Introduction
Metchnikovellidae is a family of microsporidia belonging to the monotypic class Rudimicrosporea Sprague, Reference Sprague, Bulla and Cheng1977. It unites intracellular parasites of gregarines inhabiting the intestine of polychaetes and some other marine invertebrates. At present, approximately 30 species of metchnikovellids are known. However, only eight of them were studied by modern methods, including transmission electron microscopy (TEM) (Vivier and Schrével, Reference Vivier and Schrével1973; Hildebrand, Reference Hildebrand1974; Desportes and Thèodoridés, Reference Desportes and Théodoridès1979; Ormiéres et al., Reference Ormières, Loubès and Maurand1981; Larsson, Reference Larsson2000; Larsson and Køie, Reference Larsson and Køie2006; Sokolova et al., Reference Sokolova, Paskerova, Rotari, Nassonova and Smirnov2013, Reference Sokolova, Paskerova, Rotari, Nassonova and Smirnov2014). These studies showed that the spores of metchnikovellids have distinctive structures. Unlike typical microsporidia, they do not possess a coiled polar filament, anchoring disk, polaroplast and posterior vacuole. Instead, they have short, straight and wide polar filaments, the so-called manubria, which expand into manubrial cisterns (Sokolova et al., Reference Sokolova, Paskerova, Rotari, Nassonova and Smirnov2013; Larsson, Reference Larsson, Weiss and Becnel2014). Merogony was never observed in these organisms, and it is assumed that it is absent in the life cycle (Larsson, Reference Larsson2000; Larsson and Køie, Reference Larsson and Køie2006). There are two types of sporogony. One results in the formation of so-called free spores, and the other produces the spore-containing sacs (‘cysts’). The shape, size and structure of spore sacs are believed to be genus-specific traits (Vivier, Reference Vivier1975; Larsson, Reference Larsson, Weiss and Becnel2014).
Recent phylogenomic analyses have robustly placed metchnikovellids as a basal group for ‘core’ microsporidia (Mikhailov et al., Reference Mikhailov, Simdyanov and Aleoshin2017; Galindo et al., Reference Galindo, Torruella, Moreira, Timpano, Paskerova, Smirnov, Nassonova and López-García2018; Nassonova et al., Reference Nassonova, Bondarenko, Paskerova, Kováčiková, Frolova and Smirnov2021) confirming the earlier hypothesis that these organisms represent a primitive group of Microsporidia (Vivier, Reference Vivier1975; Sprague, Reference Sprague, Bulla and Cheng1977; Weiser, Reference Weiser1977; Larsson, Reference Larsson, Weiss and Becnel2014). However, the configuration of the metchnikovellid tree remains poorly resolved, and taxon sampling is limited. To achieve progress in this field, further morphological and molecular studies of metchnikovellids are necessary.
The species, currently called Metchnikovella spiralis Sokolova, Paskerova, Rotari, Nassonova et Smirnov, Reference Sokolova, Paskerova, Rotari, Nassonova and Smirnov2014, was discovered in 1984 in the eugregarines Polyrhabdina sp. (Apicomplexa: Eugregarinida) inhabiting the intestine of polychaetes Pygospio elegans Claparède, 1863 (Polychaeta: Spionidae) from the White Sea (Rotari and Paskerova, Reference Rotari and Paskerova2007). The microsporidia possessed a unique structure – a cord spirally wrapped around the spore sac. This feature was initially suggested to be a genus-level characteristic. However, in 2010 this species was reisolated and studied by TEM (Sokolova et al., Reference Sokolova, Paskerova, Rotari, Nassonova and Smirnov2014). The obtained data showed that this organism, accord to its basic characteristics, fits the diagnosis of the genus Metchnikovella. Hence, it was described as M. spiralis. Since 2010, this species has never been seen in the original sampling site despite annual monitoring, but in 2019 it was isolated from another location in the White Sea at a distance of ca. 9 km from the original site. The description of M. spiralis was completed with new morphological data and with the results of phylogenetic analyses based on the sequence of the SSU rRNA gene.
Materials and methods
In 2019, P. elegans bristle worms were collected at the Podpahta channel (66°18.108′N, 33°37.775′E), located in the Chupa Inlet, Kandalaksha Bay, White Sea. The animals were maintained at +10°C under room light in 500 mL containers with seawater that was periodically changed. For examination, the polychaetes were individually pressed between a coverslip and an object slide to release the content of the gut under a Leica M205C dissection microscope equipped with Rottermann contrast. The obtained squashed preparations were examined using a Leica DM 2500 microscope equipped with DIC optics and a Leica DFC295 digital camera. In cases when a gregarine showing indications of infection (unusual heterogeneity of the cytoplasm, visible individual spores or spore sacs) was found, a series of optical sections from each specimen were recorded using a 100× PlanApo objective lens to collect images of the cell at different focal planes. In some cases, to increase the focal depth, we applied Z-stacking of two images made at different focal planes using Adobe Photoshop software (www.adobe.com/ru/products/photoshop.html).
