INTRODUCTION
The salmon louse, Lepeophtheirus salmonis, is an ectoparasitic copepod of Atlantic salmon (Salmo salar). Control of infections has been largely through the use of chemotherapeutic drugs that are selectively toxic to the parasite (Pike and Wadsworth, Reference Pike and Wadsworth1999). Emamectin benzoate (EMB), the active drug in SLICE®, is currently one of the most effective chemotherapeutants administered against sea lice infections (Schering Plough Animal Health, 2007) and represents the most commonly used compound to control sea lice infections in Atlantic Canada (Westcott et al. Reference Westcott, Hammell and Burka2004). SLICE® is safe for salmon when administered at a dose of 50 μg kg−1 for a 7-day period and at this dose is highly efficacious (99%) killing all parasitic stages of sea lice, i.e. chalimus, pre-adults and adults including gravid females (Stone et al. Reference Stone, Sutherland, Sommerville, Richards and Varma2000). When SLICE® is administered to fish, it is readily absorbed in the gut due to its highly lipophilic nature and is distributed into areas such as the skin and mucus (Kim-Kang et al. Reference Kim-Kang, Bova, Crouch, Wislocki, Robinson and Wu2004; Sevatdal et al. Reference Sevatdal, Magnusson, Ingebrigtsen, Haldorsen and Horsberg2005). When sea lice feed on treated fish, EMB is, presumably, absorbed through the intestinal tract and distributed into the tissues of the lice. Avermectins bind irreversibly to glutamate-gated chloride channels causing an increased flow of chloride ions into nerve cells, which results in paralysis and death of the nematodes and arthropods (Martin et al. Reference Martin, Robertson, Wolstenholme, Vercruysse and Rew2002). It is assumed that EMB acts similarly.
Members of the ATP-binding cassette (ABC) superfamily are expressed constitutively in many tissues including the intestinal epithelia, blood-brain barrier, bile canaliculi, and renal tubules in mammals (Cascorbi, Reference Cascorbi2006). They function as ATP-dependent transporters for a wide number of structurally diverse compounds including anticancer drugs, steroid hormones, cardiac drugs, immunosuppressants, antimicrobial agents and other miscellaneous agents including the macrocyclic lactone avermectins (Cascorbi, Reference Cascorbi2006). Due to the wide variety of compounds recognized, some ABC transporters, including P-glycoprotein (P-gp), play a major role in the absorption, distribution, metabolism and excretion of many drugs. Different studies have demonstrated the presence of P-gps in marine vertebrates and invertebrates using immunological methods (Hemmer et al. Reference Hemmer, Courtney and Ortego1995; Lyons-Alcantara et al. Reference Lyons-Alcantara, Lambkin, Nordmo, Lyng and Mothersill2002). Induction and elevation of P-gp has been reported in various aquatic invertebrates, including snails (Smital and Kurelec, Reference Smital and Kurelec1998), shrimp (Fulton et al. Reference Fulton, Moore, Wirth, Chandler, Key, Daugomah, Strozier, Devane, Clark, Lewis, Finley, Ellenberg, Karnaky and Scott1999), mussels, and oysters (Minier et al. Reference Minier, Akcha and Galgani1993), following exposure to xenobiotics, including pollutants. Studies directed at immunolocalization of P-gps in teleosts showed that the distribution resembled that seen in mammals (Hemmer et al. Reference Hemmer, Courtney and Ortego1995).
Selection at a P-gp allele has been implicated in ivermectin resistance development in numerous invertebrates including the trichostrongyle nematode Haemonchus contortus (Blackhall et al. Reference Blackhall, Liu, Xu, Prichard and Beech1998) and the filarial nematode Onchocerca volvulus (Ardelli et al. Reference Ardelli, Guerriero and Prichard2005). Constitutive over-expression of P-gp has been documented in ivermectin-resistant H. contortus (Xu et al. Reference Xu, Molento, Blackhall, Ribeiro, Beech and Prichard1998). P-gp expression has not previously been identified in sea lice and its detection could prove useful in understanding the potential for resistance development to SLICE®. Lobsters (Homarus americanus) served as a reference crustacean during this study due to their size, availability, and previous use as a model organism to gauge EMB toxicity (Burridge et al. Reference Burridge, Hamilton, Waddy, Haya, Mercer, Greenhalgh, Tauber, Radecki, Crouch, Wislocki and Endris2004). They demonstrated EMB toxicity at extremely high concentrations (relative to the concentrations used in the field), which is not surprising given the larger size of lobsters relative to sea lice. In this paper we report for the first time the identification and localization of a putative ABC transporter in L. salmonis using molecular and immunological methods.
