Introduction
Minerals of the tourmaline supergroup are common accessory phases occurring in numerous igneous, metamorphic and sedimentary rocks. They are complex borosilicates with compositions corresponding to the generalized chemical formula XY 3Z 6(T 6O18)(BO3)3V 3W (Henry et al., Reference Henry, Novák, Hawthorne, Ertl, Dutrow, Uher and Pezzotta2011), where X, Y, Z, T, B, V (=O3) and W (=O1) denote structural sites occupied as follows.
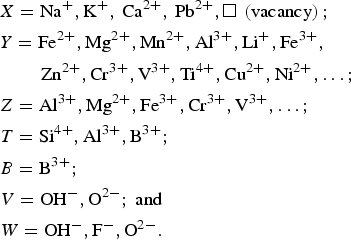
The extremely complex chemical composition results in numerous colour varieties, sometimes showing gem-quality features, although the most common Fe-bearing crystals are usually dark-brown to black.
In Poland, occurrences of tourmaline-supergroup minerals are generally limited to metamorphic and igneous rocks of the Sudetes region and crystalline rocks of the Tatra Mountains (Pieczka, Reference Pieczka1996; Gawęda et al., Reference Gawęda, Pieczka and Kraczka2002). Tourmalines in those areas are mainly very dark green to black Fe-bearing species, mainly schorl. The Góry Sowie Block is the only geological unit in Poland known for occurrences of multicoloured Li-bearing tourmalines, where they may be found in LCT-type pegmatites, e.g. in the vicinity of Gilów (Pieczka et al., Reference Pieczka, Łobos and Sachanbiński2004) and Piława Górna (Pieczka et al., Reference Pieczka, Szełęg, Łodziński, Szuszkiewicz, Nejbert, Turniak and Ilnicki2010). Additionally, a dark blue dravite was described from a small lens of a LCT-type pegmatite hosted by serpentinite of the Szklary massif (Pieczka, Reference Pieczka2007), considered to be an external representative of the Góry Sowie pegmatitic melts intruded into ultrabasic rocks of the Sudetic ophiolite before ~380 Ma (Pieczka et al., Reference Pieczka, Szuszkiewicz, Szełęg, Janeczek and Nejbert2015).
In addition to the Góry Sowie area, Wołowa Mountain in the eastern part of Karkonosze Mountains is the only locality in the Polish part of the Sudetes with occurrences of schorlitic to dravitic tourmalines, varying in colour from black through olive-green to yellow (Lis et al., Reference Lis, Stępniewski and Sylwestrzak1965). Pieczka (Reference Pieczka1996) gives brief details of Fe-bearing tourmalines from the locality; in the present paper we describe the yellowish dravitic tourmaline in term of its crystal chemistry and structure. Oxy-dravite tourmaline, the end-member species usually dominant in the dravitic tourmaline studied, was mentioned in the tourmaline classification proposal of Hawthorne and Henry (Reference Hawthorne and Henry1999). Subsequently the term was used in the classification of Mg- and Al-bearing tourmalines with the O2–-dominant W site, and recently it was approved by the International Mineralogical Association Commission on New Minerals, Nomenclature and Classification as a valid mineral of the tourmaline supergroup (Bosi and Skogby, Reference Bosi and Skogby2012, Reference Bosi and Skogby2013). Occasionally it occurs in rocks of Cr- and V-rich environments. Such tourmaline species can be enriched in V and Cr, and sometimes also in Fe3+. These elements can even be the dominant occupants at the Y site and/or Z site, e.g. in oxy-vanadium-dravite (Bosi et al., Reference Bosi, Reznitskii and Sklyarov2013a), originally described as ‘vanadium-dravite’ by Reznitsky et al. (Reference Reznitsky, Sklyarov, Ushapovskaya, Nartova, Kashaev, Karmanov, Kanakin, Smolin and Nekrasova2001), oxy-chromium-dravite, vanadio-oxy-dravite, vanadio-oxy-chromium-dravite, chromo-alumino-povondraite (Baksheev et al., Reference Baksheev, Prokofev, Yapaskurt, Vigasina, Zorina and Solovev2011; Bosi et al., Reference Bosi, Reznitskii and Skogby2012; Reference Bosi, Skogby, Hålenius and Reznitskii2013b; Reference Bosi, Reznitskii, Skogby and Hallenius2014a,Reference Bosi, Skogby, Reznitskii and Halleniusb; Reference Bosi, Reznitskii, Hålenius and Skogby2017; Reznitskij et al. Reference Reznitskii, Clark, Hawthorne, Grice, Skogby, Hålenius and Bosi2014) and bosiite (Ertl et al., Reference Ertl, Baksheev, Giester, Lengauer, Prokofiev and Zorina2016). The Wołowa Mountain tourmaline, however, features a relatively simple chemistry with negligible V and Cr, and probably absent Fe3+, which reflects the chemistry of the environment in which the tourmaline grew.
