Introduction
Ultra-wideband (UWB) communication has become attractive since the US Federal Communications Commission released the 3.1–10.6 GHz frequency band for commercial UWB systems [1]. It has been applied in different areas, such as high data rate wireless communication in short-range technology, Wireless Body Area Network (WBAN), radar, and tracking [Reference Tansarn and Kaewprapha2]. As a critical part of the UWB system, both the UWB antenna and UWB filter attract more attention owing to their advanced characteristics, such as lightweight, easy integration, and low cost.
Since the UWB system has a wide operating frequency band (3.1–10.6 GHz), it is easily interfered by narrowband signals in the band and other wireless signals near the band-edge. The unwanted in-band or out-of-band signals will affect the performance of the UWB receiver. Therefore, in order to deal with some out-of-band interference signals, band-pass filters are usually connected in series behind the antenna to improve the band-edge frequency selectivity and suppress out-of-band interference. However, the combination of the antenna and filter in series will increase the size and cost of the RF front-end. Therefore, it is of interest to design a UWB antenna with filtering function to realize miniaturization and reduce the cost of the RF front-end. As we all know, most of the antennas and filters are designed separately, and most UWB antennas design focus on the new structure [Reference Liu, Wang and Qin3, Reference Tang, Ziolkowski and Xiao4], minimization technology, band-notched function [Reference Zehforoosh, Mohammadifar, Mohammadifar and Ebadzadeh5, Reference Zhang, Cao, Huang, Alrawashdeh and Zhu6], and so on. For interference suppression, most of the UWB antennas are designed with the notch function in-band, and little attention is paid to the suppression effect of the out-of-band interference signals. Badjian et al. presented a UWB patch antenna with integrated bandpass filter [Reference Badjian, Chakrabarty, Hock and Devkumar7]. Although the frequency selection characteristics of the antenna's upper and lower sidebands have been improved by the addition of the filter, its shape factor (the ratio of the −3 dB bandwidth to the −10 dB bandwidth) is 1.21 which is still not very good. In [Reference Li and Gao8] and [Reference Sahoo, Gupta and Parihar9], the radiating patch is electromagnetically coupled to a UWB filter to achieve good frequency selectivity. The shape factor and the roll-off of the two reference antennas have been significantly improved, but the size of the antenna is 53 mm × 22 mm [Reference Li and Gao8] and 53 mm × 42 mm [Reference Sahoo, Gupta and Parihar9], which is still relatively large. In the design that we have conducted previously [Reference Yang, Xi, Zhao and Shi10], a compact slot antenna with good out-of-band suppression has also been investigated. By using the stepped impedance resonator with low-pass characteristics as a feeding line and setting an additional open slot near the stepped slot, both the upper and lower band-edge selectivity of the antenna are enhanced. The size of the proposed antenna is 24 mm × 12 mm, which is very compact. However, UWB antennas are also necessary for the rejection of unwanted narrowband interference signals, such as wireless local area network systems (WLAN) that operate with 5.15–5.35 and 5.725–5.825 GHz,and Worldwide interoperability for Microwave access (WiMAX) with the frequency of 3.3–3.7 GHz. Therefore, UWB antennas with band-notched functions to reject the unwanted narrowband interference signals are required.
As we all known, the notch function is usually achieved by cutting slot on the radiating patch [Reference Chang and Kang11–Reference Ali, Ibrahim and Machac14] or adding parasitic structure near the feeding line [Reference Guo, Tang, Xu and Ai15–Reference Sung17] or the ground plane [Reference Peng, Wang, Zhao and Liu18, Reference Shaik, Saha, Siddiqui and Antar19]. However, the notch band achieved by this method is invariant; the antenna has poor adaptability and can only be used for a specific communication system. Most recently, reconfigurable antennas have also been proposed to avoid the frequency interference [Reference Wu, Li, Wu, Shi and Cui20–Reference Yang, Xi, Hou, Shi and Yuan22]. With the reconfigurable structures providing switchable band-notches, the antenna can be operated throughout the UWB spectrum whenever there is no coexisting system while filtering out interferences when necessary. In [Reference Badamchi, Nourinia, Ghobadi and Shahmirzadi21], a compact UWB slot antenna with switchable single-/dual-band notch functions is studied. By cutting slots on the radiating patch and inserting PIN diodes between the slots, four states are obtained. In [Reference Yang, Xi, Hou, Shi and Yuan22], five p-i-n diodes are employed to bridge or open the slots, which allow the antenna to be configured into three different structures functioning as a UWB antenna, or an antenna with notched frequencies at WLAN or WiMAX band. Recently, Wu et al. proposed a band-notched UWB antenna with switchable and tunable performance. In this design, the PIN diodes are used to switch notched frequency bands, while varactors could alter the central frequency of each notch band continuously [Reference Wu, Li, Wu, Shi and Cui20].
