1. Introduction
Tectonic movement and crust–mantle interactions are most intense in subduction zones on the Earth. Subduction-related igneous rocks, as the most striking characteristics of orogenic systems, hold a key to monitoring important geodynamic processes within orogenic belts (Xiao et al. Reference Xiao, Windley, Hao and Zhai2003; Annen et al. Reference Annen, Blundy and Sparks2006; Li, Reference Li2006; Wu et al. Reference Wu, Sun, Ge, Zhang, Grant, Wilde and Jahn2011; Wilde, Reference Wilde2015). The Central Asian Orogenic Belt (CAOB) is regarded as one of the largest and most complicated Phanerozoic accretionary orogenic belts on the Earth, which extends thousands of kilometres from the Urals in the west, through Kazakhstan, northern China and Mongolia to the Okhotsk Sea in the east (Sengör et al. Reference Sengör, Natal’in and Burtman1993; Xiao et al. Reference Xiao, Windley, Hao and Zhai2003; Li, Reference Li2006; Zhou, J. B. et al. Reference Zhou, Wilde, Zhao, Zhang, Wang and Zeng2010, Reference Zhou, Wilde, Zhao and Han2018; Wilde, Reference Wilde2015; Eizenhöfer & Zhao, Reference Eizenhöfer and Zhao2018; Chai et al. Reference Chai, Ma, Santosh, Hao, Luo, Fan, Gao, Zong, Mao and Wang2020; Li et al. Reference Li, Yu, Guo and Xu2021; Liu et al. Reference Liu, Li, Ma, Feng, Guan, Li, Chen, Liang and Wen2021; Hui et al. Reference Hui, Zhang, Zhang, Qu, Zhang, Zhao and Niu2021). Previous studies have revealed that the geodynamic evolution of the CAOB is controlled by subduction, accretion, and closure processes of the Palaeo-Asian Ocean, involving a series of ophiolites, accretionary wedges, accretionary complexes, magmatic arcs, oceanic plateaus and micro-continental blocks (Fig. 1a; Sengör et al. Reference Sengör, Natal’in and Burtman1993; Jahn et al. Reference Jahn, Wu and Chen2000; Badarch et al. Reference Badarch, Cunningham and Windley2002; Windley et al. Reference Windley, Alexeiev, Xiao, Kröner and Badarch2007; Zhou et al. Reference Zhou, Wilde, Zhao, Zhang, Wang and Zeng2010; Wu et al. Reference Wu, Sun, Ge, Zhang, Grant, Wilde and Jahn2011; Xiao et al. Reference Xiao, Windley, Sun, Li, Huang, Han, Yuan, Sun and Chen2015; Wilde, Reference Wilde2015; Eizenhöfer & Zhao, Reference Eizenhöfer and Zhao2018; Chai et al. Reference Chai, Ma, Santosh, Hao, Luo, Fan, Gao, Zong, Mao and Wang2020; Hui et al. Reference Hui, Zhang, Zhang, Qu, Zhang, Zhao and Niu2021; Yang et al. Reference Yang, Li, Tong, Wang, Si, Lindagato and Zeng2022). Along with the consumption and final closure of the Palaeo-Asian Ocean, the CAOB was ultimately assembled along the northern margin of the Tarim and North China cratons (Xiao et al. Reference Xiao, Windley, Sun, Li, Huang, Han, Yuan, Sun and Chen2015; Eizenhöfer & Zhao, Reference Eizenhöfer and Zhao2018; Hui et al. Reference Hui, Zhang, Zhang, Qu, Zhang, Zhao and Niu2021). It is widely accepted that the final closure of the eastern Palaeo-Asian Ocean is recorded by the Solonker–Changchun suture zone, separating the North China Craton (NCC) to the south from the amalgamated blocks of northeast (NE) China to the north (Xiao et al. Reference Xiao, Windley, Hao and Zhai2003; Li, Reference Li2006; Zhou, J. B. et al. Reference Zhou, Wilde, Zhao, Zhang, Wang and Zeng2010, Reference Zhou, Wilde, Zhao and Han2018; Wilde, Reference Wilde2015; Eizenhöfer & Zhao, Reference Eizenhöfer and Zhao2018; Chai et al. Reference Chai, Ma, Santosh, Hao, Luo, Fan, Gao, Zong, Mao and Wang2020; Liu et al. Reference Liu, Li, Ma, Feng, Guan, Li, Chen, Liang and Wen2021). Since typical collision-related features do not develop, the detailed geodynamic processes involved in the final closure are controversial and ocean closure time is uncertain, with it spanning from Devonian to Middle Triassic times. The first model argues that the final closure of the eastern Palaeo-Asian Ocean occurred during early to middle Devonian time by bi-directional subduction, based on the Baolidao and Bainaimiao arc-accretion complexes in southern Mongolia and central Inner Mongolia, respectively (Xu et al. Reference Xu, Charvet, Chen, Zhao and Shi2013). The Carboniferous