Occurrence
The Karkonosze granite massif is the largest Variscan pluton in the Western Sudetes at the northeastern margin of the Bohemian Massif, hosted by the Izera Metamorphic Complex on the northwest, and the South Karkonosze Metamorphic Unit, the East Karkonosze Metamorphic Unit and the Kaczawa Metamorphic Complex on the northeast (Fig. 1). The East Karkonosze Metamorphic Unit comprises the Kowary–Czarnów Unit divided into the Kowary gneiss series and the Czarnów schist series and the Leszczyniec Unit (Mazur et al., Reference Mazur, Aleksandrowski, Turniak and Awdankiewicz2007). The Kowary gneiss series, which contacts the granite, is composed of gneisses, mica-schists, amphibolites, phyllites and marbles (ibid.). The region of Wołowa Góra Mountain located ~3 km SW from Kowary, marks the NE termination of the Karkonosze montane range and is the SW part of the East Karkonosze Metamorphic Unit in Poland. The region is composed mainly of leucocratic augen gneisses associated with granitic gneisses and subordinate layered gneisses of the Kowary gneiss series, surrounded on the south and west by an assemblage of chlorite-mica schists, quartzo-feldspathic schists and amphibolites, formed from differentiated igneous and volcanogenic–sedimentary ~500 Ma protoliths (Berg, Reference Berg1923; Lis et al., Reference Lis, Stępniewski and Sylwestrzak1965; Różański, Reference Różański1995; Oberc-Dziedzic et al., Reference Oberc-Dziedzic, Kryza, Mochnacki and Larionov2010). A pass between Wołowa Mountain and Czoło Mountain in the main Karkonosze range is the well-known locality of a milky quartz vein with brannerite, associated with tourmalines showing colours varying from black, found in the host granitic gneisses, through olive-green to yellow in the quartz vein itself (Lis et al., Reference Lis, Stępniewski and Sylwestrzak1965). The surrounding granitic gneisses are medium- to coarsely-crystalline, light-pinkish rocks composed of microcline, microcline perthite, quartz and pale-coloured mica, with strong albitization, which almost completely altered the primary K-feldspars, leading commonly to the formation of chessboard-twinned albite. The contact between the host granitic gneisses and the quartz vein is some metres long and 5–50 cm thick emphasized by the presence of altered dark micas and dark green tourmaline. The tourmaline occurs as radial aggregates, a few centimetres thick, composed of elongated, prismatic crystals (Lis et al., Reference Lis, Stępniewski and Sylwestrzak1965; Pieczka, Reference Pieczka1996), which become pale green inside the vein, reaching a yellowish coloration in its axial part. Brannerite, gersdorffite, pale-coloured micas, an apatite-group mineral, pyrite, pharmacosiderite and Fe oxides were recognized as the associated minerals (Lis et al., Reference Lis, Stępniewski and Sylwestrzak1965).

Fig. 1. (a) Geological sketch of the East Karkonosze Metamorphic Unit (after Pieczka et al., Reference Pieczka, Gołębiowska and Parafiniuk2009). (b) Detailed geological map of the Budniki–Wołowa Mountain region (after Różański, Reference Różański1995; fide Mochnacka et al., Reference Mochnacka, Oberc-Dziedzic, Mayer and Pieczka2008) shown as a black dotted frame in (a). The occurrence of the quartz vein with dravitic tourmaline is marked by a grey star.
Methods
Electron microprobe analysis (EMPA)
Electron microprobe analyses were performed at the Inter-Institute Analytical Complex for Minerals and Synthetic Substances at the University of Warsaw on a longitudinal section of an aggregate composed of yellowish tourmaline crystals, using a Cameca SX 100 electron microprobe operating in wavelength-dispersive mode under the following conditions: accelerating voltage of 15 kV, beam current of 20 nA, beam diameter of 2 µm, peak count-time of 20 s and background time of 10 s. Standards, diffracting crystals, analytical lines, and mean detection limits (in wt.%) were as follows: phlogophite – F (TAP, Kα, 0.17), albite – Na (TAP, Kα, 0.02), diopside – Mg (TAP, Kα, 0.02), Si (TAP, Kα, 0.02) and Ca (PET, Kα, 0.02), orthoclase – Al (TAP, Kα, 0.02) and K (PET, Kα, 0.02), rutile – Ti (PET, Kα, 0.02), rhodonite – Mn (LIF, Kα, 0.06), hematite – Fe (LIF, Kα, 0.06), V2O5 – V (LIF, Kα, 0.03), Cr2O3 – Cr (LPET, Kα, 0.04) and sphalerite – Zn (LIF, Kα, 0.09). The raw data were reduced with the ‘PAP’ routine of Pouchou and Pichoir (Reference Pouchou, Pichoir and Armstrong1985).
Atomic fractions for crystallochemical formulae were normalized on the basis of: (1) 15 Y + Z+T atoms per formula unit (apfu) or (2) Y + Z = 9 apfu for cases where large amounts of SiO2 where present, probably due to tiny inclusions of quartz on the micro- to nano-scale, with the assumed maximum Si content = 6 apfu. B2O3 was calculated assuming B = 3 apfu, and H2O, occurring as OH groups, by stoichiometry based on electroneutrality of the formulae. Table 1 gives statistics for the compositional data for the three distinguished tourmaline varieties: (1) primary tourmaline of the first generation; (2) secondary tourmaline from transverse veinlets (generation 2a); and (3) secondary tourmaline from crystals terminations in the fissures filled with quartz (generation 2b). Representative compositions of the tourmalines are given in Table 2.