Furthermore, a large amount of Millipore-filtered seawater was added under the coverslip, which was detached from the object slide. The gregarines containing free spores and spore sacs of M. spiralis were collected under the coverslip using a hair-thin tapered-tip Pasteur pipette, washed in a fresh portion of Millipore-filtered seawater and placed in 200 μL PCR tubes with 1–2 μL of water. Each tube was manually controlled for the presence of gregarine using a Leica M205C dissection microscope at high magnifications. DNA extraction in these tubes was performed using Arcturus® PicoPure® DNA Extraction Kit (Thermo Fischer Scientific, Waltham, MA, USA). The purified DNA was amplified by Multiple Displacement Amplification (MDA) using Repli-g Single Cell Amplification Kit (Qiagen, Hilden, Germany), according to the manufacturer's protocol. SSU rRNA gene was amplified using 1:10 diluted product of MDA reaction as a template with microsporidia-specific primers: 18F, 530R (Weiss and Vossbrinck, Reference Weiss, Vossbrinck, Wittner and Weiss1999) and 1353TnR (a modification of the primer V1492R) 5′-GCAGCCTTGTTACGACTT-3′. PCR program parameters were the following: initial denaturation (5 min at 95°C) followed by 35 cycles of 30 s at 95°C, 50 s at 50°C and 90 s at 72°C, followed by 7 min at 72°C for final extension. Amplicons were purified using Cleanup Mini Purification Kit (Eurogen, Moscow, Russia) or with ExoSAP-IT™ PCR Product Cleanup Reagent (Thermo Fisher Scientific, Waltham, MA, USA). The Sanger sequencing reactions were carried out using the Applied Biosystems™ BigDyeTM Terminator v3.1 Cycle Sequencing Kit (Thermo Fischer Scientific, Waltham, MA, USA) and sequenced using Applied BiosystemsTM 3500xL Genetic Analyzer (Thermo Fischer Scientific, Waltham, MA, USA). The final length of the assembled contig was 1238 bp.
The SSU rRNA gene sequence alignment was created using MUSCLE algorithm (Edgar, Reference Edgar2004) implemented in SeaView v. 4.6.1 (Gouy et al., Reference Gouy, Guindon and Gascuel2010), followed by manual polishing. The alignment included the representative set of sequences of core microsporidia from five identified clades (Vossbrinck et al., Reference Vossbrinck, Debrunner-Vossbrinck and Weiss2014), all available sequences of metchnikovellids, and a representative set of sequences of early diverging microsporidia, rozellids, aphelids and nucleariids to form a proper set of outgroups. The maximum likelihood (ML) phylogenetic analysis was performed using RAxML-HPC2 v. 8.2.12 (Stamatakis, Reference Stamatakis2014) at CIPRES portal (Miller et al., Reference Miller, Pfeiffer and Schwartz2010). GTR + γ model of evolution with 25 substitution rate categories was applied. A total of 100 independent ML inferences with distinct randomized MP starting trees were performed; the best-scoring tree was tested using non-parametric bootstrapping (1000 pseudoreplicates). Bayesian analysis was performed with MrBayes v. 3.2.6 (Ronquist et al., Reference Ronquist, Teslenko, Van Der Mark, Ayres, Darling, Höhna, Larget, Liu, Suchard and Huelsenbeck2012) at CIPRES portal using GTR model with γ correction for intersite rate variation (eight categories) and the covarion model. Trees were run as two separate chains (default heating parameters) for 15 million generations, by which time they had ceased converging (final average standard deviation of the split frequencies was <0.01), the first 25% of generations were discarded for burn-in.
The SSU rRNA gene sequence obtained in this study was deposited with the GenBank under the accession number: MW344837.
Results
Occurrence and prevalence
Among the 108 examined polychaetes, 87 specimens (80.6%) contained eugregarines Polyrhabdina sp. The number of gregarines per worm varied from 1 to 60. In 26 polychaetes (24.1%), the gregarines were infected with metchnikovellids. Of them, in 13 polychaetes (12%), the gregarines contained only the early stages of metchnikovellid proliferation, and no free spores or spore sacs were found. Hence, it was impossible to identify the infecting organism in these gregarines. The spore sacs of M. incurvata were present in gregarines from 11 polychaetes (10.2%). Two worms were parasitized with gregarines containing spore sacs of M. spiralis, which represented 1.85% of all examined polychaetes. In one case, there was a mixed infection. Gregarines containing spore sacs of either M. incurvata or M. spiralis were found in the same polychaete. However, both species have never been observed in one gregarine cell.
Light microscopy
The following variants of the infected gregarine cells were observed: (1) with free spores only, (2) with numerous free spores and a small number of spore sacs and (3) with numerous spore sacs and few clusters of free spores.
Free spores were oval, 2.5–3.5 × 2.1–2.3 μm (av. 3.1 × 2.1 μm, n = 30) in maximal dimension. They form clusters residing in parasitophorous vacuoles (Fig. 1). Spore sacs of M. spiralis were oval, 10.3–16.5 μm (av. 14.6 μm, n = 45) in length and 5.4–7.1 μm (av. 6.1 μm, n = 45) in width. The number of spore sacs varied from one to nine per gregarine cell. Each sac was enclosed individually in a spacious parasitophorous vacuole (Fig. 2A). Spore sacs were twined with a cord, which was coiled as a tightly packed spiral around them. The spiral cord had approximately 50 coils and formed a kind of outer carcass around the sac (Fig. 2B and C). The wall of the spore sac never contacted the membrane of the parasitophorous vacuole. The sacs ornamented with the spiral cord were termed ‘spiral sacs’ in Sokolova et al. (Reference Sokolova, Paskerova, Rotari, Nassonova and Smirnov2014). In the squashed preparation, parasitophorous vacuoles were sometimes squeezed out of the host cell through rupture in the gregarine pellicle. In such cases, the vacuoles were significantly increased in size, and the distance between the coils of the spiral cord was increased as well (Fig. 2D). Sometimes, in isolated parasitophorous vacuoles pressed with a coverslip, the spiral cord was relaxed and slipped out of the spore sac (Fig. 2E–G). If the vacuole containing a spore sac was destroyed, the spiral arrangement of the cord immediately relaxed completely (Fig. 2H). It was impossible to detect any contact of the cord with the surface of the sac.
