Introduction
Weeds can reduce ornamental plant growth in nursery production and landscapes by competing for nutrients, light, and water. Many researchers have documented the detrimental effects of even one weed on container-grown ornamentals (Walker and Williams Reference Walker and Williams1989) such as eclipta [Eclipta prostrata (L.) L.], which reduced the growth of ‘Fashion’ azalea (Rhododendron × ‘Fashion’) by 43% (Berchielli-Robertson et al. Reference Berchielli-Robertson, Gilliam and Fare1990). The most common method of weed control in containerized ornamental production is hand weeding and application of PRE herbicides. Hand weeding is labor intensive and may cost growers $1,200 to $4,000 ha–1 annually (Case et al. Reference Case, Mathers and Sensesac2005; Mathers Reference Mathers2003). An additional cost is the use of PRE herbicides, which must be applied four to six times per year or more for adequate control. On an average, a 22.6-kg bag of formulated granular PRE herbicide costs approximately $80 in central Florida, and most are applied at rates of approximately 224 kg ha–1, resulting in a total chemical cost of ~$800 ha–1 per application. Moreover, when broadcasting granular herbicides, up to 80% nontarget loss may be observed, as the herbicide granules are often deposited in between containers (Gilliam et al. Reference Gilliam, Fare and Beasley1992). This nontarget loss increases costs and may result in increasing herbicide concentrations in irrigation water for nurseries that reuse and recycle water (Pereira and Hostettler Reference Pereira and Hostettler1993; Samtani et al. Reference Samtani, Kling, Mathers and Case2007).
In landscape planting beds, weeds are most often controlled through use of various mulch materials and spot applications of nonselective herbicides such as glyphosate or other herbicides (Marble et al. Reference Marble, Koeser and Hasing2015a, Reference Marble, Koeser and Hasing2015b). However, use of glyphosate can result in death or severe injury to ornamentals if accidental contact occurs. The use of glyphosate in public areas has also come under scrutiny recently, and several municipalities in Florida are considering bans in publicly managed landscape areas. The lack of weed management options often results in the need for hand weeding, but most landscape maintenance companies do not have weeding crews available or offer this as a routine service.
To address these challenges, use of organic mulch materials has been thoroughly investigated as a weed management tool (Chalker-Scott Reference Chalker-Scott2007). Mechanisms responsible for weed control vary depending upon the weed species, but light exclusion (Ngouajio and Ernest Reference Ngouajio and Ernest2004), creation of a physical barrier (Teasdale and Mohler Reference Teasdale and Mohler2000), and reducing available moisture on the mulch surface (Altland et al. Reference Altland, Boldt and Krause2016) are all responsible for weed suppression in different scenarios (Chalker-Scott Reference Chalker-Scott2007). The ability of a mulch to suppress weed emergence will be directly influenced by seed position, that is, the location of weed seed in relation to the mulch layer. For example, light exclusion as a mechanism of control will be most effective on seeds positioned beneath the mulch if seeds are positively photoblastic (Ngouajio and Ernest Reference Ngouajio and Ernest2004). Similarly, when the primary mechanism of control is as a physical barrier, mulches will be more effective on seed beneath the mulch layer (Richardson et al. Reference Richardson, Gilliam, Fain and Wehtje2008). Although mulch typically increases moisture retention in the soil (Chalker-Scott Reference Chalker-Scott2007), many commonly used mulch materials retain very little moisture (Bartley et al. Reference Bartley, Wehtje, Murphy, Foshee and Gilliam2017) and thus, limit weed emergence by reducing available moisture on the mulch surface as weed seeds are deposited on top. In studies with rice hull mulch, Altland et al. (Reference Altland, Boldt and Krause2016) reported greater flexuous bittercress (Cardamine flexuosa With.) control when seeds were placed on top of rice hull mulch compared with seeds placed beneath the mulch on the soil surface. As rice hulls were determined to be very hydrophobic and held very little moisture within the mulch layer, greater control was attributed to the ability of rice hulls to limit available water to germinating seedlings when seeds were placed on the mulch surface. In contrast, flexuous bittercress seeds placed beneath the mulch layer on the soil surface had ample moisture and emerged when rice hulls were applied at shallower depths (<1.3 cm).
