Introduction
Psychotic disorders, schizophrenia being the most severe form, often present with hallucinations, delusions, disturbed thoughts, cognitive dysfunction, and a lack of insight and self-awareness. Neuroimaging studies consistently report loss of white-matter (WM) volume and microstructure integrity in patients with psychotic disorders (Whitford et al. Reference Whitford, Kubicki and Shenton2011; Peters & Karlsgodt, Reference Peters and Karlsgodt2014). Equally, although to a lesser degree, abnormalities in WM architecture are reported in populations with an increased vulnerability to develop psychotic disorders (Tan et al. Reference Tan, Arnone, McIntosh and Ebmeier2009; Bloemen et al. Reference Bloemen, de Koning, Schmitz, Nieman, Becker, de Haan, Dingemans, Linszen and van Amelsvoort2010; Jung et al. Reference Jung, Jang, Byun, An and Kwon2010; Boot & van Amelsvoort, Reference Boot and van Amelsvoort2012). This led to the hypothesis that changes in structural connectivity may be an underlying mechanism in the development of psychosis.
22q11.2 deletion syndrome (22q11DS) is one of the most frequent recurrent copy number variant disorders found in humans and associated with 30% lifetime susceptibility to develop a psychotic disorder (Murphy et al. Reference Murphy, Jones and Owen1999). The syndrome is caused by microdeletions on the 22q11.2 locus and is characterized by a typical facial appearance, cleft palate, velopharyngeal insufficiency, intellectual disabilities, and cardiac anomalies (Maynard et al. Reference Maynard, Haskell, Lieberman and LaMantia2002). The 22q11.2 locus codes for, among other things, genes implicated in neuronal migration and neurogenesis affecting WM development (Fournier et al. Reference Fournier, GrandPre and Strittmatter2001, Reference Fournier, Gould, Liu and Strittmatter2002; Maynard et al. Reference Maynard, Haskell, Peters, Sikich, Lieberman and LaMantia2003; Meechan et al. Reference Meechan, Tucker, Maynard and LaMantia2012; Perlstein et al. Reference Perlstein, Chohan, Coman, Antshel, Fremont, Gnirke, Kikinis, Middleton, Radoeva, Shenton and Kates2014). Due to the high risk for developing psychosis, 22q11DS is considered a unique model to study neurobiology of psychosis.
A similar, but acute risk rate is observed in clinically identified ultra-high risk (UHR) patients. UHR patients have 30% chance of developing a psychotic disorder within 2 years after identification (Fusar-Poli et al. Reference Fusar-Poli, Borgwardt, Bechdolf, Addington, Riecher-Rössler, Schultze-Lutter, Keshavan, Wood, Ruhrmann, Seidman, Valmaggia, Cannon, Velthorst, De Haan, Cornblatt, Bonoldi, Birchwood, McGlashan, Carpenter, McGorry, Klosterkötter, McGuire and Yung2013). They are young help-seeking individuals with either (1) attenuated psychotic symptoms, (2) brief psychotic symptoms which spontaneously remit within a week, or (3) a schizotypal personality disorder or a first-degree relative with a psychotic disorder, in combination with a significant decrease in functioning during the previous year.
Structural magnetic resonance imaging (MRI) and diffusion tensor imaging (DTI) – MRI techniques, respectively, are commonly used to assess WM volume and microstructure. Changes in WM microstructure can be quantified by measuring changes in the shape of the diffusion profile, making use of three indices: fractional anisotropy (FA), axial diffusivity (AD), radial diffusivity (RD), and mean diffusivity (MD) rate. FA reductions are considered to reflect loss of overall WM microstructure integrity (Beaulieu, Reference Beaulieu2002), and lower AD loss of axonal integrity (Song et al. Reference Song, Sun, Ju, Lin, Cross and Neufeld2003). Increases in RD indicate less restriction of diffusion which has been associated with loss of myelination, fiber organization, and/or cytoskeleton (Song et al. Reference Song, Yoshino, Le, Lin, Sun, Cross and Armstrong2005).
Only a small number of studies have investigated changes in WM volume and microstructure within these two high-risk populations. In 22q11DS patients an increasing amount of WM volume abnormalities are reported as WM develops from early childhood to adolescence (Gothelf et al. Reference Gothelf, Feinstein, Thompson, Gu, Penniman, Van Stone, Kwon, Eliez and Reiss2007; Sundram et al. Reference Sundram, Campbell, Azuma, Daly, Bloemen, Barker, Chitnis, Jones, van Amelsvoort, Murphy and Murphy2010; Kates et al. Reference Kates, Antshel, Faraone, Fremont, Higgins, Shprintzen, Botti, Kelchner and McCarthy2011), with ultimately widespread WM volume reductions of the temporal lobes, cingulum, corpus callosum (CC), insula, and occipito-parietal lobes (Chaddock et al. Reference Chaddock, Lee, Newton, Baldeweg, Frackwoiak and Skuse2006; Da Silva Alves et al. Reference Da Silva Alves, Schmitz, Bloemen, van der Meer, Meijer, Boot, Nederveen, de Haan, Linszen and van Amelsvoort2011; Radoeva et al. Reference Radoeva, Coman, Antshel, Fremont, McCarthy, Kotkar, Wang, Shprintzen and Kates2012) compared to typically developing healthy controls (HC). Changes in WM volume in these regions are reported in UHR patients compared to HC, with the most consistent finding temporal lobe WM volume reduction (Walterfang et al. Reference Walterfang, McGuire, Yung, Phillips, Velakoulis, Wood, Suckling, Bullmore, Brewer, Soulsby, Desmond, McGorry and Pantelis2008; Witthaus et al. Reference Witthaus, Brüne, Kaufmann, Bohner, Ozgürdal, Gudlowski, Heinz, Klingebiel and Juckel2008; Fusar-Poli et al. Reference Fusar-Poli, Crossley, Woolley, Carletti, Perez-Iglesias, Broome, Johns, Tabraham, Bramon and McGuire2011).