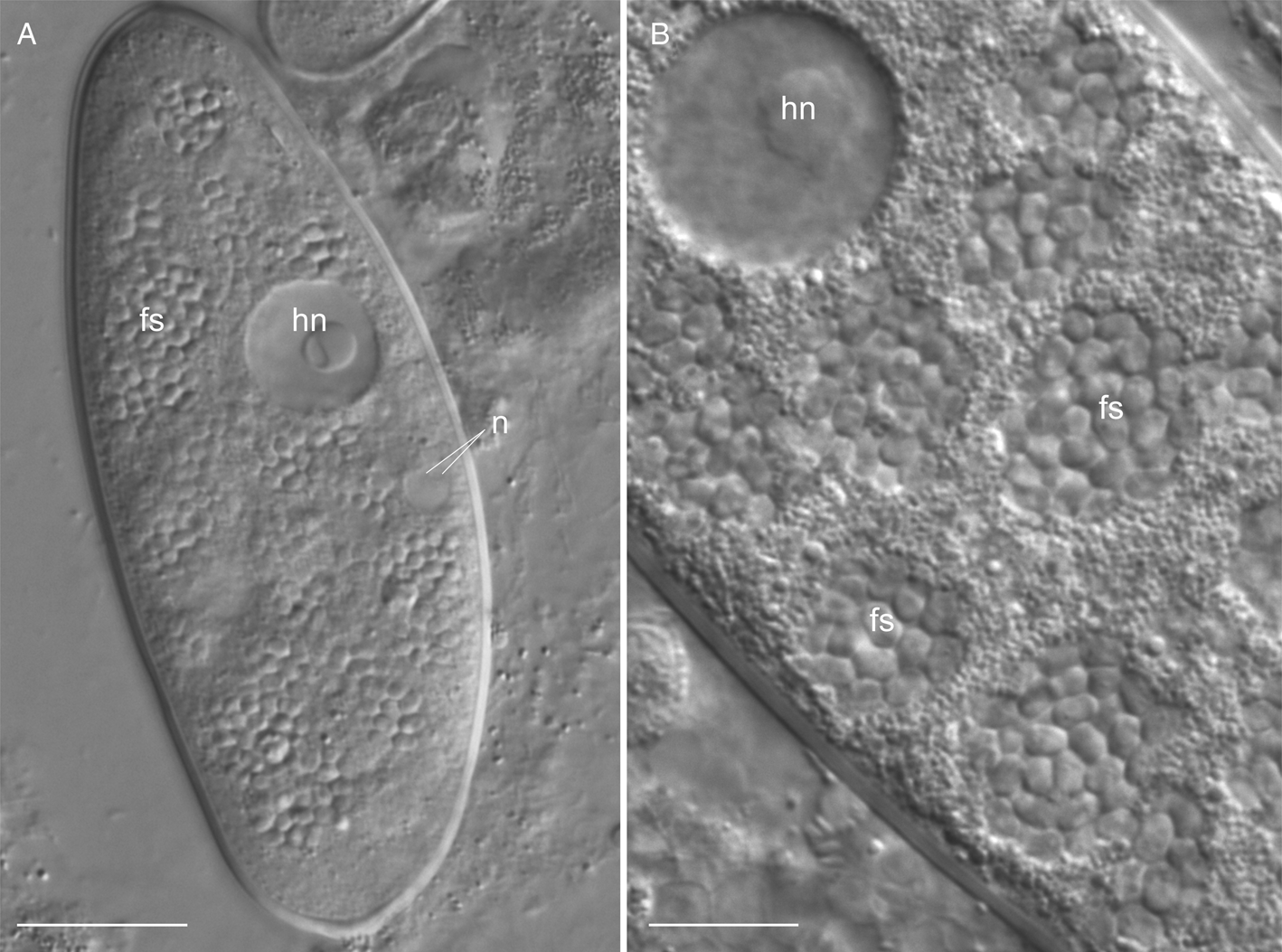
Fig. 1. Living microsporidia Metchnikovella spiralis, early stages of infection in the eugregarine Polyrhabdina sp., differential interference contrast (DIC). (A) Host cell filled with free spores of the hyperparasite; (B) clusters of free spores. fs, free spores of the metchnikovellid; hn, host nucleus; n, nucleus of sporont mother cell. Scale bars: 20 μm (A), 10 μm (B).