Regardless of the mechanism of control, mulch is usually more efficacious as depth increases. Greater mulch depths will reduce more light, create a greater physical barrier, and when acting to reduce available moisture, a greater depth reduces the chance that germinating seedlings on the mulch surface survive as moisture is limited (Teasdale and Mohler Reference Teasdale and Mohler2000). Most studies evaluating use of organic mulch materials for weed control focus on mulch type (Billeuad and Zajicek 1989; Broschat Reference Broschat2007) and different depths (Richardson et al. Reference Richardson, Gilliam, Fain and Wehtje2008; Wilen et al. Reference Wilen, Schuch and Elmore1999). However, regardless of depth, the physical properties of the material will undoubtedly play a critical role in weed control efficacy. For example, pine bark (PB) processed through a screen size large enough to be considered mulch (typically >2.5 cm) provides over 90% control of many common nursery weed species (Cochran et al. Reference Cochran, Gilliam, Eakes, Wehtje, Knight and Olive2009). In contrast, PB processed to be used as potting media (particle size often 1 to 1.3 cm) has no detrimental influence on weed growth, as evidenced by the challenging nature of weed control in container-grown ornamentals (Case et al. Reference Case, Mathers and Sensesac2005). In most cases, authors do not report the physical properties of the mulch materials they evaluated or examine possible mechanisms of control, but exceptions can be found. Bartley et al. (Reference Bartley, Wehtje, Murphy, Foshee and Gilliam2017) evaluated five different tree-derived mulch materials and reported particle size distribution for all materials. Although all mulches evaluated provided acceptable control, when all materials were applied at a 2.5-cm depth, PB provided greater spotted spurge (Euphorbia maculata L.) control compared with other mulches that contained a higher percentage of small particles (0.6 cm). Mulch particle size will invariably influence light transmittance and water-holding capacity, and coarser mulch particles, regardless of the source material for the mulch (e.g., bark, wood, straw), typically provide superior weed control (Chalker-Scott Reference Chalker-Scott2007).
A better understanding of the underlying mechanisms of control provided by common mulch materials based on physical characteristics would be advantageous for practitioners and could aid in mulch selection for different uses. Information on light exclusion ability, particle size, and water-holding capacity and how these variables contribute toward weed control is lacking for common mulch materials utilized in the landscape and nursery industries. The objective of this research was to determine how physical properties and different depths of hardwood (HW), PB, and pine straw (PS) mulch influence emergence of garden spurge and large crabgrass based on seed position (on top of or below mulch). Particle size analysis, moisture retention, and light transmittance through each mulch, and how these different variables influenced weed emergence were also investigated.
Materials and Methods
Outdoor Container Experiments
Research was conducted at the Mid-Florida Research and Education Center (28.64°N, 81.55°W) in Apopka, FL, in the summer of 2017 and repeated in the fall of 2017. Round black plastic nursery containers (28 cm diam by 23 cm height) were filled with a PB–peat mix (Fafard® 52 growing mix; SunGro Horticulture, Agawam, MA) and amended with 6 kg m–3 controlled-release fertilizer 15N-3.9P-9.9K (Osmocote® Plus; Everris, Geldermalsen, the Netherlands) based on the manufacturer’s recommended medium rate for incorporation. Prior to filling with potting soil, square holes were cut in the side of each container approximately 10 cm down from the top of the container. Square transparent plastic tubes measuring 30 by 4 by 4 cm (Sinclair & Rush Inc., Arnold, MO) were inserted into the holes in the center of each container, dividing it into two equal halves by surface area. Potting soil was then added until the soil level reached the top of the transparent tube but did not cover it. Thirty-five seeds of either large crabgrass (seed dimensions of 2.5 mm length, 0.9 mm width) or garden spurge (seed dimensions of 1 mm length, 0.5 mm width) were then surface-sown to one-half of each container, positioning seeds on the surface of the potting substrate (seeds beneath the mulch layer). Following seeding, three different types of mulch including PS (Pine Straw of Central Florida, Winter Garden, FL), PB (Timberline, Old Castle Lawn & Garden Inc., Atlanta, GA), or HW chips (Florida Select™ Natural Eucalyptus Mulch, Scotts®, Marysville, OH) were added on top of the entire substrate surface of each container at depths of 1.3, 2.5, 5, and 10 cm. Following mulching, an additional 35 seeds of large crabgrass or garden spurge were sown on the surface of the mulch layer on the other half of each container (seeds positioned on top of the mulch layer). Nonmulched controls were included for comparison. Controls were prepared by filling and seeding pots in the same manner as mulched pots, with the exception that seeds were sown directly on top of the potting substrate surface in both halves of each container, as no mulch was included. All containers were kept in full sun on an outdoor nursery pad and received 1.3 cm of irrigation per day via overhead impact sprinklers.