Table 1. Statistics for compositions of dravitic tourmalines from Wołowa Góra Mountain.

*SiO2 for the tourmaline is assumed by stoichiometry to be equal to 6 apfu (see text).
(calc.) – calculated by stoichiometry (see text); n – number of analyses; SD – standard deviation.
Mn, Cr and Zn were below detection limit; bdl – content below detection limit.
Table 2. Representative compositions of dravitic tourmalines from Wołowa Góra Mountain.

Notes: Mn, Cr and Zn are below detection limits; bdl – content below detection limit; n – number of analyses.
Crystal-structure refinement
The crystal structure of yellowish Mg-rich tourmaline was refined at the Institut für Mineralogie und Kristallographie, Geozentrum, Universität Wien, Austria. Firstly, the quality of different tourmaline crystals was checked with a Bruker APEXII diffractometer equipped with a CCD area detector and an Incoatec Microfocus Source IμS (30 W, multilayer mirror and MoKα). The crystal with the best quality was subsequently measured on this diffractometer. Single-crystal X-ray diffraction data, up to 80°2θ, were collected at room temperature, integrated and corrected for Lorentz and polarization factors with an absorption correction by evaluation of partial multiscans. The structure was refined with SHELXL97 (Sheldrick, Reference Sheldrick2008) using scattering factors for neutral atoms and a tourmaline starting model from Ertl et al. (Reference Ertl, Marschall, Giester, Henry, Schertl, Ntaflos, Luvizotto, Nasdala and Tillmanns2010) (sample R6b). Refinement was performed with anisotropic displacement parameters for all non-hydrogen atoms. Crystal data and details of the structure refinement are provided in Table 3. The various site occupancies were refined according to well-known characteristics of the tourmaline structure, and with guidance from the EMP analysis; this strategy appears sound with the resulting empirical formula being compatible with that determined from the EMP results. Magnesium and Fe were refined at the Y site, Si was fixed at the T site. The X site was refined by setting the Ca occupancy to 0.12 apfu allowing the remainder of the site to refine as Na = (0.88 – □) apfu. The occupancy of the Z site was fixed at (Al893Mg0.107); the ZMg content was estimated due to the enlarged <Z–O> distance. The occupancy of the O1 site was fixed at (O0.79F0.21), based on chemical data. The occupancy of the H1 and H3 sites were fixed at 0.33 and 1.00, respectively, based on chemical data. The positions of the H atoms bonded to the oxygen at the O1 and O3 positions in the structure were taken from the difference-Fourier map and incorporated into the refinement model; the O1–H1 and O3–H3 bond lengths were constrained to be 0.97(3) Å. The refinement converged at a R 1(F) value of 1.85% (Table 3). In Table 4, the atomic parameters and equivalent isotropic displacement parameters are listed, and in Table 5 selected interatomic distances in the refined tourmaline are presented. The crystallographic information file has been deposited with the Principal Editor of Mineralogical Magazine and is available as Supplementary material (see below).
Table 3. Crystallographical data and refinement details for oxy-dravite from Wołowa Góra Mountain.

Note: multi-scan absorption correction; refinement on F 2. Frame width, scan time, detector distance: 3°, 240 s, 35 mm. Scan mode: sets of ϖ and θ scans.
* R 1 = Σ||F o| – |F c| |/Σ|F o|
† wR 2 = {Σ[w(F o2 – F c2)2]/Σ[w(F o2)2]}1/2
w = 1/[σ2(F o2) + (aP)2 + bP], P = [2F c2 + Max(F o2,0)]/3
‡ R int = Σ|F o2 – F o2(mean)|/Σ[F o2]
§ GooF = S = {Σ[w(F o2 – F c2)2]/(n–p)}1/2
Table 4. Atom parameters in oxy-dravite from Wołowa Góra Mountain.

Note: Definition for U eq – see Fischer and Tillmanns (Reference Fischer and Tillmanns1988).
*Isotropic displacement parameters (U iso) for H1 and H3 constrained to have a U iso 1.2 times the U eq value of the O1 and O3 oxygen atoms.
Table 5. Selected interatomic distances in oxy-dravite from Wołowa Góra Mountain.

Results and discussion
Compositional data
The sample of yellowish tourmaline was collected in the year 2000 from remnants of the quartz vein cropping out on the pass between Wołowa Mountain and Czoło Mountain in the main Karkonosze range. The prismatic, yellowish crystals reach 1.5–2.0 cm in length and 2–3 mm in diameter; they are colourless in thin section, with birefringence Δ ≈ 0.015–0.017 (Fig. 2). In places, the crystals are cross-cut by fissures filled with a secondary tourmaline or the host quartz. The crystal terminations in the fissures on the border with quartz are distinctly darker in hand specimens, yellowish-olive-brown, with Δ ≈ 0.023–0.025 (Fig. 3).