and Permian–Triassic magmatic rocks along the Solonker–Changchun suture zone are interpreted to have formed in an extensional tectonic environment in response to post-orogenic or mantle plume-related geologic events (Xu et al. Reference Xu, Charvet, Chen, Zhao and Shi2013). The second model proposes that the final ocean closure took place prior to early Permian time (Zhao et al. Reference Zhao, Appel, Xu and Sukhbaatar2020), which resulted from palaeomagnetic data from the early Permian volcanic strata in the Bayandun region of northeastern Mongolia. The third model advocates that the final closure of the eastern Palaeo-Asian Ocean occurred in latest Permian or earliest Middle Triassic time along the Solonker–Changchun suture zone, following a prolonged history of subduction and accretion (Xiao et al. Reference Xiao, Windley, Hao and Zhai2003; Li, Reference Li2006; Wu et al. Reference Wu, Zhao, Sun, Wilde and Yang2007, Reference Wu, Sun, Ge, Zhang, Grant, Wilde and Jahn2011; Cao et al. Reference Cao, Xu, Pei and Zhang2011, Reference Cao, Xu, Pei, Wang, Wang and Wang2013; Yu et al. Reference Yu, Ge, Yang, Zhao, Zhang and Su2014; Eizenhöfer et al. Reference Eizenhöfer, Zhao, Zhang and Sun2014; Yuan et al. Reference Yuan, Zhang, Xue, Lu and Zong2016; Guo et al. Reference Guo, Li, Fan, Li, Zhao and Huang2016; Liu, J. et al. Reference Liu, Liu, Li, Zhao, Wang, Peng, Yang and Dou2016, Reference Liu, Wang, Liu, Liu and Lai2020; Huang et al. Reference Huang, Yan, Piper, Zhang, Yi, Yu and Zhou2018; Song et al. Reference Song, Han, Gao, Geng, Li, Meng, Han, Zhong, Li, Du, Yan and Liu2018; Eizenhöfer & Zhao, Reference Eizenhöfer and Zhao2018; Imayama et al. Reference Imayama, Koh, Aoki, Saneyoshi, Yagi, Aoki, Terada, Sawada, Ikawa, Ishigaki, Toyoda, Tsogtbaatar and Mainbayar2019; Jing et al. Reference Jing, Ge, Dong, Yang, Ji, Bi, Zhou and Xing2020 a,b; Liu et al. Reference Liu, Li, Ma, Feng, Guan, Li, Chen, Liang and Wen2021). Global plate reconstructions for the Triassic to present conducted by Müller et al. (Reference Müller, Zahirovic, Williams, Cannon, Seton, Bower, Tetley, Heine, Le Breton, Liu, Russell, Yang, Leonard and Gurnis2019) also prefer a late Permian–Triassic closure of the Palaeo-Asian Ocean. These continued accretion and collision processes resulted in the development of late Carboniferous – Early Triassic E–W-trending calc-alkaline magmatism and minor high-alkali arc-related volcanic rocks on both sides of the Solonker–Changchun suture zone (Fig. 1b; online Supplementary Material Table S1; Wu et al. Reference Wu, Sun, Ge, Zhang, Grant, Wilde and Jahn2011; Li et al. Reference Li, Wilde, He, Jiang, Liu and Zhao2014, Reference Li, Wilde, Wang, Xiao and Guo2016, Reference Li, Yu, Guo and Xu2021; Imayama et al. Reference Imayama, Koh, Aoki, Saneyoshi, Yagi, Aoki, Terada, Sawada, Ikawa, Ishigaki, Toyoda, Tsogtbaatar and Mainbayar2019; Liu, J. et al. Reference Liu, Wang, Liu, Liu and Lai2020; Jing et al. Reference Jing, Ge, Dong, Yang, Ji, Bi, Zhou and Xing2020 a,b; Hui et al. Reference Hui, Zhang, Zhang, Qu, Zhang, Zhao and Niu2021). The widespread latest late Permian to Middle Triassic adakitic magmatism in the southeastern CAOB marked the final suturing of the Palaeo-Asian Ocean (Li et al. Reference Li, Wilde, Wang, Xiao and Guo2016), with resultant thickening of the lower crust up to ∼59 km (Hui et al. Reference Hui, Zhang, Zhang, Qu, Zhang, Zhao and Niu2021). In addition, Wang et al. (Reference Wang, Xing, Xu, Teng, Xu and Yang2021) further proposed another early–middle Permian ridge subduction model based on the formation of multi-type ‘abnormal’ igneous rocks (282–268 Ma) along the western margin of the Khanka Block. The high heat condition is recorded by the decomposition reactions of magnesite constraints of Mg isotopes and high zircon saturation temperatures of felsic rocks (855–935 °C).
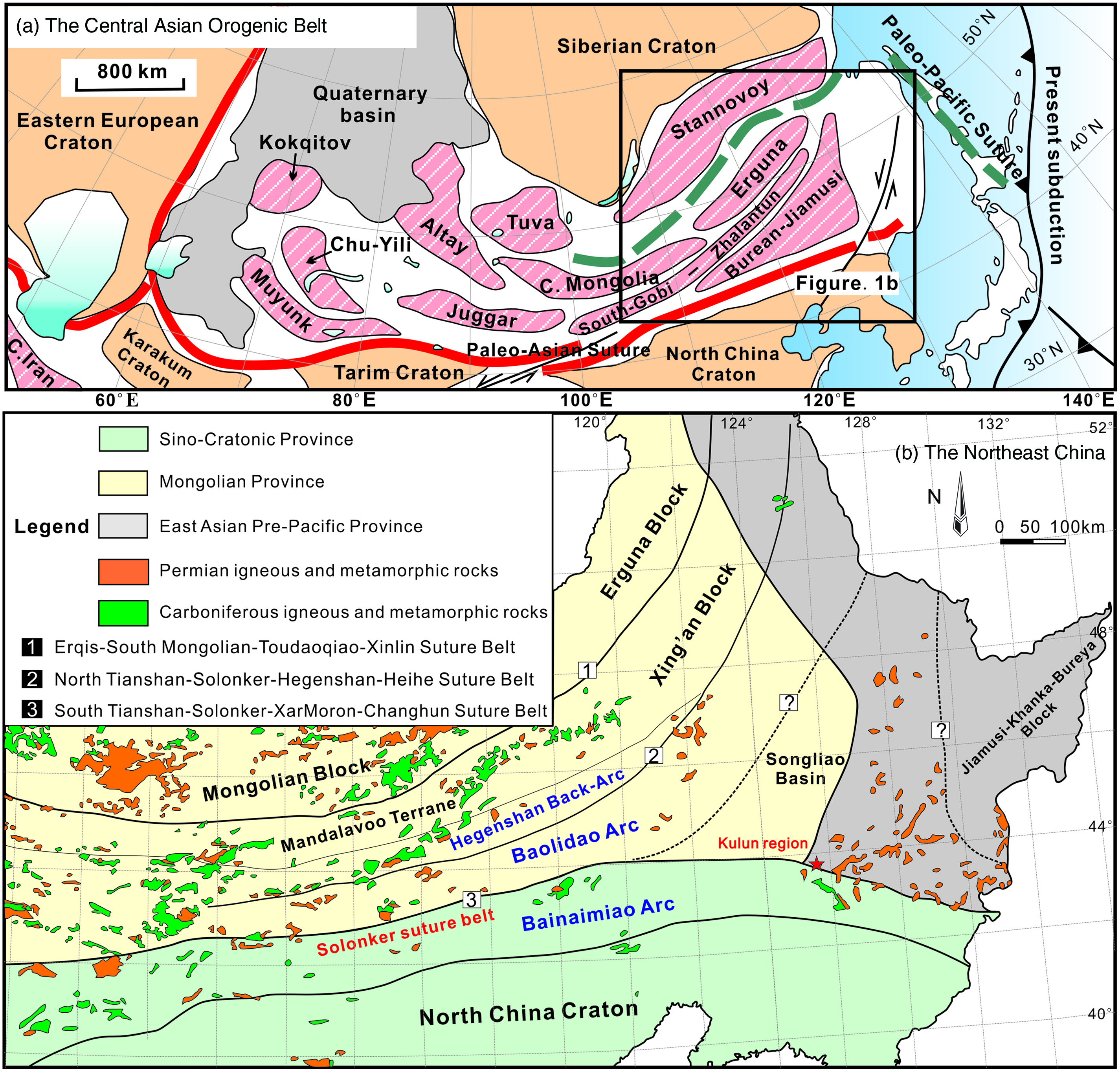
Fig. 1. (a) Tectonic sketch map of the southeastern Central Asian Orogenic Belt (modified after Li, Reference Li2006). (b) Geological sketch map of NE China (modified after Eizenhöfer & Zhao, Reference Eizenhöfer and Zhao2018).