Light measurements of photosynthetic photon flux density (in µmol m–2s–1) under the surface of each mulch treatment described previously were determined in pots seeded with large crabgrass using a light-measuring sensor (LI-191R, LICOR® Environmental Inc., Lincoln, NB). The light sensor records light over the entire 1-m length of the sensor, so tin foil and a black plastic film were wrapped around the light meter and secured with tape so that only a 15-cm portion at the end of the sensor (15% of the entire sensor length) was exposed for recording measurements. Light intensity was recorded by inserting the end portion of the sensor (15 cm) into the transparent plastic tubes in the containers until the end of the sensor reached the opposite side of the container. This allowed for readings under a 15-cm length of mulch-covered surface. As pots were 23 cm in diameter, 8 cm under the mulch (i.e., 23 – 15 = 8 cm) were not included in readings to account for light that could enter at the opening of the plastic tube. All recordings were taken at noon on clear days. For each block, ambient light levels (full sunlight, unobstructed), light within the transparent plastic tubes (nonmulched controls), and light under mulch treatments were recorded for each block. To estimate light intensity at the soil surface beneath mulch, all readings, including ambient (full light) readings, were first divided by 0.15 to account for the portion of the sensor that was covered (85 cm). Second, a correction factor was calculated based on the percent reduction in light caused by the plastic tube using readings recorded in nonmulched controls by applying the formula (ambient full light – light inside tube)/ambient light). Readings recorded from the sensor under mulch treatments were then divided by this correction factor to estimate actual photosynthetic active radiation (PAR) on the soil surface.
The experiment was a randomized complete block design with four replications for each mulch and depth combination, with factorial arrangement of three mulch types, five different mulch depths, and two weed seed positions (on top of or beneath the mulch). Light measurements and weed counts were collected every 2 wk for 12 wk after trial initiation (WAT). After weeds emerged, they were counted but were not removed. Emerged garden spurge began to produce seeds at 8 WAT, thus data collected at 10 and 12 WAT included garden spurge counts resulting from seeds naturally deposited on top of mulch layers. Weed count data were analyzed separately for each weed species. Cumulative weed counts over the 12-wk study were converted to percent reduction in relation to the no-mulch controls prior to analysis and were inspected to ensure that the assumptions of ANOVA were met. Data were arcsine transformed when needed to satisfy the assumptions of ANOVA, but back-transformed data are presented in all cases for interpretation. Data were then subjected to ANOVA using SAS® (SAS 9.4, SAS Institute, Inc., Cary, NC), with trial run and replication nested within trial run as random effects and all other variables (mulch type, depth, and seed position) and interactions between these terms as fixed factors. Contrasts were used to test linear and quadratic response trends over mulch depth and interactions with mulch depth when significance was detected. Contrast coefficients were determined using PROCL IML in SAS, as mulch depths were unequally spaced. Fisher’s protected LSD test was used to compare between individual means of experimental variables at the 0.05 probability level. Light data were analyzed after accounting for the reduction in light from the plastic tubes as described above, and all data presented are PAR after applying this correction factor. Light data were analyzed in a similar manner to weed counts but were not converted to percent reduction prior to analysis and did not include seed position as an experimental variable. Neither ambient light nor light levels from the nonmulched controls within plastic tubes were included in the analysis.
Greenhouse Experiments
In addition to the above experiment, a similar greenhouse study was conducted in 2017 and repeated in 2018 at the Mid-Florida Research and Education Center. Round black plastic nursery containers (18 cm diam by 18 cm height) were filled with substrate similar to the outdoor container experiment. Plastic corrugated sheets (Highway Traffic Supply, Pearl River, NY) 13 cm by 15 cm were placed at the center of each container, dividing each container into equal halves. Twenty seeds of large crabgrass or garden spurge were sown to one-half of each container representing seeds below the mulch layer. Mulch materials described previously were added on top of the soil in each container at depths of 1.3, 2.5, 5, and 10 cm. Another 20 seeds of either large crabgrass or garden spurge were then sown on top of the mulch as described previously. Nonmulched controls with surface-sown weed seeds but without any mulch were also included in the study for comparison as described previously. All the containers were placed inside a greenhouse where the temperature was maintained between 21 and 35C and received 0.5 cm of overhead irrigation per day throughout the trial.
Weed counts were conducted every 2 wk for 12 wk. Immediately after weeds were counted, emerged weeds were pulled using forceps to remove weeds before seed was produced. Pulling weeds prior to seed production and maintaining the trial inside a fully enclosed greenhouse allowed for a more accurate determination of weed emergence through different mulch treatments based on seed placement. This trial was a completely randomized design with eight single-pot replications per treatment. Cumulative weed counts for each species were converted to percent reduction in relation to nonmulched controls prior to analysis. Data were analyzed separately for each weed species in the same manner as the outdoor container experiment.