Looking at WM microstructure changes, recent studies report lower FA along the uncinate fasciculus (UF) (Radoeva et al. Reference Radoeva, Coman, Antshel, Fremont, McCarthy, Kotkar, Wang, Shprintzen and Kates2012) and widespread effects of lower AD within several midbrain tracts and throughout several large cortical fasciculi (Kikinis et al. Reference Kikinis, Asami, Bouix, Finn, Ballinger, Tworog-Dube, Kucherlapati, Kikinis, Shenton and Kubicki2012; Radoeva et al. Reference Radoeva, Coman, Antshel, Fremont, McCarthy, Kotkar, Wang, Shprintzen and Kates2012) in 22q11DS patients compared to HC. Recent studies also report lower RD in 22q11DS patients in these tracts (Jalbrzikowski et al. Reference Jalbrzikowski, Villalon-Reina, Karlsgodt, Senturk, Chow, Thompson and Bearden2014; Perlstein et al. Reference Perlstein, Chohan, Coman, Antshel, Fremont, Gnirke, Kikinis, Middleton, Radoeva, Shenton and Kates2014; Kates et al. Reference Kates, Olszewski, Gnirke, Kikinis, Nelson, Antshel, Fremont, Radoeva, Middleton, Shenton and Coman2015). Lower AD may point towards smaller axon diameters suggesting possible axonal malformation. DTI studies in UHR patients have reported changes in WM microstructure in the superior longitudinal fasciculus (SLF) (fronto-parietal fasciculus), midbrain projection fibers, and limbic circuitry (Peters et al. Reference Peters, de Haan, Dekker, Blaas, Becker, Dingemans, Akkerman, Majoie, van Amelsvoort, den Heeten and Linszen2008, Reference Peters, Schmitz, Dingemans, van Amelsvoort, Linszen, de Haan, Majoie and den Heeten2009, Reference Peters, Dingemans, Dekker, Blaas, Akkerman, Van Amelsvoort, Majoie, Den Heeten, Linszen and De Haan2010; Karlsgodt et al. Reference Karlsgodt, Niendam, Bearden and Cannon2009; Bloemen et al. Reference Bloemen, de Koning, Schmitz, Nieman, Becker, de Haan, Dingemans, Linszen and van Amelsvoort2010; Carletti et al. Reference Carletti, Woolley, Bhattacharyya, Perez-Iglesias, Fusar Poli, Valmaggia, Broome, Bramon, Johns, Giampietro, Williams, Barker and McGuire2012). These studies report lower FA, higher RD and overall higher MD in these tracts. No differences in AD measures have been reported in UHR to date, suggesting differences in myelination integrity, cytoskeleton, and/or organization, rather than changes in axonal integrity in UHR patients.
It is still unclear whether any of the differences found in WM volume and microstructure are specific to 22q11DS or UHR status, or which are shared. To our knowledge, this is the first study to compare WM structure between these two high-risk groups. We hypothesize that there will be shared lower temporal lobe WM volume. Additionally we expect, based on the current literature, that underlying abnormalities in WM microstructure will be different, with lower AD in the 22q11DS group, and higher RD in the UHR group.
Method
The UHR patients were recruited through the expert program for early psychosis at the Academic Medical Center (AMC) and diagnosed through the structured interview for prodromal symptoms (SIPS; Miller et al. Reference Miller, McGlashan, Rosen, Cadenhead, Cannon, Ventura, McFarlane, Perkins, Pearlson and Woods2003) by a trained psychiatrist or psychologist. An individual was identified as an UHR patient when they were help-seeking individuals with either (1) attenuated psychotic symptoms (APS), (2) brief limited psychotic symptoms (BLIPS) which spontaneously remitted within a week, or (3) a schizotypal personality disorder or a first-degree relative with a psychotic disorder combined with a significant decrease in functioning over the previous year. Criteria for APS was met if one or more of the five of positive items of the scale of prodromal symptoms (SOPS) was rated 3–5 (within the prodromal range) with symptoms beginning within the past year or increasing one or more points in the past year, and occurring at least once per week for the last month. Criteria for BLIPS were met if a rating of 6 (psychotic range) on one or more of the five positive items was scored with an onset 3 months prior and symptoms occur at least several minutes per day, at least once per month. Individuals with 22q11DS were recruited through the Dutch 22q11DS family association and several Dutch centers for clinical genetics. All 22q11DS patients were interviewed by a certified psychiatrist specialized in the 22q11DS using the Mini International Neuropsychiatric Interview (MINI; Sheehan et al. Reference Sheehan, Lecrubier, Sheehan, Amorim, Janavs, Weiller, Hergueta, Baker and Dunbar1998). For both patient groups symptom severity at time of scanning was assessed using the Positive and Negative Syndrome Scale (PANSS; Kay et al. Reference Kay, Fiszbein and Opler1987). HC subjects were recruited through advertisement. Intellectual ability was estimated using a shortened Wechsler Adult Intelligence Scale (WAIS). Age range for inclusion was set between 18 and 37 years. Participants were excluded according to following criteria; present substance use or history of abuse or dependency, neurological affliction, or pregnancy. Substance use and pregnancy were checked through urine tests. Additionally, HC subjects were excluded if they had a family history of psychotic illness or had received a DSM-IV diagnosis in the past.
The current study included 23 UHR patients, twenty-one 22q11DS patients, and 33 HC subjects. Twenty UHR patients met the criteria for APS, and three for BLIPS. Our sample included nine 22q11DS patients with a history of psychosis and 12 without a history of psychosis. All UHR patients were drug-free, and mostly drug-naive with the exception of three UHR patients that received a single dose of an antipsychotic, and four UHR patients that received brief antipsychotic treatment >3 months prior to scanning. All nine 22q11DS patients with a history of psychosis were being treated with antipsychotic medication and had a diagnosis of schizophrenia or related disorders (Schneider et al. Reference Schneider, Schaer, Mutlu, Menghetti, Glaser, Debbané and Eliez2014). One 22q11DS patient without a history of psychosis was diagnosed with a mood disorder. Use of medication and PANSS scores at time of scanning for both UHR and 22q11DS patients are given in Table 1.