MATERIALS AND METHODS
Tissue collection for RNA and protein isolation
Adult L. salmonis were collected from Atlantic salmon farms in southeastern New Brunswick. Lice were carefully removed using forceps and placed in a glass container with sea water from the location of the farm being sampled. Once in the laboratory, live lice were flash frozen and stored at −80°C.
Livers were removed from Sprague Dawley rats that had been euthanized in accordance to Canadian Council on Animal Care (CCAC) guidelines. The livers were flash frozen in liquid nitrogen and stored at −80°C until used.
RNA isolation and cDNA synthesis
Total L. salmonis RNA was isolated from 100 mg of tissue using the RNeasy kit (Qiagen, Mississauga, ON) following the manufacturer's protocol. First-strand cDNA synthesis was primed using oligo-dT primers with the Superscript III First Strand Synthesis Kit (Invitrogen, Burlington, ON) following the manufacturer's protocol.
Sea lice expressed sequence tag (EST) database search
Two L. salmonis EST databases were made available to screen for conserved motifs previously identified in the P-gp sequences of other invertebrate species. The EST libraries were located at the NRC Institute of Marine Biosciences, Halifax, NS, and at the Institute of Marine Research, Bergen, Norway. From these databases only a single clone, SL0525, was identified in the EST library in Halifax; the EST library in Bergen did not identify a clone with any homology. The SL0525 clone was sequenced commercially by ACGT Corp. (Toronto, ON).
Sequence analysis
Sequence analysis used the BLAST programs (Altschul et al. Reference Altschul, Gish, Miller, Myers and Lipman1990) to find sequence similarity to the L. salmonis SL0525 gene sequence in the GenBank database. Amino acid sequences were aligned to invertebrate P-gps using ClustalW (Thompson et al. Reference Thompson, Higgins and Gibson1994). The species used to align the SL0525 gene were H. contortus P-GP-A (GenBank Accession no. AF003908), O. volvulus P-pg-1 (GenBank Accession no. AF083642), several P-gp isoforms of Caenorhabditis elegans P-gp-1 – P-gp-4 (GenBank Accession nos. NM_059306, NM_063273, NM_077409, NM_075086, NM_076804, NM_077727, NM_077726, NM_077410, NM_077411, NM_077500, NM_077501, NM_077725, NM_001047823, NM_001047822, NM_070012) and Apis mellifera P-gp (GenBank Accession no XM_623561). For phylogenetic analysis, the NCBI database was searched for sequences encoding for various ABC proteins in nematodes and arthropods. These sequences, along with the L. salmonis genes, were aligned (using ClustalW on the BioEdit sequence alignment editor) and a 330 bp conserved amino acid sequence (encompassing the Walker A and glutamine loop regions) encoding for the conserved ATP-binding region was identified for phylogenetic analysis. Bootstrapped neighbour-joining phylogenetic analysis (1000 iterations) was performed using MEGA Version 3.1 (Kumar et al. Reference Kumar, Tamura and Nei1994).
Construction of SL0525 pGEX expression plasmid and production of fusion protein
The antigen used for SL0525 polyclonal antibody production was a fusion protein prepared from bacterial sonicates of E. coli transformed with pGEX plasmids containing the partial open reading frame (ORF) of the SL0525 gene (717 bp). Polymerase chain reaction (PCR) primers bracketing the partial SL0525 ORF with the sense primer designed to contain a BamHI restriction site (5′ Cgg atc cGA AAC GTC GCA TTT ACA G) and the antisense primer designed to contain the XhoI restriction site (5′ Gct cga gTT AAG ATT GAA CAA CAG C) were used to amplify the partial SL0525 gene. The PCR product was subcloned into the pCR®2.1 TOPO® vector (Invitrogen) and then transferred to the pGEX-4T-1 vector (Pharmacia, Kirkland, QC) double digested with BamHI and XhoI, which was then used to transform competent BL2 strain to express the glutathione S-transferase (GST) fusion protein. The SL0525 GST-fusion protein was purified to homogeneity by affinity chromatography on the GST column and cleavage of the protein from GST with thrombin (Fikrig et al. Reference Fikrig, Barthold, Kantor and Flavell1990). The concentration of the purified SL0525 protein was estimated by spectrophotometry at 280 nm and then analysed by sodium dodecyl sulfate-polyacrylamide gel electrophoresis (SDS-PAGE). Aliquots containing 200 μg of the SL0525 protein were stored at −80°C prior to use for immunization of a rabbit.
Production of polyclonal antibodies
Rabbit polyclonal antiserum to the purified SL0525 protein was prepared using a female New Zealand white rabbit (Charles River Canada, Saint-Constant, QC) in accordance with the guidelines of the CCAC following a standard protocol as described previously (Kibenge et al. Reference Kibenge, Lyaku, Rainnie and Hammell2000).