The Kulun region of eastern Inner Mongolia is located in the junction between the southeastern margin of the CAOB and the northern margin of the NCC. During final-stage evolution of the eastern Palaeo-Asian Ocean, subduction, accretion, final closure or/and collision triggered a suites of tectono-magmatic events and relevant crust–mantle interactions, which were well documented in magmatic rocks. Thus, understanding the petrogenesis of late Palaeozoic igneous rocks occurring in the northern margin of the NCC is pivotal to determining the amalgamation processes of the CAOB and the subduction to final closure history of the eastern Palaeo-Asian Ocean. Previous studies have shown that felsic rocks are widely distributed along the Solonker–Changchun suture zone (Zhang, X. H. et al. Reference Zhang, Gao, Ge, Wu, Yang, Wilde and Li2010; Liu, J. et al. Reference Liu, Liu, Li, Zhao, Wang, Peng, Yang and Dou2016, Reference Liu, Wang, Liu, Liu and Lai2020; Jing et al. Reference Jing, Ge, Dong, Yang, Ji, Bi, Zhou and Xing2020 a,b). Understanding the emplacement history of these granitic rocks and their volcanic equivalents is essential for establishing the magmatic and geodynamic evolution of the southeastern CAOB (Cao et al. Reference Cao, Xu, Pei and Zhang2011, Reference Cao, Xu, Pei, Wang, Wang and Wang2013; Yu et al. Reference Yu, Ge, Yang, Zhao, Zhang and Su2014; Li et al. Reference Li, Wilde, He, Jiang, Liu and Zhao2014, Reference Li, Wilde, Wang, Xiao and Guo2016; Liu, J. et al. Reference Liu, Liu, Li, Zhao, Wang, Peng, Yang and Dou2016, Reference Liu, Wang, Liu, Liu and Lai2020; Song et al. Reference Song, Han, Gao, Geng, Li, Meng, Han, Zhong, Li, Du, Yan and Liu2018; Jing et al. Reference Jing, Ge, Dong, Yang, Ji, Bi, Zhou and Xing2020 a,b; Li et al. Reference Li, Yu, Guo and Xu2021; Hui et al. Reference Hui, Zhang, Zhang, Qu, Zhang, Zhao and Niu2021). This is especially true for the Kulun region, which is distinguished by voluminous intermediate-acidic igneous rocks. Field observations of these magmatic rocks have identified a suite of andesites, rhyolites and diorite porphyrites. From previous studies, the late Palaeozoic magmatic rocks distributed along the Solonker–Changchun suture zone are mostly calc-alkaline; however, potassic rhyolites with distinctive geochemical and isotopic compositions have now been identified in the Kulun region (Fig. 2). Petrogenetic models proposed for these rhyolites with potassic affinity, although somewhat unclear and controversial, mainly involve the partial melting of crustal materials in active magmatic arc and continental intraplate environments. Identifying and characterizing the late Palaeozoic magmatic rocks in the Kulun region might provide insights into the geodynamic evolution of the eastern Palaeo-Asian Ocean.

Fig. 2. Geological sketch map and distribution of the late Palaeozoic magmatic rocks along the Solonker–Changchun suture zone. Numbers show all these reported age locations mentioned in online Supplementary Material Table S1.
In this contribution, zircon U–Pb analyses and whole-rock elemental and in situ zircon Hf isotopic compositions are presented for the late Palaeozoic igneous rocks of the Kulun region of the Solonker–Changchun suture zone. The aim of the study is to establish the emplacement ages, petrogenesis and source characteristics of these igneous rocks, thus elucidating subduction, accretion and reworking processes of the continental crust during late Palaeozoic time.