Table 1. Demographic and clinical variables

UHR, Ultra-high risk subjects; APS, attenuated psychotic symptoms; BLIPS, brief limited intermitted psychotic symptoms; 22q11DS, 22q11 deletion syndrome patients; p, 22q11DS patients with a history of psychosis; np, 22q11DS patients without history of psychosis; HC, healthy controls; IQ, intelligence quotient; PANSS, Positive and Negative Syndrome Scale; SIPS, structured interview for prodromal symptoms; SSRI, selective serotonin reuptake inhibitor; NRI, norepinephrine reuptake inhibitor SNRI, selective NRI; s.d.,standard deviation.
The study was approved by an independent medical ethics board of the AMC in Amsterdam. All subjects gave written informed consent after all study procedures were fully explained.
Structural MR and DTI data acquisition
Whole-brain MRI acquisition took place at the Department of Radiology (AMC, Amsterdam, The Netherlands) using a 3-T Intera MRI system (Philips, The Netherlands) equipped with a 6-channel sense head coil. Structural 3D T1-weighted high-resolution, gradient echo images were acquired; full head coverage; repetition time (TR)/echo time (TE) of 9.8/4.6; axial orientation; 120 continuous (no inter-slice gap) slices; slice thickness 2 mm; flip angle 8°; 224 × 117 mm field of view (FOV); acquisition matrix 192 × 152 × 120; acquisition voxel size 1.17 × 1.17 × 1.20 mm to identify WM, and cerebrospinal fluid for volumetric morphology measurements. DTI images were acquired using spin echo single-shot echo-planar imaging with a TR/TE of 4834/94 ms diffusion sensitivities of b = 0 and b = 1000 s/mm2; 32 diffusion gradient directions; 38 continuous (no inter-slice gap) slices; slice thickness 3 mm; 230 × 230 mm FOV; acquisition matrix 112 × 112; acquisition voxel size 2.05 × 2.05 × 3 mm.
Statistical analyses
Demographic variables
Statistical analyses were conducted using SPSS release 20 for Windows (SPSS Inc., USA). Group differences in age and PANSS scores were examined using analysis of variance (ANOVA). Group differences in gender were tested using χ2 tests. Level of statistical significance was set at p < 0.05 (two tailed).
WM volume analyses
Voxel-based morphometry analysis was conducted to investigate the differences in WM volume between the three groups. Image analysis was carried out using SPM8 (Statistical Parametric Mapping software, version 8, http://www.fil.ion.ucl.ac.uk/spm) implemented in Matlab 2010b (Mathworks, USA). Diffeomorphic Anatomical Registration Anatomical Registration using Exponentiated Lie algebra (DARTEL; Ashburner, Reference Ashburner2007) procedure was used to create a study-specific template for tissue segmentations and a high-dimensional normalization protocol. Smoothed, modulated, WM images normalized to Montreal Neurological Institute (MNI) space were used for the statistical analysis. Smoothing was carried out using isotropic Gaussian kernel of 8 mm full-width at half-maximum.
First, an F test to determine effect of group on WM volumes was conducted. Between-group differences in WM volume were estimated by fitting an analysis of covariance (ANCOVA) at each voxel that included gender, age and intra-cranial volume (ICV). Voxels and clusters were localized in MNI space.
WM microstructure parameters FA, RD, AD and MD
The work described in this paper was partially performed using the AMC Neuroscience Gateway, using resources of the Dutch e-Science Grid with the support of SURF Foundation (Shahand et al. Reference Shahand, Benabdelkader, Jaghoori, Mourabit, Huguet, Caan, van Kampen and Olabarriaga2015).
Head motion and deformations induced by eddy currents were corrected for by an affine registration of the DTI data to the non-diffusion-weighted image (DWI). The gradient directions were corrected by the rotation component of the transformation. The DWIs were resampled isotropically. Rician noise in the DWIs was reduced by an adaptive noise-filtering method (Caan et al. Reference Caan, Khedoe, Poot, den Dekker, Olabarriaga, Grimbergen, van Vliet and Vos2010). Diffusion tensors were estimated in a nonlinear least squares sense. From the tensors FA, AD, RD and MD maps were computed.
A voxel-wise statistical analysis of the DTI data was performed using tract-based spatial statistics (TBSS; Smith et al. Reference Smith, Jenkinson, Johansen-Berg, Rueckert, Nichols, Mackay, Watkins, Ciccarelli, Cader, Matthews and Behrens2006) implemented in the FMRIB Software Library 4.1.6 (University of Oxford, UK). All FA data were then aligned into a common space using the nonlinear registration tool FNIRT, which uses a b-spline representation of the registration warp field (Rueckert et al. Reference Rueckert, Sonoda, Hayes, Hill, Leach and Hawkes1999). Next, the mean FA image was created and thinned to create a mean FA skeleton, which represents the centers of all tracts common to the group. Each subject's aligned FA data was then projected onto this skeleton and the resulting data fed into voxel-wise cross-subject statistics.
Pair-wise assessed group differences among UHR, 22q11DS, and HC for FA, AD, RD and MD were assessed using randomize, a TBSS statistical tool that computes non-parametric permutations using the generalized linear model. Threshold-free cluster enhancement, was used to identify ‘clusters’ of significant results in the dataset (Nichols & Holmes, Reference Nichols and Holmes2002). The number of permutations was set to 500 using age and gender as confound regressors. Significance values were reported at p < 0.05 with correction for false discovery rate (FDR; Zalesky, Reference Zalesky2011). The principal WM tracts were derived from the Johns Hopkins University WM probabilistic tractography and ICBM-DTI-81 WM labels atlases (Mori et al. Reference Mori, Wakana, van Zijl and Nagae-Poetscher2005; Wakana et al. Reference Wakana, Caprihan, Panzenboeck, Fallon, Perry, Gollub, Hua, Zhang, Jiang, Dubey, Blitz, van Zijl and Mori2007; Hua et al. Reference Hua, Zhang, Wakana, Jiang, Li, Reich, Calabresi, Pekar, van Zijl and Mori2008). Additionally, TBSS analyses assessing FA, AD, RD and MD between 22q11DS patients with a history of psychosis and those without a history of psychosis were conducted.