In [Reference Srivastava, Mohan and Chakrabarty23] a reconfigurable UWB slot antenna for cognitive radio applications is designed. There are four switchable states in communicating that can be realized by using five PIN diodes. There are 2.8–10.7 GHz (UWB mode), 3.2–4.5, 4.3–7.8 and 7.9–11.2 GHz. Inspired by the document [Reference Srivastava, Mohan and Chakrabarty23], a simple reconfigurable UWB slot antenna with filtering function in-band and out-of-band is proposed. The basic structure of the proposed antenna is a filtering antenna with good band-edge selectivity using stepped impedance resonator feeding line. The reconfigurability is achieved by using two microstrip lines paralleling to the feeding line and two PIN diodes. Only two PIN diodes are applied to bridge or open the microstrip lines, which allow the slot antenna to be configured into four different states as an UWB antenna, or an UWB antenna with notched frequencies at WiMAX or WLAN or WiMAX and WLAN. The entire size is as compact as 24 mm × 12 mm, which is smaller than most of the proposed UWB antennas [Reference Liu, Wang and Qin3–Reference Sahoo, Gupta and Parihar9, Reference Chang and Kang11–Reference Srivastava, Mohan and Chakrabarty23]. As a part of the reconfigurable antenna, the bias circuit should be considered when designing reconfigurable antenna. This is because the bias circuit needs to be connected to the reconfigurable structure to control the switch on and off of the diode. In order to avoid the effect of DC on the radiation structure, DC blocker is usually required. Therefore, the antennas are easily affected by the antenna bias circuit and lumped element. Here, compared with our earlier work proposed in [Reference Yang, Xi, Zhao and Shi10], the proposed antenna has advantages in frequency selection for different interference environment. With the reconfigurable structures providing switchable band-notches, the antenna can be operated throughout the UWB spectrum whenever there is no coexisting system while filtering out interferences when necessary. Compared with the referenced antenna [Reference Wu, Li, Wu, Shi and Cui20–Reference Yang, Xi, Hou, Shi and Yuan22], the reconfigurable structure and the bias circuit of the proposed antenna are simple, which have no adverse effect on the size and performance of the antenna. In addition, the proposed antenna has also some other advantages in size, filtering function in-band and out-of-band, and tunable states for multiple UWB applications. To verify the performance of the final design, multiple measured and simulated results in frequency and domain are studied and analyzed. The outcomes of the experiment indicate that the proposed antenna has more choices in the face of different interference environments. The measured results of the time domain and frequency domain meet the designed requirements, which verify the model as a good candidate for UWB applications.
Antenna design
The presented filtering antenna is designed on an RT/Duroid 5880 substrate with the relative dielectric constant of 2.2 and thickness of 0.787 mm. The dimension of the proposed antenna is 24 mm × 12 mm. Figure 1 shows the geometry of proposed design. The proposed design is based on the reference antenna proposed in [Reference Yang, Xi, Zhao and Shi10]. Good out-of-band suppression and roll-off rate are obtained by using a stepped impedance resonator feeding line with low-pass characteristic and a narrow slot near the stepped slot. For the obtaining of the notch and reconfigurable function of the antenna, a pair of microstrip lines parallel to the feeding line is added to the end of the ladder slot. The current flows from the microstrip line through the switch to the ground. The notch frequency is related to the length of the current path (L s), as shown in Fig. 2. Therefore, the notched frequency of the antenna can be changed by adjusting the position of the PIN diodes. The adjustable range of notch frequency is 3.3–5.5 GHz, which is applicable to both WiMAX and WLAN bands. The longer the current path, the lower the notch frequency. The whole structure and the bias circuit are simple and not connected with the radiation structure, so the reconfigurable structure has little negative effect on the radiation characteristics of the antenna.

