pita and López-Palomino, Reference Sáenz-Pita and López-Palomino2011; Oxfordian pars. to Hauterivian in Sierra de Chiapas, Tehuantepec Isthmus, Quezada-Muñetón and Ferrusquia-Villafranca, Reference Quezada-Muñetón and Ferrusquía-Villafranca2013); and Edzna Formation (basinal, organic-rich carbonate and fine-clastic deposition recorded from subsurface at the Campeche region, on land and offshore; Tithonian, Cantú-Chapa and Ortuño-Maldonado, Reference Cantú-Chapa and Ortuño-Maldonado2003).
The revised material from Cuba was mainly retrieved by Myczyński (Reference Myczyński1989, Reference Myczyński1999), and partially re-interpreted by Pszczółkowski and Myczyński (Reference Pszczółkowski and Myczyński2003, Reference Pszczółkowski and Myczyński2010) from western Cuba, with indication of limited data of Mazapilites, but no Hybonoticeras, from the Camajuaní Belt in central Cuba (Pszczółkowski and Myczyński, Reference Pszczółkowski and Myczyński2003). Fossiliferous horizons of reference in western Cuba were reported from: (1) well-stratified gray-black limestones, with occasional fine clayey intercalations from the El Americano Member of the Guasasa Formation in Sierra de Los Órganos, which represents Tithonian to early Berriasian outer-shelf-to-upper-slope deposition; and (2) well-bedded and commonly laminated black mudstones to wackestones, with occasional intercalations of shales and siltstones, and laminated shales and marls upward, from the La Zarza Member of the Artemisa Formation in Sierra del Rosario, which are interpreted as outer ramp deposits deepening throughout younger Tithonian times. Thus, analogous paleoenvironmental conditions during the same time interval can be interpreted for both areas (Cobiella-Reguera and Olóriz, Reference Cobiella-Reguera and Olóriz2009, and references therein).
Precise citations of authors and publications involved in the particular cases analyzed are given throughout the text.
A general paleoenvironmental context can be approached taking into account climatic conditions for Mexican areas and their surroundings during Tithonian times. These have been interpreted within global contexts, including paleogeography (Fig. 2.2; Table 1), according to weather-sensitive fossils and rocks, and considering the highest sea-level phase during the Jurassic and its potential forcing (e.g., Scott, Reference Scott1984; Ross et al., Reference Ross, Moore and Hayashida1992; Weissert and Mohr, Reference Weissert and Mohr1996; Rees et al., Reference Rees, Ziegler and Valdes2000, Reference Rees, Noto, Parrish and Parrish2004; Ford and Golonka, Reference Ford and Golonka2003; Ziegler et al., Reference Ziegler, Eshel, McAllister Rees, Rothfus, Rowley and Sunderlin2003; García-Díaz, Reference García-Díaz2004; Busby et al., Reference Busby, Bassett, Steiner and Riggs2005; Sellwood and Valdes, Reference Sellwood and Valdes2006; Holz, Reference Holz2015). According to these authors, relevant features of the paleoenvironmental context were: (1) paleolatitude ranges of 0–20ºN and 0–30ºN, but smaller ranges were also proposed (10ºN to slightly more than 22ºN), favoring development of reefs and shallow carbonates in the surroundings of the Gulf of Mexico (GoM) Basin and evaporites between 15ºN and 22ºN in the area; (2) surface temperature ranging 20–36ºC, with a winter to summer mean temperature difference of ~8ºC; (3) low to moderate relief modeled for southwestern and northwestern Mexico; (4) generally low precipitation, with a regionally distinct precipitation/evaporation rate—net precipitation westward and net evaporation eastward—with aridity being higher southward; (5) however, in terms of Walter biomes, northern Mexico has been considered subtropical to dry whereas southern Mexico would be tropical to subtropical-humid; (6) global climate models indicating upwelling influence from the western coast to eastern Panthalassa Ocean; (7) major oceanic anoxic event (OAE) and organic-rich deposition across the GoM; and (8) northward drifting of 10–15° during the Tithonian. The possibility of an end to the monsoonal climate pattern, which dominated during Jurassic times, has been also considered to occur at the end of the Jurassic (Weissert and Mohr, Reference Weissert and Mohr1996).
Table 1 Bibliographic database used in support of the synthetic interpretation of paleogeography and major marine water masses shown in Figure 2.

To complement the general paleoenvironmental context expounded, it should be remembered that the Tithonian was a time interval involved in plate-boundary reorganization, in the area and worldwide (Ford and Golonka, Reference Ford and Golonka2003). Punctuated activity occurred in the Mexican convergent-transpressive margins, as well as northward in Cordilleran terranes, whereas progressive spreading with the rotation of Yucatán affected the GoM during Tithonian times (Delgado-Argote, Reference Delgado-Argote1989; Goldhamer, Reference Goldhamer1999; Bird and Burke, Reference Bird and Burke2006; Stern and Dickinson, Reference Stern and Dickinson2010; LaMaskin, Reference LaMaskin2012).
Some additional comments address the marine waters in the Mexican-GoM region. On the whole, the paleogeographic scenario for marine upper-layer waters (i.e., those inhabited by ammonites in Mexican areas during Tithonian times) was related to epicontinental seas across Mexican blocks accreted to southwestern Laurentia as part of the North American Plate. There, the scenario was of irregular, more or less restricted shelves with adjacent, more depressed, basinal areas. Local, constrained, and ephemeral epicontinental connections with proto-Caribbean water masses occurred southward (Fig. 2.2). A rather tortuous circulation of marine upper layers is envisaged across Mexican epicontinental shelves, where emerged lands occurred (Fig. 2.2). Forcing of sea surface currents by easterlies, distorted by local, irregular upwellings, would be expected, and fertilization of water masses was common during Tithonian times. Without evidence of discharges of major fluvial systems affecting these epicontinental shelves, fertilization would be rather forced through frequent volcanic activity and secondarily by winds. In contrast, fluvial influence was higher northward, in the extensional-to-transtensional system of the Border Rift and in the northern rim of the GoM. The influence of volcanism was common and related to: (1) volcanic-arc activity westward, at the western Mexico margin, (2) transtensional rifting with volcanic activity north-northwestward, (3) progressing oceanization during the opening of GoM to the east, with southward displacement of the Maya Block, and (4) associated progressing oceanic seaways connecting the Hispanic Corridor with the easternmost Panthalassa Ocean southward (Fig. 2.2). Deeper, epioceanic-oceanic water masses were located west-, east- and southward of the Mexican mainland. Deep-water conditions (currents, oxygenation, etc.) are envisaged as those corresponding to intricate rift and magmatic-arc related systems submitted during Tithonian times to limited spreading phases and block tectonics, or to transpression (and accretion), respectively—i.e., restricted ocean circulation and resulting low oxygen content, together with additional effects of common submarine volcanism and seepage. The combination of restricted marine currents, low oxygenation of bottom waters, and fertilization by volcanic activity in rifted, arc-related, and foredeep basins resulted in common deposition of organic-rich, gray-to-black sediments (calcareous shales and argillaceous-to-silty carbonates). Variation in deposition depths, clay content, and oxygenation determined differences among Tithonian source rocks, but these Tithonian sediments gave rise to the main Jurassic hydrocarbon source rocks in Mexico, the GoM, and Cuba (Viniegra, Reference Viniegra1981; Peterson, Reference Peterson1983; Santamaría-Orozco et al., Reference Santamaría-Orozco, di Primio, Pickel, Holguín and Horsfield1995; Rodríguez-Viera et al., Reference Rodríguez-Viera, Brey del Rey, Blanco Bustamante, Rodríguez-Loeches and Villavicencio1998; Ángeles-Aquino and Cantú-Chapa, Reference Ángeles-Aquino and Cantú-Chapa2001; Cole et al., Reference Cole, Yu, Peel, Taylor, Requejo, DeVay, Brooks, Bernard, Zumberge and Brown2001; Eguiluz de Antuñano, Reference Eguiluz de Antuñano2001; Guzmán-Vega et al., Reference Guzmán-Vega, Castro-Ortíz, Román-Ramos, Medrano-Morales, Valdéz, Vázquez-Covarrubias and Ziga-Rodríguez2001; Magoon et al., Reference Magoon, Hudson and Cook2001; Mancini et al., Reference Mancini, Badali, Puckett, Llinas and Parcell2001; Prost and Aranda, Reference Prost and Aranda2001; Williams-Rojas and Hurley, Reference Williams-Rojas and Hurley2001; Gaumet and Letouzey, Reference Gaumet and Letouzey2002; Cantú-Chapa and Ortuño-Maldonado, Reference Cantú-Chapa and Ortuño-Maldonado2003; Moretti, et al., Reference Moretti, Tenreyro-Perez, Linares, Lopez, Letouzey, Magnier, Gaumet, Lecomte, Lopez and Zimine2003; Santamaría-Orozco and Horsfield, Reference Santamaría-Orozco and Horsfield2003; Padilla y Sánchez, Reference Padilla y Sánchez2007; Schenk, Reference Schenk2010; Muñoz-Cisneros et al., Reference Muñoz Cisneros, Lara Rodríguez, Marino Castañón, Chávez Carcini, Román Ramos, Clara Valdés, Hernández Romano, Navarro Baca and Gómez Rodríguez2013). Paleogeographic interpretations of western areas related to active plate margins facing the Panthalassa Ocean are still unconclusive and controversial. These areas were submitted to the influence of subduction-accretion complexes—see Figure 2.2 and Table 1 for a draft and references, combining alternative interpretations about the location and eastward displacement of the Guerrero magmatic-volcanic-sedimentary-arc complex and others.
The studied section
The AL-5 section studied at the Sierra de Catorce, San Luis Potosí (23o39'1.6''N, 100o50'54.7''W; Fig. 1.2, 1.3), is 5 m thick and made of sandy to silty, grayish phosphoritic, and more or less calcareous horizons. The former is less common but more resistant to erosion. A brownish surface color results from weathering. Bedding is well defined, with strata showing flattish lower and upper surfaces, especially in silty phosphoritic horizons. Less common sandy phosphoritic horizons have more or less irregular top surfaces and local concretionary features, and seem to be the end members of upward thicking sedimentary packages. Bed thickness throughout the first 4 m (levels 0–5) shows values of 15–20 cm (46%), 10 to < 15 cm (29%), and < 10 cm (17%), without counting shaly interbeds. Microfacies in phosphoritic horizons indicate a coarse-silt-to-fine-sand matrix, with less common medium-sand and scattered coarse-sand grains. The latter are commonly phosphatized and brownish under transmitted, plane-polarized light, thus indicating collophane. The uppermost 1 m of the studied section is composed of < 10 cm thick, silty-to-very-fine-sandy horizons.
Within the paleoenvironmental and paleogeographic context provided earlier, and to consider a depositional context for the studied section AL-5, the following site effects must be considered: (1) location at a mid-range of latitude for Mexico mainland areas during the early Tithonian (i.e., ~22–23°N); (2) under east-northeast dominant winds (easterlies) forcing westward transport of surface waters with net offshore displacement in coastal waters; and (3) constituting a raised bottom (Olóriz et al., Reference Olóriz, Villaseñor, González-Arreola and Westermann1999). Hence, and in accordance with the paleoenvironmental and paleogeographic context, the AL-5 site was under warm-water masses with rather restricted circulation, and received upwelling events of variable intensity during the time corresponding to the studied interval. At first, upwelling influence could be expected from the west but, given the assumed paleogeography (Fig. 2.2), marine events geologically propagated from east or west would be forcing factors rather than prevalent winds. Restriction of wide-ranging phosphoritic deposits to particular stratigraphic intervals across north-central Mexico, without identifiable cyclicity, strengthens this interpretation. In such a context, and at a mid-shelf position, phosphoritic sediments in the AL-5 site were deposited as higher-energy inflows barging into relatively restricted water masses. The latter would be warmer than mean surface temperature (> 30ºC; see above) and with an at least dysoxic lower water column and seabed. This context resulted in increased nutrients according to the abundance of low diversified benthics (overwhelmingly abundant bivalves, and persistent, rather isolate serpulids and planolites-maker burrowers), and moderate, short-time oxygenation of lower waters and seabed (particulate organic-rich sediments) during episodes of relatively higher-than-background energy.
In accordance with the paleoenvironmental scenario presented, the ecostratigraphic interpretation of section AL-5 (Fig. 1.2, 1.3, levels 0–6) is attempted by analysis of 489 fossil remains of which 88.14% are benthics (mainly bivalves) and 11.86% are ammonites (Ammonitina, incomplete specimens and fragments). Excluded from the analysis were data obtained from the basal horizon underlying level 0 because of taphonomic condensation, i.e., biostratigraphic mixing and top surface of stratigraphic discontinuity with the studied overlying section, which most probably represents a hiatal contact. Paleodepth interpretations are controversial whether sedimentologically or paleoecologically approached. However, it is now accepted that Ammonitina inhabited upper marine waters, and estimates of preferred depths of particular groups exist, as do considerations of their relative abundances with respect to benthics and related depths in terms of dozens of meters (Ziegler, Reference Ziegler1967; Gygi, Reference Gygi1986, Reference Gygi1999; Olóriz et al., Reference Olóriz, Marques and Moliner1988, Reference Olóriz, Caracuel, Ruiz-Heras, Rodr�guez-Tovar and Marques1996, Reference Olóriz, Palmqvist and Pérez-Claros2002, Reference Olóriz, Reolid and Rodríguez-Tovar2006; Westermann, Reference Westermann1996; Westermann and Tsujita, Reference Westermann and Tsujita1999; Lewy, Reference Lewy2002; Olóriz and Villaseñor, Reference Olóriz and Villaseñor2010, and references therein). Whatever the case, we are still far from precise concerning inhabited habitat depths by distinct Ammonitina within marine upper-water masses. On this basis, and considering these limitations, figures from levels 0–6 in the AL-5 section (Fig. 1.2), showing an overabundance of bivalves and secondary records of ammonites without evidence of colonization by epizoa, indicate shallow waters (~30 m or less). Similar assemblages of macroinvertebrates were reported by Olóriz et al. (Reference Olóriz, Lara, De La Mora, Villaseñor and González-Arreola1993) from interpreted Hybonoticeras hybonotum Biozone horizons in Durango, Mexico, and by Olóriz (Reference Olóriz1992) who provided a general picture of Mexican shelves with rather shallow depths and irregular, unstable sea bottoms forcing relative abundance in macroinvertebrate assemblages with ammonites. Short term postmortem transportation and subsequent common reworking (i.e., without biostratigraphic incidence) is suggested for the ammonites analyzed (see below). In addition, the record of benthics—mainly epifaunal and infaunal bivalves (e.g., Parallelodontidae Dall, Reference Dall1898, Pteriidae Gray, Reference Gray1847, Ostreidae Rafinesque, Reference Rafinesque1815, Astartidae d’Orbigny, Reference d’Orbigny1844, Lucinidae Fleming, Reference Fleming1828, and Pleuromyidae Dall, Reference Dall1900)—shows a moderate number of articulated specimens and less fragmentation than that seen in ammonites. The total abundance of bivalves reveals a slight decrease from level 0 to level 3, and a later sixfold recovery to level 4t, which shows the highest concentration of specimens (epi- and endofauna mixed in the same stratigraphic horizon). All of this agrees with increasing life conditions for benthics and limited reworking under persistent but fluctuating upwelling conditions in shallow waters.
Materials and methods
Bed-by-bed sampling was conducted in the outcrop corresponding to the AL-5 section from the Sierra de Catorce, San Luis Potosí, Mexico (Fig. 1.3). It provided a total of 587 specimens and fragments (IGM 10190–10749), including 86% benthics (mainly bivalves, IGM 10225–10641; under study) and 14% ammonites (Ammonitina, see Systematic paleontology). The new ammonite material of Hybonoticeras and Mazapilites is 2% of the total material obtained.
Material for thin sections was selected from hand samples under stratigraphic control. All materials described and/or mentioned in The studied section and Systematic paleontology chapters are housed in the Colección Paleontológica Nacional, Museo María del Carmen Perrilliat of the Instituto de Geología, UNAM (Mexico City, Mexico). Usual procedures for preparation and study of ammonites and petrographic samples were applied, as well as those for stratigraphic and ecostratigraphic analyses in the section investigated.
A careful analysis was made of descriptions and stratigraphic interpretations in publications appearing in the early twentieth century to date. This revision is presented as selected comments of the cases of interest, with precise reproduction of original texts (relevant sentences) when appropriate. This treatment is followed by a precise, direct analysis of available types, and then by the updated interpretation of all information considered. The research conducted under these terms, comprising a careful analysis of all citations of hybonoticeratin and mazapilitin ammonites reported from Mexico and Cuba, aims to support their revision and updated interpretation.
Repositories and institutional abbreviations
The studied types correspond to the preserved specimens, illustrated or not, belonging to old and modern collections housed in the following institutions: CPC, Colección del Museo del Desierto, Saltillo, Coahuila, Mexico; ERNO, Estación Regional del Noroeste Collection, Sonora, Mexico; IGM, Colección Paleontológica Nacional, Museo María del Carmen Perrilliat, Instituto de Geología, UNAM, Mexico City; MÁFI, Magyar Állami Földtani Intézet, Budapest, Hungary; McM-J, McMaster University, Hamilton, Canada (some specimens of which are housed in the museum at the Institute of Geology, Mexico City); SNSB-BSPG, Bayerische Staatssammlung für Palaontologie und Historische Geologie, Munich, Germany; UM, University of Michigan, Ann Arbor, Michigan, USA; UNAM, Universidad Nacional Autónoma de México, Mexico City; USNM PAL, National Museum of Natural History (United States National Museum), Smithsonian Institution, Washington, DC, USA.
Abbreviations of morphological terms
Those used in morphological descriptions are: CI = costal or ribbing index, indicating the number of external, peripheral, secondary ribs per 10 primary ribs; Dm = shell diameter; ET/2 = number of external tubercles per half whorl; H = whorl height; H/Dm = whorl height to shell diameter ratio; H/W = whorl height to whorl width ratio; IT/2 = number of internal tubercles per half whorl; M = macroconch; m = microconch; U = umbilicus; U/Dm = umbilicus to shell diameter ratio; UR/2 = number of umbilical ribs per half whorl; W = whorl width.
Systematic paleontology
Class Cephalopoda Leach, Reference Leach1817
Order Ammonoidea Zittel, Reference Zittel1884
Suborder Ammonitina Hyatt, Reference Hyatt1889
Superfamily Perisphinctoidea Steinmann in Steinmann and Doderlein, Reference Steinmann1890
Family Aspidoceratidae Zittel, Reference Zittel1895
Subfamily Hybonoticeratinae Olóriz, Reference Olóriz1978
Remarks
The use of a family group name for hybonoticeratin ammonites shows rather unstable treatment and needs formal stabilization. The hybonoticeratines, or ‘hybonoten’ of classic authors (e.g., Neumayr, Reference Neumayr1873, p. 190; Reference Neumayr1878, p. [70]34), are a morphologically well defined but inconclusively known ammonite grouping. As reported by Olóriz (Reference Olóriz1978, p. 333–335), these ammonites have been interpreted in different, imprecise evolutionary relationships within aspidoceratines, which were mainly, but not exclusively, referred to as the family Aspidoceratidae in a variable sense by diverse authors (e.g., Zittel, Reference Zittel1884; Salfeld, Reference Salfeld1919; Roman, Reference Roman1938; Arkell in Arkell et al., Reference Arkell, Kummel and Wright1957; Barthel, Reference Barthel1959; Berckhemer and Hölder, Reference Berckhemer and Hölder1959; Ziegler, Reference Ziegler1959; Christ, Reference Christ1960). Moreover, hybonoticeratin ammonites have been considered to be more or less closely related to simoceratines (e.g., Spath, Reference Spath1924, Reference Spath1925, Reference Spath1930, Reference Spath1931; Arkell in Arkell et al., Reference Arkell, Kummel and Wright1957 with hybonoticeratines in the subfamily Simoceratinae Spath, Reference Spath1924 within Aspidoceratidae; Schindewolf, Reference Schindewolf1925, Reference Schindewolf1966), placed with aspidoceratines (e.g., in Aspidoceratinae by Schindewolf, Reference Schindewolf1925; Trauth, Reference Trauth1927; Arkell in Arkell et al., Reference Arkell, Kummel and Wright1957, see above), or related to an unclear, alternative origin in Simoceratinae or Aspidoceratinae but included in Simoceratinae (e.g., Spath, Reference Spath1931). Subsequent proposals suggested inconclusive evolutionary relationships within the stem Perisphinctidae Steinmann in Steinmann and Döderlein, Reference Steinmann1890, the latter being interpreted in a broad sense to include aspidoceratin and euaspidoceratin ammonites (Olóriz, Reference Olóriz1978; Callomon, Reference Callomon1981).