Clinical markers
We employed the randomize tool to identify WM tract clusters that significantly correlated with positive and negative symptom severity within the patient groups on FA, AD, and RD measures. Post-hoc correlations were assessed between FA, AD and RD and positive and negative symptom severity if similar tracts showed abnormal diffusivity in comparisons between UHR patients and HC and 22q11DS patients and HC. A mask was created from regions where both UHR and 22q11DS differed significantly from controls. Mean values of FA, AD, and RD within this mask were then correlated with normalized PANSS scores for each subscale.
All analyses were done correcting for age, gender, and brain volume with a FDR correction for multiple comparisons.
Results
Subjects and demographic variables
A summary of the participant's demographic information is listed in Table 1. The UHR, 22q11DS patients and HC did not differ in age [F = 1.52(2), p = 0.94], and gender (χ2 = 0.13, p = 0.94). UHR patients did score significantly higher on the PANSS positive symptom subscale compared to 22q11DS patients. For the other PANSS subscales no significant differences were found between the patients groups. The UHR patients had a significantly higher IQ score than the 22q11DS patients (t = 6.85, p = 0.001). No significant difference in IQ was found between the 22q11DS patients with a history of psychosis and those without a history of psychosis (t = 1.43, p = 0.17).
Whole-brain WM microstructure comparisons
Comparisons between UHR and 22q11DS subjects
UHR patients had significantly higher mean MD compared to 22q11DS patients (Fig. 1). UHR patients showed significantly bilateral lower FA in the anterior thalamic radiation (ATR), splenium and body of the CC, anterior corona radiata and posterior corona radiata (PCR). The 22q11DS patients had significantly lower AD bilaterally in the SLF, inferior fronto-occipital fasciculus (IFOF), and the body and splenium of the CC compared to UHR patients. Results show significantly higher RD in the left ATR, splenium and body of the CC, and superior corona radiata (SCR) in the UHR patients compared to 22q11DS patients.
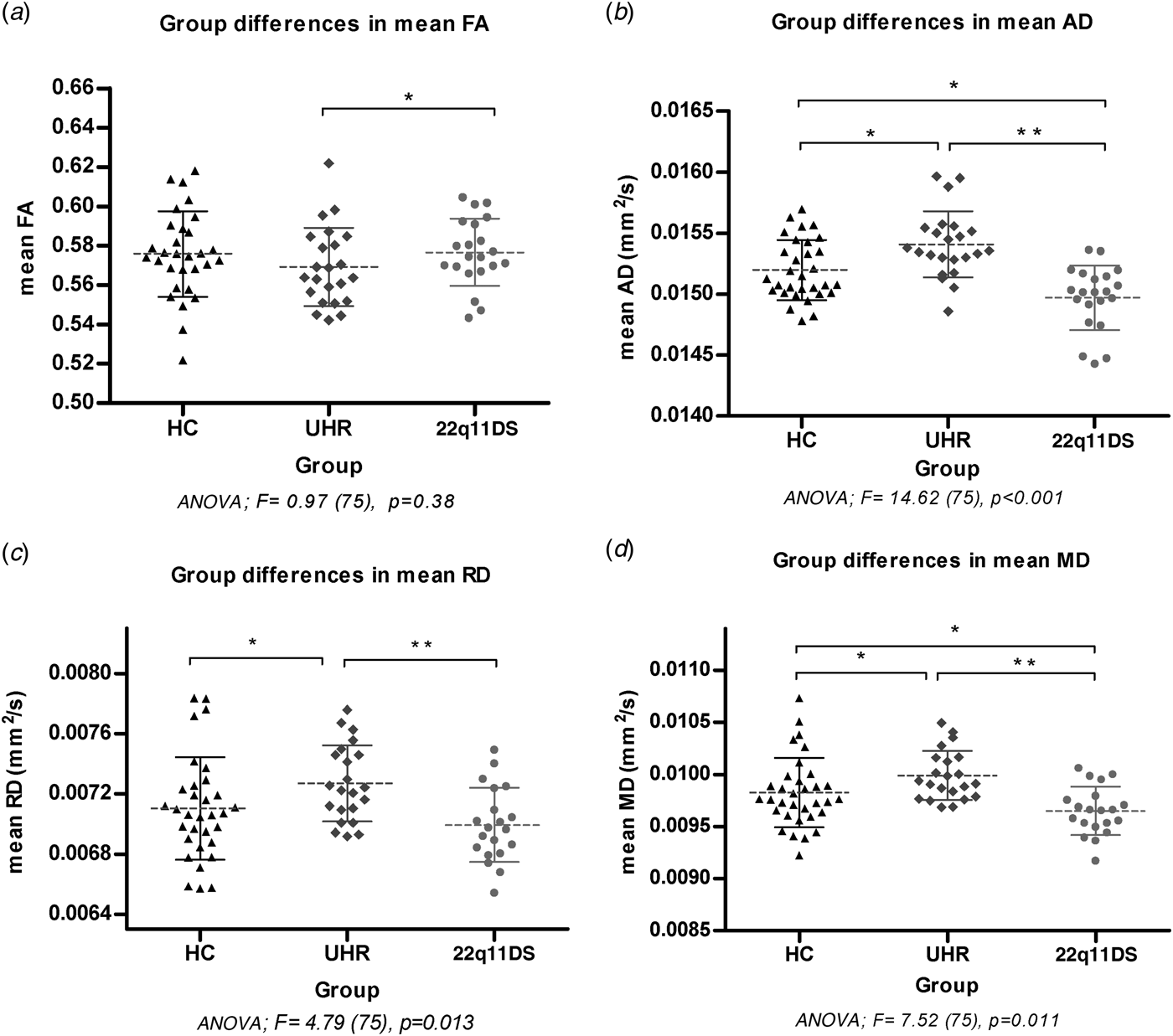
Fig. 1. Comparisons of mean DTI measures in regions of significant main effects from the TBSS analysis. The value of mean FA ranges between 0 (isotropic diffusivity) and 1(anisotropic diffusivity). The values of AD, RD and MD are expressed in mm2/s. The horizontal bars indicate the means and standard deviations per group.