Fig. 1. The structure of the fabricated reconfigurable UWB antenna and bias circuit of the PIN diodes equivalent circuit.

Fig. 2. The reflection coefficients of the proposed antenna with different current path L s.
Forward biasing the diode through a DC forward voltage (VF) of 1 V can be seen as an equivalent circuit of a series combination of inductance (L = 0.15 nH) and resistance (R s = 4.7 Ω), and when the PIN diode is off, there is one resistance of 5 kΩ parallel with a capacitance of 0.017 pF as shown in Fig. 1. An RF choke (L) of 0.15 nH in series with the diode efficiently implements RF isolation in the circuit. The series resistor is used to divide the voltage. By the use of PIN diodes instead of the switch connected with parallel microstrip line and the ground, the reconfigurable function of the antenna can be achieved by controlling PIN diodes. Four states of the proposed antenna are shown in Table 1. Simulated reflection coefficients of the four states are shown in Fig. 3. To better understand the effects of the slots and resonators behind the band-notched characteristics, the simulated surface current distributions on the presented antenna at four states are presented in Fig. 3. It is found that when the proposed antenna is working in State-I, the low-frequency current is mainly distributed at the end of the stepped slot and the narrow slot while the high-frequency current is mainly distributed at the opening edge of the stepped slot. The results of the current distribution indicate that the end of the stepped slot and the narrow slot has a more significant influence on the low frequency, and the opening stepped slot has a greater impact on the high frequency. As can be seen from Fig. 3, the position of the diode and the on-off play a decisive role in the notched bands.

Fig. 3. The reflection coefficients and the surface current distributions of four states, (a) State-I, (b) State-II, (c) State-III, (d) State-IV.
Table 1. Four configurations of the proposed antenna

The antenna is simulated and optimized using CST MICROWAVE STUDIO targeting the best UWB performance and notched function. The dimensions of the optimal antenna are manufactured and listed in Table 2.
Table 2. Dimensions of the proposed antenna

Results and discussions
The proposed antenna is manufactured and tested. In this section, the numerical and experimental results of the proposed reconfigurable antenna are presented and discussed, including its frequency-domain and time-domain analysis.
Frequency-domain analysis
Figure 4 shows the simulated and measured reflection coefficient of the four states of the proposed antenna. As is shown in Fig. 4(a), the measured bandwidth for the reflection coefficient is approximately 114% ranging from 3.1 to 11 GHz. The proposed antenna has excellent out-of-band suppression since no other resonance frequency exists outside the desired band. The ratio of the −3 dB bandwidth to the −10 dB bandwidth is 1.038, which is very close to the ideal shape factor. Figures 4(b)–4(d) demonstrate the measured and simulated reflection coefficient with the notched bands. By controlling the PIN diodes, the proposed antenna can achieve notch function in the WiMAX or WLAN as well as the notched band in the WiMAX and WLAN. It shows that the measured results matched well with the simulated results.

Fig. 4. Measured and simulated reflection coefficients for the four states of the proposed antenna, (a) State-I, (b) State-II, (c) State-III, (d) State-IV.
The simulated and measured gain and efficiency curves in four states are shown in Fig. 5. The excellent filtering characteristics in-band and out-of-band can also be observed from the gain and efficiency curves. It is observed that the proposed antenna with four states has steady gains (3.5 dBi) and efficiencies (80%) in operating frequency of 3.1–10.6 GHz, but sharply decreases at the band-edge and notched bands and has an excellent roll-off rate in band-edge.

Fig. 5. Measured and simulated gain and efficiency of the four states of the proposed antenna, (a) State-I, (b) State-II, (c) State-III, (d) State-IV.
For a further analysis of the filtering characteristics of the antenna in-band and out-of-band, the simulated input impedance of the proposed antenna in State-IV with band notch frequency at 3.5 and 5.5 GHz are given in Fig. 6. As shown in Fig. 6, in the passband, the real part of the antenna is close to 50 Ω, and imaginary curves are nearly 0 Ω. It is found that the amplitude of the real part and the imaginary part in the notch frequency (3.5 and 5.5 GHz) and the band-edge (3 and 11 GHz) have obvious changes. The variation of the impedance of the antenna in the band-edge and notch frequencies further illustrate the better suppression of out-of-band and in-band interference signals.