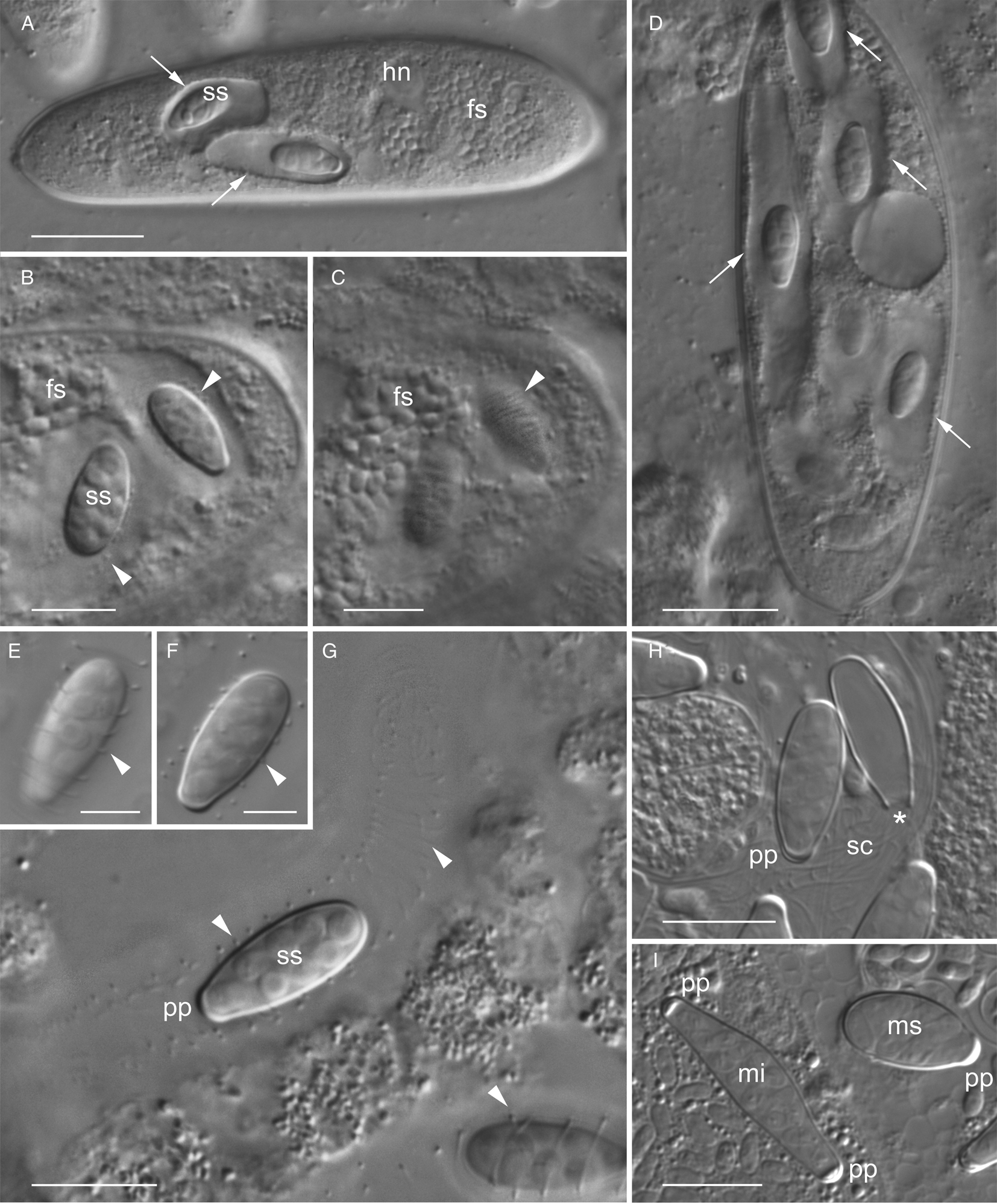
Fig. 2. Living microsporidia Metchnikovella spiralis, late stages of infection in the eugregarine Polyrhabdina sp., differential interference contrast (DIC). (A) Infected gregarine with spore sacs within the individual parasitophorous vacuoles (arrows) and numerous free spores, stacked image; (B) infected gregarine slightly pressed with the coverslip for better visualization of the intracellular parasites, arrowheads point to ‘spiral sac’ with enclosed spores. (C) The same as (B), another focal plane of the same site showing striation corresponding to the cord spirally entwined around the sac; (D) the squashed preparation showing the elongated parasitophorous vacuoles (arrows), which are releasing from the host cell; (E, F) spore sac at different focal planes, showing the coiling of the spiral cord (arrowheads); (G) an isolated parasitophorous vacuole enclosing the spore sac with spiral cord slightly relaxed and slipped out of the sac (arrowheads); (H) spore sacs with completely relaxed and unwound spiral cord and an empty spore sac, asterisk marking the place, where a detached polar plug used to be; (I) spore sacs of two species of metchnikovellids isolated from one specimen of the worm: M. spiralis with one polar plug, M. incurvata with two plugs. It is a case of mixed infection in the population of Polyrhabdina sp. fs, free spores; hn, host nucleus; mi, M. incurvata; ms, M. spiralis; pp, polar plug; sc, spiral cord; ss, spore sac. Scale bars: 20 μm (A, D), 10 μm (B, C, G–I), 5 μm (E, F).
Each spore sac contained eight oval spores, arranged in two rows and measuring 2.4–3.5 × 2.4–2.9 μm (av. 2.9 × 2.6 μm, n = 30) in length and width, respectively (Fig. 2E–G). Spore sacs had one polar plug, appearing as a thickening at one pole of the sac (Fig. 2E–I). In contrast, the spore sacs of M. incurvata found in the gregarines with mixed infection were elongated, slightly bent and had two polar plugs (Fig. 2I).
A schematic drawing of the spore sac of M. spiralis is shown in Fig. 3.