Commercial antibodies used for P-gp detection
The mAbs C219 and JSB-1 (Abcam, Cambridge, MA) are directed towards specific epitopes present in human P-gp encoded by MDR1. The C219 antibody shows a greater cross-species reactivity, but in human protein samples has been demonstrated to cross-react with phospholipid transporters encoded by MDR3. C219 also cross-reacts with a 200 kDa protein which migrates to the same position as myosin and with C-erb B2 (a tyrosine kinase receptor) which is 37 kDa in size (Liu et al. Reference Liu, Sun, Xia, Hung and Yu1997). The JSB-1 antibody is specific to MDR1 and unlike C219 shows no cross-reactivity with MDR3. JSB-1 has, however, shown cross-reactivity with pyruvate carboxylase (Rao et al. Reference Rao, Antony and Piwnica-Worms1995).
Western blot analysis
Tissues were weighed and placed in 4X volume of ice-cold Tris/KCl buffer containing a general protease inhibitor. Tissues were placed on ice and homogenized using 30 sec pulses with a hand-held homogenizer. The tissue homogenate was centrifuged at 300 g for 20 min at 4°C. The supernatant was collected and centrifuged at 100 000 g for 30 min at 4°C. The pellet containing the membrane fraction was resuspended in 250 μl of Tris/KCl buffer and stored at −20°C until required.
The isolated protein fraction was quantitated using the Bio-Rad protein assay (Bio-Rad, Mississauga, ON), based on the Bradford dye-binding procedure (Bradford, Reference Bradford1976). An aliquot of protein was added to an equal volume of 2X loading buffer. Samples were then boiled for 3 min and proteins separated by electrophoresis on a 7·5% SDS-PAGE. Electrophoresis was conducted for 4 h at 75 mA. Following electrophoresis, the samples were transferred at 25 V for 1 h onto a nitrocellulose membrane. Non-specific binding sites were blocked for 1 h with 5% non-fat dry milk in PBS after the transfer. Monoclonal antibodies, C219 and JSB-1, were diluted 1:50 and the SL0525 polyclonal antibody was diluted 1:5000 in antibody dilution buffer (phosphate-buffered saline Tween 20 (PBST) (0·1%) containing 5% non-fat dry milk). The nitrocellulose membrane was incubated with C219 or JSB-1 overnight or SL0525 for 2 h at room temperature. The membrane was washed with PBST (0·1%) (3×15 min), and incubated with a horseradish peroxidase-linked secondary anti-mouse (C219 or JSB-1) or anti-rabbit (SL0525) antibody diluted 1:5000 and 1:16 000, respectively, for 1 h followed by rinsing with PBST (0·1%) (3×15 min). The membrane was exposed to Amersham ECL detection agent according to the manufacturer's instructions and exposed to an X-ray film for visualization.
Tissue preparation for immunolocalization
Sea lice were obtained as described above. After removal from the salmon, sea lice were immediately placed in a fixative solution. Atlantic salmon and lobsters used were donated by colleagues at the Atlantic Veterinary College. Animals were euthanized according to CCAC guidelines. Fish were placed in a bath containing a lethal dose of buffered tricaine methanesulfonate at a concentration of 500 mg l−1; lobsters were injected with a lethal concentration of KCl (100 mg g−1). Intestine (lobster and salmon), hepatopancreas (lobster), kidney (salmon), and liver (salmon) were rapidly removed after euthanization and immersed in fixative. Tissues were subsequently cut into smaller pieces of 50 mm2 to ensure optimal fixation. For paraffin embedding, tissues were placed in one of each of the following fixatives for 72 h: 10% buffered neutral formalin; Bouin's fixative; Dietrich's fixative. Specimens fixed in Bouin's were rinsed for 4 h in running tap water. All tissues were then dehydrated in ethanol, cleared in xylene, and embedded in paraffin. Tissues were sectioned at 5 μm, placed on positively charged slides, and either air dried overnight or oven dried at 56°C for 3 h.
Sea lice were also placed in a variety of cryoprotecting solutions for frozen tissue sectioning. The solutions used included: glycerol/phosphate solution; sucrose/phosphate solution with sucrose final concentration of 10%, 20%, and 30%. Sea lice were embedded in Shandon Cryomatix™ (Fisher) on a freezing stage placed on a pool of liquid nitrogen. Blocks of frozen tissue in Cryomatrix™ were cut into 25–40 μm sections on a cryomicrotome at −20°C and placed on positively charged slides.