Moisture Retention of Each Mulch Type
In spring 2018, moisture retention by three different mulch materials was determined by conducting laboratory experiments at the Mid-Florida Research and Education Center based on methods previously described by Altland et al. (Reference Altland, Boldt and Krause2016) with some modification. All procedures were conducted indoors in an air-conditioned laboratory maintained at 23 C with 50% relative humidity. Two-piece plastic Buchner funnels (13.1 cm inner diam, 6.6 cm height) (Thermo Scientific, Waltham, MA) were filled with 6 cm of either PB, PS, or HW mulch that had been spread into a single layer and air-dried in the laboratory until reaching a constant weight. It was established that a 6-cm layer of air-dried PB, PS, and HW in the 13.1-cm Buchner funnel (134.7 cm2 surface area) weighed 157 g, 41 g, and 75 g, respectively, based on the average weight of four samples. These weights were used to uniformly apply each mulch material to replicate funnels. After filling the funnels with mulch, funnels were weighed again to determine the weight of mulch-filled funnels (initial dry weight). Funnels were then placed over 900-ml glass jars (Golden Harvest Mason jar, Ball Crop®, Broomfield, CO), and 171 ml of water (equivalent to 1.3 cm of irrigation) was slowly and uniformly poured over the mulch surface inside each funnel (134.7 cm2) using a glass beaker. Water passing through the mulch layer was collected in the glass jar beneath. Percentage of moisture retained within the mulch at 0 h, immediately after water was applied, was calculated by measuring water volume collected in glass jars beneath mulch after dripping ceased and dividing by 171 ml. Mulch-filled funnels were then weighed at 1 h, 4 h, and 24 h after water was applied (wet weight). Percentage of water (by weight) retained within mulch at each timing (1 to 24 h) was calculated using the formula [(wet weight – initial dry weight)/171 ml] × 100. Moisture retention percentages included moisture both initially absorbed by mulch particles as well as water held between mulch particles. As no further dripping was observed after the 0-h collection period (after initial dripping ceased), further moisture loss determined at 1 h, 4 h, and 24 h resulted from evaporation. The experiment was a completely randomized design with five replications per mulch type and was repeated twice. All data were subjected to ANOVA after ensuring that normality assumptions were met. Data were pooled, as there were no experimental run-by-treatment interactions. Fisher’s protected LSD test was performed to compare water retention within each mulch type at each timing (0, 1, 4, or 24 h), and differences were considered significant at a 0.05 significance level.
Particle Size Analysis of Mulch Materials
This trial was conducted in the same laboratory described in the moisture retention study in 2018. PB, HW, and PS mulch were first spread out in a single layer inside the laboratory and allowed to dry until reaching a constant weight. Three 100-g samples of PS, PB, and HW were then each passed through USA standard sieves (WS Tyler®, Mentor, Ohio) including screen sizes of 25, 19, 12.5, 9.5, 6.3, 3.35, 1, and <1 mm. Sieves were stacked in descending order by screen size, and 100 g of mulch were placed inside the 25-mm screen. Sieve pans were shaken for 3 min using a Ro-Tap® test sieve shaker (W.S. Tyler, Mentor, OH). After 3 min, mulch deposited in each sieve size was weighed. Particle size analysis was determined for three replicates per mulch time, and the process was repeated twice. Weights retained within each sieve size were analyzed using ANOVA in SAS, and Fisher’s protected LSD test (P ≤ 0.05) was used to determine differences in mulch type at each sieve size.
Results and Discussion
Light Penetration, Moisture Retention, and Particle Size Analysis
Results from data captured with the quantum sensor showed that number of weeks after seeding was not a significant factor (P = 0.3042), as light reductions underneath the mulch materials did not vary over the 12 wk of the study (data not shown); therefore, data were pooled across all data collection periods. Previous research indicated that mulch materials such as HW, PB, and PS degrade and decompose over time, but significant decomposition is typically not observed for 4 to 6 mo (Duryea et al. Reference Duryea, English and Hermansen1999). Over the short duration of our study (12 wk), there was insufficient time to observe a significant rate of mulch decomposition, and thus the ability of the mulch materials to block out light remained constant throughout. Across all sampling dates, there was a significant mulch type-by-depth interaction (P < 0.001). At 1.3 cm depth, HW reduced ambient full-light PAR (97%) more than PB (90%) or PS (92%) (Table 1). At 2.5 cm, HW also reduced PAR to a greater degree than PB but HW was similar to PS. No differences in PAR were observed at mulch depths of 5.1 or 10.2 cm.
Table 1. Comparison of average photosynthetically active radiation (PAR) through three different mulch types at four depths.