Comparisons 22q11DS group and control subjects
Whole-brain TBSS results show significantly higher FA in the 22q11DS group compared to HC subjects in the ATR, posterior thalamic radiation (PTR), left IFOF, CC and forceps minor. Additionally, widespread effects of bilateral lower AD in the inferior longitudinal fasciculus (ILF), IFOF, SLF, UF, sagittal striatum, right ATR and splenium of CC, and lower RD in the left SLF, and in the body and splenium of CC and PCR were found. Significantly higher MD was found in the ATR bilaterally. Whole-brain MD was significantly lower compared to HC. No significant differences were found in FA, AD, RD and MD between 22q11DS patients with a history of psychosis and those without a history of psychosis.
Comparisons of UHR group and control subjects
In the UHR patients we found significant AD in the bilateral ATR, left IFOF, left SLF, splenium and body of the CC, and SCR compared to HC. Clusters of significant lower MD were also found in the cingulum (cingulate gyrus) compared to HC. Whole-brain mean RD and MD was significantly higher compared to HC.
All findings were found at p < 0.05 FDR corrected. TBSS results are summarized in Fig. 1, and tract-specific significant clusters with mean t values in Fig. 2.

Fig. 2. Results of whole-brain TBSS voxel-wise analyses. Lower and higher DTI measures are indicated in dark to light blue and red to yellow color bars and represent significant findings at p < 0.05 FDR corrected. Lower FA: lower overall fiber microstructure integrity; higher RD: lower myelination/cytoskeleton integrity; lower AD: lower axonal integrity. The results are overlaid on the mean diffusion weighted image of all participants (image presented in grayscale) and the mean FA skeleton is depicted in green. R = L; p < 0.05 FDR-corrected, model corrected for age gender and brain segmentation volume. HC (n = 33), UHR (n = 23), 22q11DS (n = 21). t = mean t value of significant voxels. AD, Axial diffusivity; ATR, anterior thalamic radiation; CC, corpus callosum; CR, corona radiata; FA, fractional anisotropy; HC, healthy controls; IFOF, inferior fronto-occipital fasciculus; ILF, inferior longitudinal fasciculus; PTR, posterior thalamic radiation; RD, radial diffusivity; SLF, superior longitudinal fasciculus; UHR, ultra-high risk; UF, uncinate fasciculus.
WM volume comparisons
Post-hoc testing revealed significant lower total WM volume in the 22q11DS patients (mean = 0.42) compared to UHR (mean = 0.49, t = 4.32, p < 0.001) and HC (mean = 0.47, t = 3.06, p = 0.0009) subjects. Total WM volume did not differ between UHR and HC (t = 1.28, p = 0.205). No differences were found in total WM volume between non-psychotic and psychotic 22q11DS patients (mean = 0.42, t = 0.24, p = 0.63).
The 22q11DS patients showed significantly lower WM volume in the bilateral cuneus, left superior temporal gyrus, precuneus and internal capsule compared to UHR patients. Comparisons with the matched HC showed significant reduction of WM volume in the bilateral cuneus and posterior cingulum. UHR patients did not have regions of significantly lower WM volume in comparison to HC. All regions of WM differences with corresponding MNI coordinates and p values can be found in Table 2.
Table 2. Differences in WM volumes between ultra-high risk (UHR), 22q11.2 deletion syndrome (22q11DS) and healthy control (HC) groups
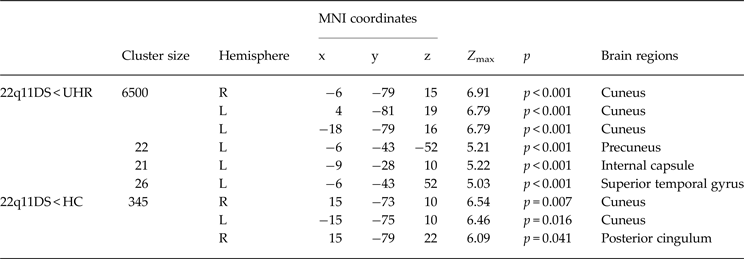
Model corrected for age, gender and intracranial volume k-threshold = 10.
Clinical markers
No significant voxel-wise correlations were found between FA, AD and RD and positive and negative symptom severity scores in either UHR, or 22q11DS.
Discussion
The current study is the first to compare WM structure of 22q11DS patients and UHR patients. Both patient populations have an increased vulnerability to develop a psychotic disorder. The main results show significantly lower MD, AD, RD and higher FA in 22q11DS patients compared to the UHR patients. HC subjects had intermediate values for MD, AD and RD compared to 22q11DS patients (lowest) and UHR patients (highest). Additionally, 22q11DS patients had significantly higher FA in the CC and ATR compared to HC.
WM microstructure changes
In line with our hypothesis, our results showed distinct patterns of WM microstructure alterations between 22q11DS and UHR patients. The 22q11DS patients had lower AD, RD, and higher FA compared to the UHR patients. Measures of AD and RD in HC subjects differed significantly from both high-risk groups, with 22q11DS patient having significantly lower AD and RD, and UHR significantly higher AD and RD (see Fig. 1). So interestingly, the two risk profiles with a similar increased vulnerability to develop psychosis are associated with directionally opposite diffusivity profiles in WM tracts. Most prominent changes were in abnormal AD in both risk groups, with less pronounced effects in FA.
Results reveal that 22q11DS and UHR patients differ the most in CC and ATR WM microstructure integrity, with 22q11DS patients having significantly higher FA. Comparisons with HC subjects showed this difference was driven by abnormally higher FA in 22q11DS patients, and abnormally higher AD in UHR patients. These regions are commonly reported to be affected throughout the development of psychosis, including in chronic psychotic patients (Peters et al. Reference Peters, de Haan, Dekker, Blaas, Becker, Dingemans, Akkerman, Majoie, van Amelsvoort, den Heeten and Linszen2008; Whitford et al. Reference Whitford, Kubicki and Shenton2011; Nakamura et al. Reference Nakamura, Kawasaki, Takahashi, Furuichi, Noguchi, Seto and Suzuki2012). Underlying changes associated with high FA include increases in myelination, microscopic deficits of axonal structures, decreases in axonal diameter, packing density and branching (Beaulieu, Reference Beaulieu2002). Abnormally higher AD is attributed to opposite type pathology, namely increases in axon diameter. Thus, lower WM microstructure integrity in the ATR and CC can be related to both genetic and clinical risk factors.