Immunohistochemistry procedure
Tissue sections were deparaffinized and rehydrated. To optimize antigenicity some sections fixed in formalin were incubated for 10 min in a sodium citrate solution buffer (pH 6·0) pre-heated to 95°C. The buffer was allowed to cool on ice for 5 min before further processing of the sections. All sections were then pre-treated for 10 min with 1% hydrogen peroxide (H2O2) in water, and rinsed in phosphate-buffered saline (PBS).
Atlantic salmon and lobster tissue sections were blocked with 5% normal horse serum (NHS) for 20 min. Sea louse tissue sections were not incubated in NHS before addition of the primary antibody. All sections were then incubated overnight at 4°C in a moist chamber with one of the following primary antibodies: C219 (1:40), JSB-1 (1:50), or SL0525 (1:16 000). The sections were washed with PBS and primary antibody was detected using either the mouse IgG (C219 or JSB-1) or rabbit IgG (SL0525) Vectastain®Elite ABC Kits (Vector Laboratories, Burlingame, CA) according to the manufacturer's instructions, with a minor modification: the secondary biotinylated antibody was used at a dilution of 1:100 in PBS. All sections were washed with PBS and incubated in a solution of 3,3′-diaminobenzidine tetrahydrochloride and H2O2 for 2 min. Negative controls included serial sections of tissue to which either (1) no primary antibody was added; (2) rabbit IgG directed towards an antigen known not to be present in any of the tissue sections had been applied at the same concentration of SL0525; (3) use of SL0525 after absorption with the antigen it was originally raised against and (4) pre-immunization serum. These controls were processed in parallel with test sections.
RESULTS
EST library products and sequence analysis
A search of 2 different sea lice EST databases for the conserved assumed P-gp motifs identified a single clone, SL0525, in one database. This clone was sequenced generating a DNA sequence of 717 bp. Alignment of the predicted amino acid sequence of SL0525 with other invertebrate P-gps showed the highest similarity within the conserved regions VSGGQ, GTSGGGKT, and VVILDE which correspond to the Signature and Walker A and B motifs (Loo et al. Reference Loo, Bartlett and Clarke2002), although the whole amino acid sequence showed low overall similarity (~35%) (Fig. 1). The BLAST search identified SL0525 as an ABC protein, with the highest amino acid sequence similarity (65%) to ABC protein subfamily F, mouse and rat GCN20 proteins. The phylogenetic analysis grouped the SL0525 and SL-Pgp1 genes with ABC-F and ABC-B respectively, thus supporting the results of the BLAST search (Fig. 2).
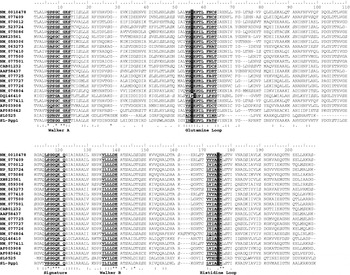
Fig. 1. Amino acid alignment of nematode and arthropod P-glycoproteins. Species aligned are Haemonchus contortus, Onchocerca volvulus, Caenorhabditis elegans, Sarcoptes scabiei, Apis mellifera and Lepeophtheirus salmonis. GenBank Accession numbers are displayed in the table. Conserved motifs Walker A and B, signature sequence, and glutamine and histidine loops are in bold and underlined. Conserved regions are indicated by (*), conserved substitutions by (:), and semi-conserved substitutions by (.). Conserved residues in the glutamine and histidine loops are highlighted.
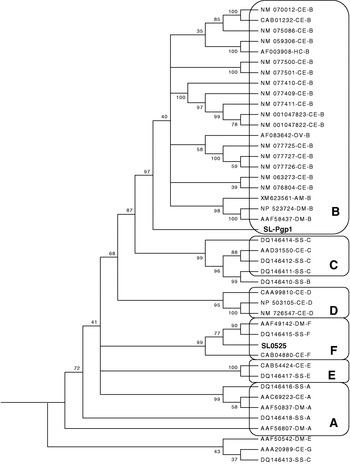
Fig. 2. Dendrogram of Lepeophtheirus salmonis and selected Sarcoptes scabiei (SS), Onchocerca volvulus (OV), Haemonchus contortus (HC), Drosophila melanogaster (DM) and Caenorhabditis elegans (CE) ABC protein ATP-binding domains. GenBank Accession numbers, species, and protein subfamily are displayed in the tree; sea lice genes can be identified by their assigned names (SL0525 and SL-Pgp1).