a PAR was measured utilizing a quantum sensor placed underneath a transparent plastic tube inserted under mulch at soil level. Measurements were recorded and averaged over 12 wk. Average PAR beneath plastic tube was 784 µmol m−1 s−1, and unobstructed PAR was 1,014 µmol m−1 s−1. Data presented show estimated PAR observed on soil surface beneath mulch after accounting for the reduction in light caused by the transparent plastic tube.
b Means within a column followed by the same letter are not significantly different according to Fisher’s protected LSD test (P ≤ 0.05).
In the moisture retention study, HW retained more moisture at all sampling periods, followed by PS and then PB, which retained the least. At 24 h after water was applied, HW retained 29% of applied water (49.6 ml), followed by PS at 22% (37.6 ml) and then PB at 14% (23.9 ml) (Table 2). This indicates that HW would be the slowest mulch material to dry following rainfall or irrigation, whereas PB would probably dry more rapidly than the other materials. Results from the moisture retention study can be explained by examining the particle size analysis. Differences were detected at each sieve size (Table 3). However, examining the percent of mulch particles present in the largest and smallest sieves shows that HW had the highest percentage of smaller particles, whereas PB and PS had higher percentages of larger particle sizes (Table 3). Porometer procedures that identify water-holding capacity of different growth substrates show that materials with smaller particle sizes hold a higher percentage of water compared with substrates composed of larger particle materials (Bilderback et al. Reference Bilderback, Stuart, Owen and Albano2005). Not surprisingly, PB and PS dried more rapidly following the simulated irrigation, because they both had more large particles, most notably at the 25-mm screen size, comprising over 50% of both materials. Higher moisture levels in soils often result in a greater incidence of weed seed germination, because critical moisture levels must be reached for some weed species to break dormancy or to germinate (Pérez-Fernández et al. Reference Pérez-Fernández, Lamont, Marwick and Lamont2000). Mulch materials that dry quickly would be expected to provide superior weed control, especially when seeds are deposited on top of the mulch as may occur in landscape settings or nursery production environments.
Table 2. Moisture retention of a 6-cm depth of three different mulch materials.

a Percent retention was calculated at 0, 1, 4, and 24 h by weighing mulch-filled Buchner funnels before (initial dry weight) and after (wet weight) 171 ml of water (equivalent to 1.3 cm of irrigation over the 134.7-cm2 surface area of the funnel) was applied by hand using the formula [(wet weight – initial dry weight)/171 ml] × 100.
b Means within a column followed by the same letter are not significantly different based on Fisher’s protected LSD test (P < 0.05).
Table 3. Particle size analysis of three mulch types used in all experiments.

a Mulch was air-dried until reaching a constant weight, and then 100-g samples were passed through sieves in descending order. Means show weights (g) collected in each sieve size.
b Means within a column followed by the same letter are not significantly different based on Fisher’s protected LSD test (P ≤ 0.05).
Outdoor Container Experiments
Garden spurge emergence was influenced by mulch type (P < 0.0001), mulch depth (P < 0.0001), and seed position (P < 0.0001), but no interactions were detected (Tables 4 and 6). Averaged over other factors, the highest reduction in weed counts was observed in PB and PS (82% and 81% reductions, respectively) whereas HW reduced weed counts by only 54%, significantly less than the other two mulch types. Results also showed a greater reduction in garden spurge emergence when seeds were positioned below the mulch layer (77% reduction) compared with seeds positioned on top of mulch (66% reduction). Over all three mulch types and both seed positions, a significant quadratic response was observed for mulch depth, indicating a nonlinear decrease in garden spurge emergence as mulch depth increased.
Table 4. Mulch type, depth, and seed position effects on emergence of garden spurge (Euphorbia hirta) in outdoor experiments.a
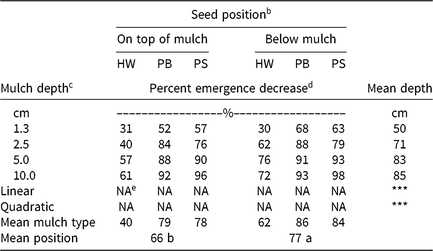
a Abbreviations: HW, hardwood; PB, pine bark; PS, pine straw.
b Refers to position of 35 garden spurge seeds. Seeds placed on top of mulch were sown directly on the mulch surface. Seeds placed below mulch were sown on soil surface beneath the mulch.
c Seeds were sown either on top of or below mulch applied at these mulch depths.
d Percent decrease was calculated based on percentage decrease in weed counts observed in a nonmulched control at 12 wk after sowing seeds.
e Asterisks represent significant linear or quadratic response at *P ≤ 0.05, **P ≤ 0.01, and ***P ≤ 0.001, based on orthogonal contrasts. NS, not significant; NA, not analyzed because of significant interactions.