Interpretations of hybonoticeratines since the middle of the past century entail common references at the family level (e.g., Aspidoceratidae by Barthel, Reference Barthel1959 and Berckhemer and Hölder, Reference Berckhemer and Hölder1959; Ziegler, Reference Ziegler1959; Schweigert et al., Reference Schweigert, Zeiss and Westermann2012 with Hybonoticeras belonging to aspidoceratids without mention of a formal taxonomic level of reference). References at the subfamily level and strictly focused on hybonoticeratines have been common since the 1970s (e.g., Hybonoceratinae, recte Hybonoticeratinae, proposed as new subfamily by Olóriz, Reference Olóriz1978), whereas reference to Simoceratinae was maintained by Sapunov (Reference Sapunov1979), Krishna (Reference Krishna1983), and Verma and Westermann (Reference Verma and Westermann1984); in turn, Pathak (Reference Pathak1993) linked hybonoticeratin ammonites to the family Simoceratidae. Hybonoticeratinae was again proposed by Callomon (Reference Callomon1981) without reference or comments on the previous proposal made by Olóriz (Reference Olóriz1978). Reference to the subfamily Hybonoticeratinae has been common since the 1980s but involving different proposals: (1) Hybonoticeratinae Olóriz, Reference Olóriz1978 (by Rossi, Reference Rossi1984; Olóriz et al., Reference Olóriz, Lara, De La Mora, Villaseñor and González-Arreola1993, Reference Olóriz, Villaseñor and González-Arreola2000; Olóriz and Villaseñor, Reference Olóriz and Villaseñor1999; Fatmi and Zeiss, Reference Fatmi and Zeiss1999; Myczyński, Reference Myczyński1999; Zell et al., Reference Zell, Beckmann and Stinnesbeck2014); (2) Hybonoticeratinae Neumayr, Reference Neumayr1878 (by Sarti, Reference Sarti1984; Vigh, Reference Vigh1984), most probably by re-interpretation of the footnote no. 1 by Neumayr (Reference Neumayr1878, p. 70[34]); (3) Hybonoticeratinae Callomon, Reference Callomon1981 (by Schlamp, Reference Schlamp1991; Pathak, Reference Pathak1993; Schlegelmich, Reference Schlegelmilch1994; Schweigert, Reference Schweigert1998); (4) Hybonoticeratinae without reference to an author (by Krishna and Pathak, Reference Krishna and Pathak1993); and (5) Hybonoticeratinae Callomon, Reference Callomon1981 (by Sarti, Reference Sarti1993; Howarth, Reference Howarth1998; Enay, Reference Enay2009, who modified the composition interpreted by Callomon to a quasi-identical coincidence with the one made by Olóriz, Reference Olóriz1978).
Olóriz (Reference Olóriz1978, p. 332–335) erected the subfamily name Hybonoceratinae (sic) with precise indication of morphological features (ICZN, 1999, art. 13.1.1, recommendation 13A), genera and species composition (ICZN, 1999, art. 11.7.1.2), and a revision of previous treatments of the alluded ‘hybonoten’ ammonites. Without an explicit statement of intention (ICZN, 1999, art. 33.2.1), and based on the valid genus-level name Hybonoticeras (ICZN, 1999, arts. 11.7.1.1, 13.2, 29.1), the correct spelling for the subfamily name is Hybonoticeratinae (ICZN, 1999, arts. 11.7.1.1, 29.3), as later prevailed and valid per se, as well as according to ICZN (1999) article 32.2.1. Hence, the original spelling Hybonoceratinae by Olóriz (Reference Olóriz1978, excepting p. 659 where Hybonoticeratinae was correctly used) can be considered an incorrect original spelling (ICZN, 1999, arts. 32.5, 32.5.1, and the example following the latter) to be formally corrected (ICZN, 1999, arts. 32.5.3, 32.5.3.3). Olóriz et al. (Reference Olóriz, Lara, De La Mora, Villaseñor and González-Arreola1993 and subsequent works by this author and collaborators, as well as by other authors; see above) used the correct spelling Hybonoticeratinae in the same sense as that proposed by Olóriz (Reference Olóriz1978). These citations therefore represent the proper use of correct original spellings (ICZN, 1999, arts. 24.2.3, 24.2.4), taking into account the proper citation of the name Hybonoticeratinae by Olóriz (Reference Olóriz1978, p. 659). In addition, the proposal made by Callomon (Reference Callomon1981) coincided with that previously made by Olóriz (Reference Olóriz1978) in the family group name selected (Hybonoticeratinae), but not in the precise evolutionary meaning of lineage relationships with older, Oxfordian taxa proposed by Callomon (Reference Callomon1981). These comments are presented as a justified emendation according to ICZN (1999) articles 19.2, 32.2.2, 32.5, 33.2.2, and 50.4.
Genus Hybonoticeras Breistroffer, Reference Breistroffer1947
Type species
Ammonites hybonotus Oppel, Reference Oppel1863 from the Lithographischer Schiefer, Solnhofen (Bavaria), Germany.
Hybonoticeras authariformis new species
urn:lsid:zoobank.org:pub:78C89620-FA2E-4DE5-985D-819421A858F9
Figures 3.1–3.3, 4.1–4.4, 5.1, 5.6, 5.9; Table 2

Figure 3 Hybonoticeras authariformis n. sp. [M], holotype, IGM 4698, bed AL-5.4t in section Alamitos 5 (AL-5), specimen AL-5.4t.1, La Caja Formation, lower Tithonian: (1) adapertural view; (2) left-lateral view showing crushed, eroded inner whorls and preserved outer whorl, which largely belongs to the body chamber (asterisk marks end of the phragmocone), with stiff, simple ribs, two rows of well-developed tubercles, local preservation of ventral tubercles (arrow), and accentuated difference in rib crowding due to slight, postmortem deformation; (3) ventral view, showing a shallow and wide venter (arrow), elongate ventral tubercles, and a wide left-lateral shoulder; note missing preservation of the ventral region to the right (dot). Scale bar = 1 cm.

Figure 4 Hybonoticeras from beds AL-5.4t and AL-5.6 in section Alamitos 5 (AL-5), San Luis Potosí, La Caja Formation, lower Tithonian. Hybonoticeras authariformis n. sp. [M]: (1) paratype, IGM 4699, specimen AL-5.4t.2, oblique-ventral view showing coarse external and ventral tubercles; (2, 3) paratype, IGM 4700, bed AL-5.4t, specimen AL-5.4t.3, lateral (2) and ventral (3) views, showing coarse external and ventral tuberculation; (4) paratype, IGM 4701, specimen AL-5.4t.4, fragment of external whorls showing stiff primary and intercalatory ribs. Hybonoticeras cf. H. pseudohybonotum, bed AL-5.4t in section Alamitos 5 (AL-5): (5–9) IGM 4707, specimen AL-5.4t.5, partially preserved as an imprint and inner cast in volume, and a fragment preserved in volume; (5) right-lateral view; (6) imprint; (7–9) plaster cast of Figure 4.6; (7) left lateral view, showing smoothed ‘quasi-V’ pattern of rib connection (arrow); (8) ventral view; (9) oblique view, showing dense crenulation of the keel; (10, 11) IGM 4711, specimen AL-5.4t.9, sample separation in two halves resulting in preservation as inner cast and imprint. Dotted white lines indicate ventral furrows. Scale bar = 1 cm (note different bar for Figs. 4.7, 4.8).
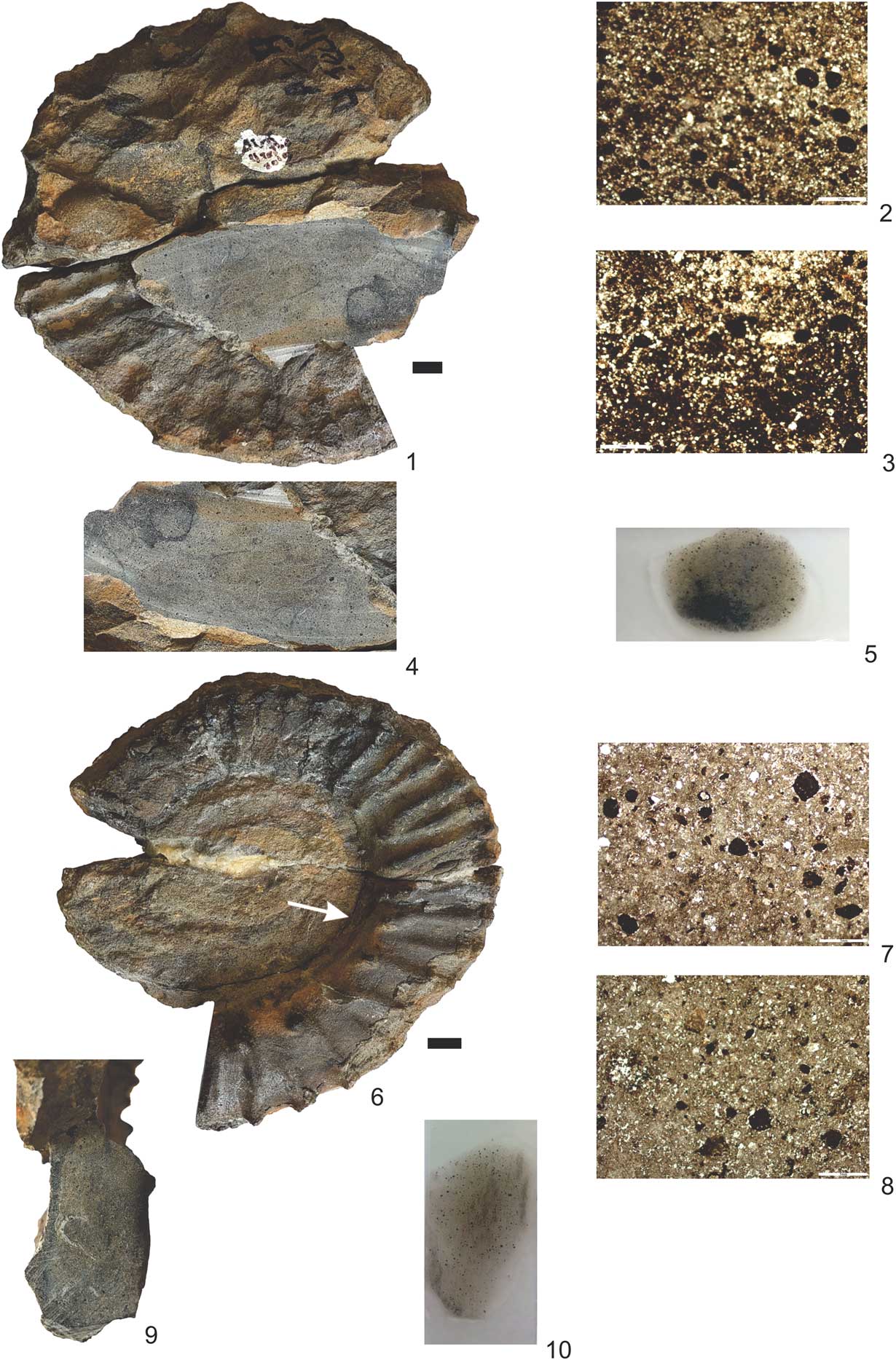
Figure 5 Preservation state of Hybonoticeras authariformis n. sp. (M) and matrix of the encasing rock from bed AL-5.4t in section Alamitos 5 (AL-5), Sierra de Catorce, San Luis Potosí, La Caja Formation, lower Tithonian, holotype, IGM 4698, specimen AL-5.4t.1: (1, 4) right-lateral view of hand sample including the described specimen and showing the sliced area in the enclosing rock (detail in Fig. 5.4); (2, 3) photomicrographs of encasing deposits showing coarse-silt to fine-sand matrix with scattered grains of collophane (darker grains) of fine and medium-sized sand grains; (5) thin-section appearance to compare with that corresponding to the infilling of the body chamber (Fig. 5.10); (6) opposite view of the same hand sample showing the left side of the described specimen with limited displacement (arrow) of the inner whorls; (7, 8) photomicrographs showing finer silty matrix with very fine, scattered medium, and rare, coarse, sand-sized grains of collophane (darker grains) in the infilling of the body chamber; (9, 10) sliced area (9) and thin-section appearance to compare with that corresponding to the encasing sediment (Fig. 5.5). Scale bars = 1 cm. See text for taphonomic features and interpretation.
Table 2 Measurements of Hybonoticeras authariformis n. sp. [M], holotype, IGM 4698, AL-5.4t.1, at five different diameters.

CI = costal or ribbing index indicating the number of external, peripheral, secondary ribs per 10 primary ribs; Dm = shell diameter; ET/2 = number of external tubercles per half whorl; H = whorl height; H/Dm = whorl height to shell diameter ratio; H/W = whorl height to whorl width ratio; IT/2 = number of internal tubercles per half whorl; U = umbilicus; U/Dm = umbilicus to shell diameter ratio; UR/2 = number of umbilical ribs per half whorl; W = whorl width; * = approximated value; - = not available. All figures in millimeters except when expressing ratios.
Holotype
IGM 4698, corresponding to specimen AL-5.4t.1, Upper Jurassic, lower Tithonian, from bed 4t in the section Alamitos 5 (AL-5) close to the village of Alamitos de los Diaz, San Luis Potosí, Mexico.
Diagnosis
Shell large, evolute. Whorl section rectangular. Two rows of well-developed tubercles on the outer whorl. Coarse ribs on the outer whorl. Wide, shallow ventral groove outlined by nodate keels. Peristome and suture line unknown.
Occurrence
Lower Tithonian, El Pastor Member La Caja Formation, Sierra de Catorce, San Luis Potosí, Mexico. Its association with Hybonoticeras pseudohybonotum Vigh, Reference Vigh1984 allows interpretation of its provenance from lowermost Tithonian horizons.
Description
The most complete specimen (holotype, IGM 4698; Fig. 3.1–3.3) shows the left flank favorable for sculpture description (see measurements in Table 2). The shell is large (Dm = 180 mm), evolute (U/Dm = 0.5–0.52), and planulate. The whorl section is rectangular (H/W = 1.4–2) with a high, abrupt umbilical wall and flat flanks (Fig. 3.1). The sculpture preserved on the outer whorl is of coarse but acute tubercles that have spiny extremes on both the umbilical edge and just below the shoulders. The number of periumbilical tubercles is constant (12; see Table 2) between 150 and 180 mm in diameter, whereas the external, ventrolateral tubercles are more numerous (20–21). Sturdy, rigid, more or less radial to rursiradiate ribs link the tubercles of the two lateral rows developed on the flanks. Intercalatory ribs with similar trajectories occur, extending over the flank to the inner third, even closer to the position of the periumbilical row of tubercles. Real ‘V’-connections of ribs (i.e., the rib connections close to the periumbilical edge) are not observed, but some cases of a ‘quasi-V’ pattern exist, in which the influence of some degree of taphonomic distortion could be present. No looped, geminate ribs were observed. The ventral region is preserved at the adapertural part of the outer whorl, showing wide and slightly raised ventrolateral areas (shoulders) without identifiable remains of riblets connecting external tubercles to the keels. Hence, the latter appear as beaded, nodated reliefs in which tubercles seem to be clavate and clearly elongated longitudinally (Fig. 3.1–3.3). The groove that occupies the midline of the venter is shallow and as wide as one-third of the venter amplitude (Fig. 3.3). At least a half whorl belongs to the body chamber. There is no preserved trace of the adapertural structure. No suture lines are preserved.
Ammonite fragments IGM 4699, 4700, 4701, and 10189 are preliminarily included in Hybonoticeras authariformis n. sp. [M]. They show coarse, rigid ribs connected to the ventrolateral tubercles, with wide, excavated inter-rib spaces, and wide, shallow ventral grooves. There is no preservation of the inner flanks. Specimen IGM 4699 (Fig. 4.1) is a fragment showing a presumed body chamber smaller than that of the holotype. It shows a wide whorl section, well-developed shoulders and prominent dentate keels, but the preservation is limited. Of particular interest is the occurrence of a potential, prorsiradiate riblet connecting a coarse ventrolateral tubercle with a crescentic, adaperturally placed relief of the keel (see below). Specimen IGM 4700 (Fig. 4.2, 4.3) is an even smaller fragment that shows the typically strong sculpture with prominent, spiny ventrolateral tubercles connected to coarse, rigid ribs, and a keel of more rounded tubercles (corresponding to the smaller shell?). Specimen IGM 4701 (Fig. 4.4) is a poorly preserved fragment showing 3 or 4 coarse, rigid ribs with eroded ventrolateral tubercles and its interpretation is tentative. Specimen IGM 10189 is an inner cast showing ~9 cm long left lateral view of a fragment belonging to a shell that could be 150 mm in diameter. The umbilicus is wide. Shell crushing is slight and the restored section shows an H/W of ~2. The flank sculpture is well preserved, but the venter is eroded. External tubercles have wide bases and are more numerous than the periumbilical ones. All tubercles are connected to stiff, coarse ribs, some of which are shorter intercalatories extending to the flank and producing ‘quasi-V’ connections with the internal range of tubercles.
Etymology
From morphological similarity with Hybonoticeras autharis (Oppel in Berckhemer and Hölder, Reference Berckhemer and Hölder1959).
Materials
One specimen with well-preserved outer whorls (IGM 4698, holotype), and four fragments (IGM 4699–4701 and 10189, paratypes).
Preservation
Evaluation of potential reworking is crucial before interpreting the biostratigraphic meaning of ammonite records. Hence, special relevance is given to the taphonomic analysis of the best-preserved specimen, the designed holotype.
Holotype IGM 4698 is preserved in volume as an inner cast showing a silty-to-fine sandy, grayish, and more-or-less calcareous matrix including very fine, scattered medium sand grains, and rare coarse sand grains, the latter two grain-size classes being commonly phosphatized (see below). The four fragments—paratypes IGM 4699 (AL-5.4t.2), IGM 4700 (AL-5.4t.3), IGM 4701 (AL-5.4t.4), and IGM 10189 (AL-5.5.1)—are inner casts tentatively included in the new species and likewise preserved.
Crushing was not relevant for paleontological identification. A case of multiphased taphonomic history has been identified and can be interpreted for holotype IGM 4698, and its paleontological identification is supported by careful analysis of the left and ventral views (Fig. 3.2, 3.3).
Taphonomic observations conducted on holotype IGM 4698 show differential preservation, more or less affecting half of the preserved outer whorl. The adapertural half whorl is preserved in volume whereas the aboral one is comparatively crushed. Moreover, the inner whorls in left lateral view were slightly displaced from the plane of shell coiling and symmetry (Fig. 5.6). Analysis of the right side shows rather severe crushing of the adapertural half outer whorl, which forced a corresponding reduction in shell thickness (Fig. 5.1, 5.9). Thus, flattening of the right side of the outer whorl impedes any direct evaluation of shell thickness for this part of the inner cast, which belongs to the body chamber.
The taphonomic features just described can be interpreted according to a taphonomic course that includes common reworking of an incompletely infilled shell, and can be summarized as follows: (1) horizontal to slightly subhorizontal settling of the shell with the right side upward; (2) partial sedimentary infilling affecting the body chamber and, most probably, a large part of the phragmocone; no observations were available for evaluating the precise degree of sedimentary infilling within the chambered part of the shell, but incomplete infilling of the phragmocone would determine low internal support for the right side of the shell (settled as the roof of the carcass) against the sedimentary load and related dissolution; (3) rapid burial hampering shell colonization by epibionts during background depositional conditions; (4) an early burial phase close to the sea bottom, increasing unfavorable conditions for colonization by macroepibionts while the shell underwent progressive dissolution; in a short time and within a benthos-rich substrate, the combination of dissolution and increased sedimentary load forced limited plastic deformation of the upper (right) side (the relative roof of the settled shell), therefore, the body chamber and presumably the most prominent parts of the phragmocone were affected on their right side; (5) sedimentary overburden determined limited crushing of the upper (right) side of the shell in both the body chamber and the phragmocone; most probably, more severe crushing affected the phragmocone (limited observation); (6) early lithification progressed, resulting in formation of the inner cast (steinkern), which reproduced a partially modified shell morphology on the right side; (7) common reworking, i.e., reworking below biostratigraphic resolution, exhumed and redeposited the steinkern, with no significant difference between the silty-to-fine sandy and the more or less calcareous matrix that previously infilled the interior of the empty shell and the sediment outside the steinkern (Fig. 5, note the coarse-silty-to-fine-sandy and more or less calcareous matrix outside the inner cast, including fine sand with a variable abundance of medium and less common coarse sand grains [Fig. 5.2–5.5], whereas the equivalent matrix in the body chamber infilling includes very fine and more scattered medium sand grains, and rare coarse sand grains [Fig. 5.6–5.8, 5.10]); brownish grains are made of collophane showing moderate thermal alteration; a diluted hydrochloric acid reaction is similar for the matrix of the encasing rock and the infilling of the body chamber; (8) final settling after reworking, now with the left side upward and under background depositional conditions, i.e., no special, relevant difference in matrix composition between the steinkern and enclosing sediments (see details in point 7); (9) burial related to reworking rapid enough to impede macroscopic colonization of the steinkern by epibionts, e.g., epizoa such as common serpulids recorded from the same horizon, an endo- rather than epibenthos-rich seabed; (10) final settlement of the steinkern with the left side upward, hence, the left side is better preserved than the overturned right side, which inherited crushing from the pre-reworking burial phase; and (11) sedimentary overburden forcing increased pressure selectively on the umbilical and slightly concretionary plug, thus resulting in limited downward, oblique displacement of the inner cast corresponding to the phragmocone exposed in the umbilicus.