Production of SL0525 polyclonal antibody
In order to obtain a specific antibody for use to detect the corresponding protein in sea louse preparations, a purified SL0525 protein was prepared and used to immunize a rabbit. Cells of BL21 E. coli transformed with pGEX-4T-1 vector alone and induced with 0·1 mm isopropyl D-thiogalactopyranoside (IPTG) produced a protein of 26 kDa (GST protein; Fig. 3A, lane 1). E. coli transformed with pGEX-4T-1-SL0525 plasmid and induced with IPTG produced a protein of around 53 kDa (GST–SL0525 fusion protein; Fig. 3A, lanes 2 and 3). Purification using a glutathione–Sepharose column and thrombin cleavage yielded uncontaminated SL0525 protein (Fig. 3B). Western blotting showed that the SL0525 protein could be specifically recognized by the polyclonal antibody against this protein. There was no cross-reactivity with the GST portion of the fusion protein, indicating that a highly efficient protein purification procedure was performed for the SL0525 protein (Fig. 3C). Pre-immunized rabbit serum was used as a negative control and did not react with the SL0525 protein.
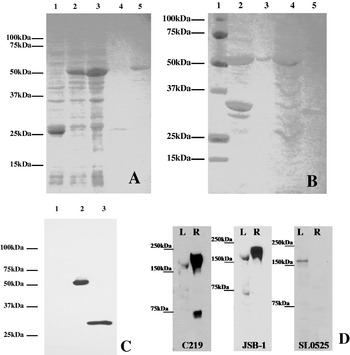
Fig. 3. (A and B) GST-fusion protein analysis run on a 12% SDS-PAGE stained with Coomassie Blue dye. (A) Solubilization of GST fusion protein and purification using glutathione beads; Lane 1, IPTG-induced control GST protein cell lysate; Lanes 2 and 3, IPTG-induced GST fusion protein cell lysate; Lane 4, Control GST protein elution after purification with glutathione beads; Lane 5, GST fusion protein elution after purification with glutathione beads. (B) Purification of SL0525 protein: Lane 1, Protein ladder; Lane 2, IPTG-induced GST fusion protein cell lysate; Lane 3, GST fusion protein; Lane 4, Bacterial protein elution with GST fusion protein removed; Lane 5, Thrombin cleaved SL0525 protein. (C) Specificity analysis of anti-GST-SL0525 antiserum using Western blotting. Lane 1, GST protein; Lane 2, GST-SL0525 protein; Lane 3, Thrombin cleaved SL0525 protein. (D) Immunoreactivity in both sea lice and rat liver using C219, JSB-1. and comparison to the protein detected by the SL0525 antibody (Western blot analysis). L: 50 μg adult sea louse membrane protein; R: 50 μg rat liver membrane protein.
Protein detection using Western blot analysis
Using either of the C219, JSB-1 or SL0525 antibodies each detected a single band of ~160 kDa in the sea louse protein sample (Fig. 3D), which is consistent with the molecular mass for P-gp. In the control rat liver protein sample, a single band of ~170 kDa was consistently detected with C219 and JSB-1; this is the molecular mass expected for P-gp in rats. The SL0525 polyclonal antibody did not react with any rat protein. The C219 monoclonal antibody showed cross-reactivity to a smaller unknown protein of ~70 kDa in the rat liver, presumably possessing similar epitope homology to the C219 P-gp binding site. The JSB-1 monoclonal antibody showed weak cross-reactivity to a smaller unknown protein of ~70 kDa in the sea louse preparations. Incidentally, neither C219 nor JSB-1 showed cross-reactivity to the partial SL0525 protein, suggesting that the epitopes recognized by either antibody are not present within this portion of the sea louse protein. C219 reacted only weakly with an ~170 kDa protein from salmon intestine, but did not react with lobster intestinal tissue proteins (results not shown). JSB-1 antibody only reacted with the ~170 kDa band obtained from rat liver.
Tissue processing and ABC transporter antibody immunostaining characteristics
Of the 3 fixatives, only the formalin-fixed tissues exhibited staining reactions in sea lice, Atlantic salmon, and American lobster. Antigen retrieval using a sodium citrate buffer enhanced the signal intensity of all antibodies, although this process was not essential for a staining reaction. Air drying the tissue sections rather than heat drying was essential for a staining reaction since there was no staining of heat-dried tissues. Cryoprotection of sea lice for frozen tissue sectioning using any of the solutions did not sufficiently preserve the integrity of the sea lice for successful staining.
Immunolocalization of ABC transporters in sea lice
Although crustaceans, sea lice are copepods and therefore do not possess a hepatopancreas to compare to the staining seen in the lobster tissue. Organ selection in sea lice was not an issue due to the ability to use whole body sections containing numerous organs. The primary interest was to determine if the intestinal tract expressed ABC transporters; other organs in the plane of section were also examined. All antibodies showed immunoreactivity in the intestinal tract of the sea lice, although the reaction gained using C219 was far weaker and less defined than that observed using JSB-1 or SL0525 (Fig. 4). No immunoreactivity was observed with any of the negative controls (omission of primary antibody shown in Figs. 4D, 5D, 6D).