Table 5. Mulch type, depth, and seed position effects on emergence of large crabgrass (Digitaria sanguinalis) in outdoor experiments.a

a Abbreviations: HW, hardwood; PB, pine bark; PS, pine straw.
b Refers to position of 35 large crabgrass seeds. Seeds placed on top of mulch were sown directly on the mulch surface. Seeds placed below mulch were sown on soil surface beneath the mulch.
c Seeds were sown either on top of or below mulch applied at these mulch depths.
d Percent decrease was calculated based on percentage decrease in weed counts observed in a nonmulched control at 12 wk after sowing seeds.
e Asterisks represent significant linear or quadratic response at *P ≤ 0.05, **P ≤ 0.01, and ***P ≤ 0.001, based on orthogonal contrasts. NS, not significant; NA, not analyzed because of significant interactions.
f Means of mulch type within a row and treatment variable followed by the same letter are not significantly different according to Fisher’s protected LSD test (P ≤ 0.05).
Table 6. Mulch type, depth, and seed position interactions with percent emergence for garden spurge (Euphorbia hirta) and large crabgrass (Digitaria sanguinalis) in outdoor experiments.

a ANOVA was performed to test for significance of main effects and interactions. Effects are considered significant at P ≤ 0.05.
A significant mulch type-by-depth-by-placement interaction was detected for large crabgrass (Tables 5 and 6). When seeds were positioned on top of the mulch, PB and PS reduced large crabgrass emergence more so than HW at depths of ≥2.5 cm. However, no difference in mulch type was detected at the shallowest depth of 1.3 cm, and emergence reductions were <60% in all three mulch types. A significant quadratic response was detected for both PB and PS for seeds positioned on top of the mulch but not for HW, indicating that large crabgrass emergence was similar at depths of 1.3 to 10 cm when seeds were positioned on top of HW. When seeds were positioned on top of mulch, PB (97% reduction) and PS (81% reduction) reduced emergence more so than HW (55%) at 2.5 cm, but no differences in mulch type were detected at depths of 5 or 10 cm, and all three mulch types reduced emergence by more than 98%. Similarly, significant quadratic responses in emergence reduction were observed for all three mulch types, indicating a nonlinear decrease in large crabgrass emergence as mulch depth increased.
Greenhouse Experiments
Significant mulch type-by-depth-by-seed position interactions were detected for both garden spurge and large crabgrass (Tables 7, 8, and 9). In garden spurge, no differences in emergence of top-positioned seed were detected between PB or PS at depths of 1.3, 5, or 10 cm, and both mulch types reduced emergence more than HW at all depths. Significant linear or quadratic emergence responses to mulch depth were also observed in both PB and PS, but not for HW, indicating that garden spurge emergence was similar in HW regardless of depth when seeds were positioned on top of mulch. When garden spurge seeds were positioned below mulch, no differences in mulch type were detected at any depth. Similar to outdoor container experiments, garden spurge emergence decreased in a mostly quadratic manner for all three mulch types, indicating a nonlinear decrease in emergence as depth increased.
Table 7. Mulch type, depth, and seed position effects on emergence of garden spurge (Euphorbia hirta) in greenhouse experiments.a

a Abbreviations: HW, hardwood; PB, pine bark; PS, pine straw.
b Refers to position of 20 garden spurge seeds. Seeds placed on top of mulch were sown directly on the mulch surface. Seeds placed below mulch were sown on soil surface beneath the mulch.
c Seeds were sown either on top of or below mulch applied at these depths.
d Percent decrease was calculated based on percentage decrease in cumulative weed counts observed in a nonmulched control at 12 wk after sowing seeds.
e Means of mulch type within a row and seed placement followed by the same letter are not significantly different according to Fisher’s protected LSD test (P ≤ 0.05).
f Asterisks represent significant linear or quadratic response at *P ≤ 0.05, **P ≤ 0.01, and ***P ≤ 0.001, based on orthogonal contrasts. NS, not significant; NA, not analyzed because of significant interactions.
Table 8. Mulch type, depth, and seed position effects on emergence of large crabgrass (Digitaria sanguinalis) in greenhouse experiments.a

a Abbreviations: HW, hardwood; PB, pine bark; PS, pine straw.
b Refers to position of 20 large crabgrass seeds. Seeds placed on top of mulch were sown directly on the mulch surface. Seeds placed below mulch were sown on soil surface beneath the mulch.
c Seeds were sown either on top of or below mulch applied at these depths.
d Percent decrease was calculated based on percentage decrease in cumulative weed counts observed in a nonmulched control at 12 wk after sowing seeds.
e Means of mulch type within a row and seed placement followed by the same letter are not significantly different according to Fisher’s protected LSD test (P ≤ 0.05).
f Asterisks represent significant linear or quadratic response at *P ≤ 0.05, **P ≤ 0.01, and ***P ≤ 0.001, based on orthogonal contrasts. NS, not significant; NA, not analyzed because of significant interactions.