Fig. 2. A fragment of the quartz vein from Wołowa Góra Mountain with yellowish oxy-dravite tourmaline (scale bar = 2 cm).

Fig. 3. Back-scattered electron images of yellowish tourmaline from Wołowa Góra Mountain Abbreviations: Trmi – primary tourmaline (oxy-dravite), Trmii – secondary tourmaline crystallizing in close transversal veinlets (fluor-dravite), Trmiii – secondary tourmaline crystallizing in open fissures as crystals terminations (dravite), later filled by quartz.
Statistics for the compositional data for the three distinguished tourmaline varieties are given in Table 1 and representative analyses of the tourmalines in Table 2. The primary tourmaline (Trmi) has high SiO2 and MgO contents, ranging between 37.04–38.50 wt.% and 10.33–11.52 wt.%, respectively; rather moderate Al2O3 and Na2O of 32.03–35.01 wt.% and 1.80–2.70 wt.%, accompanied by subordinate TiO2 (0.28–0.83 wt.%), FeO (0.22–0.61 wt.%) and V2O3 (up to 0.43 wt.%). The amount of F ranges from 0.10 to 0.78 wt.%. In contrast, the secondary Fe-enriched tourmalines Trmii and Trmiii have typical SiO2 contents (35.83–36.39 and 36.12–36.56 wt.%, respectively), slightly decreased Al2O3 (31.78–32.59 and 30.26–31.28 wt.%) and Na2O (2.37–2.48 and 2.22–2.35 wt.%), much higher than in the main tourmaline variety, but similar FeO (7.42–8.07 and 7.19–8.48 wt.%) and MgO (6.53–6.62 and 7.22–7.69 wt.%), increased TiO2 (0.45–0.54 and 0.75–1.08 wt.%) and CaO (0.43–0.54 and 0.79–1.14 wt.%). Fluorine increases up to 0.82–0.94 wt.% in the Trmii tourmaline, and locally up to 1.04 wt.% in the Trmiii variety. B2O3 and H2O complete the analyses (10.46–10.56 wt.% and 2.91–3.01 wt.%, and 10.48–10.60 wt.% and 2.93–3.09 wt.%, respectively).
All three tourmalines have the Y sites dominated by Mg although to different degrees. The primary Trmi tourmaline has very high values of the Mg/(Mg + Fe) ratio, of the order of 0.97–0.99; the remaining two tourmalines are similar to each other for this ratio with values of 0.59–0.61 and 0.60–0.66, respectively, but distinctly lower than those related to Trmi. All tourmaline varieties are alkali-group tourmalines plotting close to the Na corner in the ternary system X-site vacancy – Na – Ca, with a spread of data along the Na–Ca side (Fig. 4a). The W site of Trmi is partly occupied by O2– (0.31–0.59 pfu), and partly by OH– (0.06–0.52 apfu) and F– (0.05–0.40 pfu), with varying proportions of bivalent WO2– and monovalent W(OH + F)– anions. Therefore, it only partly meets requirements for an oxy-species (oxy-dravite), whereas the hydroxy-species (dravite) with W(OH + F) > WO and OH > F is more common (Fig. 4b). However, oxy-dravite is commonly the dominant end-member component in this tourmaline. Tourmaline from transverse veinlets cutting the crystals, Trmii, always has W(OH + F) > WO and OH < F, thus is a fluor-species, whereas Trmiii tourmaline, forming crystal terminations in fissures filled with quartz, always has W(OH + F) > WO with varying OH and F amounts, and is a fluor-species grading to a hydroxy-species.

Fig. 4. Classification of tourmalines from Wołowa Góra Mountain in term of: (a) X-site occupants; and (b) W-site occupants. Symbols: yellow crosses – Trmi, the primary tourmaline; brown crosses – Trmii, tourmaline from transverse veinlets; black crosses – Trmiii, tourmaline from the crystal terminations in fissures.
The covariation between Al and Mg + Fe as the main components at the octahedral Y and Z sites is presented in Fig. 5a. A strong negative linear relationship (r 2 = 0.96) corroborates the dominant role of one of the typical tourmaline-supergroup substitutions of deprotonation type, Mg(Fe)2+ + (F,OH)– → Al3+ + O2–, and an insignificant role of the second possible substitution of alkali-vacancy type, Na+ + Mg(Fe)2+ → □ + Al3+, due to the almost similar X-site vacancies, 0.13, 0.13 and 0.10 apfu on average in the Trmi–Trmiii varieties, respectively. Along with textural relationships and Mg/(Mg + Fe) values of 0.97–0.99, it indicates that the oxy-species participating significantly in the composition of the primary tourmaline had been replaced by the secondary Fe-enriched fluor-tourmaline and hydroxy-tourmaline species, with the decreased Mg/(Mg + Fe) values. Additionally, increasing Fe is mainly a result of the simple isovalent substitution Fe2+ → Mg2+ (r 2 = 0.94; Fig. 5b), and not the substitution Fe3+ → Al3+ (Fig. 6). The latter has been well-documented, e.g. in the form of continuous solid-solution between end-members oxy-dravite and povondraite by Žáček et al. (Reference Žáček, Frýda, Petrov and Hyršl2000).