In line with our whole-brain analysis, clusters of higher FA in the ATR and CC are also reported in in a tractography study comparing adolescent 22q11DS patients with HC (Perlstein et al. Reference Perlstein, Chohan, Coman, Antshel, Fremont, Gnirke, Kikinis, Middleton, Radoeva, Shenton and Kates2014). This study finds age effects did not explain the significantly higher FA in these tracts. These patients were younger than those included in the current study showing FA changes persist in adulthood. This tractography study also related the higher FA to greater positive symptom severity, suggesting lower FA to be protective in 22q11DS. Moreover, the degree of increased FA was related to variation of the NOGO-66 receptor gene coded on 22q11.2. Deficient expression of this receptor leads to less inhibition of axonal sprouting and myelin-mediated axonal growth, which can underwrite microstructure development with high FA (Fournier et al. Reference Fournier, GrandPre and Strittmatter2001; Maynard et al. 2002). Although we did find a similar positive correlation between FA and positive symptom severity in this tract, it was not significant. Possibly our patients had too few positive symptoms to reliably assess their relation to FA.
Looking at the composite factors AD and RD of FA in 22q11DS, results show widespread bilateral abnormal lower AD and RD throughout the cortical fasciculi. If AD decreases at a faster rate than RD, higher measures of FA are found, such as in the ATR and CC. Potentially this higher FA is driven by abnormal AD and RD, suggesting cortical fasciculi have thin axons with low diameters (Beaulieu, Reference Beaulieu2002). Enhanced neuronal sprouting and the myelin-mediated growth due to lower NOGO-66 receptor expression may lead insufficient axonal development and diameter.
The bilateral pattern of lower AD and RD reported in 22q11DS in the current study is in line with results reported by earlier studies investigating WM microstructure in adolescent and young adult 22q11DS patients in comparison to HC subjects (Radoeva et al. Reference Radoeva, Coman, Antshel, Fremont, McCarthy, Kotkar, Wang, Shprintzen and Kates2012; Jalbrzikowski et al. Reference Jalbrzikowski, Villalon-Reina, Karlsgodt, Senturk, Chow, Thompson and Bearden2014; Perlstein et al. Reference Perlstein, Chohan, Coman, Antshel, Fremont, Gnirke, Kikinis, Middleton, Radoeva, Shenton and Kates2014; Kates et al. Reference Kates, Olszewski, Gnirke, Kikinis, Nelson, Antshel, Fremont, Radoeva, Middleton, Shenton and Coman2015). Large cortical fasciculi mature from adolescence until late adulthood and play a critical role in maturation of processing speed (Peters et al. Reference Peters, Ikuta, Derosse, John, Burdick, Gruner, Prendergast, Szeszko and Malhotra2014). Our results suggest that these early decreases in AD and RD measures in fasciculi maturation persist as cortical fasciculi reach full maturation in late adulthood. A sibling study attributed these early changes specifically to 22q11DS, rather than familial inheritance (Radoeva et al. Reference Radoeva, Coman, Antshel, Fremont, McCarthy, Kotkar, Wang, Shprintzen and Kates2012; Perlstein et al. Reference Perlstein, Chohan, Coman, Antshel, Fremont, Gnirke, Kikinis, Middleton, Radoeva, Shenton and Kates2014). In UHR patients we found the opposite pattern (higher AD and RD) compared to 22q11DS, further supporting lower AD and RD to be specific to 22q11DS. Taken together our results and those of previous studies suggest lower axonal integrity and/or fiber coherence may be specific to the 22q11DS.
Lower AD and RD may also be attributed to smaller axon bundle diameters, but also to axonal degeneration and/or damage (Schwartz et al. Reference Schwartz, Cooper, Fan, Jawad, Chin, Nissanov and Hackney2005; Harsan et al. Reference Harsan, Poulet, Guignard, Steibel, Parizel, de Sousa, Boehm, Grucker and Ghandour2006; Paus, Reference Paus2010; Paus et al. Reference Paus, Pesaresi and French2014). The underlying cause of these microstructural changes are still debatable, but include – infection, inflammation, trauma, and ischemia (Barazany et al. Reference Barazany, Basser and Assaf2009; Shereen et al. Reference Shereen, Nemkul, Yang, Adhami, Dunn, Hazen, Nakafuku, Ning, Lindquist and Kuan2011; Sasaki et al. Reference Sasaki, Pasternak, Mayinger, Muehlmann, Savadjiev, Bouix, Kubicki, Fredman, Dahlben, Helmer, Johnson, Holmes, Forwell, Skopelja, Shenton, Echlin and Koerte2014; Colpak et al. Reference Colpak, Kurne, Oguz, Has, Dolgun and Kansu2015). Previous studies suggested that the observed pattern of lower AD and RD were fitting with DTI investigations of ischemia (Beaulieu, Reference Beaulieu2002; Song et al. Reference Song, Sun, Ju, Lin, Cross and Neufeld2003; Bakiri et al. Reference Bakiri, Burzomato, Frugier, Hamilton, Káradóttir and Attwell2009). The wide range of cardiac anomalies, from which 22q11DS patients frequently suffer, may lead to lower perfusion and ischemia in the brain causing axonal damage (Radoeva et al. Reference Radoeva, Coman, Antshel, Fremont, McCarthy, Kotkar, Wang, Shprintzen and Kates2012; Perlstein et al. Reference Perlstein, Chohan, Coman, Antshel, Fremont, Gnirke, Kikinis, Middleton, Radoeva, Shenton and Kates2014; Kates et al. Reference Kates, Olszewski, Gnirke, Kikinis, Nelson, Antshel, Fremont, Radoeva, Middleton, Shenton and Coman2015). Future studies should prospectively link changes in WM microstructure to variations of genes located at 22q11.2 and psychotic symptoms, to discern which changes in 22q11DS are driving development of psychosis.