2. Geological background and sample descriptions
The formation of the Palaeo-Asian Ocean commenced in early Neoproterozoic time, following the break-up of the Rodinia supercontinent (Khain et al. Reference Khain, Bibikova, Salnikova, Kröner, Gibsher, Didenko, Degtyarev and Fedotova2003; Cawood et al. Reference Cawood, Wang, Xu and Zhao2013). The consumption, accretion and final closure of the Palaeo-Asian Ocean led to the formation of the CAOB, including the amalgamations of the North China and Tarim cratons to the south, the East European and Siberian cratons to the north, and micro-continental blocks, island arcs and accretionary wedge (Sengör et al. Reference Sengör, Natal’in and Burtman1993; Jahn et al. Reference Jahn, Wu and Chen2000; Li, Reference Li2006; Zhou, J. B. et al. Reference Zhou, Wilde, Zhao, Zhang, Wang and Zeng2010, Reference Zhou, Wilde, Zhao and Han2018; Wilde, Reference Wilde2015; Eizenhöfer & Zhao, Reference Eizenhöfer and Zhao2018; Chai et al. Reference Chai, Ma, Santosh, Hao, Luo, Fan, Gao, Zong, Mao and Wang2020; Li et al. Reference Li, Yu, Guo and Xu2021; Liu et al. Reference Liu, Li, Ma, Feng, Guan, Li, Chen, Liang and Wen2021; Hui et al. Reference Hui, Zhang, Zhang, Qu, Zhang, Zhao and Niu2021; Wu et al. Reference Wu, Li, Chew, Liu and Guo2021). The NE China region in the southeastern segment of the CAOB (Fig. 1a, b; Xiao et al. Reference Xiao, Windley, Hao and Zhai2003; Li, Reference Li2006) had experienced complicated assembling processes of several microcontinents, involving the Jiamusi Block in the east, the Xing’an and Songnen–Zhangguangcai blocks in central parts, and the Erguna Block in the northwest (Xiao et al. Reference Xiao, Windley, Hao and Zhai2003, Reference Xiao, Windley, Huang, Han, Yuan, Chen, Sun, Sun and Li2009; Li, Reference Li2006; Zhou, J. B. et al. Reference Zhou, Wilde, Zhao, Zhang, Wang and Zeng2010, Reference Zhou, Wilde, Zhao and Han2018; Wilde, Reference Wilde2015). The Erguna Block is proposed to have collided with the Xing’an Block along the Tayuan–Xiguitu suture zone during the early Palaeozoic period, forming the united Erguna–Xing’an block (Ge et al. Reference Ge, Wu, Zhou and Zhang2005). This composite block then collided with the Songnen–Zhangguangcai Range Block along the Hegenshan–Heihe suture zone, although the timing of this collision event remains controversial (Badarch et al. Reference Badarch, Cunningham and Windley2002; Li, Reference Li2006; Zhou, J. B. et al. Reference Zhou, Wilde, Zhao, Zhang, Wang and Zeng2010, Reference Zhou, Wilde, Zhao and Han2018). Previous researches focusing on the Hegenshan–Heihe suture indicated that the final collision occurred in middle Palaeozoic time, followed by lithospheric extension of the northwestern Songnen–Zhangguangcai Block in the late Palaeozoic period (Bao et al. Reference Bao, Chen and Zhang1994; Robinson et al. Reference Robinson, Zhou, Hu, Reynolds, Wenji and Yang1999; Nozaka & Liu, Reference Nozaka and Liu2002). Recent studies, however, have proposed that the final collision did not occur prior to early Permian time, but instead in latest Permian or earliest Triassic time (Miao et al. Reference Miao, Fan, Liu, Zhang, Shi and Guo2008; Yu et al. Reference Yu, Ge, Zhang, Zhao, Zhang and Yang2017). Furthermore, the amalgamation of the Songnen–Zhangguangcai and Jiamusi blocks is evidenced by the Heilongjiang Complex, a N–S belt extending from Luobei through Yilan to Mudanjiang in Heilongjiang Province (Dong et al. Reference Dong, Ge, Yang, Ji, He, Zhao and Xu2018). The final amalgamation of these two eastern units mainly occurred during earliest Cretaceous time by the divergent double subduction of the Mudanjiang oceanic plate (Dong et al. Reference Dong, Ge, Yang, Ji, He, Zhao and Xu2018). The Nadanhada Terrane is generally regarded as an accretionary complex of the Mino–Sikhote Alin terrane related to low-latitude subduction of the Palaeo-Pacific Plate (Wilde, Reference Wilde2015; Zhou, J. B. et al. Reference Zhou, Wilde, Zhao and Han2018; Lan et al. Reference Lan, Li, Guo, Li, Liu, Liu, Santosh, Cao, Zhang and Wang2022).
The late Palaeozoic igneous rock samples analysed in this study were previously assigned to the Ordovician Kulun Formation (Fig. 3), and comprise mainly andesites, rhyolites, and diorite porphyrites. A nearly 8 m wide diorite porphyrite occurring in the Kulun region is shown in Figure 4a, b. The diorite porphyrite samples have a porphyritic texture and massive structure, and contain phenocrysts (∼15 vol. %) of plagioclase, quartz, and alkali feldspar, with accessory minerals of titanite, apatite, and zircon. The groundmass has a cryptocrystalline structure and mineral components similar to the phenocrysts (Fig. 4c, d). The andesite is grey-white and displays a porphyritic texture and massive structure, with phenocrysts (∼8 vol. %) of plagioclase and minor amphibole (Fig. 4e, f). The rhyolite occurs in layers in the field outcrops (Fig. 4g) and has a porphyritic texture (Fig. 4h), consisting of phenocrysts (∼10 vol. %) of alkali feldspar and quartz, with a felsic groundmass (Fig. 4i, j). The groundmass is dominated by plagioclase and cryptocrystalline materials (Fig. 4i, j). Furthermore, there are also extensive pyroclastic rocks in the field outcrops of the Kulun region (Fig. 4k, l).

Fig. 3. Geological sketch map of the Kulun region, with the sample locations shown.

Fig. 4. Representative field outcrops and photomicrographs of the late Palaeozoic magmatic rocks in the Kulun region. Pen for scale is 13.5 cm long. Coin for scale is 2.5 cm diameter.
3. analytical methods
The measurement of U–Th–Pb isotopes for the volcanic rock samples (17KL14, 17KL19 and 17KL24) and diorite porphyrite sample (16KL012) were carried out by laser ablation inductively coupled plasma mass spectrometry (LA-ICP-MS). Geochemical analyses for the diorite porphyrite samples and volcanic rock samples were conducted by X-ray fluorescence (XRF). Hf isotope analyses for all these samples were performed by multi-collector (MC)-ICP-MS (Fig. 5). More detailed descriptions of the analytical procedures are presented in online Supplementary Material S1.