Fig. 5. Compositional relationships in the Wołowa Góra Mountain tourmalines: (a) Al vs. Mg + Fe, (b) Fe vs. Mg, (c) Ca vs. F covariation. Symbols as in Fig. 4.

Fig. 6. Compositions of the Wołowa Góra Mountain dravitic tourmalines in the ternary system Mg–Al–Fetotal. Symbols as in Fig. 4.
The X site is mainly filled with Na; another X-site occupant is Ca or the site remains unfilled, i.e. occurs as vacancies. In the primary Trmi dravite, Ca displays a weak positive correlation with F (r 2 = 0.47) (Fig. 5c), which, at least partly indicates that the elevated XCa contents can be a partial result of Ca2+ + Mg(Fe)2+ + F– → XNa+ + ZAl3+ + WOH– substitution, leading to the presence of end-member fluor-uvite, CaMg3(Al5Mg)(Si6O18)(BO3)3(OH)3F, and a hypothetical end-member fluor-feruvite, Ca${\rm Fe}_{\rm 3}^{{\rm 2 +}} $(Al5Mg)(Si6O18)(BO3)3(OH)3F. Successively, the X-site vacancy is a result of a simple substitution □ + Al3+ → XNa+ + YMg(Fe)2+ of the foitite type, and indicates the compositional importance of the magnesio-foitite and foitite components, Na(Mg2Al)Al6(Si6O18)(BO3)3(OH)3(OH) and Na(
${\rm Fe}_{\rm 2}^{{\rm 2 +}} $Al)Al6(Si6O18)(BO3)3(OH)3(OH), respectively, in compositions of the tourmalines.
Careful inspection of Fig. 6 indicates that the compositions of all the tourmaline varieties studied can be explained as complex solid-solutions of six end-member pairs: (dravite/schorl) + (fluor-dravite/fluor-schorl) + (oxy-dravite/oxy-schorl) + (magnesiofoitite/foitite) + (uvite/feruvite) + (fluor-uvite/fluor-feruvite). Although assigning hypothetical end-members to the compositions is strongly dependent on the order of species calculations, abundances of two of the pairs can be evaluated easily on the basis of the X□ and WO2– contents (magnesiofoitite + foitite, and oxy-dravite + oxy-schorl, respectively). The results show that the composition of the primary yellowish tourmaline Trmi, is dominated by the oxy-dravitic component (~31–59 mol.%), only yielding the components dravite or fluor-dravite in single analytical spots. Successively, the secondary tourmaline Trmii, with Mg only slightly exceeding Fe2+, Al close to 6 apfu and WF > WOH ≈ WO2– is always dominated by fluor-dravite + fluor-schorl, whereas Trmiii fluctuates between dravite + schorl and fluor-dravite + fluor-schorl. The abundance of oxy-dravite + oxy-schorl components decreases from the main tourmaline variety, where the species dominate, down to tourmaline forming transverse veinlets and finally to tourmaline on terminations of crystals in open fissures. In contrast, the abundance of dravite + schorl components increases in the opposite direction, with an episode of dominance of fluor-dravite + fluor-schorl during crystallization in transverse fissures within crystals of the primary dravite.
Hawthorne (Reference Hawthorne1996) presented an explanation for the presence of O2– at the W site of the tourmaline structure, a characteristic feature of oxy-dravite, which can be satisfied for 3Al or 2Al + Mg local arrangements of the Y-octahedra triad. This requirement can be fulfilled by Mg–Al disorder induced through the substitution W(OH)– + YMg2 + ZAl ↔ WO2– + YAl2 + ZMg, leading to the end-member oxy-dravite, Na(Al2Mg)(Al5Mg)(Si6O18)(BO3)3(OH)3O = NaAl3(Al4Mg2)(Si6O18)(BO3)3(OH)3O. The equation formally provides an opportunity to evaluate the degree of the disordering from the microprobe results, because, according to Hawthorne (Reference Hawthorne1996), the WO2– content should be equal to the smaller of the two quantities: YAl/2 or ZMg. However Bosi (Reference Bosi2011), based on analysis of short-range constraints in the tourmaline structure, demonstrated that the smaller of two values YR 3+/2 and ZR 2+ may not match the content of oxygen at the W site.