In addition, the dominant-to-nearly-exclusive preservation of other ammonites in the section is as fragments of inner casts of haploceratines and perisphinctines that are difficult to identify at the genus level (IGM 4702–4706, 10190–10224), fragments of Hybonoticeras (IGM 4699, 4707, 4709, 4711, 4770, 4701, 4708, 4710, 10189), and more complete Mazapilites (IGM 4713–4715), all showing variable degrees of erosion but no differences with respect to the silty-to-fine sandy, grayish ochre, and more or less calcareous encasing rock.
Remarks
Hybonoticeras authariformis n. sp. [M] is similar to H. autharis as illustrated by Berckhemer and Hölder (Reference Berckhemer and Hölder1959, pl. 5, fig. 18). Both are large, evolute hybonoticeratines, showing rather radial to prorsiradiate ribs, strong ventrolateral tubercles, and shallow, wide ventral grooves. No other hybonoticeratines are comparable in these terms. Hybonoticeras authariformis n. sp. [M] differs from H. autharis in developing coarser, less crowded sculpture, in which periumbilical tubercles are much more prominent, and are clavate on the keels of the holotype. The ‘V’-connection of ribs might seem to be more common in H. autharis according to the specimen illustrated by Berckhemer and Hölder (Reference Berckhemer and Hölder1959, pl. 5, fig. 18), but neither this illustration nor that of the lectotype proposed by Berckhemer and Hölder (Reference Berckhemer and Hölder1959, p. 32) and illustrated by Quenstedt (Reference Quenstedt1888, pl. 124, fig. 14 = Ammonites perarmatus Quenstedt, Reference Quenstedt1888) allow for a conclusive interpretation. In contrast, careful analysis of a plaster cast of the specimen illustrated by Berckhemer and Hölder (Reference Berckhemer and Hölder1959, fig. 18) confirms that no real ‘V’-connections occur, that two consecutive intercalatories could be developed showing external, lateroventral tubercles, that there are periumblical tubercles unconnected to ribs, and that the keels show spiny tubercles. In addition, prosiradiate ribblets, or incipient reliefs, on the shoulders commonly connect ventrolateral tubercles to those of the keels in large, mature specimens of H. autharis, a feature that cannot be conclusively determined in H. authariformis n. sp. [M] due to limited preservation of the ventral region in the holotype (other fragments are too incomplete). The interpretation by Spath (Reference Spath1931, p. 646) of the specimen of H. ammonites autharis illustrated by Oppel (Reference Oppel1863, pl. 71, fig. 4) as being “typical of [H.] autharis,” and later proposed as a lectotype by Zeiss (Reference Zeiss2001, p. 63), as well as that by Malinowska (Reference Malinowska1989, pl. 5, figs. 3, 4) of an assumed juvenile of the species H. autharis, reinterpreted as H. knopi (Neumayr, Reference Neumayr1873) by Schweigert et al. (Reference Schweigert, Krishna, Pandey and Pathak1996), do not help to clarify the range of variation in the sculpture of H. autharis. On the preserved body chamber, the ventral tuberculation in H. authariformis n. sp. [M] appears to be more elongated, less oblique, and spinier than those shown in the adapertural quarter of the preserved outer whorl in the specimen illustrated by Berckhemer and Hölder (Reference Berckhemer and Hölder1959, fig. 19).
Hybonoticeras hybonotum has been interpreted in a broad sense, including closely related and inconclusively known forms (see paleobiogeographic range in Fig. 2.1). Such an interpretation can be recognized in the illustrations and/or synonymy lists by Oppel (Reference Oppel1863), Benecke (Reference Benecke1866), Favre (Reference Favre1877), Di Stefano (Reference Di Stefano1883), Valduga (Reference Valduga1954), Barthel (Reference Barthel1959), Berckhemer and Hölder (Reference Berckhemer and Hölder1959), Collignon (Reference Collignon1959, at least for H. laevigatum Collignon, Reference Collignon1959), Stephanov (Reference Stephanov1959), Enay et al. (Reference Enay, Martin, Monod and Thieuloy1971, Reference Enay, Hantzpergue, Soussi and Mangold2005), Olóriz (Reference Olóriz1978), Sapunov (Reference Sapunov1979), Mariotti et al. (Reference Mariotti, Nicosia, Pallini and Schiavinotto1979), Rossi (Reference Rossi1984), Sarti (Reference Sarti1984, Reference Sarti1985), Verma and Westermann (Reference Verma and Westermann1984), Geyssant in De Wever et al. (Reference De Wever, Geyssant, Azema, Devos, Duée, Manivit and Vrielynck1986), Howarth (Reference Howarth1992, Reference Howarth1998), Pathak (Reference Pathak1993), Wierzbowski (Reference Wierzbowski1994), Benzagaggh and Atrops (Reference Benzagaggh and Atrops1997), Caracuel and Olóriz (Reference Caracuel and Olóriz1999), Cecca (Reference Cecca1999), Benzagaggh (Reference Benzagaggh2000), Zeiss (Reference Zeiss2001), Enay (Reference Enay2009), Fözy et al. (Reference Fözy, Janssen and Price2011), Grigore (Reference Grigore2011), and Schweigert et al. (Reference Schweigert, Zeiss and Westermann2012), among others. Revision based on well-preserved material collected bed-by-bed is needed to propose a precise interpretation (e.g., Zeiss, Reference Zeiss2001), which must be based on population-level data to reach a conclusive evaluation of phenotype variability in the Oppel species. As usually interpreted, H. hybonotum did not develop such coarse, stiff, crowded ribbing, and its keels could be made of more rounded tubercles. The latter are well expressed in the ventral region illustrated by Oppel (Reference Oppel1863, pl. 71, fig. 1) in the specimen proposed as lectotype by Zeiss (Reference Zeiss2001), and are nicely shown in a color photo of this specimen kindly provided by G. Schweigert (Staatliches Museum für Naturkunde Stuttgart).
Hybonoticeras pseudohybonotum is another inconclusively known species commonly occurring with hybonoticeratines. No well-preserved inner whorls are known either from recently collected material or from specimens interpreted as closely related forms (e.g., Zeiss, Reference Zeiss2001). On the basis of the phenotypic variability envisaged by the accepted conspecific relationships among Ammonites hybonotum Benecke (Reference Benecke1866, pl. 11, fig. 1a–c), H. pseudohybonotum (see Vigh, Reference Vigh1984, pl. 2, fig. 1), H. hybonotum beneckei Geyssant (in De Wever et al., Reference De Wever, Geyssant, Azema, Devos, Duée, Manivit and Vrielynck1896, p. 160, pl. 1, figs. 2, 3), and H. aff. H. pseudohybonotum (see Haberl et al., Reference Haberl, Schairer, Schweigert and Zeiss1999, p. 15, pl. 1, fig. 1a–d), no comparable ribbing is seen with respect to H. authariformis n. sp. [M].
Lower Tithonian Hybonoticeras species reported from the Betic Cordillera, southern Spain (Olóriz, Reference Olóriz1978), and included in the H. hybonotum group, commonly show somewhat more involute shells and no comparable crowded, coarse, rigid ribbing (except in H. hybonotum autharis)—their interpretation at the species or subspecies levels accords with the preference of given authors in any case. Specimens representing H. autharis in southern Spain developed less common intercalatory ribs, and less massive whorl sections than those of H. authariformis n. sp. [M]. Other lower Tithonian hybonoticeratin species reported from southern Spain by Olóriz (Reference Olóriz1978) have different keels, whorl sections, and sculpture.
Hybonoticeras species from the uppermost Kimmeridgian Hybonoticeras beckeri Biozone, do not develop comparable sculpture, and the ventral groove is narrower and more excavated.
Hybonoticeras authariformis n. sp. [M] was collected with fragmented material morphologically close to H. pseudohybonotum from beds 4 and 5 in the AL-5 section, 110 cm and 20 cm below H. sp. gr. H. hybonotum and Mazapilites mexicanus, which were retrieved from bed 6 at the AL-5 section. The suggested biostratigraphic horizon for H. authariformis n. sp. [M] is basal Tithonian, which lends precision to the age interpretation of the single illustrated co-occurrence of Hybonoticeras and Mazapilites in Mexico (this paper) as occurring very early during the Tithonian but not during the earliest Tithonian.
As for determining the association between the highest sea levels during the Jurassic and colonization of epicontinental shelves by Hybonoticeras (Olóriz et al., Reference Olóriz, Lara, De La Mora, Villaseñor and González-Arreola1993, Reference Olóriz, Villaseñor and González-Arreola2000; Schweigert et al., Reference Schweigert, Krishna, Pandey and Pathak1996; Enay, Reference Enay2009), more complete material is necessary to the reliable evaluation of the paleobiogeographic meaning of the Mexican records (Fig. 2.1). Because no other record of H. authariformis n. sp. [M] is known, the new species is considered endemic of Mexico. The recognition of a case of endemism in macroconchiate Hybonoticeras from Mexico adds new information to the previous identification of phenotype deviations and endemism among microconchiate Hybonoticeras (Olóriz et al., Reference Olóriz, Villaseñor, González-Arreola and Westermann1999, Reference Olóriz, Villaseñor and González-Arreola2000), and opens new perspectives to revise records of Hybonoticeras in epicontinental shelves adjacent to water masses related to the Tethyan Ocean.
Hybonoticeras cf. H. pseudohybonotum Vigh, Reference Vigh1984

Figure 6 Hybonoticeras cf. H. pseudohybonotum, bed AL-5.4t in section Alamitos 5 (AL-5), San Luis Potosí, La Caja Formation, lower Tithonian: (1, 2) IGM 4709, specimen AL-5.4t.7; (1) lateral view of the imprint; (2) plaster cast of Figure 6.1; (3) IGM 4708, specimen AL-5.4t.6, ventral view showing crenulate keels; (4, 5) IGM 4710, specimen AL-5.4t.8, lateral (4) and ventral (5) views (see text for explanation of lettering). Hybonoticeras sp. gr. H. hybonotum, IGM 4712, specimen AL-5.6.1, bed AL-5.6 in section Alamitos 5 (AL-5): (6) lateral, and (7) ventral views showing smoothed but dense crenulation of the keel (white arrow) and coarse external tubercles (dotted circles). Dotted white lines indicate ventral furrows. Scale bar = 1 cm.
cf. 1886 Ammonites hybonotum Benecke, p. 187, pl. 11, fig. 1a–c.
cf. 1984 Hybonoticeras pseudohybonotum Reference VighVigh, p. 73, 179, pl. 2, fig. 1.
cf. 1986 Hybonoticeras hybonotum beneckei; Reference De Wever, Geyssant, Azema, Devos, Duée, Manivit and VrielynckDe Wever et al., p. 160, pl. 1, figs. 2, 3.
cf. 2001 Hybonoticeras cf. H. pseudohybonotum; Reference ZeissZeiss, p. 64, text-figs. ?6, 7.
Holotype
MÁFI J.9762, Ammonites hybonotum (Benecke, Reference Benecke1886, p. 187, pl. 11, fig. 1a–c), Diphyakalk, lower Tithonian, from Volano, Roveredo, southern Alps, southern Tirol, Italy.
Diagnosis
Medium-sized to large shell. Moderately evolute. Rectangular whorl section. Two rows of lateral, well-developed tubercles. Rather coarse, rigid ribs on the outer whorls. Riblets connecting ventrolateral tubercles with the keels. Inner whorls inconclusively known. Peristome preceded by a large constriction and assumed to be simple.
Occurrence
Hybonoticeras pseudohybonotum characterizes lowermost Tithonian horizons in sub-Mediterranean Europe. The studied material is suggested to belong to equivalent lowermost horizons of the Tithonian in Sierra de Catorce, San Luis Potosí, Mexico.
Description
The material collected is incomplete or fragmented, and its preservation barely allows identification of morphological features that point to the species proposed by Vigh (Reference Vigh1984). The inner cast IGM 4707 (Fig. 4.5–4.9) is partly deformed by crushing. It is ~ 90 mm in diameter, and corresponds to a relatively complete outer whorl of an evolute shell. The inner whorls cannot be identified. The preserved outer whorl shows flattened flanks with well-developed periumbilical tubercles and more numerous ventrolateral tubercles (Fig. 4.7). The keel is crenulate and riblets connect the ventrolateral tubercles to the small, ventral crenulations (Fig. 4.8, 4.9). Ribs are not well preserved but they exist, even showing a probable case of looping (Fig. 4.9).
Specimen IGM 4711 (Fig. 4.10, 4.11) of ~65 mm diameter and coiling degree of 40% was partially preserved as an inner cast and imprint after sample separation into two halves. It shows rigid ribbing on the inner whorls, coarse but taphonomically smoothed ribbing on the outer whorl, and fine tuberculation on the keels. Due to the limitations of preservation, the interpretation of this specimen is only tentative.
The inner cast IGM 4709 (Fig. 6.1, 6.2) is severely flattened and its preservation barely allows for identification of fine crowded ribs that connect two rows of lateral tubercles. A case of rib looping could exist. Fine prorsiradiate and concave forward ribs occur in intertubercular spaces on the shoulder. No information could be obtained about the inner whorls.
Fragment IGM 4708 (Fig. 6.3) belongs to a ventral region in which a midventral groove, as wide as one-third the width of the venter, is outlined by two crenulate keels connected by prorsiradiate riblets to well-developed ventrolateral tubercles.
Specimen IGM 4710 (Fig. 6.4, 6.5) shows three adjacent fragments (Fig. 6.4a–c) potentially belonging to the same evolute shell with a broken outer whorl (note preservation of the inner whorls in the lowermost fragment of Fig. 6.4c). In the larger one (upper fragment of the preserved outer whorl in Fig. 6.4a), an incomplete, flat flank can be observed, with two well-developed periumbilical tubercles connected to rigid, simple ribs, as well as a possible case of ‘quasi-V’ connection (Fig. 6.4a, arrow); a third periumbilical tubercle was damaged during sampling; the ventral region has a very wide midventral groove outlined by two poorly preserved keels and shoulders (Fig. 6.5a). This fragment looks similar to Hybonoticeras aff. H. pseudohybonotum illustrated by Haberl et al. (Reference Haberl, Schairer, Schweigert and Zeiss1999, pl. 1, fig. 1). The second, slightly smaller fragment (Fig. 6.4c, lower right fragment) shows an incomplete, flat right flank with three periumbilical tubercles. The third fragment (Fig. 6.4b, lowermost fragment) is severely eroded (smoothed?) and barely allows identification of middle to inner whorls with two potential ventrolateral tubercles.
Materials
Four incomplete specimens and fragments: IGM 4707 (AL-5.4t.5), 4708 (AL-5.4t.6), 4709 (AL-5.4t.7), and 4710 (AL-5.4t. 8), preserved as inner casts. In addition, IGM 4711 (AL-5.4t.9) is preserved as an inner cast and imprint.
Remarks
Hybonoticeras pseudohybonotum is an inconclusively known species which is usually interpreted on the basis of the illustrations provided by Benecke (Reference Benecke1866), Vigh (Reference Vigh1984), and De Wever et al. (Reference De Wever, Geyssant, Azema, Devos, Duée, Manivit and Vrielynck1986). All of these illustrations show inner whorls either idealized (Benecke, Reference Benecke1866) or not well preserved (Vigh, Reference Vigh1984; De Wever et al., Reference De Wever, Geyssant, Azema, Devos, Duée, Manivit and Vrielynck1986). Subsequent interpretations of closely affiliated forms (e.g., Haberl et al., Reference Haberl, Schairer, Schweigert and Zeiss1999; Zeiss, Reference Zeiss2001) did not improve this situation, because no inner whorls were preserved. According to Zeiss (Reference Zeiss2001, p. 63), this species of the H. hybonotum group developed subtle but typical connections among ventrolateral tubercles and the keels; the author held that this feature serves to identify H. pseudohybonotum compared to other members of the H. hybonotum group. As is usual with hybonoticeratines, precise knowledge at the species level is inconclusive at present.
Insofar as the incomplete specimens collected in Mexico allow for morphological comparison, the crushed specimen of Hybonoticeras cf. H. pseudohybonotum illustrated by Zeiss (Reference Zeiss2001, fig. 7) would appear to be the closest relative. This interpretation is reinforced by the occurrence of well-developed ventrolateral tubercles from which riblets connect with the keels. In addition, the wide ventral groove serves to relate the lowermost Tithonian European species of reference with the Mexican specimens.
In addition to lateral sculpture in the European specimens included in Hybonoticeras pseudohybonotum, the occurrence of a wide ventral groove, the type of keels, and the ventrolateral tuberculation shown by Vigh’s species and the Mexican specimens relate them to lower Tithonian hybonoticeratines. Hybonoticeras pseudohybonotum has been interpreted as representing a basal Tithonian horizon (Zeiss, Reference Zeiss2001, p. 69). According to present information, a closely equivalent biostratigraphic horizon is envisaged for the fragmentary material collected in Mexico, but more precise biostratigraphy is needed both in Europe and Mexico to arrive at a conclusive interpretation.
Hybonoticeras pseudohybonotum and close forms have been interpreted as characterizing the lowermost horizons of the lower Tithonian in Tethyan, Mediterranean, and sub-Mediterranean areas. Lacking more complete specimens, the scarce and fragmentary material obtained in the Mexican Al-5 section impedes any reliable paleobiogeographic interpretation, and a mere mention of morphological similarity with the species erected by Vigh would apply. Among lower Tithonian Hybonoticeras of the H. hybonotum group in southern Spain showing ‘quasi-V’ connections of ribs, H. robustum Olóriz, Reference Olóriz1978 clearly differs in showing a more equidimensional section; coarser, stiffer and more regular sculpture; and coarser tubercles on the keels (Olóriz, Reference Olóriz1978, p. 342–344, pl. 33, fig. 1).
As previously stated, the combination of favorable, high sea level, and widespread colonizations of epicontinental shelves by Hybonoticeras (Olóriz et al., Reference Olóriz, Lara, De La Mora, Villaseñor and González-Arreola1993, Reference Olóriz, Villaseñor and González-Arreola2000; Schweigert et al., Reference Schweigert, Krishna, Pandey and Pathak1996; Enay, Reference Enay2009) do not allow determination of precise paleobiogeographic dynamics and the meaning of the Mexican records described. More complete information on population data and composition of the ammonite assemblage is needed.
Hybonoticeras sp. gr. H. hybonotum (Oppel, Reference Oppel1863)
gr.1863 Ammonites hybonotus Reference OppelOppel, p. 254, pl. 71, figs. 1, 2.
gr.1877 Ammonites (Aspidoceras) hybonotus Benecke; Favre, p. 58, pl. 8, fig. l.
gr.1954 Waagenia sp. cf. hybonota; Reference ValdugaValduga, p. 26, pl. 6, fig. 1.
gr.1959 Hybonoticeras hybonotum; Reference BarthelBarthel, p. 63, text-fig. 7a–c.
pars.gr.1959 Hybonoticeras hybonotum sensu lato; Reference Berckhemer and HölderBerckhemer and Hölder, p. 30.
gr.1959 Hybonoticeras hybonotum; Reference StephanovStephanov, pl. 1, fig. 3, pl. 2, figs. 3, 4.
gr.1979 Hybonoticeras hybonotum; Reference SapunovSapunov, p. 161, pl. 51, fig. 1.
gr.1984 Hybonoticeras hybonotum hybonotum; Reference SartiSarti, p. 508, pl. 3, fig. 2a, b.
pars.gr.1978 Hybonoticeras (Hybonoticeras) hybonotum; Reference OlórizOlóriz, p. 336, pl. 33, fig. 2.
gr.1993 Hybonoticeras hybonotum; Reference PathakPathak, p. 127, pl. 1.
gr.1994 Hybonoticeras hybonotum; Reference WierzbowskiWierzbowski, p. 231, pl. 4, fig. 6a, b.
gr.1997 Hybonoticeras (Hybonoticeras) hybonotum; Reference Benzagaggh and AtropsBenzagaggh and Atrops, pl. 4, fig. 4.
pars.gr.1999 Hybonoticeras sp. gr.hybonotum; Reference Caracuel and OlórizCaracuel and Olóriz, p. 588, pl. 6, fig.10.
gr.2001 Hybonoticeras hybonotum; Reference ZeissZeiss, p. 63.
gr.2011 Hybonoticeras hybonotum; Reference Fözy, Janssen and PriceFözy et al., pl. 6, figs. 10, 11.
Lectotype
SNSB-BSPG AS I 567 (Oppel, Reference Oppel1863, pl. 71, fig. 1), Lithographischer Schiefer, lower Tithonian, Solnhofen, Bavaria, Germany, designated by Zeiss (Reference Zeiss2001).
Diagnosis
Shell large, evolute, with two rows of well-developed, lateral tubercles. Ribbing of variable strength, inconclusively known. Wide and shallow ventral groove. Peristome unknown but assumed to be simple.
Occurrence
Hybonoticeratines of the Hybonoticeras hybonotum group characterize the lower Tithonian elsewhere. Here, the analyzed Hybonoticeras sp. gr. H. hybonotum is suggested to represent lower but nonbasal horizons of the lower Tithonian at the investigated section AL-5.
Description
Fragment IGM 4712 (Fig. 6.6, 6.7) belongs to an outer whorl (body chamber?) of a large shell and is preserved as inner cast. The only preserved parts are the external portion of a flat flank, two coarse ventrolateral tubercles, and a wide, shallow groove on the midventer. The keels are crenulated but taphonomically smoothed.
Materials
One fragment of an inner cast, IGM 4712, specimen AL-5.6.1 from bed 6 in the section Alamitos 5 (AL-5).