Table 9. Mulch type, depth, and seed position interactions with percent emergence for garden spurge (Euphorbia hirta) and large crabgrass (Digitaria sanguinalis) in greenhouse experiments.

a ANOVA was performed to test for significance of main effects and interactions. Effects are considered significant at P ≤ 0.05.
Similar to results observed in garden spurge, PB or PS reduced large crabgrass emergence of top-positioned seed more than HW at all depths (Table 7, 8, and 9). Whereas PS reduced large crabgrass emergence more than PB at 1.3 cm depth, no differences were observed between PB and PS at any other depth. Contrast analysis showed that whereas a quadratic response was observed for depth in PB, no depth response was observed in PS or HW. HW generally failed to provide a reduction in emergence compared with nonmulched containers, as evidenced by negative values (indicating growth increases) at depths of 1.3 and 10 cm. In contrast, no depth response was observed in PS, but large crabgrass emergence was reduced by 67% to 92% at depths of 1.3 to 10 cm. When seeds were placed on top of mulch, PB and PS reduced emergence more than HW at a depth of 1.3 cm, but no differences were observed between different mulch types at depths of ≥2.5 cm. Large crabgrass emergence decreased linearly as PB depth increased. Quadratic responses were observed for depth in both HW and PS, indicating that large crabgrass emergence decreased nonlinearly as depth increased.
Data from outdoor container and greenhouse experiments indicate that mulch type, depth, and seed position will significantly influence emergence of garden spurge and large crabgrass. In regards to seed position, when seeds are placed on top of mulch with physical properties that allow for germination and/or emergence, depth will probably not be a significant factor in weed emergence. No depth response was observed in emergence of garden spurge in greenhouse experiments or large crabgrass in either experiment when seeds were placed on top of HW. Although moisture levels were not monitored in outdoor or greenhouse experiments, moisture retention and particle size analysis results indicate that HW retained moisture longer than PB or PS. As emergence of both large crabgrass and garden spurge tend to increase as soil moisture increases (King and Oliver Reference King and Oliver1994; Sauerborn et al. Reference Sauerborn, Koch and Krage1988), it is likely that HW physical characteristics, and consequently greater moisture retention, contributed to the higher rates of emergence in HW compared with PB or PS. As PB and PS retained less moisture than HW, this would explain the ability of PB and PS to reduce emergence of seeds deposited on top of mulch more so than HW at almost all depths evaluated.
Although depth was not a significant factor in emergence of seeds positioned on top of HW, depth was significant for PB and PS. Based on physical properties and moisture retention results, it is likely that PB and PS reduced emergence of top-positioned seed primarily through moisture reduction. This is similar to the flexuous bittercress control reported when seeds are deposited on top of rice hull mulch (Altland et al. Reference Altland, Boldt and Krause2016). When mulch provides weed suppression through moisture reduction, depth will probably be a significant factor. Depths of at least 2.5 cm were required for PB and PS to achieve at least 60% reduction in emergence of garden spurge and crabgrass in outdoor and greenhouse experiments. This result is similar to previous findings with flexuous bittercress, yellow woodsorrel (Oxalis stricta L.) (Richardson et al. Reference Richardson, Gilliam, Fain and Wehtje2008), and spotted spurge (Cochran et al. Reference Cochran, Gilliam, Eakes, Wehtje, Knight and Olive2009), in which PB depths of at least 2.5 cm were needed to reduce germination of top-positioned seed. At PB depths of ≤1.3 cm, data reported by Cochran et al. (Reference Cochran, Gilliam, Eakes, Wehtje, Knight and Olive2009) and Richardson et al. (Reference Richardson, Gilliam, Fain and Wehtje2008) are in agreement with data reported here, suggesting that many weed species will be able to germinate and could possibly root into soil beneath such a shallow mulch depth. Shallow mulch depths (≤1.3 cm) may also allow seeds to be washed beneath mulch layers where they may germinate and emerge from the soil beneath the mulch, as the physical barrier would be minimal.