Fig. 5. Plots of (a) total alkali versus SiO2 diagram (TAS, Peccerillo & Taylor, Reference Peccerillo and Taylor1976); (b) SiO2 versus Zr/TiO2 *0.0001 (Winchester & Floyd, Reference Winchester and Floyd1977); (c) A/NK (molar ratio Al2O3/(Na2O + K2O)) versus A/CNK (molar ratio Al2O3/(CaO + Na2O + K2O)) (Maniar & Piccoli, Reference Maniar and Piccoli1989); (d) K2O versus SiO2 (Peccerillo & Taylor, Reference Peccerillo and Taylor1976) for the late Palaeozoic magmatic rocks in the Kulun region along the Solonker–Changchun suture zone. The data for Permian granites along the Solonker–Changchun suture zone are from Jing et al. (Reference Jing, Ge, Dong, Yang, Ji, Bi, Zhou and Xing2020 a,b and references therein). Typical potassic rhyolite data are from Samuel et al. (Reference Samuel, Moussa and Azer2007), Hu et al. (Reference Hu, Li, Wang, Xie and Wu2013), Ding et al. (Reference Ding, Zhang, Dong, Yan, Lin and Jiang2015) and Chen et al. (Reference Chen, Shi, Fan, Zou, Liu, Huang, Gong, Yi and Wu2016).
4. Analytical results
4.a. Zircon U–Pb dating and in situ zircon Hf isotope analyses
Four representative diorite porphyrite, andesite, and rhyolite samples were selected for zircon U–Pb and Lu–Hf analyses, with the data presented in online Supplementary Material Tables S2 and S3. All these selected zircons have relatively high Th/U ratios (online Supplementary Material Table S2), implying a magmatic origin (Koschek, Reference Koschek1993). The cathodoluminescence (CL) images of zircons from the late Palaeozoic magmatic rocks and associated concordia plots are presented in Figures 6 and 7. It is worth noting that some discordant U–Pb zircon ages show a higher 207Pb/235U ratio, which was probably affected by radioactive Pb-loss in zircon.

Fig. 6. Cathodoluminescence (CL) images of zircons from the late Palaeozoic magmatic rocks in the Kulun region, with U–Pb analytical spots indicated by red circles.

Fig. 7. U–Pb concordia diagrams showing zircon ages obtained by LA-ICP-MS for the late Palaeozoic magmatic rocks in the Kulun region, with weighted mean ages and MSWD shown in each figure. The red ellipses represent age points which are used for the calculation of weighted mean age, whereas the white ellipses represent age points for captured zircons.
Sample 16KL012 is diorite porphyrite collected from the northern quarry in the Kulun region. The 206Pb–238U ages of 20 analytical points range from 1946 Ma to 279 Ma (online Supplementary Material Table S2), and yield a weighted mean 206Pb–238U age of 281.0 ± 1.7 Ma (n = 10; MSWD = 1.3) (Fig. 7a), which is considered the crystallization age of the diorite porphyrite. The older zircons varying from 1946 to 296 Ma are considered to be captured zircons. The 403–296 Ma zircons probably come from earlier Carboniferous to Devonian magmatic rocks, whereas the 1946–1876 Ma zircons are more likely from the ancient NCC. The 176Hf/177Hf ratios of ten zircon grains from the early Permian (∼281 Ma) diorite porphyrite vary from 0.282529 to 0.282880. Their ϵ Hf(t) values and T DM2 ages range from –2.6 to +9.7 and from 1466 to 684 Ma, respectively (Fig. 8a, b; online Supplementary Material Table S3).

Fig. 8. (a) ϵHf(t) versus T (Ma) diagram for zircons from the late Palaeozoic magmatic rocks in the Kulun region. CAOB – Central Asian Orogenic Belt; YFTB – Yanshan Fold-and-Thrust Belt (Yang et al. Reference Yang, Wu, Shao, Wilde, Xie and Liu2006). (b) Detailed distribution of samples on ϵHf(t) versus T (Ma) diagram. Hf isotopic data for the Permian granites along the Solonker–Changchun suture zone are from Jing et al. (Reference Jing, Ge, Dong, Yang, Ji, Bi, Zhou and Xing2020 a,b and references therein).
Sample 17KL14 is andesite collected from the northern quarry in the Kulun region. LA-ICP-MS zircon U–Pb analysis results show the 206Pb–238U ages of 23 analytical points vary from 2149 to 275 Ma (online Supplementary Material Table S2). Only three zircon results yield 206Pb–238U ages of 276 ± 5 Ma, 275 ± 5 Ma and 275 ± 5 Ma (Fig. 7b), which are interpreted as the crystallization age of the andesite (ca. 276 Ma). The other zircons ranging from 376 to 301 Ma and 2149 to 1814 Ma are considered to be captured zircons, which probably come from the earlier Carboniferous magmatic rocks and southern ancient NCC.
Sample 17KL19 is rhyolite collected from the western quarry in the Kulun region. The 206Pb–238U ages for 23 analytical points are in the range of 1910 to 271 Ma (online Supplementary Material Table S2), and yield a weighted mean 206Pb–238U age of 274.8 ± 0.9 Ma (n = 22; MSWD = 0.54; Fig. 7c). This weighted mean age represents the crystallization time of the rhyolite. The older c. 1910 Ma zircon is probably from the ancient NCC. The 176Hf/177Hf ratios of 18 zircon grains from the early Permian (∼275 Ma) rhyolite vary from 0.282727 to 0.282786. Their ϵ Hf(t) values and T DM2 ages vary from –1.6 to +0.5 and from 1683 to 1494 Ma, respectively (Fig. 8a, b; online Supplementary Material Table S3).
Sample 17KL24 is rhyolite collected from the western quarry in the Kulun region. Twenty-five spots conducted on 25 zircon grains produced 206Pb–238U ages varying from 2465 Ma to 271 Ma (online Supplementary Material Table S2), and yielded a weighted mean 206Pb–238U age of 274.9 ± 0.8 Ma (n = 24, MSWD = 0.35) (Fig. 7d), which represents the time of volcanic eruption. The other zircon with a 206Pb–238U age of 2465 ± 27 Ma is regarded as a captured zircon from the southern NCC. The 176Hf/177Hf ratios for 18 zircon grains from the early Permian (∼275 Ma) rhyolite range from 0.282024 to 0.282361. Their ϵ Hf(t) values and T DM2 ages vary from –20.8 to –9.0 and from 3578 to 2524 Ma, respectively (Fig. 8a, b; online Supplementary Material Table S3).