Remarks
Hybonoticeras hybonotum is an imperfectly known species due to the extremely incomplete illustration provided by Oppel (Reference Oppel1863), and subsequent interpretations (see above). However, the sculpture and type of ventral groove make it possible to refer the described fragment to the group of H. hybonotum. No hybonoticeratines of the uppermost Kimmeridgian beckeri Zone developed a comparable type of shell and ventral region.
Specimen IGM 4712 was collected with two specimens interpreted as belonging to the morphological group of Mazapilites mexicanus. Thus, the co-occurrence of Hybonoticeras and Mazapilites in Mexico is demonstrated for the first time by specimens retrieved from the same bed and section (bed 6 in section AL-5.6).
Forms belonging to the group of Hybonoticeras hybonotum characterize the lower Tithonian elsewhere in Tethyan areas, and in those epicontinental areas under the influence of waters connected to the Tethyan Ocean. More complete material is necessary to determine the precise paleobiogeographic dynamics and meaning of the Mexican records within the context of the accepted relationships among the highest sea levels during Late Jurassic times and the colonization of epicontinental shelves by Hybonoticeras (e.g., Olóriz et al., Reference Olóriz, Lara, De La Mora, Villaseñor and González-Arreola1993, Reference Olóriz, Villaseñor and González-Arreola2000; Schweigert et al., Reference Schweigert, Krishna, Pandey and Pathak1996; Enay, Reference Enay2009).
Notes for an updated evaluation of the genus Mazapilites
Analysis of the typical Mexico-Caribbean, endemic genus Mazapilites is of special interest because of its inconclusive stratigraphic relationship with the worldwide genus Hybonoticeras.
Burckhardt (Reference Burckhardt1919 in 1919–1921) erected the genus Mazapilites for coarse-ribbed oppeliids found at the Cañón del Toboso, Durango. Burckhardt identified four new species and included a fifth one previously described as Eurynoticeras zitteli Burckhardt, Reference Burckhardt1906 from several locations across the Sierras de Mazapil and Santa Rosa (Burckhardt, Reference Burckhardt1906).
Present knowledge of Mexican Mazapilites is limited to material housed at IGM (18 specimens and fragments), and at ERNO (one specimen). These specimens belong to the collections of Burckhardt (Reference Burckhardt1906, four specimens and three fragments; 1919–1921, five specimens and two fragments, here reillustrated in Fig. 7), Peña-Muñoz (Reference Peña-Muñoz1964, two specimens), Verma and Westermann (Reference Verma and Westermann1973, one specimen), Olóriz et al. (Reference Olóriz, Villaseñor, González-Arreola and Westermann1999, one specimen), and Villaseñor et al. (Reference Villaseñor, González-León, Lawton and Aberhan2005, one specimen) (Fig. 8). The loose, and lost, syntype illustrated by Del Castillo and Aguilera (Reference Del Castillo and Aguilera1895, two specimens illustrated) was available in the form of a plaster cast provided by USNM PAL (Fig. 8.2). Two more specimens, plus one dubious fragment, were recently collected by the authors from bed 6 at the AL-5 section (Fig. 8.5–8.8). Hence, a total of 23 specimens and fragments were available for this revision.

Figure 7 Mazapilites spp. from the Burckhardt collection (Reference Burckhardt1906, from the Sierras de Mazapil and Santa Rosa, northern Zacatecas State, and 1919–1921 from Cañón del Toboso, eastern Durango State), lower Tithonian. Mazapilites zitteli: (1) IGM 246; (2) IGM 245a = 7166; (3) IGM 245; (4) IGM 246c = 7170; (5) IGM 246B = 7169; (6) IGM 245; (7) IGM 246a = 7168. Mazapilites tobosensis: (8) IGM 1567. Mazapilites symonensis: (9) IGM 1562; (10) IGM 1563. Mazapilites crassicostatus: (11) IGM 2684; (12) IGM 1564. Mazapilites sp.: (13) IGM 1566; (14) IGM 1565, from Burckhardt (1919–Reference Burckhardt1921). White dots indicate last preserved suture lines; asterisks mark beginnings of the preserved body chambers. Scale bar = 1 cm. See text for interpretations.

Figure 8 Mazapilites mexicanus, Sierra de Catorce, San Luis Potosí State and Rancho la Colgada, Sonora State, lower Tithonian: (1) ERNO 8031, right-lateral view, Rancho La Colgada, Sonora State, Cucurpe Formation, lower Tithonian (see Villaseñor et al., Reference Villaseñor, González-León, Lawton and Aberhan2005); (2) USNM PAL 103295, right-lateral view, plaster cast (see Del Castillo and Aguilera, Reference Del Castillo and Aguilera1895, pl. 6, fig. 8); (3) McM-J1506/380 = IGM 2746, left-lateral view (see Verma and Westermann, Reference Verma and Westermann1973); (4) IGM 6292, right-lateral view (see Olóriz et al., Reference Olóriz, Villaseñor, González-Arreola and Westermann1999); (5, 6) from bed AL-5.6 in section Alamitos 5 (AL-5), San Luis Potosí, La Caja Formation, lower Tithonian; (5) IGM 4714, right-lateral, and (6) imprint views; (7) IGM 4713, right-lateral view. Mazapilites? sp., bed 6 in the section Alamitos 5 (AL-5), San Luis Potosí, La Caja Formation, lower Tithonian: (8) IGM 4715, fragment of body chamber. Mazapilites symonensis, Durango State (Peña-Muñoz, Reference Peña-Muñoz1964), lower Tithonian: (9) IGM 7335, right-lateral view; (10) IGM 1216-1, left-lateral view of the nearly complete specimen. White dots mark last preserved suture lines; asterisks mark beginnings of the preserved body chambers. Scale bar = 1 cm. See text for interpretations.
Careful revision of Burckhardt’s collection (Reference Burckhardt1906, 1919–Reference Burckhardt1921) was undertaken to approach a preliminary, updated evaluation of genus Mazapilites at the species level. Burckhardt’s descriptions and illustrations reveal interesting traits in his collection of Mazapilites, which is the largest reported to date, comprising specimens preserved in volume (Fig. 7):
(1) Phragmocone preservation overwhelmingly dominates (Burckhardt, Reference Burckhardt1906, p. 110; 1919–Reference Burckhardt1921, p. 4–11), and only four cases of partial and crushed body-chamber preservation are known (Burckhardt, 1919–Reference Burckhardt1921, p. 4, pl. 1, fig. 9, p. 7, pl. 2, figs. 1, 6; note that the partial body chamber shown by Burckhardt, 1919–Reference Burckhardt1921, pl. 1, fig. 2, is lost). A fourth case of body-chamber preservation was reported by Peña-Muñoz (Reference Peña-Muñoz1964, pl. 1, fig. 1; Fig. 8.9) and is found in the Peña-Muñoz collection, showing a left-lateral view of a somewhat crushed specimen preserved in siltstone.
(2) Sculpture smoothing was recognized in the adult body chamber of Mazapilites symonensis Burckhardt, Reference Burckhardt1919 (Burckhardt, 1919–Reference Burckhardt1921, p. 3–5, pl. 1, fig. 9; Fig. 7.10). Another smoothed body chamber was illustrated by Burckhardt from a specimen collected from the Cañón del Toboso (Burckhardt, Reference Burckhardt1921, pl. 1, figs. 1–5, especially figs. 1–3 for the type), but the body chamber of this specimen is lost and only the phragmocone is preserved in the Burckhardt collection (Fig. 7.9). The specimen of M. symonensis illustrated by Peña-Muñoz (Reference Peña-Muñoz1964, pl. 1, fig. 1; Fig. 8.10) shows the same appearance, with sculpture smoothing of the body chamber. However, sculpture smoothing cannot be considered typical of the genus Mazapilites because coarsely sculptured body chambers are known for M. crassicostatus Burckhardt, Reference Burckhardt1919 (Burckhardt, 1919–Reference Burckhardt1921, p. 8, pl. 2, figs. 1, 6; Fig. 7.11, 7.12).
(3) Ribbing composed of simple ribs and unstable, defectively realized ‘bifurcations’ was identified (Fig. 7.1–7.12), resulting in the occurrence of more or less clear intercalatory ribs (Burckhardt, Reference Burckhardt1906, p. 108, 109; 1919–Reference Burckhardt1921, p. 3, 5, 7–10). Variable rib coarseness was identified within a given species (Burckhardt, Reference Burckhardt1906, p. 6, Eurynoticeras = Mazapilites zitteli; Burckhardt, Reference Burckhardt1919, p. 8, M. zitteli, p. 9, 10, M. tobosensis Burckhardt, Reference Burckhardt1919). Although sculpture (i.e., ribbing) was considered similar among the species described (Burckhardt, 1919–Reference Burckhardt1921, p. 3), a slight difference in sculpture was used for species identification (Burckhardt, 1919–Reference Burckhardt1921, p. 8 for M. crassicostatus vs. coarse-ribbed M. zitteli; p. 9 for M. crassicostatus vs. Mazapilites sp. indet. illustrated on pl. 2, figs. 5, 8, 9; and p. 10 for M. carinatus Burckhardt, Reference Burckhardt1919 vs. M. tobosensis). Rib crowding was considered variable (Burckhardt, Reference Burckhardt1906, 1919–Reference Burckhardt1921).
(4) Whorl sections change from more or less oval to acute during ontogeny, but ovate whorl sections can persist throughout ontogeny, and some cases of acute whorl sections (“forme d’une fleche”) were identified in the preserved outer whorls (Burckhardt, Reference Burckhardt1906, p. 108; 1919–Reference Burckhardt1921, p. 3).
(5) Suture line analysis was detailed, revealing some degree of suture variability in Burckhardt’s descriptions (Burckhardt, Reference Burckhardt1906, p. 109, 110; 1919–Reference Burckhardt1921, p. 3, 5, 6, 8, 10, 11), the systematic significance of which, at the species level, was correctly subordinated to shell morphology and sculpture (Burckhardt, Reference Burckhardt1906, p. 110, but see Burckhardt, 1919–Reference Burckhardt1921, p. 6). In addition, shell morphology and sculpture were recognized as resulting in polymorphic combinations, especially for his species M. zitteli (“bastante polimorfa,” Burckhardt, 1919–Reference Burckhardt1921, p. 2, 6).
(6) Venter ‘tricarination’ with variable development occurs during an ephemeral ontogenetic phase in some of the species erected based on material collected from the “capas superiores con Mazapilites” at the Cañón del Toboso, Sierra de Symón, Durango (Burckhardt, 1919–Reference Burckhardt1921, p. 2, 3, and p. 4, 5 for M. symonensis, p. 7 for M. crassicostatus, p. 9 for M. tobosensis, and p. 10 for M. carinatus; Burckhardt referred this section or ‘locality’ as belonging to the Zacatecas state).
Observations on the type material available from old collections and the recently collected material additionally show that:
(7) No complete specimens exist, because crushing affected the four existing cases of incomplete body-chamber preservation (a fifth one is an isolated, recently collected fragment of dubious interpretation) and, therefore, very limited information is available about the body chamber’s sculptural variability.
(8) Smoothed and sculptured body chambers developed in Mazapilites (e.g., M. symonensis and M. crassicostatus, respectively), but one doubt is evident: was sculpture smoothing in the body chamber exclusive of M. symonensis or was it a typical feature of final growth? Hence, the well-sculptured body chamber known in M. crassicostatus could indicate incomplete growth or serve to identify a smaller, separate species.
(9) Ribbing consists mainly of simple and intercalatory ribs, which sometimes connect to umbilical or primary ribs at the midflank inflexion (‘knickung’ or ‘codo’ of Burckhardt, 1919–Reference Burckhardt1921, p. 5, 7, 8). The more accentuated the rib inflexion, the clearer these connections are, the connections being realized in aboral or adapertural position with respect to the umbilical or primary ribs; very common, even dominant, is the occurrence of obscure or incomplete rib connections.
(10) Burckhardt’s species Mazapilites crassicostatus shows a difference in rib coarseness on the inner whorls, whereas specimens with coarser ribs at the same shell size and collected from the same ‘locality’ (Cañón del Toboso) were interpreted as a potential new species of Mazapilites (e.g., Burckhardt, 1919–Reference Burckhardt1921, p. 9, pl. 2, figs. 5, 8, 9). Updated reinterpretation cannot deny the possibility of intraspecies diversity, as was suggested for M. zitteli by Burckhardt (1919–Reference Burckhardt1921, p. 2).
(11) The general, ontogenetic trend for higher and narrower whorl sections is less accentuated in Mazapilites crassicostatus (e.g., Burckhardt, 1919–Reference Burckhardt1921, p. 7).
(12) The thorough analysis of suture lines made by Burckhardt was correctly interpreted in general (but see Burckhardt, 1919–Reference Burckhardt1921, p. 6), although without paying particular attention to the potential bias forced by preservation in silty-limy to fine sandy, phosphoritic deposits.
(13) Venter ‘tricarination’ seems to be a constructional effect rather than one related to real tuberculation; it is affected by preservation and the convexity of flanks, and can disappear early or late in ontogeny (see also Burckhardt, 1919–Reference Burckhardt1921, p. 2, 3, 5, 7, 9–11). There are cases of real, subtle, subrounded to elongated tubercles only at the midventral line (Fig. 9.4?, 9.5?, 9.6); the ventrolateral swellings mentioned by Burckhardt (e.g., Burckhardt, 1919–Reference Burckhardt1921, ‘hinchamientos’ on p. 2, 3, 9 and ‘ensanchamientos’ on p. 5, 7, 11) are not real tubercles, yet show longitudinal elongation, and seem to be related to the differentiation of relatively well-developed, distinct shoulders—i.e., the more accentuated the angular transition between the flanks and the ventral region, the more enhanced the ventrolateral reliefs (Fig. 9.4, 9.6 vs. 9.1, 9.2, 9.5) identified between 30 and 40 mm in the revised material. An exception is a case at 60 mm diameter in the plaster cast USNM PAL 103295 corresponding to the type of Mazapilites mexicanus illustrated by Del Castillo and Aguilera (Reference Del Castillo and Aguilera1895, pl. 6, fig. 8).

Figure 9 Ventral views of specimens of Mazapilites showing variable development of the ventral tubercles and ventrolateral reliefs (prominences) presumably related to shell inflation: (1) M. mexicanus, USNM PAL 103295, plaster cast, San Luis Potosí State, Sierra de Catorce, lower Tithonian (see Del Castillo and Aguilera, Reference Del Castillo and Aguilera1895, pl. 6, fig. 8); (2) M. crassicostatus, IGM 1564, eastern Durango State, Cañón del Toboso, lower Tithonian; (3) Mazapilites? sp., IGM 4715, San Luis Potosí State, Sierra de Catorce, lower Tithonian; (4) M. crassicostatus, IGM 2684, eastern Durango State, Cañón del Toboso, lower Tithonian; (5) M. tobosensis, IGM 1567, eastern Durango State, Cañón del Toboso, lower Tithonian; (6) M. symonensis, IGM 1562, eastern Durango State, Cañón del Toboso, lower Tithonian. Scale bar = 1 cm. See text for explanation.
(14) Isolated, incomplete, large inner casts affected by variable crushing and erosion, collected by Verma and Westermann (Reference Verma and Westermann1973, pl. 26, fig. 7; Fig. 8.3), Olóriz et al. (Reference Olóriz, Villaseñor, González-Arreola and Westermann1999, pl. 7, figs. f, g; Fig. 8.4), and Villaseñor et al. (Reference Villaseñor, González-León, Lawton and Aberhan2005, pl. 4, fig. d; Fig. 8.1) from silty-sandy and phosporitic deposits, are large phragmocones between 60 and 90 mm shell diameter. These specimens show comparatively little sinuous ribbing with low intercalatories typical of the morphological group represented by Mazapilites mexicanus, but the absence of body chamber preservation impedes their conclusive interpretation at the species level. Small (~30 mm) phragmocones preserved in siltstone are difficult to interpret (e.g., Peña-Muñoz, Reference Peña-Muñoz1964, pl. 1, fig. 2; Fig. 8.9).
(15) Measurements of shell diameter, coiling degree, and ribbing conducted on the type material available from old collections and the recently collected material are represented in Table 3 and Figures 10 and 11. The obtained information indicates that:

Figure 10 Bivariate plots of studied Mazapilites: (1) umbilicus (U) vs. shell diameter (Dm); (2) U/Dm ratio vs. shell diameter (Dm). C and A = Del Castillo and Aguilera, Reference Del Castillo and Aguilera1895; V and W = Verma and Westermann, Reference Verma and Westermann1973, from San Luis Potosí State, Sierra de Catorce, lower Tithonian. See text for explanation.

Figure 11 Ribbing curves and costal index of studied Mazapilites: (1) CI vs. Dm; (2) ER/2 vs. Dm; (3) UR/2 vs. Dm. Shell diameter (Dm) in millimeters. Abbreviations as in Figure 10. See text for explanation.
Table 3 Measurements (in mm, rounded decimal figures) of Mazapilites spp. from various authors, as indicated, in chronological order for each species. Measurements of the new material obtained (two specimens) are added at the end of the table.

Abbreviations as in Table 1; + = phragmocone diameter.
(15.1) The range of shell size in specimens belonging to the nominal species of Mazapilites are 32 mm for M. carinatus (a single, lost phragmocone), close to 40 mm for M. tobosensis (the single known phragmocone), 33–65 mm for M. zitteli (seven existing phragmocones), 47–72 mm for M. crassicostatus (two existing specimens with preserved body chambers), 61–86 mm for M. mexicanus (five existing phragmocones, a plaster cast, and a fragment of dubious interpretation), and 38?–68–119 mm for M. symonensis (two existing phragmocones and two specimens, including body-chamber preservation; interpretation of the smallest phragmocone is dubious). Of six nominal species, four are represented by small to medium-sized phragmocones (up to 65 mm), but one species (M. crassicostatus) includes two specimens with body chambers of <70 mm; one species (M. mexicanus) is represented by medium-sized to large phragmocones; and a single species (M. symonensis) includes adult body-chamber preservation. The interpretation of small phragmocones is inconclusive (e.g., Peña-Muñoz, Reference Peña-Muñoz1964, pl. 1, fig. 2; Fig. 8.9).
(15.2) Coiling degrees expressed by the U/Dm ratio (Fig. 10.1) are uniform among the nominal species of Mazapilites, and show a clear trend for wider umbilici at larger shell diameters (~60 mm and larger), as well as some cases of <60 mm in M. mexicanus and M. symonensis (Fig. 10.1, 10.2). The use of the U/Dm ratio vs. Dm (Fig. 10.2) reveals a general, decreasing trend during growth, except for some phragmocones between 40 and 86 mm interpreted as M. mexicanus, and in some cases interpreted as M. symonensis (Fig. 10.2).
(15.3) Ribbing was investigated in terms of the number of periumbilical or primary ribs per half whorl (UR/2), the number of external ribs per half whorl (ER/2), and the costal or ribbing index represented as the number of external ribs per 10 primary ribs (CI) (Fig. 11).
CI behavior (Fig. 11.1) is cogruent with that shown by ER/2 (Fig. 11.2), even featuring deviations in the same two small phragmocones mentioned as exceptions for ER/2 curves.
ER/2 (Fig. 11.2) displays a general trend for increasing numbers of external ribs up to ~90 mm diameter, at which point sculpture smoothing is observed together with wider rib interspaces and a proliferation of lirae affecting body chambers in large shells (M. symonensis). Three exceptions are the small phragmocone (<40 mm) interpreted as M. symonensis by Peña-Muñoz (Reference Peña-Muñoz1964, pl. 1, fig. 2), the most probably the immature specimen of M. crassicostatus with crushed body chamber illustrated by Burckhardt (1919–Reference Burckhardt1921, pl. 2, fig. 1), and an incomplete specimen (<60 mm) interpreted as M. mexicanus collected by the authors from the Al-5 section (IGM 4714; Fig. 8.5, 8.6).
UR/2 (Fig. 11.3) illustrates the incidence of shell size in the number of primaries, which are especially strong in some nominal species and can show dense crowding of fine striations early during ontogeny (<30 mm). A rather constant number of primary ribs occurs in phragmocones between 30 and 60 mm (with only two rib fluctuations). A slight increase in variation in primary rib crowding is recorded between 60 and 90 mm (four rib fluctuations). There is a clear decrease in the number of primary ribs in larger shells, related to sculpture smoothing of adult body chambers (at present only known from M. symonensis). The overall intranominal species variability in the number of primary ribs is higher for M. mexicanus and M. symonensis, which are the two large-shelled Mazapilites species.