The application of the short-range constraints for yellowish dravite Trmi predominated with the oxy-dravitic component indicates that for WO2– = 0.46(8) apfu (average; Table 1), the Y(Al + Ti + V) content should range between 1.26–1.58 apfu, with the mean content of 1.42 apfu (in the calculation Ti4+ was treated along with trivalent octahedral cations Al3+ and V3+). For the secondary fluor-dravite–fluor-schorl and dravite–schorl tourmalines Trmii and Trmiii, with WO2– of 0.29(5) and 0.29(3) apfu, the predicted mean Y(Al + Ti) contents are equal to 1.07 apfu. The values suggest the following populations at the Y and Z sites of the tourmalines:



Crystal structure of the primary tourmaline with a predominant oxy-dravite component
The total number of the electrons in the X, Y, Z, B and T sites derived from structural analysis, 225.1(0.4) e–, corresponds within 1 standard deviation (SD) range to the number of electrons derived from the average chemical composition of the tourmaline, 225.4(1.2) e– (Table 1b). The B site is occupied only with boron, as the refined mean bond length <B–O> = 1.374(1) Å is compatible with a distance of 1.374(2) Å evaluated by Bosi and Lucchesi (Reference Bosi and Lucchesi2007) for tourmalines with the site completely filled with B3+. Similarly the T site, although showing the shortened <T–O> distance of 1.617(1) Å, is filled only with Si. An average T–O distance significantly below 1.620 Å can indicate the presence of tetrahedrally-coordinated B3+ substituted for Si, however, a preliminary refinement with a released T-site occupancy showed no significant amounts of IVB. Bosi and Lucchesi (Reference Bosi and Lucchesi2007) proposed a reliable and statistically significant value of <Si–O> = 1.619(1) Å for the T site fully occupied by silicon, which is, within the standard deviation, identical to our measured <T–O> distance. The refined X-site scattering, corresponding to 0.744(7) Na + 0.120 Ca apfu, agrees within 1 sd with an occupancy of the site by 0.75(6) Na + 0.12(6) Ca apfu (Table 1). Both the refined Z-site scattering equal to 77.4 e– as well as the extended <Z–O> distance up to 1.9247(7) Å indicate Mg-Al disorder among the Y and Z sites. Along with the W-site occupation, O0.46OH0.33F0.21, all the results describe the disordered distribution of ions in the structure of this tourmaline, which is characteristic for a tourmaline predominant with the oxy-dravite component. As there is only 1 e– difference between Mg and Al, the ZMg content was estimated as 0.64(2) apfu through intersection of the refined <Z–O> distance using the variation in <Z–O> as a function of the Z(Al + Fe3+) content presented by Lussier et al. (Reference Lussier, Ball, Hawthorne, Henry, Shimizu, Ogasawara and Ota2016). Ferrous iron was refined at the Y site with the content of 0.153(9) apfu. The content agrees with the sum of Ti + Fe + V = 0.12(2) apfu in the mean chemical composition. The YMg and YAl contents were estimated on the basis of the Y-site scattering, 38.14(13) e–, diminished by 2.78 e–, i.e. the total electron number for 0.06Ti + 0.04Fe + 0.01 V apfu. The difference of 3 apfu at the Y site gives YMg and YAl scattering of 35.36(13) e– and leads to the following population of the Y triad: (Mg2.11Al0.78Ti0.06Fe0.04V0.01)Σ3. The population agrees within ~1.0 SD of the refined Y-site scattering with the Y-site population estimated as the difference between the total numbers of Al and Mg and the evaluated Z-site population. Finally, the structural formula of Trmi tourmaline from Wołowa Mountain, with a significant oxy-dravite component, can be presented as (Na0.75Ca0.12□0.13)Σ1(Mg1.93Al0.95Ti0.06${\rm Fe}_{{\rm 0}{\rm. 04}}^{{\rm 2 +}} $ V0.01)Σ3(Al5.38Mg0.62)Σ6B3Si6O27(OH)3(O0.46OH0.33F0.21)Σ1.
Origin
In the petrological diagrams of Henry and Guidotti (Reference Henry and Guidotti1985), showing the relationships between the composition of a tourmaline and its parent-magmatic or metamorphic rock, the Wołowa Góra dravitic tourmalines plot in terms of Al, Fe and Mg in the fields of aluminous metapelites and metapsammites, low-Ca ultramafics and (Cr,V)-rich metasediments (Fig. 7). Occurrences of V- and Cr-poor or -free oxy-dravite are linked mainly to quartz-mica schists (Bosi and Skogby, Reference Bosi and Skogby2013), mica schists (Henry and de Brodtkorb, Reference Henry and de Brodtkorb2009; Čopjakova et al., Reference Čopjakova, Škoda and Vašinová-Galiova2012), cordierite-bearing schists (Redler et al., Reference Redler, Irouschek, Jeffries and Gieré2016), and metamorphosed evaporite cap rocks (Henry et al., Reference Henry, Kirkland, Kirkland, Novák and Hawthorne1999; Žáček et al., Reference Žáček, Frýda, Petrov and Hyršl2000), whereas its Cr- and V-bearing varieties are linked to black shales, calcareous metasediments and graphite quartzites (e.g. Bosi and Lucchesi, Reference Bosi and Lucchesi2004; Bačik et al., Reference Bačík, Méres and Uher2011; Cempírek et al., Reference Cempírek, Houzar, Novák, Groat, Selway and Šrein2013). In the case of Wołowa Mountain, the primary