Fig. 6. Simulated input impedances of the proposed antenna with band-notches in 3.5 and 5.5 GHz.
The radiation properties of the proposed antenna are measured by the use of anechoic chamber as shown in Fig. 7. Figure 7 plots the measured and simulated radiation patterns of the proposed antenna in State-I mode at 3.1, 6, and 10 GHz, respectively. It is found that the measured results are in good agreement with the simulated results and exhibit quasi-omnidirectional radiation pattern in operating frequencies. Again, the radiation patterns in E-plane at 10 GHz become imbalanced, which is mainly caused by the imbalance of current distribution at higher frequencies and instability of dielectric constant at high frequency. The experimental results of the reflection coefficient, gain, and efficiency show that the proposed antenna has good filtering characteristics in-band and out-of-band.

Fig. 7. Measured and simulated radiation patterns (a) 3.1 GHz, (b) 6 GHz, (c) 10 GHz.
Time-domain analysis
In the application of UWB, the performance cannot be judged solely from the results of bandwidth and pattern. Having a good time-domain performance in the operating frequencies is also very important for the UWB antenna. For the examination of the time-domain characteristics of the proposed antenna, two identical antennas are used as transmitter and receiver, which are placed in face-to-face with a distance of 50 cm. In order to avoid repeated discussion, we chose State-IV which has better filtering characteristics in-band and out-of-band as the object of discussion.
Figure 8 shows the simulated group delay of two State-IV antennas with PIN diodes D1 and D2 on with a 50 cm distance. As can be seen here, the group delay in the operating frequencies (except for the notched bands) is close to 1.5 ns with small (within ±0.3 ns) variations. Maximum result of group delay is about 4 ns at the band-edge and the notched band. This confirms that the proposed antenna has a good linearity in the operating frequencies and filtering performance of the interference signals in-band and out-of-band.
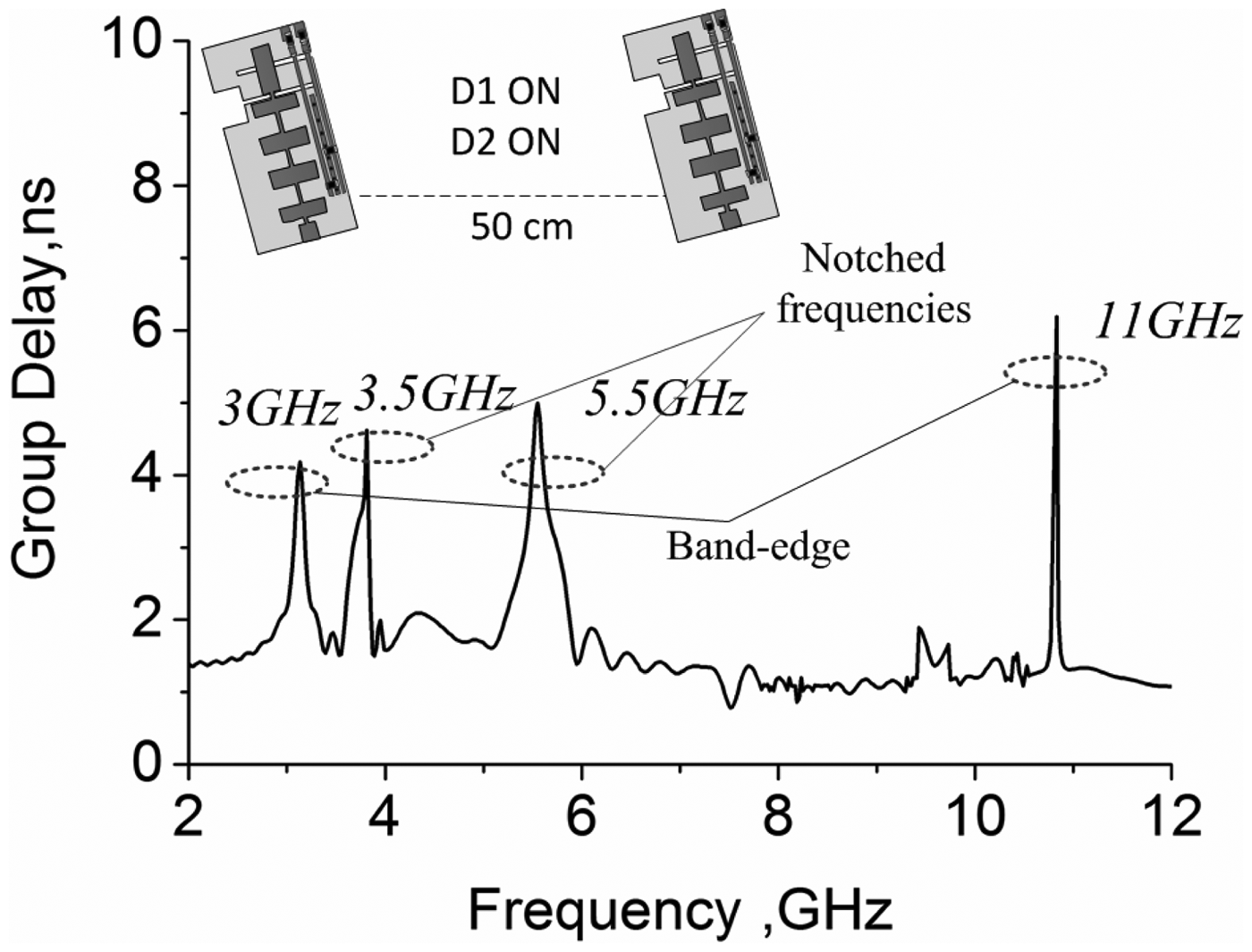
Fig. 8. Group delay of antenna in State-IV.
Figure 9 shows the magnitude and phase characteristics of two identical antennas placed face-to-face. It is observed in Fig. 9(a) that the magnitude characteristic of the antenna in operating frequencies is stable, and the fluctuation is small in the working frequency band. It is also noticed that the magnitude changes sharply in the notched band and band-edge. Phase S 21 for two same State-IV antennas placed face-to-face are also shown in Fig. 9(b). It is found that the plot exhibits a linear variation of phase in the operating band except for notched bands.