Based on a revision of available material interpreted as nominal species of the genus Mazapilites by various authors, some traits can be outlined for an updated yet preliminary interpretation: (1) shell type, in terms of coiling and whorl section, is well established in Mazapilites; shell type variability and sculpture coarseness could be combined and interpreted at the intraspecies level with a reduction of nominal species; (2) the whorl section covaries with ribbing coarseness, showing coarser sculpture in wider shells (at the ventral area), resulting in a relatively high phenotype variability (e.g., Burckhardt, Reference Burckhardt1906, p. 2, 6); M. mexicanus could represent an intermediate case; (3) ventral ‘tricarination’ is essentially a constructional effect because no real ventrolateral tuberculation occurs (Fig. 9); (4) the sculptural pattern in Mazapilites is fairly constant in terms of rib crowding in phragmocones, however, variable inflexion of primary ribs at the midflank forcing connections with external ribs occurs and, therefore, the number of intercalatories has a rather unstable pattern (Figs. 7, 8); in fact, variation in ribbing within and among the nominal species is incompletely known; (5) a relatively large intraspecies variability was originally suggested for M. zitteli, the sole nominal species “trouvé dans les conches phosphoritiques rouges de la Sierra de Mazapil en grande abondance” (Burckhardt, Reference Burckhardt1906, p. 108, with 10 specimens illustrated on pl. 29 and 30 if pl. 29, fig. 15 is considered as a separate specimen from those shown in his figs. 16, 17); (6) a majority of nominal species of Mazapilites are known from phragmocones, and the interpretation of smaller ones is inconclusive; the lack of body chamber in larger specimens impedes evaluation of the potential relationship with specimens (adults) having preserved body chambers; (7) body-chamber preservation is rare, therefore, knowledge about ontogeny is inconclusive, with the exception of one of the existing nominal species; (8) specimens with body-chamber preservation are only known for Burckhardt’s species M. crassicostatus and M. symonensis, the former probably pertaining to immature specimens whereas the latter shows adult, mature specimens (Figs. 7.10, 8.10); (9) no precise stratigraphy is available for most of the records of Mazapilites; this suggests incomplete knowledge about the biostratigraphic ranges of the nominal species, and makes difficult any attempt to interpret potential evolutionary lineages; and (10) measurements of coiling and ribbing conducted on the available material of the Mexico-Caribbean endemic genus Mazapilites indicate that reinterpretation at the species level calls for a thorough revision based on new material collected bed-by-bed.
Discussion (with a chronological revision of previous reports of Hybonoticeras and Mazapilites from Mexico and Cuba, and updated interpretation of their co-occurrence)
On the whole, a major difficulty identified during this revision concerns the level of stratigraphic accuracy characterizing pioneer and relevant works in the area. The reference section at the Cañón del Toboso (Burckhardt, 1919–Reference Burckhardt1921), which provided the original material on which the genus Mazapilites was erected, is a good example. Relevant information provided by Burckhardt (1919–Reference Burckhardt1921) in his introductory chapter indicated the provenance of the studied material and the timing of publication. Later, revealing data were provided by Böse (Reference Böse1923), who showed interesting information on: (1) the provenance of Upper Jurassic fossils from the Sierrita de Symón, and the publication context (Böse, Reference Böse1923, p. 5, 6); (2) the use and meaning of stratigraphic terms such as ‘capa,’ ‘banco,’ and ‘horizonte,’ used in his field surveys and most probably revealing standard procedures among good field geologists and paleontologists (Böse, Reference Böse1923, p. 19–26); and (3) the respective accuracy of the collection of Upper Jurassic fossils and field stratigraphy, to derive their potential influence in Burckhardt’s work (Böse, Reference Böse1923, p. 63, 64). Finally, Burckhardt (Reference Burckhardt1930, p. 55, 56, fig. 17) provided precise information on the origin of the first stratigraphic section known from the Cañón del Toboso at the Sierra de Symón (see earlier comments by Böse, Reference Böse1923). As expected, differences in sampling strategies, the influences of the assumed scientific authority at the time, and other factors, determined interpretations until the last decade of the past century, and made precise biostratigraphic control and correlation difficult tasks. In the following text, we will attempt clarification.
The following revision of publications focused on the genera Hybonoticeras and Mazapilites collected by various authors in the Mexico-Caribbean area is presented chronologically according to their original interpretations. The corresponding reviews will follow the original interpretations in each of the contributions considered.
Unfortunately, reports of the genus Hybonoticeras from Cuba are comparatively recent (Myczyński, Reference Myczyński1998; Pszczółkowski and Myczyński, Reference Pszczółkowski and Myczyński2010, p. 232), scarce, poorly preserved, and, therefore, barely illustrated (Myczyński, Reference Myczyński1999, figs. 5.1, 5.2, 6; Pszczółkowski and Myczyński, Reference Pszczółkowski and Myczyński2010, fig. 17.1). Such a situation impedes a conclusive revision. The co-occurrence of Hybonoticeras with Mazapilites in Cuba has been not illustrated, although an Hybonoticeras-Mazapilites Biozone was first proposed by Myczyński (Reference Myczyński1999, fig. 3) for Sierra de los Órganos in western Cuba. These topics will be considered in the last two subsections.
The occurrence of Hybonoticeras in Mexico
As early as 1906, Burckhardt (Reference Burckhardt1906, p. 103–105) identified “Waagenia sp. ind. (plusieurs espèces),” the taxon name erected by Neumayr (Reference Neumayr1878) for the group of ‘hybonoten’ that he separated from the genus Aspidoceras Zittel, Reference Zittel1868, and which was subsequently replaced by the new taxon name Hybonoticeras proposed by Breistroffer (Reference Breistroffer1947).
Concerning Waagenia, Burckhardt (Reference Burckhardt1906, p. 103) noted “assez nombreux ... exemplaires ne permettent pas une détermination certaine, car on n’en peut pas apprécier les dimensions ni observer les cloisons.” Among the five specimens illustrated by Burckhardt (Reference Burckhardt1906, pl. 27, figs. 1–5) as Waagenia sp. indet. from Mazapil, Zacatecas, a single specimen was considered identical to W. harpephora Neumayr in Fontannes, Reference Fontannes1879 (Burckhardt, Reference Burckhardt1906, pl. 27, fig. 1). Numerous specimens were interpreted as close to W. harpephora, but only two were illustrated (Burckhardt, Reference Burckhardt1906, pl. 27, figs. 2, 3). A single specimen was interpreted as probably conspecific with W. knopi (see Burckhardt, Reference Burckhardt1906, pl. 27, fig. 5), and one as related to W. beckeri (see Burckhardt, Reference Burckhardt1906, pl. 27, fig. 4). All of the illustrated specimens were incomplete and/or small, and were preserved in siltstone. Burckhardt (Reference Burckhardt1906, p. 178) correlated his “argiles à Waagenia” with the “calcaires à Waagenia beckeri” that he correctly identified with the “sommet du Kimmeridgien” in southeastern France.
Revision of the types illustrated by Burckhardt (Reference Burckhardt1906, p. 104, 105, pl. 27, figs. 1–5) indicates that on the basis of the illustrated material, the interpretation of Waagenia (= Hybonoticeras) was basically correct in reference to forms morphologically close to, or belonging to, the groups of H. beckeri (see Burckhardt, Reference Burckhardt1906, pl. 27, fig. 4; Fig. 12.4) and H. harpephorum (Neumayr, Reference Neumayr1873) (sensu Neumayr, Reference Neumayr1873 and Fontannes, Reference Fontannes1879; Burckhardt, Reference Burckhardt1906, pl. 27, figs. 1–3; Fig. 12.1, 12.2, 12.5). More debatable is Burckhardt’s interpretation of the specimen that he related to H. knopi (see Burckhardt, Reference Burckhardt1906, pl. 27, fig. 5; Fig. 12.3). Even assuming general correctness in Burckhardt’s interpretations, morphological traits in the ammonites that he illustrated point to a more or less evident separation from the European types mentioned (local to regional effects?). Thus, Mexican specimens related to the group of H. harpephorum, rather than to Neumayr’s type, show a greater frequency of bifurcations on the umbilical edge and of intercalatory ribs (including the specimen he envisaged as close to the H. knopi group), along with coarser and less rigid ribbing (Fig. 12.1–12.3, 12.5). The specimen that Burckhardt related to the group of H. beckeri shows a more evolute shell with more stiff, simple ribs on the inner whorls than in Neumayr’s type, and on slightly later whorls, regular, coarse geminate (looped) ribs suggesting morphological relationships with H. beckeri ornatum (Spath, Reference Spath1931) (or simply H. ornatum, depending on the preference of given authors), but lacking its typical, fine, irregular, geminate ribbing on the inner whorls. Therefore, the occurrence of a local variant of the group of H. beckeri sensu stricto is probable (Fig. 12.4). The age of the species cited is late to latest Kimmeridgian elsewhere in the world, but a conclusive interpretation of phenotypic differences with respect to the aforementioned European species will depend on new material collected bed-by-bed.
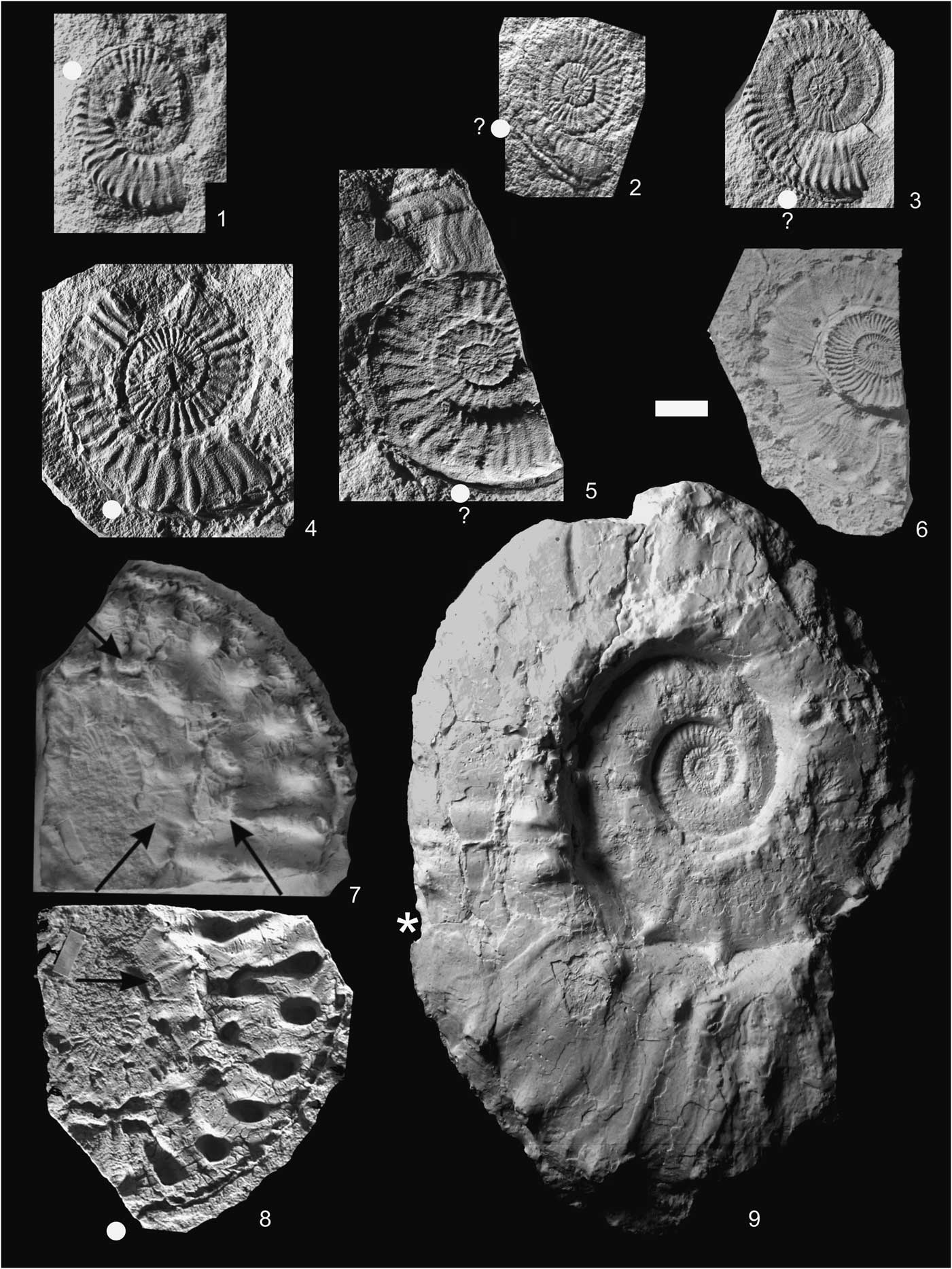
Figure 12 Macroconchiate Hybonoticeras from Burckhardt (Reference Burckhardt1906, from Sierras de Mazapil and Santa Rosa, northern Zacatecas State, uppermost Kimmeridgian), Burckhardt (1919–Reference Burckhardt1921, from Cañón del Toboso, eastern Durango State, most probably from horizons close to the Kimmeridgian-Tithonian boundary), and Imlay (Reference Imlay1939, from the northern side of the Cañón del Toboso, Sierra del Chivo, eastern Durango State, most probably from the uppermost Kimmeridgian): (1) Waagenia sp., IGM 242, from Burckhardt (Reference Burckhardt1906, pl. 27, fig. 2); (2) W. sp., IGM 281, from Burckhardt (Reference Burckhardt1906, pl. 27, fig. 1); (3) W. sp., IGM 241, from Burckhardt (Reference Burckhardt1906, pl. 27, fig. 5); (4) W. sp., IGM 240, from Burckhardt (Reference Burckhardt1906, pl. 27, fig. 4); (5) W. sp., IGM 196, from Burckhardt (Reference Burckhardt1906, pl. 27, fig. 3); (6) W. sp. indet. (lost specimen; reproduced from Burckhardt, 1919–Reference Burckhardt1921, pl. 4, fig. 11); (7, 8) W. cf. W. hybonota, IGM 1707, from Burckhardt (1919–Reference Burckhardt1921, pl. 4, fig. 12), plaster cast of the original imprint (7) and original imprint (8), black arrows indicate lumped ribs and the beginning of intertubercular ribs that could result in potential ‘quasi-V’ rib connections due to the higher number of outer tubercles; (9) W. parrasensis, plaster cast of UM 17623, from Imlay (Reference Imlay1939, pl. 3, fig. 12). White dots indicate last preserved suture lines; asterisks mark beginnings of the preserved body chambers. Scale bar = 1 cm. See text for updated reinterpretation.
Burckhardt (1919–Reference Burckhardt1921, p. 14, pl. 4, fig. 11; Fig. 12.6) described a single ammonite imprint from the Cañón del Toboso, Durango, as Waagenia sp., and suggested its close similarity with Ammonites autharis, the only one of the two species of hybonoticeratines erected by Oppel (Reference Oppel1863) with a known lateral view. Burckhardt (1919–Reference Burckhardt1921, p. 14, 15) emphasized the pattern of ribbing and tuberculation, as well as the rather irregular, sinuous, fine ribs preserved on the outer whorl. Burckhardt (1919–Reference Burckhardt1921, p. 15, 61, 64, 65) furthermore indicated its provenance from the “capas inferiores con Mazapilites,” which he correlated with the base of his Portlandian, interpreted as equivalent to the “capas superiores de Crussol,” the “zona de la Oppelia lithographica,” and the “capas de Solnhofen” containing A. autharis. Because the Mexican specimen is lost, only the illustration can be analyzed.
A second isolated imprint collected at the Pico de Teyra, Zacatecas, was briefly commented on and illustrated by Burckhardt (1919–Reference Burckhardt1921, footnote on p. 15, and pl. 4, fig. 12; Fig. 12.7, 12.8), yet he did not provide stratigraphic data or the precise section, and interpreted this record as “Waagenia cfr. hybonota Oppel sp.” Burckhardt (1919–Reference Burckhardt1921) emphasized observations on the outer whorl, especially the occurrence of two rows of prominent tubercles linked by prominent ribs, which he interpreted as closely resembling forms illustrated by Oppel (Reference Oppel1863, pl. 71, figs. 1–3), Benecke (Reference Benecke1866, pl, 11, fig. 1), and Favre (Reference Favre1877, pl., 8, fig. 1). Subsequently, Burckhardt (Reference Burckhardt1930, p. 69) simply referred to this record from the Pico de Teyra as gathered from the “couches a Mazapilites” without offering more precise comments about its provenance from a particular section.
Callomon (Reference Callomon1992, p. 267) assumed Burckhardt’s (1919–Reference Burckhardt1921, p. 14, 15, pl. 4, fig. 11; Fig. 12.6) interpretation of one specimen as close to Waagenia autharis. Revision of Burckhardt’s illustration shows that the sculpture on the inner whorls might be compatible with that seen in a young specimen of Hybonoticeras autharis illustrated by Oppel (Reference Oppel1863, pl. 71, fig. 5), however, ribs in the Mexican type are subtly rursiradiate (preservation effects?), whereas they are radial to slightly prorsiradiate in the German specimen shown by Berckhemer and Hölder (Reference Berckhemer and Hölder1959; see also Zeiss, Reference Zeiss2001). Moreover, the irregular, fine ribbing preserved on the outer whorl of the Mexican specimen is very different from that of H. autharis by Oppel (Reference Oppel1863) and resembles Hybonoticeras of the H. beckeri group, especially if the looping of the ribs is real (e.g., just at the beginning, the middle, and the last part of the outer whorl in what appears entirely to be a phragmocone; Fig. 12.6). In such a case, updated age interpretation points to latest Kimmeridgian rather than Tithonian horizons.
Burckhardt (1919–Reference Burckhardt1921, p. 64, 65) interpreted the “capas inferiores con Mazapilites” as the stratigraphic interval that provided his Waagenia sp. indet. (“cfr. W. autharis Oppel sp.”), but he did not provide the precise thickness of this interval or the relative location of this specimen. An early Tithonian age was assumed by Burckhardt (1919–Reference Burckhardt1921, p. 65) for his Waagenia. The interpretation of this incomplete specimen is not conclusive at present and a latest Kimmeridgian age is rather favored.
The interpretation of Waagenia cf. W. hybonota by Burckhardt (1919–Reference Burckhardt1921, p. 15, pl. 4, fig. 12; Fig. 12.7, 12.8) of the isolated imprint gathered at the Pico de Teyra casts doubts. Burckhardt (1919–Reference Burckhardt1921) alluded to types illustrated by Oppel (Reference Oppel1863), Benecke (Reference Benecke1866), and Favre (Reference Favre1877). However, Oppel’s illustrations of Ammonites hybonotus are not appropriate for comparison because they are exclusively ventral views; the Benecke type shows very different inner whorls; and the Favre type has no preserved inner whorls or suture lines. Revision of the imprint interpreted as “Waagenia cfr. hybonota Oppel sp.” housed at the IGM (Fig. 12.7, 12.8) shows that: (1) it belongs entirely to the imprint of a phragmocone; (2) the first three whorls had simple, straight ribs; (3) small outer protuberances or tubercles could occur at < 10 mm, whereas small outer tubercles at 10 mm preceded the occurrence of inner tubercles on the umbilical edge; (4) there were looped ribs on the penultimate whorl of the specimen, and a structure compatible with the potential development of ‘quasi-V’ rib connections at the end of the outer whorl is preserved on the imprint, given the first occurrence of intertubercular ribs and the higher number of outer tubercles; (5) the keels were made of two rows of crowded, small, spiny, and rather oblique tubercles; and (6) no observations can be made about the ventral groove. If the comparisons made by Burckhardt (1919–Reference Burckhardt1921) are suggestive of what today is interpreted as Hybonoticeras pseudohybonotum or other close forms, the features enumerated above discard this possibility, given the clear difference in the inner whorls of Burckhardt’s type with respect to Benecke’s and Vigh’s types. Furthermore, the near absence of well-preserved inner whorls in specimens recently interpreted as related to H. pseudohybonotum (e.g., Haberl et al., Reference Haberl, Schairer, Schweigert and Zeiss1999; Zeiss, Reference Zeiss2001) deserves attention when interpreting Vigh’s species. The combination of the early occurrence of looped ribs and later coarse tuberculation could support a relationship between the imprint described by Burckhardt (1919–Reference Burckhardt1921) and the group of H. beckeri extraspinatum Berckhemer and Hölder, Reference Berckhemer and Hölder1959 (or simply H. extraspinatum, according to some authors). However, crushing of the inner cast and the difficulty of precise observation of the preserved penultimate whorl and of the inner whorls, which are partially recovered or not preserved, limits interpretation of the actual separation of the umbilical tubercles from the line of whorl overlap. A reasonable interpretation within the present state of knowledge seems to be a form close to H. sp. gr. H. beckeri extraspinatum. Hybonoticeras interlaevigatum Berckhemer, Reference Berckhemer1922 could be another close form, but this species is not well known from the illustrations of juveniles by Berckhemer (Reference Berckhemer1922, pl. 1, fig. 15) and Berckhemer and Hölder (Reference Berckhemer and Hölder1959, fig. 16), the lectotype proposed by Zeiss (Reference Zeiss2001, p. 65; Berckhemer and Hölder, Reference Berckhemer and Hölder1959, pl. 3, fig. 12), and the larger specimens illustrated by Schweigert et al. (Reference Schweigert, Krishna, Pandey and Pathak1996, fig. 5d) and Schweigert (Reference Schweigert2000, pl. 2, fig. 4). Hence, a latest H. beckeri Chron age is favored against the earliest H. hybonotum Chron, and its provenance from horizons very close to the Kimmeridgian-Tithonian boundary could apply.
Burckhardt (Reference Burckhardt1930) merely reproduced his previous interpretations about Mexican Waagenia (= Hybonoticeras), and no complementary revision applies.