dravite associated with secondary fluor-dravite/fluor-schorl and dravite/schorl tourmalines, occurs within a small quartz vein (a silica-bearing mobilizate) hosted in granitic gneisses of the Kowary unit, adjacent (within a distance of a dozen to a few hundred metres) to chlorite-mica schists, quartzo-feldspathic schists and amphibolites that form the Skalny Stół massif with Czoło Mountain and Wołowa Mountain (Fig. 1). The well-known amphibolites from the nearby Budniki area, have an alkali-basalt character and correspond to recent within-plate basalts (Oberc-Dziedzic et al., Reference Oberc-Dziedzic, Kryza, Mochnacki and Larionov2010). They contain disseminated Ti mineralization in the form of rutile–ilmenite–titanite aggregates several millimetres in size, and trace sulfide mineralization composed of pyrrhotite, pentlandite, gersdorffite, cobaltite, arsenopyrite, pyrite, chalcopyrite, sphalerite, galena, marcasite, and traces of wolframite and scheelite (Mochnacka et al., Reference Mochnacka, Oberc-Dziedzic, Mayer and Pieczka2008). Along with the quartzo-feldspathic schists they are considered differentiation products of a Late Cambrian to Early Ordovician bimodal volcanism with basic lavas and tuffs, acidic tuffs and intercalations of acidic and basic tuffs deposited as a volcano-sedimentary succession very probably during the ~500 Ma intense magmatic activity. The suite is interpreted as the magmatic products of Cambro–Ordovician rifting processes at a passive continental margin (Oberc-Dziedzic et al., Reference Oberc-Dziedzic, Kryza, Mochnacki and Larionov2010 and references therein). Furthermore, all the rocks underwent Early Variscan regional metamorphism (360–340 Ma; 550–600°C, P ~6 kbar), and younger contact metamorphism (325–310 Ma; 540–635–470°C) induced by the Variscan intrusion of the Karkonosze granite (Mochnacka et al., Reference Mochnacka, Oberc-Dziedzic, Mayer and Pieczka2008; Oberc-Dziedzic et al. Reference Oberc-Dziedzic, Kryza, Mochnacki and Larionov2010 and references therein).

Fig. 7. Plot of the Wołowa Góra Mountain Mg-rich tourmalines in the petrogenetic Mg–Al–Fetotal diagram of Henry and Guidotti (Reference Henry and Guidotti1985). Description of the fields: 1 – Li-rich granitoid pegmatites and aplites; 2 – Li-poor granitoids, pegmatites and aplites; 3 – Fe-rich quartz-tourmaline rocks (hydrothermally-altered granitic rocks); 4 – Al-rich metapelites and metapsammites; 5 – Al-poor metapelites and metapsammites; 6 – Fe 3+-rich quartz-tourmaline rocks; calc-silicates and metapelites; 7 – low-Ca ultramafics and (Cr,V)-rich metasediments; 8 – metacarbonates and metapyroxenites.
Textural and compositional relationships observed for the yellow dravitic tourmaline indicate that oxy-dravite is its basic component, carrying traces of V, which is also present in the chemical compositions of rutile, ilmenite and titanite in the nearby Budniki area (Mochnacka et al., Reference Mochnacka, Oberc-Dziedzic, Mayer and Pieczka2008). Thus, it is most likely that this tourmaline was grown from an Al- and Mg-rich protolith, which could have been derived from a highly metasomatically altered prior protolith, most probably related to the silicic volcaniclastic material of the bimodal volcanism. During the regional metamorphism, the protolith released a (H2O,B,F)-bearing fluid, which mobilized the most soluble components (mainly SiO2 and some other oxides) and propagated them into the surrounding rocks, leading to the crystallization of a Mg-rich tourmaline (with a significant oxy-dravite component) in quartz veins. Fluor-dravite and dravite, two secondary tourmalines crystallized during a hydrothermal overprint that can be related to later stages of the regional metamorphism, or even early stages of the contact metamorphism. Such late growth in the form of elongate zones and termination zones is usually associated with a late influx of B-bearing fluids that initiate nucleation of a new tourmaline on the fracture surface or partially replacing the earlier tourmaline generation (e.g. Henry et al., Reference Henry, Dutrow and Selverstone2002). An influx of late B-bearing fluid satisfactorily explains the enrichment in Fe, and also in Ca and Ti of the secondary fluor-dravite and schorl-dravite in relation to the primary (oxy-)dravite as a result of a few stages of partial decomposition and recrystallization of amphiboles, plagioclases and Ti-bearing minerals present in the nearby Budniki area, and remobilization of Ca2+, Ti4+ and Fe to the fluids at elevated temperature (Mochnacka et al., Reference Mochnacka, Oberc-Dziedzic, Mayer and Pieczka2008). This also explains why in the quartz vein from Wołowa Mountain oxy-dravite is a rare tourmaline, found only in the form of relics – small accumulations of the primary unaltered metamorphic dravite–oxy-dravite in inner parts of the vein. In the outer part of the vein, the tourmaline that was affected strongly by the fluids changed into the dominant dark green fluor-dravite/fluor-schorl and schorl-dravite, and black schorl in the host gneisses.