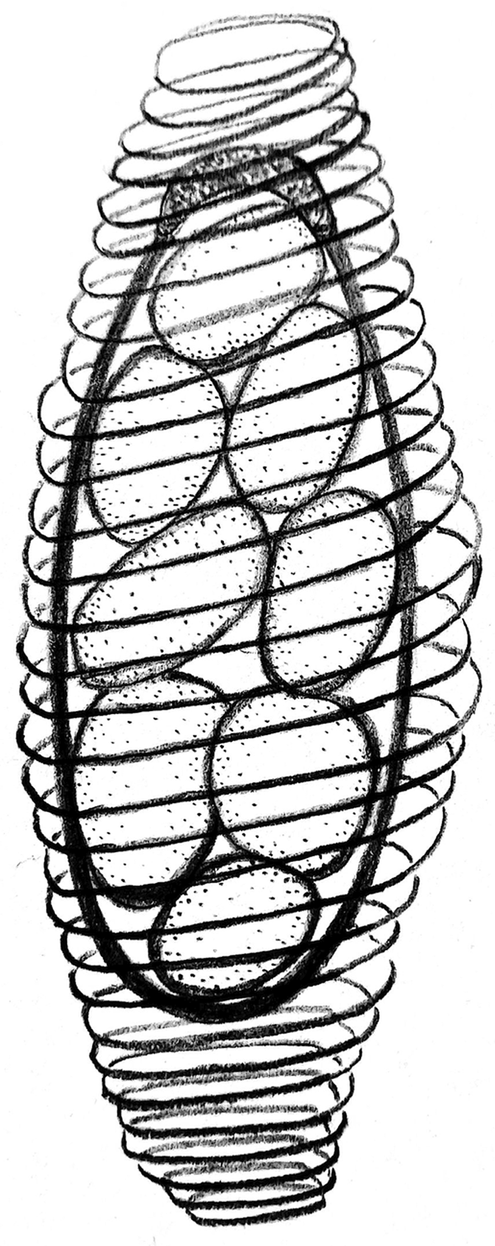
Fig. 3. A schematic drawing of the Metchnikovella spiralis spore sac. The number of spiral cord coils in the diagram is deliberately halved to show the contents of the spore sac.
Molecular phylogeny
In the reconstructed SSU rRNA tree (Fig. 4), all main clades of ‘core’ microsporidia (sensu Vossbrinck et al., Reference Vossbrinck, Debrunner-Vossbrinck and Weiss2014, Fig. 6.3a) were robustly recovered, excluding clade 2. The latter was split into two groups. The group Paranosema/Antonospora was retrieved as a sister to clade 3 with rather high support, whereas the group Nematocida/Ovavesicula constituted a highly supported basal lineage of the core microsporidia. This topology is congruent with recently published phylogenies, where the splitting of clade 2 into two distinct lineages was also observed (Mikhailov et al., Reference Mikhailov, Simdyanov and Aleoshin2017; Williams et al., Reference Williams, Hamilton, Jones and Bass2018; Nassonova et al., Reference Nassonova, Bondarenko, Paskerova, Kováčiková, Frolova and Smirnov2021). The position of the Hamiltosporidium/Neoflabelliforma group within clade 3 (as was shown in Vossbrinck et al., Reference Vossbrinck, Debrunner-Vossbrinck and Weiss2014) was not recovered, which is congruent with the results of Mikhailov et al. (Reference Mikhailov, Simdyanov and Aleoshin2017) and Nassonova et al. (Reference Nassonova, Bondarenko, Paskerova, Kováčiková, Frolova and Smirnov2021). With rather high support in Bayesian analysis (but with low support in the ML trees), this group was retrieved as a sister to a well-supported assemblage uniting clade 4 and clade 5.

Fig. 4. SSU rRNA phylogeny of Microsporidia and related lineages including the sequence of Metchnikovella spiralis retrieved in this study (in bold). Black blobs indicate support values of ⩾0.99 Bayesian posterior probability (PP) and ⩾99% ML bootstrap (BS). PP and BS values are indicated above the branches; support values PP ⩽0.50 and BS <50% are not shown; ‘–’ indicates that the pattern of branching was not recovered by one of the reconstruction methods used. The major microsporidian clades established by Vossbrinck et al. (Reference Vossbrinck, Debrunner-Vossbrinck and Weiss2014, Fig. 6.3a) are labelled. Dotted line shows the clade 2, which occurred to be paraphyletic. The clades with environmental sequences are labelled as LazJam x with Latin numerals according to the designations introduced by Lazarus and James (Reference Lazarus and James2015); the designations of the clades are according to Corsaro et al. (Reference Corsaro, Michel, Walochnik, Venditti, Müller, Hauröder and Wylezich2016, Reference Corsaro, Walochnik, Venditti, Hauröder and Michel2020), Ishida et al. (Reference Ishida, Nozaki, Grossart and Kagami2015), Nagahama et al. (Reference Nagahama, Takahashi, Nagano, Abdel-Wahab and Miyazaki2011), Richards et al. (Reference Richards, Leonard, Mahé, Del Campo, Romac, Jones, Maguire, Dunthorn, De Vargas, Massana and Chambouvet2015) are also used. Isolated and described taxa from these lineages are indicated.
Metchnikovellids constituted a robustly supported group branching at the base of ‘core’ microsporidia. The metchnikovellid sequences formed two sister clades. One was highly supported (1.00/100%) and comprised the sequences of Amphiacantha spp., environmental clone p1_44 (GenBank KX214678) and M. spiralis. The second clade was only moderately supported (0.89/67%) and encompassed available sequences of Amphiamblys spp. and two other Metchnikovella species. This topology is congruent with the hypothesis of Larsson (Reference Larsson, Weiss and Becnel2014), who proposed the transfer of the representatives of the genus Amphiacantha from a single family Metchnikovellidae into a separate family Amphiacanthidae.