Imlay (Reference Imlay1937, p. 600–604) described four sections and mentioned six fossiliferous localities from La Casita Formation throughout the middle part of Sierra de Parras, Cohahuila. No Waagenia species were cited in his fossiliferous localities (Imlay, Reference Imlay1937, p. 602, 603) nor in his Waagenia beds (Imlay, Reference Imlay1937, p. 604, table 3). Only his report of separate stratigraphic intervals for Waagenia and Mazapilites is relevant here.
Imlay (Reference Imlay1939, p. 12) reported Waagenia sp. from Puerto Blanco, Sierra de Santa Rosa, associated with involute aspidoceratids that he identified as Physodoceras Hyatt, Reference Hyatt1900. He suggested a youngest middle Kimmeridgian age, at the very top of the Streblites tenuilobatus Biozone, just below the Aulacostephanus eudoxus-Neochetoceras steraspis Biozone (sensu Spath, Reference Spath1933 in 1927–1933) that he used for correlation (Imlay, Reference Imlay1939, p. 21, tables 1, 2). Moreover, Imlay (Reference Imlay1939, p. 31, 32) erected W. parrasensis (Fig. 12.9) among the ammonites collected from concretions in the La Casita Formation at Sierra de Parras, Coahuila, without providing precise stratigraphic data about the section studied. He interpreted his single specimen as being close to W. hybonota, and referred to Oppel’s illustrations (Oppel, Reference Oppel1863, pl. 71, figs. 1–3). However, these illustrations only show ventral regions that have been the subjects of variable interpretations since Oppel’s times, including the most recent one by Zeiss (Reference Zeiss2001). Imlay (Reference Imlay1939, p. 32) considered W. beckeri to be less closely related morphologically to the new Mexican species. Imlay (Reference Imlay1939, table 10) placed his W. parrasensis at the top of his middle Kimmeridgian, just below the stratigraphic interval characterized by Torquatisphinctes Spath, Reference Spath1924 and Mazapilites.
Examination of a cast of the type of Waagenia parrasensis (UM 17623; Fig. 12.9) is congruent with the original description, but also shows smoothed looping of the ribs on the adoral part of the preserved incomplete body chamber. This feature, plus those following, are relevant in an updated interpretation of a secondary, subordinate meaning of the Imlay type: (1) the differential development of tuberculation on the penultimate whorl, which shows spiny and well-developed external tubercles; (2) the fading of subtle ribs before reaching the shoulders; and (3) the wide umbilical wall severely affected by crushing in the preserved outer whorl. On this basis, and with the limits imposed by the unpreserved inner whorls of the holotype of Hybonoticeras beckeri extraspinatum (see Berckhemer and Hölder, Reference Berckhemer and Hölder1959, p. 30, pl. 4, fig. 17), the type of the subspecies erected by Berckhemer and Hölder (Reference Berckhemer and Hölder1959) is the morphologically closest macroconchiate Hybonoticeras, most probably revealing a lineage relationship. In contrast, a clear difference in sculpture exists between the inner whorls of Imlay’s type and those in the H. extraspinatum-interlaevigatum group. Therefore, assuming age correlation for phenotype similarity among Hybonoticeras species from different areas, the envisaged morphological relationships among the types described by Imlay, Berckhemer and Hölder, and Berckhemer point to a late beckeri Chron age for H. parrasensis according to data retrieved from European sections (e.g., Berckhemer and Hölder, Reference Berckhemer and Hölder1959; Olóriz, Reference Olóriz1978; De Wever et al., Reference De Wever, Geyssant, Azema, Devos, Duée, Manivit and Vrielynck1986; Schweigert et al., Reference Schweigert, Krishna, Pandey and Pathak1996; Schweigert, Reference Schweigert1998).
According to Imlay (Reference Imlay1939, p. 22, 35), ammonites associated with his Waagenia parrasensis were Torquatisphinctes? aff. T. diversecostatus (Burckhardt, Reference Burckhardt1919) and Subdichotomoceras? sp. (Imlay, Reference Imlay1939, p. 22), or Aulacosphinctoides? (Subdichotomoceras?) sp. (Imlay, Reference Imlay1939, p. 35). The last was interpreted as an immature specimen of the large perisphinctids that Burckhardt (1919–Reference Burckhardt1921) identified as Perisphinctes Waagen, Reference Waagen1869 and P. (Aulacosphinctes), such as P. alexii Burckhardt, Reference Burckhardt1919 and P. diversecostatus (see Imlay, Reference Imlay1939, p. 22, 35), together with Glochiceras sp., Aulascosphinctoides? (Subdichotomoceras?) sp. and Aulacosphinctoides aff. A. diversecostatus (see Imlay, Reference Imlay1939, table 10). These identifications point to rather incomplete ammonites showing a more or less loosely coiled, ‘colubrinoid’ shell and sculpture on the inner whorls, and simple, Torquatisphinctes-like ribbing in comparatively more globose, incomplete shells, thus being of no help in determining precise biostratigraphy.
Imlay (Reference Imlay1943, p. 531, 532) provided a chart of “faunal divisions of northern Mexico,” including a Waagenia interval, and reported many small specimens of Waagenia spp. collected from shales and sandtones in Placer de Guadalupe, eastern Chihuahua. He interpreted these records as identical to those illustrated by Burckhardt (Reference Burckhardt1906, p. 103–105) as Wagenia sp., highlighting similarities with W. harpephora, W. knopi, and W. beckeri. Although Imlay (Reference Imlay1943) maintained his previous biostratigraphic interpretations (Imlay, Reference Imlay1939), he noticed a significant increase in thickness between his beds with Waagenia and those containing ammonites that he identified as Subplanites Spath, Reference Spath1925, Virgatosphinctes Uhlig, Reference Uhlig1910, and Kossmatia Uhlig, Reference Uhlig1910. Imlay (Reference Imlay1943) did not provide illustrations of the Waagenia spp. collected in Placer de Guadalupe or data about other accompanying ammonites at locality 17254 sampled by the Texas Bureau of Economic Geology staff. Reinterpretation of the Waagenia spp. that Imlay (Reference Imlay1943) reported without illustration from his locality 17254 in the Placer de Guadalupe district would be difficult. His report does not allow for precise age interpretations, and any attempt would be inconclusive because no information exists about associated ammonites. The information provided by Imlay (Reference Imlay1943) can only be considered as occurrence data.
Imlay (Reference Imlay1953, p. 50) reported Waagenia as the only ammonite genus collected from brownish shales including a limestone bed intercalated at the Vereda del Quemado section, Sierra de La Caja, north of Mazapil, Zacatecas. He considered the stratigraphic interval to be 30 m thick, and to represent his middle Kimmeridgian. Because Imlay (Reference Imlay1953) did not mention species-level identifications or precise biostratigraphy, no precise, updated interpretation is available, and the information that he provided can only be considered as occurrence data. The same applies to that provided by Imlay (Reference Imlay1965) who merely mentioned a biostratigraphic unit with Hybonoticeras.
Erben (Reference Erben1957: pl. 2) interpreted a correlation chart with a “faunal sequence in Mexico,” partially following previous stratigraphic correlations (Imlay, Reference Imlay1953, reported as Reference Imlay1952) and showing a Waagenia interval below a Mazapilites interval. These stratigraphic intervals were correlated with the biozone of Gravesia gravesiana (d’Orbigny, Reference d’Orbigny1850) and G. gigas (Zieten, Reference Zieten1830) for the former, and with the stratigraphic interval defined by the total range of Subplanites sp.-S. wheatleyensis-Pectinatites pectinatus-Pavlovia rotunda-Pavlovia pallasioides for the latter. Updated interpretation indicates that the former stratigraphic interval basically corresponds to the lowermost Tithonian hybonotum Zone, whereas the latter embraces lower levels of the lower Tithonian up to the lowermost upper Tithonian and the lower middle Volgian according to authors (e.g., Verma and Westermann, Reference Verma and Westermann1973; Gerasimov et al., Reference Gerasimov, Kuznetsova, Mikhailov and Uspenskaya1975; Zeiss, Reference Zeiss2003; Rogov and Zakharov, Reference Rogov and Zakharov2009). Thus, the former stratigraphic interval refers to that typical for lower Tithonian hyonoticeratines, but in absence of paleontological descriptions, no interpretation at the species level, which is relevant for precise biostratigraphy, is possible. The information provided by Erben (Reference Erben1957) can only be considered as occurrence data.
Enay (Reference Enay1962) approached a general context for correlation of Mexican and European fauna based on a revision of the literature available at the time. Enay (Reference Enay1962, p. 364, table 4) used data compiled by Arkell (Reference Arkell1956) to interpret a lower stratigraphic interval with Hybonoticeras in Mexico, for which he suggested a latest Kimmeridgian age corresponding to the beckeri Zone. This interpretation was basically correct based on the information available at the time, and agrees, on the whole, with the results of the present revision.
Imlay (Reference Imlay1980) mentioned Hybonoticeras, without illustration, from subsurface records of eastern and northeastern Tamaulipas, these probably being the most northeastern records reported from Mexico. They were indirectly interpreted as lowermost Tithonian (Imlay, Reference Imlay1980, p. 33), which agrees with his correlation charts (Imlay, Reference Imlay1980, figs. 16, 21) assuming the co-occurrence of the youngest Hybonoticeras and the oldest Mazapilites (with doubts for the Gulf of Mexico region and nearby areas; Imlay, Reference Imlay1980, fig. 21). As for the cases above, no biostratigraphic or species-level interpretations are available, and the information provided by Imlay (Reference Imlay1980) can be considered only as occurrence data.
Imlay (Reference Imlay1984, p. 10, fig. 6) interpreted the record of Hybonoticeras in northern and eastern Mexico as latest Kimmeridgian to earliest Tithonian in age, and placed below Mazapilites. In the absence of paleontological descriptions, this information can only be considered as occurrence data with basic mention of the relative stratigraphic, topological, position between these two genera.
Contreras et al. (Reference Contreras, Gómez Luna, Martínez Cortés and González Casildo1991) illustrated two macroconchiate specimens of Hybonoticeras within a compilation of Mexican ammonites sponsored by the Instituto Mexicano del Petróleo. These specimens, gathered from Aramberri, southeastern Nuevo León, and Los Alamitos, Sierra de Catorce, Zacatecas, were respectively interpreted as H. (Hybonoticeras) beckeri aff. H. beckeri and H. (Hybonoticeras) beckeri aff. H. beckeri harpephorum. In the absence of data about precise sections and stratigraphic horizons, they were interpreted to indicate the upper Kimmeridgian.
Examination of the illustrations by Contreras et al. (Reference Contreras, Gómez Luna, Martínez Cortés and González Casildo1991: RF-15 and Mc-5) shows two specimens interpreted as belonging to the Hybonoticeras beckeri group, indicating the upper Kimmeridgian. Without data about the precise section, stratigraphy, or potential body-chamber preservation, H. (Hybonoticeras) beckeri aff. H. beckeri, with provenance from Nuevo León, shows well-developed looping of rather strong ribs, and a narrow ventral groove. It is therefore better related to H. (Hybonoticeras) beckeri ornatum, or H. (H.) ornatum according to some authors, most probably as a local and coarsely ribbed variant. Lacking stratigraphic information as for the first specimen, H. (Hybonoticeras) aff. H. beckeri harpehorum, from Sierra de Catorce, Zacatecas, shows flattened flanks, rather rigid, rursiradiate ribs with strong, prorsiradiate inflexion on the shoulders, fine tuberculation on both the umbilical edge and the mentioned inflexion points, and a wide ventral groove delimited by two keels resulting from a small, oblique, acute serration. This fragmentary specimen, which in lateral view resembles some of Burckhardt’s Waagenia revised above (Fig. 12.1–12.3), most probably represents a new morphospecies related to the lineage of H. harpephorum, from which the most outstanding difference is the wide, shallow ventral groove (also see previous comments on Waagenia sp. illustrated by Burckhardt, Reference Burckhardt1906; Fig. 12.1–12.3). On the assumption of a phenotype-age correlation with European records of Hybonoticeras, a latest Kimmeridgian (uppermost beckeri Zone) is envisaged for the first specimen from Nuevo León, and a latest Kimmeridgian to earliest Tithonian age could probably apply for the second recovered from Zacatecas, considering recent findings by the authors in Sierra de Catorce, Zacatecas (this paper and research in progress).
Callomon (Reference Callomon1992) provided a new attempt at approaching a general context of correlation between Mexican and European faunas based on revisions of the available literature. Callomon (Reference Callomon1992, p. 267, table 12.3) interpreted his M9 faunal horizon to represent the “beds with Waagenia of Mazapil and Symón” to be correlated with the beckeri Zone, but he recognized uncertainty in a more precise correlation due to the scarcity of data about associated ammonites.
The interpretation by Callomon (Reference Callomon1992) of his M9 faunal horizon was basically correct, and he was especially right in highlighting doubts concerning reaching a precise biostratigraphic interpretation. Of particular interest is restricting the correlation potential of the “beds with Waagenia of Mazapil and Symón” to beckeri Zone horizons, because fossils indicating the lower part of the beckeri Zone have been not documented to date (Villaseñor et al., Reference Villaseñor, Olóriz and González-Arreola2000, p. 251, fig. 2; Reference Villaseñor, Olóriz, López Palomino and López-Caballero2012, p. 257, fig. 2). Callomon (Reference Callomon1992, p. 267) interpreted that all the Hybonoticeras figured from Symón (i.e., the Cañón del Toboso section, but restricted to illustrations by Burckhardt, Reference Burckhardt1906) seem to indicate the beckeri Zone. However, he (Callomon, Reference Callomon1992, p. 267) accepted the real occurrence in Mexico of Hybonoticeras cf. H. autharis in his M11 faunal horizon “beds with Waagenia and Mazapilites” at Toboso based on data by Burckhardt (1919–Reference Burckhardt1921, pl. 4, fig. 11). In this case, Callomon referred to Hybonoticeras from the Cañón del Toboso section to propose correlation with an upper part of the hybonotum Zone in his table 12.3 (potential protraction of the top of M11 within the lowermost horizon of the Semiformiceras darwini Biozone would result from a drawing artifact?). He interpreted the microconch of H. hybonotum as H. cf. H. autharis (but see above for updated reinterpretation). Based on the envisaged occurrence of a single specimen of the assumed H. cf. H. autharis, a precise correlation of the M11 faunal horizon proposed by Callomon (Reference Callomon1992, table 12.3) within the hybonotum Zone would be difficult.
Based on field surveys across north-central Mexico, Olóriz (Reference Olóriz1992, p. 104–105) interpreted a paleoenvironmental context of shallow to moderate water depths, 30–80 m, and fluctuating barrier effects related to bottom topography, marine currents, and water types to be common during the Late Jurassic in north-central and northeastern Mexico. He related the typical record of unbalanced ammonite assemblages dominated by one or two taxa as revealing moderate-to-strong Platform Effect conditions (Olóriz, Reference Olóriz1986, Reference Olóriz1988, Reference Olóriz1990), which also resulted in variable degrees of endemism during Hybonoticeras and Mazapilites times. Subsequent data based on bed-by-bed sampling support the paleoenvironmental and eco-evolutive scenario that Olóriz (Reference Olóriz1992) interpreted, even for hybonoticeratin ammonites (Olóriz and Villaseñor, Reference Olóriz and Villaseñor1999; Olóriz et al., Reference Olóriz, Villaseñor and González-Arreola2000; Villaseñor et al., Reference Villaseñor, Olóriz, López Palomino and López-Caballero2012).
Olóriz et al. (Reference Olóriz, Lara, De La Mora, Villaseñor and González-Arreola1993) reported and illustrated the first records of Hybonoticeras registered bed-by-bed from Mexico. Hybonoticeras (Hybonoticeras) beckeri harpephorum, Hybonoticeras (Hybonoticeras) beckeri extraspinatum, Hybonoticeras sp. gr. H. beckeri, and Hybonoticeras (Hybonotella) sp. were retrieved from transitional deposits between the La Caja and La Casita formations at the Barranquito del Alacrán section, in the surroundings of Cuencamé, Durango. The hybonoticeratines collected were interpreted as latest Kimmeridgian to earliest Tithonian in age.
Revision of previous works indicates that this was the first attempt to approach a precise identification of the Kimmeridgian-Tithonian boundary based on bed-by-bed sampling of ammonites in Mexico. Olóriz et al. (Reference Olóriz, Lara, De La Mora, Villaseñor and González-Arreola1993) first reported uppermost Kimmeridgian beckeri Zone Hybonoticeras collected bed-by-bed in Mexico, and used a single specimen of Subplanitoides siliceum (Quenstedt, Reference Quenstedt1857) recorded slightly above Hybonoticeras sp. gr. H. harpephorum (= Hybonoticeras sp. of Olóriz et al., Reference Olóriz, Lara, De La Mora, Villaseñor and González-Arreola1993, pl. 2, fig. 1) to interpret the Kimmeridgian-Tithonian boundary. Lacking new contributions refining the Kimmeridgian-Tithonian boundary during the two previous decades (Cecca and Zeiss, Reference Cecca and Zeiss1994), Olóriz et al. (Reference Olóriz, Lara, De La Mora, Villaseñor and González-Arreola1993) interpreted this boundary following the available information and usual stratigraphic interpretations at the time (e.g., Hölder and Ziegler, Reference Hölder and Ziegler1959; Zeiss, Reference Zeiss1968; Ohmert and Zeiss, Reference Ohmert and Zeiss1980). The preliminary knowledge about phenotype characterization and precise ranges of Hybonoticeras in Mexico, plus the limited information provided by associated perisphinctids and haploceratids, determined the use of the single specimen of Subplanitoides siliceum to correlate with the Lithacoceras ulmense Biozone and to interpret the base of the Tithonian (see citations above and compare the revised biozonation scales proposed by Hantzpergue et al., Reference Hantzpergue, Atrops and Enay1991, and Geyssant and Enay, Reference Geyssant and Enay1991). Existing information from Mexico at that time gave no records of H. hybonotum or hybonoticeratines belonging unequivocally to the hybonotum Zone from the section studied at the Barranquito del Alacrán, or elsewhere in the Mexican Altiplano. At the same time, and working on material gathered from southern Germany, Schweigert (Reference Schweigert1993) lent support to an older proposal of a Subplanitoides siliceum Subbiozone at the top of the beckeri Zone (e.g., Roll, Reference Roll1931), although interpretations of S. siliceum as a lower Tithonian species persisted afterward (e.g., Schlegelmilch, Reference Schlegelmilch1994). Subsequently, Schweigert et al. (Reference Schweigert, Krishna, Pandey and Pathak1996) maintained the proposal by Schweigert (Reference Schweigert1993), despite some doubts about the occurrence of S. siliceum homeomorphs or related forms in the uppermost Kimmeridgian (e.g., Geyssant, Reference Geyssant1997; Hantzpergue et al., Reference Hantzpergue, Atrops and Enay1997). More recently, German authors reinforced the proposal of the real occurrence of the siliceum Subzone at the top of the Kimmeridgian (Schweigert, Reference Schweigert1998; Zeiss, Reference Zeiss2001). In the event of confirmation of an uppermost subzone based on S. siliceum-like forms at the top of the Kimmeridgian, and in absence of new data from the same section, the Kimmeridgian-Tithonian boundary interpreted by Olóriz et al. (Reference Olóriz, Lara, De La Mora, Villaseñor and González-Arreola1993) at the Barranquito del Alacrán section could move up. Although data collected from this section thus far do not offer precision in this sense, a new horizon for the Kimmeridgian-Tithonian boundary placed above in this section is feasible according to new information regarding Hybonoticeras collected bed-by-bed in other sections of the Mexican Altiplano (research in progress). Such an interpretation would reinforce the overwhelming dominance of records of uppermost Kimmeridgian hybonoticeratines in the Mexican Altiplano.
Olóriz and Villaseñor (Reference Olóriz and Villaseñor1999) identified five new species of microconchiate Hybonoticeras from sections investigated bed-by-bed in the Mexican Altiplano. As is usual in Mexican sections, limitations related to preservation in siltstones, impoverished ammonite assemblages composed of incomplete specimens, and the lack of records of macroconchiate H. hybonotum and associated hybonoticeratines, impeded the precise interpretation of the Kimmeridgian-Tithonian boundary in the absence of other diagnostic ammonites. Hence, the biostratigraphic ranges of these new species were interpreted to embrace the uppermost Kimmeridgian and the lowermost Tithonian. New data recently obtained from these and other sections in the Mexican Altiplano confirm that at least two of the new species described, H. aff. H. striatulum Berckhemer and Hölder, Reference Berckhemer and Hölder1959 [m] and H. geminatum Olóriz and Villaseñor, Reference Olóriz and Villaseñor1999 [m], seem to be restricted to the uppermost Kimmeridgian, upper beckeri Zone.
Olóriz et al. (Reference Olóriz, Villaseñor and González-Arreola2000) reported the first population-level study of microconchiate hybonoticeratines to compare European populations of Hybonoticeras mundulum (Oppel, Reference Oppel1865) [m] with Mexican ones retrieved from sections sampled bed-by-bed in the Durango and Zacatecas states. The collected material provided valuable information about local-regional intraspecies diversity and the possibility of relating differential phenotype trends to differences in paleonvironmental contexts, as well as a means to approach the paleobiogeographic dynamics underlying those colonization events. At present, no new data have been obtained to reach a more accurate biostratigraphy for these ammonites in Mexico.