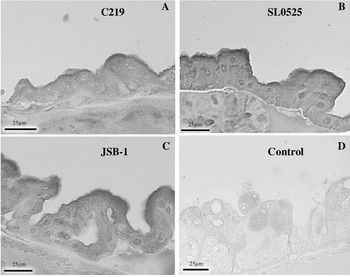
Fig. 4. Immunolocalization of ABC transporters in Lepeophtheirus salmonis intestine with (A) C219, (B) SL-0525, and (C) JSB-1. (D) Represents intestine where primary antibody was omitted from the protocol.
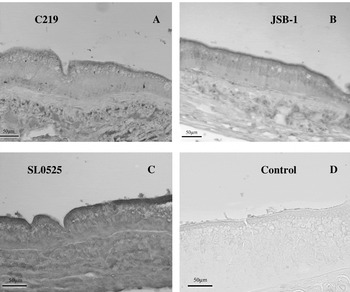
Fig. 5. Immunolocalization of ABC transporters in Homarus americanus intestinal tissue with (A) C219, (B) JSB-1, (C) SL0525. (D) Represents a control where primary antibody was omitted from the protocol.
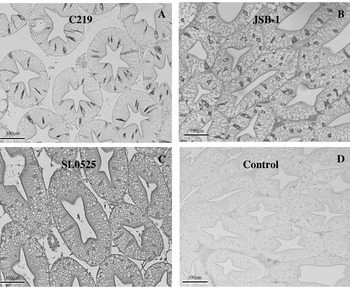
Fig. 6. Immunolocalization of ABC transporters in Homarus americanus hepatopancreatic tubules. (A) C219 reacts strongly with F cells and weakly with luminal epithelial surface, (B) JSB-1 reacts strongly in F cells and weakly with luminal epithelial surface, (C) SL0525 reacts weakly with F cells and strongly with luminal surface. (D) Represents a control where primary antibody was omitted from the protocol.
Immunolocalization of ABC transporters in lobster tissues
All antibodies showed specific staining of the luminal surface of the intestinal epithelium, although the staining intensities varied between antibodies. Both JSB-1 and SL0525 generated stronger immunostaining than C219 (Fig. 5). The hepatopancreas showed immunoreactivity for ABC transporters within the F-cells (identified on the basis of their morphological characteristics as discussed by Sousa et al. (Reference Sousa, Cuartas and Petriella2005)) and along the luminal surface of the tubules (Fig. 6). SL0525 showed a stronger staining of the luminal surface than either the C219 or JSB-1. F-cells were intensely stained with C219 and JSB-1 (Fig. 6A and B) and weakly stained with SL0525 (Fig. 6C).
Immunolocalization of ABC transporters in Atlantic salmon tissues
Immunohistochemical staining with C219 in the salmon liver was localized to the bile canaliculi (Fig. 7A). Neither JSB-1 nor SL0525 showed any staining of the canalicular surfaces of the hepatocytes. C219 was also the only antibody to generate a signal in some of the renal tubules (Fig. 7B). Both C219 and JSB-1 specifically stained the luminal surface of intestinal epithelia with C219 showing a far greater intensity of stain reaction than that generated by JSB-1 (compare Fig. 7C and D). The polyclonal antibody SL0525 again demonstrated no immunoreactivity in these salmon tissues. All tissues where primary antibody was omitted from the protocol showed no staining reaction.
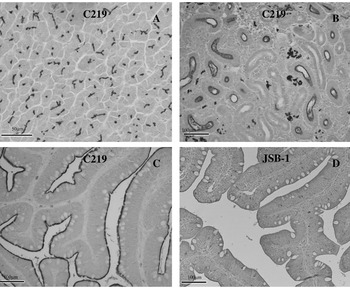
Fig. 7. Immunoreactivity of C219 and JSB-1 against P-gp in Salmo salar. (A) C219 reacts with the canalicular surfaces of the hepatocytes, (B) C219 reacts with the luminal surface of many renal tubules, (C) C219 reacts strongly with the surface of intestinal epithelial, (D) JSB-1 reacts weakly with the intestinal epithelium.
DISCUSSION
Identification and characterization of candidate resistance genes, as well as detection of ABC transporters using immunological methods, are important preliminary steps to investigate potential mechanisms of EMB resistance in sea lice. Alterations to P-gp and increased mRNA levels have been demonstrated in ivermectin-resistant H. contortus (Xu et al. Reference Xu, Molento, Blackhall, Ribeiro, Beech and Prichard1998) and selection at a P-gp allele following ivermectin treatment has been observed in H. contortus (Blackhall et al. Reference Blackhall, Liu, Xu, Prichard and Beech1998) and, more recently, O. volvulus (Ardelli et al. Reference Ardelli, Guerriero and Prichard2006a). Ardelli et al. (Reference Ardelli, Guerriero and Prichard2006b) further characterized the gene for a half-size ABC transporter whose expression was influenced by ivermectin, suggesting that ivermectin is selecting against several ABC transporter genes, not just the genes for P-gp.