Metchnikovella spiralis did not form a sister branch to either M. incurvata or M. dogieli, as might be expected, and the sequences of Metchnikovella spp. did not form a monophyletic clade.
The positions of Chytridiopsis typographi and Nucleophaga spp. were unstable in the tree, which is consistent with our previous analysis (Nassonova et al., Reference Nassonova, Bondarenko, Paskerova, Kováčiková, Frolova and Smirnov2021) and with the results obtained by Mikhailov et al. (Reference Mikhailov, Nassonova, Shishkin, Paskerova, Simdyanov, Yudina, Smirnov, Janouškovec and Aleoshin2021). In the Bayesian analysis, Nucleophaga spp. had a more basal position than C. typographi, yielding the topology demonstrated by Corsaro et al. (Reference Corsaro, Wylezich, Venditti, Michel, Walochnik and Wegensteiner2018, Reference Corsaro, Walochnik, Venditti, Hauröder and Michel2020). However, in the ML trees, the pattern of branching was inverted [in some ML trees the grouping of C. typographi within clade X (sensu Lazarus and James, Reference Lazarus and James2015) was observed]; in either case, the support was low (PP 0.60–0.74; BS 40–66%) and the backbone of the tree in the intermediate zone between long-branch and short-branch microsporidia (sensu Bass et al., Reference Bass, Czech, Williams, Berney, Dunthorn, Mahé, Torruella, Stentiford and Williams2018) was not resolved, which may have been caused by the rapid evolutionary rate and highly derived character of the C. typographi SSU rRNA sequence.
The topology of the lower part of the SSU rRNA tree corresponded to that observed in recent studies (Lazarus and James, Reference Lazarus and James2015; Corsaro et al. Reference Corsaro, Michel, Walochnik, Venditti, Müller, Hauröder and Wylezich2016, Reference Corsaro, Wylezich, Venditti, Michel, Walochnik and Wegensteiner2018, Reference Corsaro, Walochnik, Venditti, Hauröder and Michel2020; Grossart et al., Reference Grossart, Wurzbacher, James and Kagami2016; Mikhailov et al., Reference Mikhailov, Simdyanov and Aleoshin2017; Stentiford et al., Reference Stentiford, Ramilo, Abollo, Kerr, Bateman, Feist, Bass and Villalba2017; Bass et al., Reference Bass, Czech, Williams, Berney, Dunthorn, Mahé, Torruella, Stentiford and Williams2018; Williams et al., Reference Williams, Hamilton, Jones and Bass2018; Nassonova et al., Reference Nassonova, Bondarenko, Paskerova, Kováčiková, Frolova and Smirnov2021). The main clades of rozellids, including those classified as short-branched microsporidia by Bass et al. (Reference Bass, Czech, Williams, Berney, Dunthorn, Mahé, Torruella, Stentiford and Williams2018), were recovered mostly with their usual composition.
Discussion
Identification and occurrence of M. spiralis
The unique structure of the spore sacs, including the presence of the spiral cord ('spiral sacs’), the size of the sacs and of the individual spores and the number of spores in each sac left no doubt that for the first time since 2010 the species M. spiralis was isolated albeit from a different sampling site in the White Sea (the distance between Levin Reach, the site of isolation in 2010, and Podpahta channel, the site of isolation in 2019, is approximately 9.33 km by sea).
According to a survey in 2010, when 333 worms were examined, only three polychaetes contained gregarines with M. spiralis (0.9%), while 11 polychaetes contained M. incurvata-infected gregarines (3.3%) (Rotari et al., Reference Rotari, Paskerova and Sokolova2015). In the current study, M. spiralis was detected in 1.85% of the studied polychaetes, while M. incurvata was found in 10.2% of worms. This supports the suggestion that M. incurvata (as a species probably introduced from the Atlantic region) turned out to be more competitive and invasive than M. spiralis (Rotari et al., Reference Rotari, Paskerova and Sokolova2015).
The mixed invasions of two metchnikovellids were found in host populations, but gregarine cells infected with both hyperparasites at once have never been observed. This may be a result of the low occurrence of mechnikovellids or suppression of the development of one hyperparasite by another. For example, M. incurvata may inhibit M. spiralis development. It might be dealing with post-invasion modifications of the gregarine pellicle resulting in its resistance to the penetration of the second species or with fast proliferation of the first (‘pioneer’) parasite resulting in a rapid depletion of resources of the host cell and inhibition of any further parasite proliferation.
Parasitophorous vacuole and spiral cord
According to Sokolova et al. (Reference Sokolova, Paskerova, Rotari, Nassonova and Smirnov2014), the space around the spore sac in the parasitophorous vacuole of M. spiralis is filled with fine filamentous material. The spiral cord seems to consist of the same compacted and twisted filamentous material and is suggested to be a derivate of the external layer of the spore sac wall. The spiral cord probably serves as an external skeleton of the sac, which may help to maintain the volume of the parasitophorous vacuole in the gregarine cell (Sokolova et al., Reference Sokolova, Paskerova, Rotari, Nassonova and Smirnov2014). The elongation of vacuole and uncoiling of the spiral cord observed under a light microscope occurred due to coverslip pressure and can be considered an artefact; however, it remains unclear how this cord behaves under natural conditions when the spore sac is released in the environment.