Zell et al. (Reference Zell, Beckmann and Stinnesbeck2014) gave the last reported record of macroconchiate Hybonoticeras from Mexico since those illustrated by Olóriz et al. (Reference Olóriz, Lara, De La Mora, Villaseñor and González-Arreola1993). The specimens registered in a complex ‘coquinite’ (shell bed) containing marine invertebrates and vertebrates along with wood at the Sierra del Jabalí, Coahuila, were interpreted as H. sp. gr. H. beckeri of a latest Kimmeridgian age.
Examination of data presented by Zell et al. (Reference Zell, Beckmann and Stinnesbeck2014) and plaster casts of their types indicates unfavorable preservation (see below), as could be expected from interpreted processes forcing the taphonomic condensation noticed by Zell et al. (Reference Zell, Beckmann and Stinnesbeck2014, p. 102). Their interpretation as Hybonoticeras sp. gr. H. beckeri of a latest Kimmeridgian age is supported by the occurrence of looped ribs and chordate keels. Limited knowledge about complete perisphinctids of the same age in Mexico complicate the use of the ammonites illustrated by Zell et al. (Reference Zell, Beckmann and Stinnesbeck2014) to assist in age interpretation of their Hybonoticeras specimens. Plaster casts of specimens CPC 1132, 1133, and 1134, illustrated by Zell et al. (Reference Zell, Beckmann and Stinnesbeck2014, fig. 5.1–5.3), show severe crushing and dominance of very limited preservation, making their precise interpretation difficult. CPC 1132 has inner, distorted whorls with strong ribs and tubercles, some of which have spiny projections, and a ventral region with crowded, slightly oblique undulations bounding a narrow, deep groove (enhanced by severe crushing perpendicular to the plane of coiling?); unfortunately, only the left side of the shell is available for sculptural analysis, and the preserved outer whorl is eroded, showing a possible geminate rib at its adoral extreme. All of the traits recognized show a combination of features known in forms belonging mainly to the species group of H. beckeri, and secondarily to that of H. knopi. CPC 1133 looks similar to the specimen illustrated in situ by Zell et al. (Reference Zell, Beckmann and Stinnesbeck2014, fig. 3.4), showing the opposite left-lateral view. It is poorly preserved and partially covered by sediment but allows one to identify irregular, very strong ribbing and tuberculation, possible evidence of geminate ribs, zigzag connections, and forward projection of ribs on the shoulders with obscure connections to a chordate, barely preserved keel. This specimen could be related to the group of H. ornatum (or H. beckeri ornatum, according to some authors). CPC 1134 is the smallest and best-preserved incomplete specimen. Its exposed left side shows strong sculpture with geminate ribs, and a local adoral projection of ribs on the shoulders connecting to minute, oblique nodations, or crenulations, of a chordate and relatively prominent keel. All of these traits point to the group of H. beckeri. On the whole, analysis of these plaster casts opens the possibility for occurrence of new local, coarsely sculptured forms related to the group of H. beckeri, but better-preserved material is needed to be conclusive. Whatever the case, there is no evidence of lower Tithonian forms among the Hybonoticeras reported by Zell et al. (Reference Zell, Beckmann and Stinnesbeck2014).
The occurrence of Mazapilites in Mexico
According to the revision presented in the Systematic paleontology chapter, the following considerations especially focus on reports of geographic occurrences and stratigraphic interpretations according to authors.
Mazapilites is widely distributed from northwestern Mexico (Cucurpe, northern Sonora) to north-central Mexico (Sierra de Parras, Coahuila; Sierra del Chivo and Cañón del Toboso, eastern Durango; Sierras de Mazapil and Santa Rosa, northern Zacatecas; Sierra de Catorce, San Luís Potosí), northeastern Mexico (eastern Sierra Madre, westward from Ciudad Victoria, Tamaulipas, and east-northeastern Tamaulipas), central Mexico (Cuesta de Huasmazontla, Querétaro), eastern Mexico (Tamanzunchale, southeastern San Luis Potosí; eastern Puebla; Poza Rica, northern Veracruz); and southeastern Mexico (southern Veracruz; Campeche Shelf) (citations below).
Of the six ‘species’‘ included in Mazapilites, five erected by Burckhardt (Reference Burckhardt1906, 1919–Reference Burckhardt1921) and one other originally described as Pulchellia mexicana by Aguilera in Del Castillo and Aguilera (Reference Del Castillo and Aguilera1895), M. tobosensis appears to be the one most frequently identified in Mexican sites. Mazapilites tobosensis has been reported from eastern Durango, southeastern San Luis Potosí and eastern Puebla (Burckhardt, 1919–Reference Burckhardt1921; Imlay, Reference Imlay1939; Peña-Muñoz, Reference Peña-Muñoz1964; Cantú-Chapa, Reference Cantú-Chapa1971, Reference Cantú-Chapa1984), followed by M. symonensis from eastern Durango and northern Zacatecas (Burckhardt, 1919–Reference Burckhardt1921; Imlay, Reference Imlay1939; Peña-Muñoz, Reference Peña-Muñoz1964; Villaseñor, Reference Villaseñor1991) and M. mexicanus from northern San Luis Potosí and northern Sonora (Del Castillo and Aguilera, Reference Del Castillo and Aguilera1895; Verma and Westermann, Reference Verma and Westermann1973; Olóriz et al., Reference Olóriz, Villaseñor, González-Arreola and Westermann1999; Villaseñor et al., Reference Villaseñor, González-León, Lawton and Aberhan2005; this paper). Mazapilites zitteli (see Burckhardt, Reference Burckhardt1906, Reference Burckhardt1930; Imlay, Reference Imlay1937, Reference Imlay1953) is geographically restricted to separate areas, in a moderately large one in northern Zacatecas, Sierra de Parras, Coahuila (Imlay, Reference Imlay1937), and in western Tamaulipas. Burckhardt’s ‘species’ M. carinatus and M. crassicostatus are known only from a small sector in eastern Durango (Burckhardt, 1919–Reference Burckhardt1921). Mazapilites sp. has been reported from across the entire geographic range of the genus in Mexico, including the submerged areas of the Campeche Shelf in southeastern Mexico (Cantú-Chapa and Ortuño-Maldonado, Reference Cantú-Chapa and Ortuño-Maldonado2003).
Mazapilites was originally interpreted as representing the base of the Portlandian, in which Burckhardt (1919–Reference Burckhardt1921, correlation chart between p. 71 and 72) included his “arcillas con Waagenia” recorded at the Mazapil region. Burckhardt (Reference Burckhardt1930, table 10) interpreted the “couches à Waagenia” to be below the “couches à Mazapilites,” but included Waagenia in the lower part of the latter.
Imlay (Reference Imlay1937, p. 603) cited Mazapilites sp. and M. cf. M. zitteli, together with Katroliceras sp., Idoceras? sp., and Belemnopsis sp. from his fossiliferous locality 46 in La Casita deposits at Cañón del Órgano, Sierra de Parras, Coahuila, but no section was provided. This ammonite assemblage characterized his Mazapilites beds (Imlay, Reference Imlay1937, p. 604, table 3). Imlay (Reference Imlay1943, p. 531) interpreted his “faunal divisions of northern Mexico” including a Mazapilites interval characterizing the upper Kimmeridgian sensu Spath (Reference Spath1933); he used Mazapilites just above an interval with Waagenia (= Hybonoticeras), but he did not describe Mazapilites from Placer de Guadalupe, Chihuahua. Imlay (Reference Imlay1953, p. 49) reported Mazapilites from finely bedded sandstones, calcareous marls, and brownish sands belonging to the La Casita Formation, 4 km west of Huizachal, southwestern Tamaulipas. From the same 15 m thick stratigraphic interval, Imlay (Reference Imlay1953, p. 49) mentioned ammonites that today would be interpreted to be late Tithonian, even early Berriasian in age—“Kossmatia, Berriasella, Corongoceras, Micracanthoceras, Mazapilites y Aulacosphinctes.” Imlay (Reference Imlay1953, p. 50) identified Mazapilites and Aspidoceras from reddish phosphoritic limestones, 1.5 m thick, which were interpreted to represent his upper Kimmeridgian. Imlay (Reference Imlay1953, p. 57) identified Mazapilites among other Kimmeridgian ammonites from the middle part of the Taman Formation, recorded as 500 m thick at the Valle del Río Moctezuma, southwestward from Tamazunchale, southeastern San Luis Potosí. In these three cases, no species-level interpretations or precise biostratigraphy were provided by Imlay (Reference Imlay1953). Imlay (Reference Imlay1965) interpreted Mazapilites as an endemic representative of the Pacific Realm (Imlay, Reference Imlay1965, p. 1024, fig. 1) and most probably endemic to the Gulf of Mexico region, although the latter was not clearly stated (Imlay, Reference Imlay1965, p. 1030–1032). Imlay (Reference Imlay1965) assumed a middle to late Kimmeridgian age (sensu Arkell, Reference Arkell1956) for Mazapilites based on his previous interpretations.
Subsequent attempts to interpret the range of Mazapilites offer variable degrees of accuracy, and were rarely based on bed-by-bed sampling. More often, Mazapilites has been reported from horizons above those containing Hybonoticeras, but Olóriz et al. (Reference Olóriz, Villaseñor, González-Arreola and Westermann1999) envisaged the lower boundary of the Mazapilites Biozone to be within the hybonotum Zone (see also Imlay and Jones, Reference Imlay and Jones1970; Imlay, Reference Imlay1980). Based on bed-by-bed sampling in Sierra de Catorce, San Luis Potosí, these authors recorded Mazapilites from stratigraphic horizons that Olóriz et al. (Reference Olóriz, Villaseñor, González-Arreola and Westermann1999) considered to have equivalents within the Virgatosimoceras albertinum-Semiformiceras darwini Biozone. Villaseñor et al. (Reference Villaseñor, González-León, Lawton and Aberhan2005) suggested equivalent horizons for its record in Sonora. Villaseñor et al. (Reference Villaseñor, Olóriz, López Palomino and López-Caballero2012) suggested a biostratigraphically undetermined, imprecise boundary between the biostratigraphic units characterized by Hybonoticeras and Mazapilites in Mexico.
The upper range of Mazapilites has been interpreted at several more or less precise levels within the lower Tithonian. Enay (Reference Enay1962, table 4) assumed the last occurrence of Mazapilites to be clearly above of that of Hybonoticeras, at least within Semiformiceras semiforme-Haploceras verruciferum Biozone horizons, without eliminating uncertainty because elucidating the precise range of Mazapilites was out of focus. Subsequently, based on Burckhardt and Imlay’s works, Enay (Reference Enay1973) interpreted the youngest Mazapilites to be of late albertinum-darwini Chron, if correlation of the sub-Mediterranean Franconites vimineus Biozone with the Mediterranean standard of Zeiss (Reference Zeiss2003) is accepted. Cantú-Chapa (Reference Cantú-Chapa1971) and Verma and Westermann (Reference Verma and Westermann1973) interpreted the uppermost Mazapilites to be upper lower Tithonian (three-fold division). Imlay (Reference Imlay1980, fig. 16) added a question mark to the top of his unit with Mazapilites, which could be correlated with a major part of the albertinum-darwini Zone of the Mediterranean Tethys, except for its lowermost horizons and an indeterminate part of its younger levels.
Callomon (Reference Callomon1992: 267) proposed his M12 faunal horizon with Mazapilites (“beds with Mazapilites”) above the last occurrence of Hybonoticeras in Mexico. He did not suggest stratigraphic continuity between these two biostratigraphic horizons, as was depicted in his table 12.3 without precise comments in text (Callomon, Reference Callomon1992, p. 267). Hence, the lower M12a faunal horizon of M. symonenis proposed by Callomon (Reference Callomon1992) was correlated with a stratigraphic interval embracing the albertinum-darwini Zone except for a lower-middle part (thus, roughly equivalent to the epicontinental vimineus and Danubisphinctes palatinus biozones of the Secondary Standard Biochronostratigraphic Scale for the Mediterranean Province in the Tethyan Realm of Geyssant, Reference Geyssant1997, table 13, and to the vimineus Zone of Zeiss, Reference Zeiss2003, fig. 5). The M12b faunal horizon with M. zitteli was correlated with the semiforme-verruciferum Zone except, perhaps, its lowermost and uppermost parts (thus, roughly equivalent to a lower, corresponding part of the epicontinental Sublithacoceras penicillatum-Virgatosimoceras rothpletzi Biozone of Geyssant, Reference Geyssant1997, p. 100, table 13, and equivalent levels of Zeiss, Reference Zeiss2003, p. 92, fig. 5). Highlighting limitations for precise biostratigraphic interpretations, Callomon (Reference Callomon1992, p. 267) expressed uncertainty about the real stratigraphic order of his faunal horizons M12a and M12b. Given that the older M11 faunal horizon “beds with Waagenia and Mazapilites” of Callomon (Reference Callomon1992, p. 267 = H. autharis M11 in table 12.3) was correlated with the upper-to-uppermost hybonotum Zone, this implies a stratigraphic range for Mazapilites beginning in lower Tithonian horizons below the top of the hybonotum Zone to the top of the semiforme-verruciferum Zone—i.e., levels within the lower-to-mid-lower Tithonian (two-fold division; Olóriz, Reference Olóriz1978; Enay, Reference Enay2009) or within the lower and lower–middle Tithonian (three-fold division; Geyssant, Reference Geyssant1997; Zeiss, Reference Zeiss2003; Villaseñor et al., Reference Villaseñor, Olóriz and González-Arreola2000, Reference Villaseñor, Olóriz, López Palomino and López-Caballero2012).
Cantú-Chapa (Reference Cantú-Chapa2001, p. 9) suggested a Mazapilites event in Mexico that characterized the top of the lower Tithonian (two-fold division), thus indicating the youngest age assumed for the last Mazapilites species and, therefore, levels younger than previously considered by Cantú-Chapa (Reference Cantú-Chapa1971). Recent data obtained by the authors at Cañón de San Matias indicate the occurrence of Mazapilites in horizons equivalents to the M10 faunal horizon of Callomon (Reference Callomon1992, p. 267, table 12.3), which ranges from stratigraphic horizons close to the Kimmeridgian-Tithonian boundary to a large part of the hybonotum Zone, according to the correlation proposed by Callomon (Reference Callomon1992).
At present, it can be established that there are no conclusive data to interpret the precise biostratigraphic range of Mazapilites in Mexico. On the whole, Mazapilites biostratigraphy is poorly known due to information derived from very scarce, imprecise data provided by bed-by-bed sampling, together with geographically separate records without associated ammonites of interest for high-resolution biostratigraphy. Hence, new data collected bed-by-bed are needed to establish the precise stratigraphic range of Mazapilites in Mexico. Of special interest is the reinterpreted biostratigraphy that resulted from the previous revision of Burckhardt’s Waagenia (= Hybonoticeras), because it opens the possibility of a latest Kimmeridgian age for the oldest Mazapilites in Mexico.
The co-occurrence of Hybonoticeras and Mazapilitesin Mexico
The analyses just presented for reinterpreting the reported and existing material of Mexican Hybonoticeras and Mazapilites have shown how distinct, and limited, the present knowledge is in terms of precise biostratigraphy and correlation potential, as well as the differential degree of uncertainty that applies to their taxonomic interpretation at the species level. According to the revision facilitated above, the co-occurrence of Waagenia (= Hybonoticeras) and Mazapilites in Mexico was based on a single imprint of Waagenia sp., interpreted by Burckhardt (1919–Reference Burckhardt1921) as an indication of the base of his Portlandian (= lowermost Tithonian), without giving precise stratigraphy supporting his interpretation. Hence, the real co-occurrence of Waagenia and Mazapilites—i.e., collected from the same horizon—is doubtful if strictly based on the information provided by Burckhardt (1919–Reference Burckhardt1921). Supporting this evaluation is the fact that all new species of Mazapilites erected by Burckhardt (Reference Burckhardt1919 in 1919–1921, p. 4–11) were collected from his “capas superiores con Mazapilites,” and no single Mazapilites identified at the species level was reported from the “capas inferiores con Mazapilites,” in which Waagenia sp. was collected as a single imprint without additional stratigraphic detail. Thus, reference to material gathered from the “capas inferiores con Mazapilites” could indicate poor preservation, poor stratigraphy, loose specimens, or a combination of all factors.
In contrast to the inconclusive information provided by Burckhardt (1919–Reference Burckhardt1921) about the co-occurrence of Waagenia and Mazapilites, Burckhardt (Reference Burckhardt1930) gave comprehensive data both about records of Waagenia without Mazapilites, and vice versa. The alluded information agrees with: (1) his scheme of stratigraphic correlation for the Upper Jurassic in Mexico and southeastern France (Burckhardt, Reference Burckhardt1930, p. 64, table 5), in which he correlated the interval with Waagenia with the “Zone à beckeri,” and that corresponding to Mazapilites with the “Zone à Oppelia lithographica (couches de Solhofen)”; (2) the biostratigraphic synthesis for the Upper Jurassic in central and northern Mexico (Burckhardt, Reference Burckhardt1930, table 6); and (3) key fauna in his “faciès à Céphalopodes preponderant” in the synthesis about the main facies of the Mexican Jurassic (Burckhardt, Reference Burckhardt1930, table 9). On this basis, a reasonable assumption is that Burckhardt (1919–Reference Burckhardt1921, Reference Burckhardt1930) envisaged, rather than demonstrated, the co-occurrence of Waagenia (= Hybonoticeras) and Mazapilites, which he supported with very limited and at present questionable data (see above comments about the data of Burckhardt, 1919–Reference Burckhardt1921).
Imlay (Reference Imlay1937, p. 604, table 3) clearly interpreted the record of Waagenia (Waagenia beds) below that of Mazapilites (Mazapilites beds) in Sierra de Parras, Coahuila, northeastern Mexico, but later (Imlay, Reference Imlay1939) added unclear information in evaluating the co-occurrence of Waagenia (= Hybonoticeras) and Mazapilites in Mexico. In fact, the difference between his text and the corresponding stratigraphic column and chart with the distribution of ammonites (Imlay, Reference Imlay1939, p. 10, 22, fig. 4, table 2) is clearly identifiable and, therefore, his contribution could include uncertainty. The relevance of this report of the co-occurrence of Waagenia (= Hybonoticeras) and Mazapilites in Mexico is that the stratigraphic interpretation made by Imlay (Reference Imlay1939) for records considered below the eudoxus-steraspis Zone (see above) refers rather to the present upper Kimmeridgian beckeri Zone, which could indicate the occurrence of Mazapilites in the uppermost Kimmeridgian, thus dating its co-occurrence with Hybonoticeras of latest Kimmeridgian age.
Comparison of three correlation charts provided by Imlay (Reference Imlay1965, fig. 6a; 1980, p. 32, fig. 21; 1984, p. 10, fig. 6) shows inconclusive information and changing interpretation on the co-occurrence of Waagenia (= Hybonoticeras) and Mazapilites in Mexico—in the first case, showing co-occurrence (Imlay, Reference Imlay1965, fig. 6a); then showing separate horizons (Imlay, Reference Imlay1980, p. 32) to support co-occurrence, based on data without illustrations by Imlay (Reference Imlay1939, p. 10, 11), while showing co-occurrence with doubts (Imlay, Reference Imlay1980, fig. 21); and finally showing Hybonoticeras below Mazapilites with an allusion to different ages (early Tithonian and late early Tithonian, respectively), and without any mention of co-occurrence (Imlay, Reference Imlay1984, p. 10, fig. 6).
Attempts by other authors interpreting a potential co-occurrence of Hybonoticeras and Mazapilites in Mexico, without analyzing new data collected bed-by-bed, merely assumed Burckhardt’s and/or Imlay’s interpretations, which did not provide supporting illustrations. Thus, based on data by Arkell (Reference Arkell1956), Enay (Reference Enay1962, p. 364, 365, table 4) interpreted the upper and wider stratigraphic interval of Hybonoticeras in Mexico to include a combined record of Hybonoticeras and Mazapilites, characterizing the older early Tithonian. Enay (Reference Enay1962, p. 365, table 4) assumed that “l’apparition du genre particulier Mazapilites (couches à Hybonoticeras et Mazapilites) marquerait, au Mexique, le dèbut du Tithonique,” and gave precise indication of the European fauna used for correlation (Enay, Reference Enay1962, table 4). Cantú-Chapa (Reference Cantú-Chapa1963, p. 16) assumed that “Mazapilites (Oppelidae caractéristique du Mexique) est très remarquable dans cette partie du Kimméridgien supérieur (ou Portlandien inférieur?) comprise entre la Zone à beckeri (limite inf.) et le Tithonique (limite sup.).” Enay (Reference Enay1963, p. 4) promoted his previous hypothesis of “au Mexique, l’assemblage Hybonoticeras-Mazapilites caractériserait la base du Tithonique.” Callomon (Reference Callomon1992, p. 265, 267, table 12.3) approached the interpretation of ammonite horizons in Mexico as a discontinuous succession that he tentatively correlated with the European standard biochronostratigraphic scales; unfortunately, Callomon did not provide precise biostratigraphic comments for all of the ‘faunal horizons’ to support their correlation depicted in his table 12.3. His M11 faunal horizon, labeled H. autharis in a table (see Callomon, Reference Callomon1992, table 12.3), and “beds with Waagenia and Mazapilites” at Toboso, Sierra de Symón in text (Callomon, Reference Callomon1992, p. 267), assumed the co-occurrence of Waagenia (= Hybonoticeras) and Mazapilites. Accordingly, a middle hybonotum Chron age would correspond to the oldest record of Mazapilites in Mexico (Callomon, Reference Callomon1992, table 12.3).