The yellowish colour of the primary Mg-rich tourmaline and yellowish-olive-brown colour observed in the secondary tourmaline varieties result from varying amounts of Fe2+ and Ti4+ in these tourmalines. The Fe/Ti ratio ranges from very low values of 0.4–1.7 in the primary tourmaline, through 17–19 in the secondary tourmalines from transverse veinlets, to 9–11 in the crystal's termination. In accordance with the spectroscopic data of Mattson and Rossman (Reference Mattson and Rossman1988) and da Fonseca-Zang et al. (Reference da Fonseca-Zang, Zang and Hofmeister2008), these data suggest that the colouration is mainly a result of the Fe2+–Ti4+ charge-transfer transitions. The transitions are responsible for the absorption at ~24,000 cm–1 and the yellowish-brown to brown colour. In the primary tourmaline, the Fe content is tiny and the probability of the Fe2+–Ti4+ charge-transfer transition is very small, which even at a relatively high Ti4+(d0) content only results in yellowish colouration. The increase of Fe2+ in the secondary tourmalines elevates the probability of the Fe2+–Ti4+ transitions and absorption at ~24,000 cm–1; however, an absorption at ~14,000 cm–1 appears simultaneously which is responsible for a blue colour, originating mainly from the spin-allowed crystal-field transitions of Fe2+ (Mattson and Rossman, Reference Mattson and Rossman1988; da Fonseca-Zang et al., Reference da Fonseca-Zang, Zang and Hofmeister2008). The colouration of greenish tints is a superposition of both types of absorptions, and may occur at relatively high contents of Fe2+ and elevated Ti4+.
The presence of oxy-dravite in stratiform layers of tourmalinites hosted in mica schists was also noted in the southern, Czech part of the Kowary–Izera unit (Čopjakova et al., Reference Čopjakova, Škoda and Vašinová-Galiova2012). This oxy-dravite tourmaline, associated with dravite + schorl and foitite + magnesiofoitite, has also been characterized by high values of the Mg/(Mg + Fe) ratio of 0.81–0.99, and comparable contents of Na (0.59–0.85 apfu), Ca (0.03–0.19 apfu) and X-site vacancy (0.04–0.39 apfu) as well as calculated WO2– (0.25–0.87 apfu), resulting in a slightly higher average content of the oxy-dravitic component (53 mol.%). However, this oxy-dravitic tourmaline was interpreted as pre-metamorphic, formed during the interaction of an Al- and Mg-rich volcano-sedimentary protolith with B-rich fluids, and the later schorl-dravite tourmaline was interpreted to be metamorphic.
Conclusions
Yellowish dravitic tourmaline, occurring in a quartz vein hosted by gneisses of the Kowary unit on the pass between Wołowa Mountain and Czoło Mountain in the main Karkonosze range, SW Poland, represents the dravite species with a dominant oxy-dravite component. Crystals of the tourmaline are cross-cut, and in the fissures fluor-dravite/fluor-schorl grading into dravite/schorl crystallized as secondary tourmaline varieties. All the tourmalines have a metamorphic origin. The primary dravite grading into oxy-dravite formed during Variscan prograde metamorphism under the action of a released (H2O,B,F)-bearing fluid, which mobilized the most soluble components of the altered silicic volcaniclastic material of the Late Cambrian to Early Ordovician bimodal volcanism as protholiths for adjacent quartzo-feldspathic schists and amphibolites, and propagated them into the surrounding gneisses of the Kowary unit. Fluor-dravite and dravite, both tourmalines enriched in Fe, Ca and Ti, crystallized in the later stages of regional metamorphism, or even early stages of contact metamorphism caused by the intrusion of the Karkonosze granite, associated with the partial decomposition and recrystallization of amphiboles, plagioclases and Ti-bearing minerals and remobilization of the elements, which is observed in the host amphibolite. The primary dravitic tourmaline is characterized by: (1) extremely high predominance of Mg over Fe [Mg/(Mg + Fe) = 0.97–0.99]; (2) WO2– contents reaching ~0.59 apfu, resulting in the dominance of the oxy-dravitic component over other tourmaline end-member species (fluor-dravite, dravite, magnesio-foitite, uvite, fluor-uvite); and (3) Mg–Al disorder between the octahedral Y and Z sites on the order of ~0.64 apfu, corroborated by the increased <Z–O> distance up to ~1.925 Å and the measured unit-cell parameters a = 15.916(1) Å and c = 7.180(1) Å.
Acknowledgements
We thank the reviewers Ferdinando Bosi, Darrel J. Henry and Peter Leverett, and the Principal Editor Peter Williams for constructive comments on this manuscript. The studies were supported by the AGH UST grant no. 11.11.140.158 to AP and by the Austrian Science Fund (FWF) projects no. P 26903-N19 and P 31049-N29 granted to AE, and by the National Science Centre (Poland) grant 2017/27/N/ST10/01579 to MS.
Supplementary material
To view supplementary material for this article, please visit https://doi.org/10.1180/minmag.2017.081.069.