This study reports the expression and efficient purification of a sea louse ABC protein fragment, using the GST-fusion protein expression system (Fikrig et al. Reference Fikrig, Barthold, Kantor and Flavell1990), which was then used to immunize a rabbit for the production of antiserum. This GST-fusion protein system has been applied widely in many fields of research. A major limitation of this system is the fact that many GST fusion proteins are partially or completely insoluble after lysis in non-ionic detergents (Frangioni and Neel, Reference Frangioni and Neel1993). During this study we used a method which utilizes the sodium salt of the alkyl anionic detergent N-laurylsarcosine previously demonstrated to effectively solubilize GST fusion proteins while still maintaining protein activity (Frangioni and Neel, Reference Frangioni and Neel1993). Because the GST handle was cleaved off the fusion protein using thrombin, the resulting polyclonal antibody was only directed against the SL0525 protein and not the GST portion of the fusion protein. This specificity was confirmed using Western blot analysis. The lack of cross-reactivity to GST also meant that its application in either Western blot analysis or immunohistochemistry would result in the specific binding to SL0525 epitopes without the uncertainty of cross-reactivity to GST proteins. This method represents an efficient method for the production of a protein-specific antibody and would be advantageous in immunological studies of invertebrate species where commercially available specific antibodies are very limited.
A distinctive characteristic of ABC transporters is the presence of a nucleotide binding domain containing several conserved regions; Walker A (GXXGXGK[S/T]), Walker B (XXXXD[E/D]), Signature (LSGGQ), glutamine loop and histidine loop (Qian et al. Reference Qian, Wei, Liu and Yang2006). The SL0525 sequence contains both Walker A/B and Signature motifs. In both loop regions of the SL0525 sequence, the conserved glutamine and histidine residues are substituted by glutamic acid. The significance of substituting glutamine or histidine with a negatively charged amino acid is difficult to predict, although, as these are regions within the NBD they would not affect substrate specificity. However, nucleotide binding could be affected. The identification of this gene and tissue localization of this protein is very significant for our investigation into potential multidrug transporters in L. salmonis. Additional research has investigated both the copy number and expression levels of this gene using quantitative RT-PCR (Tribble et al. Reference Tribble, Burka and Kibenge2007). Most importantly, that study showed a significant increase in SL0525 gene transcription in response to EMB exposure, thus implicating the role of this protein to EMB protection.
Western blot analysis
An ~160 kDa protein from sea lice was weakly immunoreactive to C219 and JSB-1 monoclonal antibodies, compared to expression of strong immunoreactivity in the rat liver controls. C219 and JSB-1 did not, however, stain proteins from salmon or lobster intestine. We are not certain whether this is due to the specificity of the monoclonal antibodies for the organs and species to which the antibodies were raised or due to the dilution of the ABC transporters in the intestinal and sea lice protein samples. Similar discrepancies have been seen when comparing polyclonal antibodies to nitric oxide synthase isoforms on different target tissues from various animals (Coers et al. Reference Coers, Timens, Kempinga, Klok and Moshage1998). Enrichment of the cell type may provide enhanced detection. The SL0525 polyclonal antibody detected a protein corresponding to the same size P-gp detected by both C219 and JSB-1. The SL0525 polyclonal antibody-specific protein determined from the Western blot results suggested that this gene has the molecular size expected for L. salmonis P-gp. However, the low homology with known P-gps and the inability of SL0525 to react with rat P-gp suggest that an ABC transporter, not necessarily P-gp was isolated. Characterization as ABC-F is not very likely since the predicted size of ABC-F proteins is only ~80 kDa. The expression pattern of some ABC-F proteins seems to be ubiquitous; they are probably not associated with membranes, having no transmembrane domain, and appear to be involved in control of translation in eukaryotes (Kerr, Reference Kerr2004). Further characterization is obviously necessary to confirm sequence assignment. We have recently isolated another putative ABC transporter gene, designated SL-Pgp1 (GenBank Accession no. EF093796), with ~73% similarity with P-gps of other species. This gene was also up-regulated in the presence of EMB (Tribble et al. Reference Tribble, Burka and Kibenge2007). Further characterization of this gene is in progress.