4.b. Geochemistry
4.b.1. Andesites of the Kulun Formation
The analysed andesites of the Kulun Formation have SiO2 = 58.80–62.36 wt %, MgO = 1.44–1.51 wt %, Al2O3 = 14.03–17.10 wt % and TiO2 = 0.64–0.97 wt % contents, with aluminium saturation index (A/CNK = molecular Al2O3/(CaO + Na2O + K2O)) values of 0.45 to 1.15 (Fig. 5c; online Supplementary Material Table S4). The total rare earth element (∑REE) contents of these andesites are 120.0–187.6 ppm, with (La/Yb)N and δEu values ranging from 5.68 to 9.53, and 0.68 to 0.76, respectively (Fig. 9b; online Supplementary Material Table S4). Furthermore, they are also enriched in large ion lithophile elements (LILEs) (Rb, Ba and K) and depleted in high-field-strength elements (HFSEs) (Nb, Ta and Ti) in the primitive mantle-normalized trace-element diagrams (Fig. 9a).

Fig. 9. (a, c) Primitive mantle-normalized trace-element patterns and (b, d) chondrite-normalized REE patterns for the late Palaeozoic magmatic rocks in the Kulun region along the Solonker–Changchun suture zone. Primitive mantle-normalized and chondrite-normalized values are from Sun & McDonough (Reference Sun, McDonough, Saunders and Norry1989). The data for Permian granites along the Solonker–Changchun suture zone are from Jing et al. (Reference Jing, Ge, Dong, Yang, Ji, Bi, Zhou and Xing2020 a,b and references therein). Typical potassic rhyolite data are from Samuel et al. (Reference Samuel, Moussa and Azer2007), Hu et al. (Reference Hu, Li, Wang, Xie and Wu2013), Ding et al. (Reference Ding, Zhang, Dong, Yan, Lin and Jiang2015) and Chen et al. (Reference Chen, Shi, Fan, Zou, Liu, Huang, Gong, Yi and Wu2016).
4.b.2. Rhyolites of the Kulun Formation
The rhyolites presented in this study have variable compositions with SiO2 = 65.42–76.22 wt %, Al2O3 = 12.47–23.56 wt % and high K2O (4.18–6.69 wt %), low MgO (0.36–0.44 wt %) and very low Na2O (0.20–0.39 wt %) contents (online Supplementary Material Table S4). All these rhyolite samples are calc-alkaline, and plot in the field of rhyolite on the total alkali (K2O + Na2O) versus SiO2 diagram (TAS; Fig. 5a). Our studied rhyolites show obviously high K2O/Na2O ratios (17.16–22.93), and are classified as potassic rhyolites according to the Na2O versus K2O diagrams proposed by Foley et al. (Reference Foley, Venturelli, Green and Toscani1987). The potassium-enriched characteristic is also supported by their relatively high Th (13.6–32.5 ppm) and low Co (1.7–2.1 ppm) contents (online Supplementary Material Table S4; Hastie et al. Reference Hastie, Kerr, Pearce and Mitchell2007). Furthermore, these rhyolite samples show distinctly elevated Al2O3 contents (up to 23.56 wt %) and show a strongly peraluminous affinity, with A/CNK values ranging from 2.34 to 2.96 (Fig. 5c). These rhyolite samples are enriched in light rare earth elements (LREEs) and have relatively variable ∑REE contents (99.3–332.5 ppm), with (La/Yb)N ratios and δEu values of 5.66–12.39 and 0.46–0.76, respectively (Fig. 9d). In the primitive mantle-normalized trace-element diagrams, the rhyolite samples show coherent patterns, with depletions in Ba, Nb, Ta, Sr, P and Ti, and enrichments in Rb, Th, U, K, Pb, Zr and Hf (Fig. 9c).
4.b.3. Diorite porphyrites
The analysed diorite porphyrites have SiO2 = 56.87–57.91 wt %, MgO = 1.93–2.11 wt %, Al2O3 = 13.77–13.96 wt % and K2O + Na2O = 4.85–4.94 wt %. These rocks show metaluminous affinities, with A/CNK values ranging from 0.39 to 0.42 (Fig. 5c; online Supplementary Material Table S4). In the primitive mantle-normalized patterns, these diorite porphyrite samples are characterized by depletion in HFSEs (e.g. Nb, Ta and Ti) and Ba and Sr, and enrichment in LILEs (e.g. Rb, Th, U and K; Fig. 9a), with negative Eu anomalies (δEu = 0.61–0.65; Fig. 9b).
5. Discussion
5.a. Petrogenesis of the early–middle Permian magmatic suites in the Kulun region
On the basis of petrographic and geochemical studies, the studied early Permian magmatic suites in the Kulun region may be divided into two categories: (1) calc-alkaline andesites and diorite porphyrites; and (2) rhyolites with potassic affinity.
5.a.1. Early Permian andesites and diorite porphyrites
Several models have been proposed to explain the petrogenesis of andesites and diorite porphyrites, including: (1) partial melting of mantle wedge peridotites metasomatized by subduction-related fluids or/and melts (Deng et al. Reference Deng, Liu, Feng, Xiao, Su, Zhao, Kong and Cao2010); (2) fractional crystallization of mantle-derived basaltic magmas (Maury et al. Reference Maury, Andriambololona and Dupuy1978; Zhang, J. H. et al. Reference Zhang, Zhang, Wilde, Yang and Chen2010); (3) mixing of mantle- and crust-derived magmas or crustal contamination processes (Cantagrel et al. Reference Cantagrel, Didier and Gourgaud1984); and (4) partial melting of mafic lower crust (Jung et al. Reference Jung, Hoernes and Mezger2002).