In contrast to the inconclusiveness of previous information, and the absence of the corresponding illustrations, a real case of co-occurrence of Hybonoticeras and Mazapilites in Mexico has been shown for the first time by recent bed-by-bed sampling in Sierra de Catorce, San Luis Potosí (AL-5 section; this paper). This co-occurrence is illustrated with ammonite records from horizons that can be correlated with a much lower, but not the lowermost, part of the hybonotum Zone in the secondary biochronostratigraphic scale for the Tithonian in Mediterranean and sub-Mediterranean Europe (e.g., Geyssant, Reference Geyssant1997). At present, the new information provided in this paper is limited and must be complemented with data from other sections investigated bed-by-bed in Mexico.
The occurrence of Mazapilites in Cuba
Mazapilites has long been considered endemic to Mexico (e.g., Burckhardt, 1919–Reference Burckhardt1921, p. 2, 65; Reference Burckhardt1930, p. 109; Cantú-Chapa, Reference Cantú-Chapa1963, p. 16, Reference Cantú-Chapa1979, p. 21; Enay, Reference Enay1973, p. 300), typical among Upper Jurassic ammonite genera from the Gulf of Mexico region, and representative of the Pacific Realm (Imlay, Reference Imlay1965, p. 1030). Mazapilites was even considered to be a South American taxon by Berckhemer and Hölder (Reference Berckhemer and Hölder1959, p. 73), although Cantú-Chapa (Reference Cantú-Chapa2001, p. 9) cited Berckhemer and Hölder (Reference Berckhemer and Hölder1959) in envisaging the occurrence of Mazapilites in Germany as the single one identified outside from Mexico.
Houša and De la Nuez (Reference Houša and De la Nuez1972, Reference Houša and De la Nuez1973) first proposed a Mazapilites Zone for western Cuba, and Houša and De la Nuez (Reference Houša and De la Nuez1975, p. 57) characterized the oldest Tithonian horizon with “Mazapilites, Protancyloceras, Pseudolissoceras, Physodoceras etc.,” interpreting its correlation “approximately at the level of the Mexican upper Kimmeridgian.” Records of Mazapilites were subsequently reported and illustrated from Sierra de los Órganos in western Cuba by Myczyński (Reference Myczyński1989, p. 53, 92), who interpreted the occurrence of M. zitteli (see Myczyński, Reference Myczyński1989, pl. 2, figs. 1–3, 5). This author reported Mazapilites sp., Protancyloceras aff. P. gracile (Oppel, Reference Oppel1865), Neochetoceras sp., and Lithacoceras (?), among other ammonites with minor relevance for biostratigraphy, from an interval 2.5–3 m thick in the lower part of the El Americano Member. This ammonite assemblage would most likely indicate lowermost horizons within the Tithonian. Myczyński (Reference Myczyński1989, p. 93) reported M. zitteli and M. sp. from the lower part of the El Americano Member in sections B and C of the Hacienda el Americano. Unfavorable preservation complicates the interpretation of M. sp. (Myczyński, Reference Myczyński1989, pl. 2, fig. 4, pl. 5, fig. 7) recovered from the lower part of the El Americano Member. Myczyński (Reference Myczyński1989, p. 56) reported M. zitteli with Pseudolissoceras zitteli (Burckhardt, Reference Burckhardt1903), among other ammonites, from a 6 m thick stratigraphic interval in section C at Hacienda el Americano, mentioning the possibility of their provenance from slightly different horizons (Myczyński, Reference Myczyński1989, fig. 6). The latter can be confirmed by the record of P. zitteli as being identified “slightly above the Mazapilites Zone” (Myczyński, Reference Myczyński1989, p. 85). Limited preservation impedes analysis of the illustrations of P. zitteli provided by Myczyński, except for the specimen collected at the Loma Ferretero section, LF, in Sierra del Rosario (Myczyński, Reference Myczyński1989, pl. 10, fig. 4b). Myczyński (Reference Myczyński1989, p. 67, footnote 2) indicated that “the genus Mazapilites in Cuba seems to represent a zone younger than the Mexican Hybonoticeras Biozone, as it is concurrent in Cuba not with Hybonoticeras, but with Protancyloceras, whence Pseudolissoceras is present too.” Pszczołkowski and Myczyński (2003, and references therein) reported, without illustration, Mazapilites spp. from Guaniguanico sections in western Cuba, and M. symonensis “?” from the Camajuaní Belt in central Cuba.
Despite being of indubitable value, Cuban data for Mazapilites cannot support precise biostratigraphic interpretation and correlation, as shown below.
Summary remarks on the co-occurrence of Hybonoticeras and Mazapilites in the Mexico-Caribbean area
Co-occurrence of Hybonoticeras and Mazapilites has been proposed for both Mexico and Cuba, and their confirmation would be of interest for interpreting the underlying paleobiogeographic dynamics if precise biostratigraphy were available.
Burckhardt (1919–Reference Burckhardt1921) reported the co-occurrence of Waagenia (= Hybonoticeras) and Mazapilites within the lower range of the latter (“capas inferiores con Mazapilites”) in the Mexican Altiplano. However, the absence of detailed sections with reference to particular beds and the precise thickness at which these genera were recorded makes any approach for obtaining biostratigraphic details inconclusive according to the demands of present-day biostratigraphy. Burckhardt (1919–Reference Burckhardt1921, p. 64) considered a 20 m thick section for the complete stratigraphic interval with Mazapilites: 17 m were poor in fossils, and the remaining 3 m would contain the “capas inferiores con Mazapilites” at the bottom, and the “capas superiores con Mazapilites” at the top, but without specifying the respective thicknesses of these ‘beds.’
Burckhardt (Reference Burckhardt1930, p. 45–165) provided summarized data and conclusions from his 25 years of research on the Upper Jurassic in Mexico. This author clearly placed his “argiles à Waagenia” below the “couches à Mazapilites,” but supported co-occurrence of these genera in the Sierra de Santa Rosa, Mazapil, Zacatecas (Burckhardt, Reference Burckhardt1930, p. 68, table 6), and in the Sierras de Symón and Ramirez, Zacatecas-Durango (Burckhardt, Reference Burckhardt1930, p. 56, fig. 17, table 6). The former case refers to the “couches à Mazapilites ... probablement représentées par la partie supérieur des ‘argiles à Waagenia’” (Burckhardt, Reference Burckhardt1930, p. 68). The second evokes two imprints obtained in 1919—that of W. sp. indet. (“cfr. W. autharis Oppel sp.”) collected from the mentioned “capas inferiores con Mazapilites” at the Cañón del Toboso (Burckhardt, 1919–Reference Burckhardt1921, p. 64; see above), and reported as “Waagenia cfr. autharis” by Burckhardt (Reference Burckhardt1930, p. 69), and another isolated record found at the Pico de Teyra (Burckhardt, Reference Burckhardt1930, p. 69) and interpreted to come from the same “capas inferiores con Mazapilites” without reference to precise section and biostratigraphy.
Imlay (Reference Imlay1937, table 3; Reference Imlay1939, table 2) clearly identified two consecutive stratigraphic intervals, a lower one with Waagenia (= Hybonoticeras) and an upper one with Mazapilites. Imlay (Reference Imlay1939) referred to the latter as a common stratigraphic interval in the upper Kimmeridgian that he correlated with the lower part of the eudoxus-steraspis Zone of Spath (Reference Spath1933, as cited by Imlay, Reference Imlay1939, table 1). From the northern side of the Cañón del Toboso, Sierra del Chivo, Imlay (Reference Imlay1939, p. 10, 22, table 6) reported the co-occurrence of W. sp. (then Waagenia, and finally W. cf. W. autharis), Mazapilites sp., and Torquatisphinctes sp. from gray shales (coll. 18 of Imlay, Reference Imlay1939), although he indicated a separate lower horizon for W. sp. in the corresponding stratigraphic column (Imlay, Reference Imlay1939, fig. 4). As was the case for previous reports of co-occurring Waagenia (= Hybonoticeras) and Mazapilites in Mexico, this author did not provide illustrations of the specimens of Waagenia and Mazapilites recorded at the Cañón del Toboso section.
Imlay (Reference Imlay1943, p. 531) interpreted his “faunal divisions of northern Mexico” with a Waagenia interval below the Mazapilites interval (see above).
Imlay (Reference Imlay1965, table 6a) assumed a limited overlap of Hybonoticeras and Mazapilites with no additional comments, because his text was focused on faunal differentiation and potential forcing factors. Mazapilites and other Upper Jurassic genera from Mexico and Cuba were suggested as representatives of the Pacific Realm that he accepted following Arkell (Reference Arkell1956) and not related to latitude (Imlay, Reference Imlay1965, p. 1024).
Imlay and Jones (Reference Imlay and Jones1970, fig. 2) used a correlation chart showing a small stratigraphic interval with Hybonoticeras and Mazapilites, but they did not comment on these genera.
Enay (Reference Enay1973, table 1) envisaged a limited overlap of the ranges of Mazapilites and Hybonoticeras based on the contributions by Burckhardt and Imlay, without specification (Enay, Reference Enay1973, p. 306, noting the omission of publications cited by Arkell, Reference Arkell1956), but he did not consider the occurrence of Mazapilites outside of Mexico.
Imlay (Reference Imlay1980, p. 32) alluded to his previous data (Imlay, Reference Imlay1939, p. 10, 22) on the co-occurrence of Waagenia (= Hybonoticeras) and Mazapilites in the Cañón del Toboso, Sierra del Chivo, Durango. Imlay (Reference Imlay1980, figs. 16, 21) assumed the possibility of the youngest records of Hybonoticeras overlapping the earliest ones of Mazapilites and the so-called Virgatosphinctes and Subdichotomoceras Spath, Reference Spath1925 in Mexico, as well as the earliest record of Mazapilites with V. aguilari (Burckhardt, Reference Burckhardt1906) in the Gulf of Mexico region and surrounding areas. Imlay (Reference Imlay1984, p. 10, fig. 6) interpreted the record of Hybonoticeras in northern and eastern Mexico to be of latest Kimmeridgian to earliest Tithonian in age, and to occur below Mazapilites of late-early Tithonian age, without mention of the potential co-occurrence that he had previously assumed.
Houša and De la Nuez (Reference Houša and De la Nuez1972, Reference Houša and De la Nuez1973) first characterized a stratigraphic interval with Mazapilites in Cuba (the oldest Tithonian ammonite horizon of Houša and De la Nuez, Reference Houša and De la Nuez1975). Subsequently, Myczyński (Reference Myczyński1989, p. 67, footnote 2), without records of Hybonoticeras in Cuba, envisaged the records of Cuban Mazapilites to be younger than those of Hybonoticeras in Mexico. Pszczółkowski and Myczyński (Reference Pszczółkowski and Myczyński2010, fig. 18) reported the co-occurrence of H. spp. and M. zitteli throughout the lower part of the range of the latter in Cuba. Crucial information came from earlier research: Myzcyński (Reference Myczyński1998; also mentioned by Pszczółkowski and Myczyński, Reference Pszczółkowski and Myczyński2010, p. 232) first reported Hybonoticeras from Cuba. Myzcyński (Reference Myczyński1998, p. 114) mentioned two specimens of H. (Hybonoticeras) sp. gr. H. beckeri ~1.60 m above Mazapilites from the El Americano Member of the Guasasa Formation at the lowermost Hacienda El Americano section in Sierra de los Órganos, as well as one specimen of H. sp. ~120 cm in diameter, and several specimens of H. (Hybonotella) cf. H. mundulum striatulum, from the La Zarza Member of the Artemisa Formation at the La Catalina section in western Sierra del Rosario. Myczyński (Reference Myczyński1999, p. 103–106) described and illustrated macro- and microconchiate Hybonoticeras from Cuba (most probably those reported by Myczyński, Reference Myczyński1998), and proposed a lowermost Tithonian Hybonoticeras-Mazapilites Zone for Sierra de los Órganos, whereas the lower boundary of the Hybonoticeras (Hybonotella) Biozone in Sierra del Rosario was considered uncertain. Myzcyński (Reference Myczyński1999, fig. 5.1, 5.2) described and illustrated H. (Hybonoticeras) sp. gr. H. beckeri from El Americano section at Sierra de los Órganos, and H. (Hybonotella) cf. H. mundulum striatulum from La Catalina section in Sierra del Rosario. Poor preservation limited their interpretation at the species and subspecies level, respectively (Myczyński, Reference Myczyński1999, p. 103, 105). The specimen of H. (Hybonoticeras) sp. gr. H. beckeri reported from the El Americano section is difficult to interpret from its illustration. According to the description provided by Myczyński (Reference Myczyński1999, p. 103, 105), showing dominant simple ribs at ~80 mm shell diameter, this specimen corresponds to Hybonoticeras harpephorum or to a local related form, the age of which could be latest Kimmeridgian rather than earliest Tithonian, but preservation impedes a conclusive interpretation. In the absence of clear records of upper Kimmeridgian or lower Tithonian macroconchiate hybonoceratines (see below), age uncertainty could be unsolvable in this case. Given that this specimen was collected 1.6 m above Mazapilites (Myczyński, Reference Myczyński1999, p. 96), confirmation of a sound age reinterpretation is relevant for reinterpreting the older biostratigraphic range of Mazapilites in the area, and the lowermost Tithonian horizon recorded in Cuba. In fact, Myczyński (Reference Myczyński1999) correctly used age interpretations accepted at the time to reveal uncertainty about discrimination of an upper Kimmeridgian from a lowermost Tithonian interval based exclusively on the Hybonoticeras specimens that he described. Therefore, he used the occurrence of Mazapilites to interpret a lower Tithonian age (e.g., Myczyński, Reference Myczyński1989) for his H. (Hybonoticeras) sp. gr. H. beckeri from El Americano section, while correctly interpreting a Late Kimmeridgian to earliest Tithonian age for the known record of H. (Hybonotella) cf. H. mundulum striatulum from La Catalina section (Myczyński, Reference Myczyński1999, p. 96, 105). Hence, lowermost hybonotum Zone horizons were envisaged but not demonstrated by the record of clear hybonotum Zone hybonoticeratines and/or other ammonites with potential, reliable biostratigraphic correlation outside Cuba.
Pszczółkowski and Myczyński (Reference Pszczółkowski and Myczyński2010, p. 232) reported macro- and microconchiate Hybonoticeras from the southern Rosario succession: H. (Hybonoticeras) sp. and H. (Hybonotella) cf. H. mundulum striatulum from the La Catalina section, without illustration (probably referring to the specimen described and illustrated by Myczyński, Reference Myczyński1999, figs. 5.2, 6). Pszczółkowski and Myczyński (Reference Pszczółkowski and Myczyński2010, p. 232) also mentioned H. (Hybonotella) gr. mundulum striatulum, and H. gr. mundula striatula (Pszczółkowski and Myczyński, Reference Pszczółkowski and Myczyński2010, p. 241, fig. 17), which was identified from partly recrystallized remains, 5–6 mm in size, in microfacies from the Loma Redonda section (Pszczółkowski and Myczyński, Reference Pszczółkowski and Myczyński2010, fig. 17.1). Pszczółkowski and Myczyński (Reference Pszczółkowski and Myczyński2010, p. 238, 239, fig. 2) confirmed the Hybonoticeras-Mazapilites Zone for western Cuba, the bottom of which coincided with the drowning of carbonate shelves and could be correlated with a relevant occurrence of mazapilitines on inner shelves (Pszczółkowski and Myczyński, Reference Pszczółkowski and Myczyński2010, fig. 21).
Unfortunately, illustrations of Cuban Hybonoticeras and Mazapilites are too limited for an in-depth evaluation of their precise biostratigraphic significance.
In the context of this revision, the oldest records of Mazapilites could belong to the uppermost Kimmeridgian in the Mexico-Caribbean area according to revised data by Burckhardt (1919–Reference Burckhardt1921), Imlay (Reference Imlay1939), and Myczyński (Reference Myczyński1999), and, therefore, the stratigraphic range of its co-occurrence with Hybonoticeras would embrace, at least, stratigraphic horizons from the uppermost Kimmeridgian, upper beckeri Zone (e.g., Imlay, Reference Imlay1939, without illustration) to the lower Tithonian, lower hybonotum Zone (this paper, with illustration). New data collected bed-by-bed are needed to establish the precise stratigraphic location of the upper range of Mazapilites.
Conclusions
A thorough bibliographic revision has revealed how present-day biohorizon biostratigraphy clashes with the stratigraphic accuracy that characterizes relevant pioneer works. Differences in sampling strategy, scientific authority, and other factors have conditioned interpretations over time, making precise biostratigraphic control, interpretation, and correlation difficult tasks today. New data obtained from sampling bed-by-bed have led to revision of some keystones of Upper Jurassic biostratigraphy in the Mexico-Caribbean area, in this case paying special attention to improving the approach to the Kimmeridgian-Tithonian boundary.
The hybonotum Zone pro parte is clearly identified in Mexico for the first time based on hybonoticeratin ammonites, with illustration of macroconchiate Hybonoticeras collected bed-by-bed from Sierra de Catorce, San Luis Potosí.
Hybonoticeras authariformis n. sp. [M] designates the oldest Tithonian horizon identified in Mexico. Macroconchiate Hybonoticeras species interpreted as indicating the basal Tithonian in epicontinental Europe are recorded from the same horizon containing H. authariformis n. sp. [M] in the section investigated, in the surroundings of Alamitos de los Díaz, San Luis Potosí, México, and below horizons with forms closer to H. hybonotum.
Reported records of Hybonoticeras from Mexico are revised, with special attention paid to macroconchiate specimens. Upper beckeri Zone hybonoticeratines are well itemized, whereas the record of unequivocal hybonotum Zone hybonoticeratines has remains elusive.
Phenotype deviation and cases of endemism identified among Mexican hybonoticeratines open new ways to interpret Hybonoticeras as a well-known colonizer of epicontinental shelves during the highest sea levels in Jurassic times. The paleoenvironmental reconstruction proposed will assist future research, which must be focused on population levels and the meaning of endemism, and which must be based on data recovered bed-by-bed elsewhere, before proposing a conclusive interpretation about the records of hybonoticeratines from distant areas.
This revision of the existing material of Mazapilites reveals limitations for an updated interpretation of the nominal species included in this genus. Uncertainty persists about intraspecies diversity and the potential relationships among the existing nominal species described from Mexico, and no precise stratigraphy is available at this level. New material collected bed-by-bed, featuring the preservation of adult body chambers, is necessary to put forth a more conclusive interpretation of this genus.
In contrast to common interpretations, the first occurrence of Mazapilites in the Mexico-Caribbean area could belong to the uppermost Kimmeridgian, upper beckeri Zone horizons, but new material based on bed-by-bed sampling is needed to be conclusive. At present, no conclusive data exist on the last occurrence of Mazapilites.
The co-occurrence of Hybonoticeras and Mazapilites in Mexico is illustrated for the first time by material gathered from horizons belonging to the lower Tithonian, lower but not lowermost hybonotum Zone, slightly above the oldest Tithonian horizon identified by the record of macroconchiate Hybonoticeras. Previous interpretations of this co-occurrence were not demonstrated but rather envisaged from records reported without precise stratigraphy nor illustration, except for a single report without illustration gathered from interpreted late Kimmeridgian deposits in Mexico (Imlay, Reference Imlay1939). On this basis, the co-occurrence of Hybonoticeras and Mazapilites in Mexico would embrace, at least, uppermost Kimmeridgian (upper beckeri Zone) and lower Tithonian (lower hybonotum Zone) horizons.
Existing information about hybonoticeratines and mazapilitines from Cuba is limited and cannot be conclusively interpreted, and their co-occurrence cannot be demonstrated. However, the revision of Myczyński (Reference Myczyński1999) suggests the potential occurrence of Mazapilites in upper Kimmeridgian horizons in Cuba, paralleling that reported from Mexico, and reinforces the possibility of combined occurrence with Hybonoticeras in the future.
At present, evaluation of the oldest co-occurrence of Hybonoticeras and Mazapilites in Mexico-Caribbean areas remains an open question in terms of precise biohorizon biostratigraphy. The results obtained here improve upon previous knowledge on the records of Hybonoticeras and Mazapilites in Mexico-Caribbean areas, providing biostratigraphic precision in the interpreted range of macroconchiate Hybonoticeras, and favoring precision in long-distance correlations.
Acknowledgments
This contribution benefited from comments and suggestions made by the editors B. Hunda, H.D. Sues, M. Yacobucci, and J. Kastigar, and the detailed revisions conducted by M. Boughdiri (University of Carthage), C. Sarti (University of Bologna), V. Venari (University of Buenos Aires), and two more anonymous reviewers. We are indebted to V. Romero Mayen for her assistance at the IGM. Our thanks to I. López-Caballero and M.A. Argáez for their help and facilities during field surveys and sample preparation, and A. Altamira for photographs of the material analyzed. J. Sanders is acknowledged for her linguistic assistance. G. Schweigert (Staatliches Museum für Naturkunde Stuttgart) is acknowledged for information about type material housed in German institutions. This research was supported by the UNAM [Project PAPIIT IN105311-3 and IN102016] and the Ministerio de Industria Economía y Competitividad (MINECO), Spain [Project CGL2012-39835].