To date, there are only few studies investigating AD and RD in UHR patients compared to HC. In line with our results, two other studies report significantly higher RD in fronto-parietal fasciculi compared to HC (Carletti et al. Reference Carletti, Woolley, Bhattacharyya, Perez-Iglesias, Fusar Poli, Valmaggia, Broome, Bramon, Johns, Giampietro, Williams, Barker and McGuire2012; Clemm von Hohenberg et al. Reference Clemm von Hohenberg, Pasternak, Kubicki, Ballinger, Vu, Swisher, Green, Giwerc, Dahlben, Goldstein, Woo, Petryshen, Mesholam-Gately, Woodberry, Thermenos, Mulert, McCarley, Seidman and Shenton2013). A study by Carletti et al. (Reference Carletti, Woolley, Bhattacharyya, Perez-Iglesias, Fusar Poli, Valmaggia, Broome, Bramon, Johns, Giampietro, Williams, Barker and McGuire2012) reports a linear relationship of lower AD and RD across HC (lowest), UHR patients (intermediate), and patients who have developed a first psychosis (highest) (Carletti et al. Reference Carletti, Woolley, Bhattacharyya, Perez-Iglesias, Fusar Poli, Valmaggia, Broome, Bramon, Johns, Giampietro, Williams, Barker and McGuire2012). This longitudinal study shows that progressive increases in AD and RD were related to the development of psychotic symptoms. In both these studies UHR patients were receiving antipsychotic treatment at time of scanning. In our study all UHR patients were free from medication at scanning, and we are the second to report higher AD along increases in RD in UHR patients.
Abnormal increases in AD and RD may reflect myelin degradation and increases in axon diameters (Song et al. Reference Song, Sun, Ramsbottom, Chang, Russell and Cross2002; Paus, Reference Paus2010; Paus et al. Reference Paus, Pesaresi and French2014). Ischemia and infection could damage myelin leading to higher RD in humans (Song et al. Reference Song, Sun, Ramsbottom, Chang, Russell and Cross2002; Schwartz et al. Reference Schwartz, Cooper, Fan, Jawad, Chin, Nissanov and Hackney2005; Harsan et al. Reference Harsan, Poulet, Guignard, Steibel, Parizel, de Sousa, Boehm, Grucker and Ghandour2006). However, as mentioned earlier, these processes have also been associated with lower RD due to swelling of myelin sheaths (Song et al. Reference Song, Sun, Ju, Lin, Cross and Neufeld2003; Shereen et al. Reference Shereen, Nemkul, Yang, Adhami, Dunn, Hazen, Nakafuku, Ning, Lindquist and Kuan2011; Colpak et al. Reference Colpak, Kurne, Oguz, Has, Dolgun and Kansu2015). Changes occurring to myelin cannot be determined using the DTI technique. A recent validation study shows T1 mapping can reliably measure brain myelin content (Stüber et al. Reference Stüber, Morawski, Schäfer, Labadie, Wähnert, Leuze, Streicher, Barapatre, Reimann, Geyer, Spemann and Turner2014). Future studies should be done using T1 mapping to investigate myelin content.
Our results show increases in both AD and RD in UHR patients which may be associated with lower neuronal differentiation (Braskie et al. Reference Braskie, Jahanshad, Toga, McMahon, de Zubicaray, Martin, Wright and Thompson2012), in which neurotropic factors play an important role. Expression of neurotropic factors is particularly important for brain myelination in adolescence (Braskie et al. Reference Braskie, Jahanshad, Toga, McMahon, de Zubicaray, Martin, Wright and Thompson2012). Lower expression of neurotropic factor in adolescence may lead to lower neuronal differentiation, but also lower myelin integrity. Several environmental factors impact the level of neurotropic factor expression including stress and inflammation (Mondelli et al. Reference Mondelli, Cattaneo, Belvederi Murri, Di Forti, Handley, Hepgul, Miorelli, Navari, Papadopoulos, Aitchison, Morgan, Murray, Dazzan and Pariante2011). Although we do not know which underlying process is causing the higher RD and AD in our UHR sample, it is nevertheless interesting that the underlying process of abnormalities in diffusivity in UHR is associated with very different WM micropathology (larger axon diameter) compared to 22q11DS (smaller axon diameter).
WM volume
Analysis of total WM volume showed 22q11DS patients had lower total WM volume compared to UHR patients, and HC. UHR patients did not differ in total WM volume from HC subjects. These findings were corrected for ICV differences to correct for smaller brain sizes in 22q11DS (Sinderberry et al. Reference Sinderberry, Brown, Hammond, Stevens, Schall, Murphy, Murphy and Campbell2013). WM volume maturation peaks in the early twenties (Mitelman et al. Reference Mitelman, Brickman, Shihabuddin, Newmark, Hazlett, Haznedar and Buchsbaum2007; Paus, Reference Paus2010), thus aside from differing in WM microstructure, genetic risk factors in 22q11DS seem associated with pathological total WM volume maturation on a whole, which is not evident in UHR patients.