The early Permian andesites and diorite porphyrites investigated in this study contain high SiO2 and total alkali (Na2O + K2O) contents, with prominently low contents of MgO and transition metal elements (e.g. Cr, Co and Ni) and Mg no. values, indicating that their primitive magmas were unlikely to directly generate by partial melting of primitive mantle (Sun & McDonough, Reference Sun, McDonough, Saunders and Norry1989). This is because that partial melting of such mantle sources at a diverse range of pressures and degrees of partial melting might only generate mafic to high-Mg intermediate melts (Hofmann, Reference Hofmann1988). Furthermore, if these andesites and diorite porphyrites presented in this study were formed through fractional crystallization of mantle-derived basaltic magmas, then the volume of the exposed basaltic materials in the Kulun region would greatly exceed that of the intermediate rocks; however, this is not the case. Based on the relatively narrow ranges of concentrations of TiO2 (0.64–0.97 wt %), MgO (1.44–2.11 wt %) and Fe2O3T (4.36–5.99 wt %), the small plotting areas (TAS diagram; Fig. 5a) and the lack of significantly negative Eu anomalies (δEu = 0.61–0.76), fractional crystallization did not play a crucial role during their petrogenesis. Mixing between mantle- and crust-derived magmas or crustal contamination processes could explain the origin of the andesites and diorite porphyrites. Captured zircon from the andesites and diorite porphyrites could support this model. The mixing hypothesis was excluded, however, based on the following evidence: (1) mafic microgranular enclaves and chemical variations resulted from mixing mechanisms are absent; (2) on the La/Sm versus SiO2 and Th/La versus SiO2 plots, the SiO2 contents show no correlation with La/Sm or Th/La ratios, indicating that crustal contamination was limited during the magmatic evolution (online Supplementary Material Fig. S1); and (3) Zr and Hf, which are typically enriched during crustal contamination, are absent. The minor contamination indicated by the captured zircons might be explained by the mixing of zircons concentrated in some sedimentary rocks.
We consider that the last model based on partial melting of mafic lower crustal materials best reflects the petrogenesis of the andesites and diorite porphyrites. As mentioned above, the rocks are classified as high-K to medium-K calc-alkaline series, and characterized by enrichments in LREEs and LILEs, and depletions in HFSEs and heavy rare earth elements (HREEs). Their low MgO contents, Mg no. values, low Cr and Ni concentrations, together with similar REE and trace-element patterns to those of bulk continental crust, thus exhibit a crustal affinity (Fig. 9a, b; Rudnick & Gao, Reference Rudnick, Gao and Rudnick2003). Furthermore, the Rb/Sr, Ti/Y and Ti/Zr ratios of these rocks vary from 0.26 to 0.46, 141.19 to 248.14, and 15.13 to 19.33, respectively, and all these ratios are representative of crustal values, implying that their primitive magmas were originated from the partial melting of lower crustal materials (Rudnick, Reference Rudnick1995; Sun & McDonough, Reference Sun, McDonough, Saunders and Norry1989). The andesites and diorite porphyrites have relatively high HREEs (Y > 22.9 ppm), which together with their relatively flat HREE patterns (Fig. 9b), preclude the presence of garnet as a residual phase in their source. They also have relatively low Sr contents of <400 ppm (292.4–390.4 ppm), elevated Yb contents (2.6–2.9 ppm) and weakly negative Eu anomalies (0.61–0.76), suggesting an affinity with low-Sr and high-Yb type rocks as defined by Zhang et al. (Reference Zhang, Wang, Li, Wang and Jia2006). These characteristics, together with their weak concave-upward shape of middle REEs and negative Eu anomalies (Fig. 9a, b), indicate amphibolite as a residual facies in the magma source. Experimental petrology has shown that if hornblende is the main residual phase, the corresponding temperature and pressure conditions during partial melting are 10∼12.5 kbar and 800∼950 °C (i.e. crustal depth is 30∼40 km). Thus, the generation of intermediate igneous rocks in the Kulun region requires relatively low-pressure partial melting of amphibolitic lower crust at a crustal depth of 30–40 km (Qian & Hermann, Reference Qian and Hermann2013). Furthermore, zircons from the andesites and diorite porphyrites show heterogeneous in situ zircon Hf isotopic compositions, with ϵHf(t) values and T DM2 ages ranging from –2.6 to +9.7, and 1466 to 684 Ma, respectively, indicating the partial melting of mixed sources of juvenile crust and recycled ancient Meso-Neoproterozoic crustal materials of the NCC (Fig. 8a, b; Yang et al. Reference Yang, Wu, Shao, Wilde, Xie and Liu2006).
5.a.2. Rhyolites with potassic affinity
The rhyolite samples presented in this study from the Kulun region are characterized by high SiO2 (65.42–76.22 wt %), K2O (4.18–6.69 wt %) and Al2O3 (12.47–23.56 wt %) contents, low MgO (0.36–0.44 wt %) and very low Na2O (0.20–0.39 wt %) contents, with K2O/Na2O ratios of 17.16–22.93. These rhyolites could further be classified as potassic group with K2O/Na2O >2 according to the Na2O versus K2O diagrams presented in Foley et al. (Reference Foley, Venturelli, Green and Toscani1987). However, typical ultrapotassic rocks defined in Foley et al. (Reference Foley, Venturelli, Green and Toscani1987) should have shown high K2O (>3 wt %), MgO contents >3 wt % and K2O/Na2O >2 for whole-rock analyses, with high Mg no. values. These ultrapotassic rocks retrieved by a literature review carried out by Foley et al. (Reference Foley, Venturelli, Green and Toscani1987) are grouped into three major geochemical end-member groups, including lamproites, kamafugites, and rocks with high CaO and Al2O3 occurring in orogen zones (Miller et al. Reference Miller, Schuster, Kltzli, Frank and Purtscheller1999; Williams et al. Reference Williams, Turner, Pearce, Kelley and Harris2004; Liu et al. Reference Liu, Zhao, Zhu, Niu, Depaolo, Harrison, Mo, Dong, Zhou, Sun, Zhang and Liu2014). The third group of rocks have low Mg no. due to fractional crystallization, but their primary magmas also show characteristics of mantle derivation. These ultrapotassic magmatic rocks, such as lamprophyre dykes presented in Liu, Z. C. et al. (Reference Liu, Wang, Liu, Liu and Lai2020) are important indicators for extensional tectonic environments, such as post-collisional extension, continental rift, and intraplate rift.