Tissue processing for immunohistochemistry
Bouin's and Dietrich's have been used as fixatives for the immunodetection of P-gp in both rainbow trout (Oncorhynchus mykiss) and sheepshead minnow (Cyprinodon variegates) (Hemmer et al. Reference Hemmer, Courtney and Benson1998; Sturm et al. Reference Sturm, Ziemann, Hirsch-Ernst and Segner2001). Using these fixatives in our laboratory did not generate a specific reaction for P-gp, which could be attributed to either the uniqueness of the specific species being used or the conditions used differing in some aspect. The effectiveness of formalin, a fixative which is commonly used in the clinical setting for the majority of mammalian P-gp studies was not evaluated by Hemmer et al. (Reference Hemmer, Courtney and Benson1998) or Sturm et al. (Reference Sturm, Ziemann, Hirsch-Ernst and Segner2001).
Numerous groups have successfully used frozen tissue sections for immunodetection of P-gp (Georges et al. Reference Georges, Bradley, Gariepy and Ling1990; Volk et al. Reference Volk, Potschka and Loscher2005); no previous studies have been conducted on crustaceans. The use of a variety of cryoprotection solutions were unable to maintain and preserve the sea louse tissue well enough to allow for cryosectioning.
Immunolocalization of ABC transporters
Both sea lice and lobsters showed many similarities in the staining pattern and signal intensity generated for each of the antibodies used. The localization of the staining on the epithelial lining of the intestinal tract of the sea lice was the primary interest of this study as the intestine is the initial absorption site for emamectin and thus a critical site for decreased drug absorption should ABC transporters, including P-gp, be over-expressed. Smith and Prichard (Reference Smith and Prichard2002) also localized P-gp mRNA primarily in the intestinal tract of H. contortus and suggested over-expression as a potential mechanism of ivermectin resistance. The staining pattern generated in sea lice using the SL0525 appears to mimic that seen with JSB-1. Western blot analysis using SL0525 has shown this antibody to detect the same size ~160 kDa protein(s) in sea lice membrane fractions as that detected by both C219 and JSB-1. These data thus add further to the evidence that this polyclonal antibody is generated against a sea louse ABC transporter. Further characterization of the SL0525 protein including obtaining its complete sequence is still required to confirm function.
The hepatopancreas in lobsters provides the functions which in mammals are provided separately by the liver and pancreas. The presence of an ABC transporter on the luminal surface, as suggested by the immunoreactivity observed, would function to eliminate various compounds from the organism (as in the liver and kidneys of the salmon). The F-cells of the hepatopancreas also showed strong immunoreactivity; however, the cytoplasmic distribution suggests that this is not an ABC transporter, but an alternative protein sharing a common epitope for antibody binding. These cells are believed to be involved in the synthesis and secretion of digestive enzymes (Al-Mohanna et al. Reference Al-Mohanna, Nott and Lane1985; Sousa et al. Reference Sousa, Cuartas and Petriella2005).
The salmon tissues showed expression of P-gp in all 3 tissues (liver, kidney, and intestine) investigated. The staining patterns generated by C219 compare well to those seen in other vertebrate species. C219 was the only antibody able to specifically detect P-gp in all 3 tissues with JSB-1 only generating a weak signal in the intestinal tissue. This suggests that P-gp in Atlantic salmon contains the highly conserved amino acid sequence VQEALD and/or VQAALD, whereas the epitopes recognized by JSB-1 and SL0525 are either not as well conserved or masked during histological processing. Based on our findings C219 should be chosen for any further immunohistological studies of P-gp expression in formalin fixed Atlantic salmon tissues. SLICE® is administered to salmon as an in-feed treatment. Once ingested EMB is readily absorbed in the gut and distributed to the skin, mucus and blood which are fed upon by the sea lice (Sevatdal et al. Reference Sevatdal, Magnusson, Ingebrigtsen, Haldorsen and Horsberg2005). The expression levels of P-gp and other ABC transporters at the host and parasite level may therefore have an impact on the efficacy of SLICE®.
This report represents the first documented evidence for the expression of a putative ABC transporter in the sea louse parasite, its Atlantic salmon host, and the American lobster. The expression of this protein in sea lice does not imply that they are resistant to EMB, but demonstrates that sea lice have one of the mechanisms present whose over-expression has been documented to be responsible for resistance development in other invertebrate species. The ability to analyse ABC transporter expression at both the host and parasite level allows treatment failure related to drug resistance to be investigated more comprehensively and possible treatment alternatives assessed.
We are grateful to Drs Neil Ross and Stewart Johnson, NRC Institute for Marine Biosciences, Halifax, NS, and Dr Frank Nilsen, Institute of Marine Research, Bergen, Norway, for access to their sea lice EST library databases. Appreciation goes to Nicole MacDonald and Monique Saleh for their excellent technical assistance. The project was supported by grants from AquaNet (Canadian Centre of Excellence in Aquaculture), NSERC, and Elanco Animal Health.