Fig. 9. Measured magnitude and phase S 21 with a pair of identical antennas in State-IV, (a) magnitude, (b) phase S 21.
In Fig. 10 the input and received signals are shown. To reduce the signal distortion caused by signal bandwidth and impedance mismatch, the short pulse is used to excite the UWB antenna, which is a fifth-order derivative of the Gaussian pulse and is given by [Reference Yang, Xi, Hou, Shi and Yuan22, Reference Pozar24]

where, C is the amplitude parameter spectral density suggested by FCC and σ = 51 ps to ensure that the shape of the spectrum complies with the FCC mask. The time-domain diagram of the pulse signal is shown in Fig. 10. All the excitation signals used in the time-domain analysis of UWB antennas are this short pulse.

Fig. 10. Time-domain characteristics of UWB antenna in two configurations, (a) face-to-face, (b) side-to-side.
For a better analysis of the time-domain characteristics of the antenna, two states (State-I and State-IV) of the proposed antenna are studied in two cases, which are face-to-face, side-to-side. The received signals of the two states antenna in different cases are shown in Fig. 10. It can be seen that the UWB antenna with the notched function will introduce ringing distortion in both the cases face-to-face and side-to-side display, but it is not the same case in the UWB antenna without notched band. This is mainly due to the impedance mismatch of the antenna in the notch band. In addition, the antenna received signal amplitude and waveform in the side-to-side and face-to-face are relatively close. It can be seen that the antenna performs well in the time domain and has the better adaptability to meet the application of UWB.
To better evaluate the time-domain characteristics of the UWB antenna, the correlation coefficients ρ is given as follow,

where τ is a delay which is varied to make numerator in (2) maximum. S 2(t) is the received pulse, S 1(t) is the input pulse. Using (2), the fidelity factor of two states (State-I and State-IV) is calculated when the antennas were placed in face-to-face and side-to-side condition. The calculated correlation factors of State-I and State-IV are compared in Table 3. It can be observed that both the face-to-face and side-to-side received signals of the two states are very similar to the input signal, and the correlation factor of the UWB antenna is bigger than the UWB antenna with the notched band. It is because the impedance mismatching at the notched band will introduce ringing distortion.
Table 3. The calculated correlation factors of two configurations in face-to-face and side-to-side