To date, the spiral cord of M. spiralis remains a unique example of such a complex spore sac structure among metchnikovellids. To a certain extent, it may be possible to compare it with the ornamentation of sporophorous vesicles of ‘core’ Microsporidia; however, this kind of structure is also rare. For example, Trichodubosqia epeori possesses sporophorous vesicles with needle-like appendages approximately 20 μm long ‘with internal material similar to collagen’ (Batson, Reference Batson1982). In contrast to rare external structures, different kinds of structures and inclusions are often observed in the episporontal space of many species of ‘core’ microsporidia (Vávra and Larsson, Reference Vávra, Larsson, Weiss and Becnel2014). For instance, Trichoctosporea pygopellita and Agglomerata volgensae have fibrous projections of sporoblasts, connecting the exospore with the envelope of the sporophorous vesicle (Larsson, Reference Larsson1994; Larsson and Voronin, Reference Larsson and Voronin2000). The functional significance of such structures is also unclear.
Possible relationships with the genus Amphiacantha
Phylogenetically, the sequence of the SSU rRNA gene M. spiralis turned out to be closer to those belonging to the representatives of the genus Amphiacantha rather than that of Metchnikovella spp. This is surprising to a certain extent because these two genera are very different in morphology and biology. Members of the genus Amphiacantha have spindle-shaped spore sacs, terminating as thread-like prolongations. They do not have polar plugs. The length of sacs may exceed the width more than ten times, and they contain approximately 50 roundish spores (Caullery and Mesnil, Reference Caullery and Mesnil1914; Larsson, Reference Larsson2000). Species of the genus Amphiacantha develop directly in the host cytoplasm, and parasitophorous vacuoles have never been observed in the course of their development and around the mature spore sacs. Therefore, differences are numerous and basic, and it is impossible to suggest that M. spiralis might be a member of the genus Amphiacantha.
However, theoretically, it is possible to consider a homology of the spiral cord in M. spiralis with the thread-like prolongations of the spindle-shaped spore sacs of Amphiacantha. Logically, we can speculate that the spiral cord in M. spiralis originated as a result of the further development of long terminal threads seen in Amphiacantha spore sacs, which start to coil around the sac. The similarity of the fine structure of the cord with the material of the external sac wall, noted by Sokolova et al. (Reference Sokolova, Paskerova, Rotari, Nassonova and Smirnov2014), and the putative site of contact of the cord with the envelope of the spore sac shown in the same study (Sokolova et al., Reference Sokolova, Paskerova, Rotari, Nassonova and Smirnov2014; Fig. 4C) may be an indication in favour of this suggestion. Thus, molecular phylogeny probably indicates the relationships between the species, showing cord-like structures as a part of the spore sac. However, the sequences of SSU rRNA gene in metchnikovellids are highly divergent, and unambiguous alignment of some regions of the SSU gene is almost impossible in these organisms. The number of available sequences of metchnikovellids is very low, and all of them represent relatively long branches in the phylogenetic tree. This might result in the underestimation of genetic distances and complicates the task of the phylogenetic reconstruction. The involvement of additional markers (e.g. phylogenomics data) and/or expanding the taxon sampling is highly desirable to further progress towards a reconstruction of robust phylogeny of metchnikovellids and the taxonomical conclusions.
Taxonomic issues
Metchnikovella spiralis was found in 1984 in the eugregarine Polyrhabdina sp. from the gut of the polychaete P. elegans. The species, due to the unusual spiral ornamentation around the spore sac (as was visible in the LM optics of that time), was presumed to belong to a new genus, which was provisionally named ‘Vivierus spiralis’ (Rotari, Reference Rotari1988). Later, this name (as ‘Vivieria spiralis’) appeared in the abstract of the Proceedings of VII European Congress of Protistology, published in the journal ‘Protistology’ (Rotari and Paskerova, Reference Rotari and Paskerova2007). Because neither of these names was published according to the International Code of Zoological Nomenclature rules (ICZN, 4th edition, Chapter 3, Article 9), the genus name ‘Vivierus’ (‘Vivieria’) cannot be considered validly published.
Sokolova et al. (Reference Sokolova, Paskerova, Rotari, Nassonova and Smirnov2014) argued that the species studied was identical to one previously described under the name ‘Vivieria spiralis’. However, this study showed that it fits the formal diagnosis of the genus Metchnikovella based on the following characteristics: (1) length of the spore sacs does not exceed ten times the width; (2) a small number (eight) of sac-bound spores per sac; and (3) oval sac-bound spores (Hildebrand and Vivier, Reference Hildebrand and Vivier1971; Vivier and Shrével, Reference Vivier and Schrével1973; Sokolova et al., Reference Sokolova, Paskerova, Rotari, Nassonova and Smirnov2013, Reference Sokolova, Paskerova, Rotari, Nassonova and Smirnov2014). Taking into account the similarity of several ultrastructural characteristics with Metchnikovella (oval sac-bound spores, horseshoe-shaped nucleus, ornamentation of the exospore of free spores, cysts with polar thickening), it was decided that there is no reason to establish a new genus for this isolate (Sokolova et al., Reference Sokolova, Paskerova, Rotari, Nassonova and Smirnov2014, p. 1118).
The definition of the genus Metchnikovella is very wide. In light of modern data, this genus looks rather heterogeneous compared with the relatively narrowly defined genera Amphiamblys and Amphiacantha (reviewed in Nassonova et al., Reference Nassonova, Bondarenko, Paskerova, Kováčiková, Frolova and Smirnov2021). Dogiel (Reference Dogiel1922) suggested a new genus, Caulleryetta, for metchnikovellids whose spore sacs possess only one plug. Larsson (Reference Larsson, Weiss and Becnel2014) supported this suggestion, although the validity of the genus Caulleryetta was not justified by other researchers (e.g. Vivier and Schrével, Reference Vivier and Schrével1973; Vivier, Reference Vivier1975).