When seeds of either species were positioned below mulch, depth responses were typically nonlinear but significant for all three mulch types. In greenhouse experiments, there was no difference in garden spurge emergence among the three different mulch types regardless of depth, and no difference between mulch types in large crabgrass emergence at depths of ≥2.5 cm. Significant depth responses in emergence would be expected for seeds positioned below mulch, as both a greater physical barrier and more light would be reduced at higher depths (Penny and Neal Reference Penny and Neal2003). Garden spurge is considered positively photoblastic, with only 1% germination reported in darkness (Rooden et al. Reference Rooden, Akkermans and van der Veen1970). In contrast, many Digitaria spp. can germinate in total darkness, although germination is reduced compared with high light levels (Chauhan and Johnson Reference Chauhan and Johnson2008). We observed significant reductions in both species as mulch depth increased, suggesting that in addition to limiting light, the creation of a physical barrier is an important mechanism of weed control provided by mulch. Data from the present study agree with previous reports by Teasdale and Mohler (Reference Teasdale and Mohler2000) suggesting that depths >2.5 cm would sufficiently reduce light levels and provide a greater physical barrier to emergence of seeds below mulch; thus, mulch type would become less significant at greater depths. It should be noted that many weed species germinate and grow through mulch applied at much greater depths, especially in the case of perennial species such yellow nutsedge (Cyperus esculentus L.) (Chen et al. Reference Chen, Strahan and Bracy2013). Therefore, higher depths may be needed for other weed species, or may be used in addition to PRE herbicides for more thorough control (Marble Reference Marble2015). It is also important to note that HW reduced light to a greater degree than did PB or PS at 1.3 cm as a result of having a larger percentage of smaller particle material, which tends to reduce light levels more than larger particle materials applied a similarly shallow depth (Teasdale and Mohler Reference Teasdale and Mohler2000). However, any advantage that HW may provide in terms of suppressing light at a depth of 1.3 cm may be lost, as acceptable control was not achieved until a 2.5-cm depth was applied in most cases. Furthermore, the creation of a physical barrier, especially a physical barrier that dries rapidly following rainfall or irrigation, may be more important than the reduction of light for species that can germinate in darkness. This is evidenced by a greater reduction in large crabgrass emergence by PB and PS at depths of 1.3 or 2.5 cm, even as HW reduced light to a greater degree.
Depending upon seed position and depth, emergence of garden spurge and large crabgrass was affected by mulch type. However, particle size, and not the actual material comprising the mulch, is probably the most important factor in determination of the weed-suppressive ability of mulch if equal depths are applied. In many previous studies, authors only report weed control achieved with different mulch materials (e.g. wood chips, pine straw, pine bark nuggets, etc.) but omit particle size analysis or other physical characteristics from the discussion. All these organic mulch materials can be processed in many different ways, and this processing and the resulting particle size distribution present in the mulch will probably have a profound influence on the ability of the mulch to control weed growth.
The preponderance of data suggest that nursery growers and landscape contractors should choose mulch that contains a high percentage of large (>25 mm) particles, so that mulch materials dry as soon as possible after rainfall or irrigation events. When applied at depths of at 5 cm, mulch composed of mostly large (>25 mm) particles resulted in an 80% reduction in weed emergence over 12 wk regardless of seed position. Other mulch materials that have much smaller particle sizes could also be suitable if they dry rapidly following irrigation or rainfall, such as rice hulls (Altland et al. Reference Altland, Boldt and Krause2016). For existing seeds in soils (below the mulch layer), depth will probably be the most important factor in weed control, and it is likely practitioners would observe few differences in mulch type in regards to germination of existing seeds. However, use of mulch composed of smaller particles such as HW would probably result in faster degradation (Duryea et al. Reference Duryea, English and Hermansen1999) and result in greater weed growth when weed seeds are deposited on top of the mulch over time. Although mulch durability was not evaluated in our study, previous research has shown that bark materials are slower to decompose than wood products or PS (Duryea et al. Reference Duryea, English and Hermansen1999). Applying mulch at depths at higher levels, such as 5 cm, would allow more time to pass in between subsequent mulch applications to maintain mulch at needed levels once the mulch begins to decompose (Duryea et al. Reference Duryea, English and Hermansen1999). As container nursery growers theoretically begin with weed-free potting media, use of PB would be most appropriate, as it provided a high level of weed control when seeds were placed on top of the mulch, and would probably be easier to apply than PS in small containers. In landscapes, any of these mulch materials could be applied for suppression of existing seed banks, but PB or PS may offer advantages for seeds that are deposited on top of the mulch over time. Although HW provided a high level of control of weed seeds placed below the mulch layer, control would probably increase if this material was processed to be composed of large particle sizes.
Acknowledgments
The authors wish to acknowledge Annette Chandler for technical assistance and the University of Florida/Institute of Food and Agricultural Science Center for Landscape Conservation and Ecology for partial funding support for this project.
Conflicts of Interest
No conflicts of interest are declared.