Conclusion
In this paper, a compact design of reconfigurable filtering UWB-slot antenna with switchable band-notched function has been proposed. The basic structure of the proposed antenna is a stepped slot antenna which has good band-edge selectivity and good out-of-band suppression. The reconfigurability is achieved by using two microstrip lines paralleling to the feeding line and two PIN diodes. There are four states which can be achieved by controlling the PIN diodes on and off. To verify the performance of the final design, multiple measured and simulated results in frequency and time domain are studied and analyzed. The measured results agreed very well with simulation. Compared with other reconfigurable UWB antenna, the proposed design is simple and convenient to implement. Additionally, the proposed antenna has also other attractive features such as good band-edge selectivity, compact size, independent reconfigurable structure which do not connect with the radiation structure, and has little effect on the radiation performance of the proposed design. The outcomes of the experiment indicate that the proposed antenna to be a good candidate for UWB applications.
Acknowledgement
This work was supported in part by the Doctoral Innovation Fund of Xi'an University of Technology (310-252071805), Key research and development plan of Shaanxi Province (2017ZDXM-GY-117), and Xi'an Science and Technology Plan Project (2017080CG/RC043(XALG013)), (2017080CG/RC043(XALG014)), (2017080CG/RC043(XALG032)).
Hailong Yang received the B.S. in Communicating Engineering from Heze University, Heze, China, in 2012. M.S degrees in Communicating Engineering from Xi'an University of Technology, Xi'an, China, in 2015. He is currently working toward the Ph.D. degree at Xi'an University of Technology. His recent research interests include ultra-wideband antennas, reconfigurable antennas, and ultra-wideband filters.
Xiaoli Xi (M'2010) received the B.S degrees in Applied Physics from the University of Defense Technology, Changsha, China, in 1990. M.S degrees in Biomedical Engineering from Fourth Military Medical University, Xi'an, China, in 1998, and a Ph.D. degree in Electrical Engineering from Xi'an Jiaotong University, Xi'an, China, in 2004. She is currently a Professor at the Department of Electric Engineering, Xi'an University of Technology, Xi'an, China. Her recent research interests include wave propagation, antenna design, and communication signal processing.
Lili Wang received the B.S. degree in the Electromagnetic Field and Microwave Technology from Beijing University of post and telecommunications, Beijing, China, in 1990. M.S degrees in Electrical Engineering at Xi'an University of Technology, Xi'an, China, in 2004. He is now an Adjunct Professor at the Department of Electric Engineering, Xi'an University of Technology. Her recent research interests include wave propagation and antenna design.
Yuchen Zhao received the B.S., M.S., and Ph.D. degrees in Electronic Science and Technology from Northwestern Polytechnical University, Xi'an, China, in 2007, 2010, and 2014, respectively. He joined the Faculty of Electronic Engineering Department, Xi'an University of Technology, Xi'an, China, in 2014. His research interests include wave propagation and effective medium theories.
XiaoMin Shi received the B.S., M.S., and Ph.D. degrees from Xi'an University of Technology, Xi'an, China, in 2010, 2013, and 2017, respectively. She joined the Communication Engineering Department, Xi'an Shiyou University, Xi'an, in 2017. Her research interests include the analysis and design of microwave filters and RF passive circuits.
Yanning Yuan received the B.S. in Electronic Information from Liaoning Shihua University, Fushun, China, in 2004, M.S. degrees in Communicating Engineering from Xi'an University of Technology, Xi'an, China, in 2007. She joined the Shaanxi Lingyun Science and Technology Co., Ltd. and served as a project manager subsequently. She is currently a full-time research worker at the Xi'an University of Technology. Her research interests include ultra-wideband antennas, multi-frequency antenna, and RF circuit.