Focally, 22q11DS patients have lower superior temporal gyrus volumes than UHR patients, although comparisons with HC show it is within the normal range. Equally UHR patients show no significant differences with HC in temporal WM volume (see Table 2). Thus 22q11DS patients seem relatively more predisposed to lower superior temporal lobe WM volume compared to UHR patients, although this effect is not large. Lower temporal WM volume has been related to increased psychotic symptom severity (Da Silva Alves et al. Reference Da Silva Alves, Schmitz, Bloemen, van der Meer, Meijer, Boot, Nederveen, de Haan, Linszen and van Amelsvoort2011) in 22q11DS; however, it is much better investigated in UHR patients. Longitudinal studies in UHR patients show progressively lower temporal WM volumes are associated with transition to psychosis (Walterfang et al. Reference Walterfang, McGuire, Yung, Phillips, Velakoulis, Wood, Suckling, Bullmore, Brewer, Soulsby, Desmond, McGorry and Pantelis2008; Sun et al. Reference Sun, Phillips, Velakoulis, Yung, McGorry, Wood, van Erp, Thompson, Toga, Cannon and Pantelis2009; Bloemen et al. Reference Bloemen, de Koning, Schmitz, Nieman, Becker, de Haan, Dingemans, Linszen and van Amelsvoort2010; Fusar-Poli et al. Reference Fusar-Poli, Crossley, Woolley, Carletti, Perez-Iglesias, Broome, Johns, Tabraham, Bramon and McGuire2011). Taken together, we find no evidence for specific genetic, or clinical risk factors related to regional reductions of temporal WM volume in adult patients. This structural change consistently reported in patients with psychotic disorders (Kubicki et al. Reference Kubicki, Carley and Shenton2012; Haijma et al. Reference Haijma, Van Haren, Cahn, Koolschijn, Hulshoff Pol and Kahn2013) may be more associated with a shared underlying process resulting in lower WM volumes, such as environmental risk factors for psychotic disorders, including urbanization, drug use, and/or social exclusion (van Os et al. Reference van Os, Rutten and Poulton2008; van Os & Kapur Reference van Os and Kapur2009).
The current study does identify lower cuneus and posterior cingulum WM volume to be related specifically to genetic risk factors in 22q11DS. Fronto-temporal dysconnectivity is a key finding in psychotic disorders and alterations in posterior cingulum connectivity may contribute to increased vulnerability to develop psychosis (Pettersson-Yeo et al. Reference Pettersson-Yeo, Allen, Benetti, McGuire and Mechelli2011). These findings are in line with other studies reporting abnormalities in posterior brain regions in 22q11DS (Boot & van Amelsvoort, Reference Boot and van Amelsvoort2012). Lower WM volumes of these regions have been associated with poor outcome in psychotic disorders (Mitelman et al. Reference Mitelman, Shihabuddin, Brickman, Hazlett and Buchsbaum2003), and have been implicated in cognitive pathology, consciousness, and reflections upon self (Cavanna & Trimble, Reference Cavanna and Trimble2006; Ghosh et al. Reference Ghosh, Basu, Kumaran and Khushu2010), symptoms also found in adults with 22q11DS (Henry et al. Reference Henry, van Amelsvoort, Morris, Owen, Murphy and Murphy2002).
Strengths and limitations
A major strength of the current study is that it investigates adult WM volume and microstructure in UHR and 22q11DS patients and HC subjects. This is relevant to understanding outcome of early WM microstructure and volume changes in 22q11DS and UHR as the brain matures, and whether these changes are related to genetic or clinical risk factors. In addition, we included large amount of age and gender matched patients and HC, and performed additional stringent statistical correction for age and gender. Differences in WM volumes may influence WM microstructure measures due to partial volume effects, therefore, we also corrected for brain segmentation volume differences between the subjects in the TBSS analysis. Last, the current study is a whole-brain TBSS assessment of FA, AD, RD and MD using 3-T field strength. The majority of studies are hypothesis-driven tractography assessments at lower field strengths giving lower spatial resolution.
A limitation of the current study, however, was the significant difference in positive symptom severity between UHR and 22q11DS patients, which may in part explain differences in microstructure indices reported. Importantly, the groups were matched on negative symptom severity. Current literature shows that negative, rather than positive symptom severity, predict transition to psychosis (Gee & Cannon, Reference Gee and Cannon2011; Díaz-Caneja et al. Reference Díaz-Caneja, Pina-Camacho, Rodríguez-Quiroga, Fraguas, Parellada and Arango2015). Thus, our results are not biased by a possibly more frequent transition to psychosis in either group. Additionally, differences in IQ might partially explain the differences between UHR and 22q11DS in WM. Moreover, much like other studies in the field, there was no follow up data to assert which role the genetic or clinically distinct changes play in the transition to psychosis.
Potentially the current results were also influenced by the clinical diversity and use of antipsychotic medication in the 22q11DS sample. Nevertheless, genetically this group is homogenous, and therefore, we expect the more substantial part of the reported variance in WM indices to be related to their micro deletion. In 22q11DS effects of antipsychotic treatment on WM microstructure have not yet been investigated longitudinally making it difficult to evaluate potential influence on the data. Moreover, findings in non-deleted first-episode psychosis patients on effects of antipsychotic medication on WM indices are somewhat inconclusive. One study has reported significantly reduced FA in the bilateral anterior cingulate and anterior corona radiate of the frontal lobe after 6 weeks of antipsychotic treatment (Wang et al. Reference Wang, Cheung, Deng, Li, Huang, Ma, Wang, Jiang, Sham, Collier, Gong, Chua, McAlonan and Li2013); however, these effects seemed to precipitate into more subtle effects of lower FA in the occipital and parietal tracts in patients being treated for a longer duration (Szeszko et al. Reference Szeszko, Robinson, Ikuta, Peters, Gallego, Kane and Malhotra2014). Our 22q11DS patients received antipsychotic treatment for a longer duration and therefore, our measures of FA and AD may be underestimated in these tracts in comparison to the antipsychotic free UHR subjects.
In conclusion, despite comparable heightened risk for developing a psychotic disorder, UHR and 22q11DS patients have specific differences in WM microstructure and volume. Our results suggest that different risk profiles may have different (micro)structural WM characteristics and that a separate endophenotype involving lower axonal integrity seems more plausible for the 22q11DS group, whereas involvement of myelin structures may be more prominent in the UHR group. Future longitudinal studies should aim to track changes in AD and RD in high-risk groups over time, and evaluate how these changes relate to the development of psychosis.
Acknowledgements
This work was supported by the European Commission in Brussels, Belgium (QLGU-CT-2001–01081), by the Dutch Organisation for Health Research and Development (ZonMw) and by personal grants to Thérèse van Amelsvoort [NWO-Veni grant 2006 (916.76.048)] and the Dutch Brain Foundation [grant number 15F07(2).55]. The funders had no role in study design, data collection and analysis, decision to publish, or preparation of the manuscript. The Dutch e-Science Grid is supported by the SURF Foundation.
Declaration of Interest
None.