The main difficulty in verifying the taxonomy of metchnikovellids is the lack of modern data on the type species Metchnikovella spionis Caullery & Mesnil, 1897, and Metchnikovella (‘Caulleryetta’) mesnili Dogiel, Reference Dogiel1922. This is one of the main reasons why modern researchers prefer to utilize older systems based on the original classification by Caullery and Mesnil (Reference Caullery and Mesnil1914, Reference Caullery and Mesnil1919) supported by Vivier (Reference Vivier1975). Switching between different systems before obtaining molecular data for key representatives of the genus Metchnikovella seems to be premature.
Metchnikovella spiralis has oval spore sacs with only one polar plug, so based on this character, it could be classified as Caulleryetta. However, M. dogieli also has one polar plug but does not group with M. spiralis in the SSU rRNA tree. The latter species cannot be classified in the genus Amphiacantha; however, it shows some affinities with this lineage. Most likely, the suggestion to establish a new genus for M. spiralis, as proposed by Rotari and Paskerova (Reference Rotari and Paskerova2007), is reasonable; however, we would prefer not to propose such a revision before phylogenomic data confirm this position of M. spiralis. The practical solution at the moment is to leave the paraphyletic genus Metchnikovella as it is defined by Vivier (Reference Vivier1975) unless molecular data on the type species of this genus become available. For substantial revision of the genus, data on the type species are highly desirable.
Taxonomic summary of M. spiralis should be corrected as the single polar plug of the spore was not mentioned in the original description of M. spiralis. This feature is one of the important discriminating criteria and should be included in the diagnosis of this species. Sokolova et al. (Reference Sokolova, Paskerova, Rotari, Nassonova and Smirnov2014) concluded that the free sporogony sequence occurred at the same time as the sac-bound sporogony, while our observations show that the free sporogony precedes the sac-bound one.
Taxonomic summary
Metchnikovella spiralis Sokolova et al., Reference Sokolova, Paskerova, Rotari, Nassonova and Smirnov2014, emend.
This species differentiates from congeners and other metchnikovellids by the unique structure of the spore sac: a light-reflecting thick wall with one polar thickening and an external carcass composed of dense cord spirally coiled around the sac, visible in the light microscope as regularly arranged striations on the surfaces of the sac. On ultrathin sections, the external envelope can be seen as tangential or cross-sectioned filaments regularly arranged in the lumen of parasitophorous vacuole between the sac wall and membrane of the vacuole. Free sporogony occurs before sac-bound sporogony. Other details are described in Sokolova et al. (Reference Sokolova, Paskerova, Rotari, Nassonova and Smirnov2014).
Type locality: Levin reach, Chupa Inlet, Kandalaksha Gulf of the White Sea, Russia (66°17.878′N, 33°27.774′E).
Type host: eugregarines Polyrhabdina sp. (Apicomplexa: Eugregarinida) from the polychaete P. elegans Claparède, 1863 (Polychaeta: Spionidae).
Locality and host used in the emended diagnosis: Podpahta Channel, Chupa Inlet, Kandalaksha Bay, White Sea (66°18.108′N, 33°37.775′E); Polyrhabdina sp. from P. elegans.
Type material: as designated in Sokolova et al., Reference Sokolova, Paskerova, Rotari, Nassonova and Smirnov2014.
Deposition of materials used in the emended diagnosis. LM images of eugregarines infected with M. spiralis are in the image collection of the Department of Invertebrate Zoology, St Petersburg State University. Figures 1 and 2 (this publication) show some specimens. Frozen purified genomic DNA of the infected gregarines as well as the individual infected gregarine cells fixed in 96% ethanol are stored in the same department.
Gene sequences: SSU rDNA gene sequence of M. spiralis has been deposited in GenBank under accession number MW344837.
Acknowledgements
The authors thank the staff of Educational and Research Station ‘Belomorskaia’ of Saint Petersburg University (SPbU) for providing facilities for field sampling and material processing, as well as for their kind and friendly approach. This study utilized equipment of the Core Facility Centres ‘Biobank’, ‘Development of Molecular and Cell Technologies’ and ‘Culture Collection of Microorganisms’ of the Research Park of Saint Petersburg State University. We are grateful to anonymous reviewers for their valuable comments on the manuscript.
Author contribution
E.V.F. and E.S.N. suggested an overall concept and design of the study, conducted molecular work, performed molecular phylogeny, drafted the manuscript; E.V.F. and G.G.P. performed the field work, dissection of polychaetes, isolation of the parasites; A.V.S. and E.V.F. did light microscopy and single-cell manipulations. All authors contributed to the improvement of the draft and accepted the final version of the manuscript.
Financial support
This study was financially supported by the Russian Science Foundation – project No. 19-74-20136 (molecular work, phylogenetic analysis) and by the Russian Foundation for Basic Research – project No. 18-04-01359 (field work, dissection, light microscopy).
Conflict of interest
None.
Ethical standards
This article does not contain any studies with human participants or warm-blooded animals performed by any of the authors. The Educational and Research Station ‘Belomorskaia’ of Saint Petersburg University at the White Sea has permission to collect invertebrate animals for scientific work on its own territory and other sites situated around the station. The invertebrates of interest are neither endangered nor protected species within the region. Animal handling and dissecting was performed at cold temperature to avoid distress and unnecessary suffering.