However, elevated SiO2 and total alkali (Na2O + K2O), low MgO, and transition metal element (e.g. Cr, Co and Ni) contents, enrichments in LREEs, high positive Zr–Hf anomalies and depletions in Nb and Ta preclude a primitive mantle origin (Rudnick, Reference Rudnick1995). We argue that these rhyolite samples may be a result of partial melting of crustal materials. Our conclusion is based on the following evidence. Firstly, zircons from intermediate igneous rocks show heterogeneous Hf isotopic compositions, with ϵHf(t) values and T DM2 ages ranging from –2.6 to +9.7, and 1466 to 684 Ma, respectively. However, zircons from the rhyolite samples show wide variations in Hf isotopic data, with ϵ Hf(t) values and T DM2 ages of –20.8 to +0.5 and 3578∼1494 Ma, respectively. Since radiogenic isotopic compositions are not changed by partial melting or fractional crystallization of magma, thus they can be used to trace the nature of the mantle source. The rhyolite samples have obvious negative ϵ Hf(t) values, implying that their magma source might contain more ancient crustal materials of the NCC. Then, the absence of voluminous coeval basaltic or andesitic materials within the Kulun region precluded the possibility of extensive fractional crystallization model. In addition, the rhyolite samples in the Kulun region show high Th and U contents (13.6–32.5 ppm and 3.8–7.7 ppm, respectively), high Th/Ce ratios (0.21–0.36) and low Eu/Eu* ratios (<1) that are comparable with upper crustal values (Rudnick & Fountain, Reference Rudnick and Fountain1995; Rudnick & Gao, Reference Rudnick, Gao and Rudnick2003). Together with their trace-element and REE patterns that are similar to those of upper crust, we suggest that these rhyolites were derived from the partial melting of upper crust (Kerrich et al. Reference Kerrich, Polat, Wyman and Hollings1999). All the evidence indicates that the magma source of the studied rhyolites is dominated by Archaean–Mesoproterozoic ancient crustal components with minor juvenile crustal contributions.
Therefore we turn our attention to the most striking features of these rhyolite samples in the Kulun region, that is strong Na depletions and relative K enrichments, and resultant high K2O/Na2O ratios (17.16–22.93). To date, several hypotheses have been proposed to explain the K2O enrichments and Na2O depletions for the potassic affinity, including weathering, geochemical alteration, fractional crystallization and high-temperature K-metasomatism (McLennan, Reference McLennan1993; Ennis et al. Reference Ennis, Dunbar, Campbell and Chapin2000).
The rhyolite samples in the Kulun region investigated in this study have relatively low loss on ignition values (mean = 2.21 wt %). These rhyolites still retain their primary magmatic texture, structure and mineral associations, even though weak mineral alteration of sericitization and kaolinitization has occurred (Fig. 4g, j). Furthermore, Th is considered as being immobile during low-grade alteration of igneous rocks; thus, it has been employed to monitor other trace elements (Gibson et al. Reference Gibson, Kirkpatrick, Emmermann, Schmincke, Pritchard, Oakley, Thorpe and Marriner1982). Analyses show that SiO2, MgO, P2O5 Al2O3, almost all of REEs, Nb, Ta, Ti, Zr, and Hf correlate well with Th (online Supplementary Material Table S4), implying their relative immobilities. In addition, K2O, Rb, Sr and Ba also display good correlations with Th (online Supplementary Material Table S4), further excluding the influence of geochemical alteration during post-eruption processes.
Available studies have shown that high-temperature K-metasomatism can result in the formation of magmas with potassic affinity, which are characterized by increased K2O, Rb, and Zn contents, a decrease in Na2O contents, as well as depletions in REEs (Kinnaird et al. Reference Kinnaird, Bowden, Ixer and Odling1985). The analysed rhyolites, however, have Rb contents ranging from 188.2 to 277.8 ppm, clearly lower than those of rhyolites with potassic characteristics resulting from K-metasomatism (≥1000 ppm; Samuel et al. Reference Samuel, Moussa and Azer2007). All these characteristics do not support a model of high-temperature K-metasomatism. Previous studies have also shown that the fractional crystallization of plagioclase could account for the generation of potassic magmas (Shan et al. Reference Shan, Niu, Yu and Zeng2007). These rhyolites with potassic affinity from the Kulun region show variations in major and trace elements and have negative anomalies of Ba, Sr and Eu (0.46–0.76), indicating that the primary magma had undergone a certain degree of fractional crystallization. The positive correlations between Sr and Ba and δEu versus Ba, together with negative correlations between Sr versus SiO2 (online Supplementary Material Table S4), further suggested that the fractional crystallization indeed occurred during genetic evolution, as plagioclase is enriched in Eu and Sr (Fig. 10a–d; Rapp et al. Reference Rapp, Shimizu and Norman2003). However, in consideration of the obviously low Rb/Sr ratios (0.66–0.91) for these rhyolites, the removal of plagioclase from the felsic magmas was minor and consequently had a limited effect on the very low Na2O contents (Ajaji et al. Reference Ajaji, Weis, Giret and Bouabdellah1998).

Fig. 10. Diagrams of (a) Sr versus SiO2, (b) Ba versus SiO2, (c) Ba versus Sr, and (d) Ba versus δEu for the late Palaeozoic magmatic rocks in the Kulun region along the Solonker–Changchun suture zone. Typical potassic rhyolite data are from Samuel et al. (Reference Samuel, Moussa and Azer2007), Hu et al. (Reference Hu, Li, Wang, Xie and Wu2013), Ding et al. (Reference Ding, Zhang, Dong, Yan, Lin and Jiang2015) and Chen et al. (Reference Chen, Shi, Fan, Zou, Liu, Huang, Gong, Yi and Wu2016).
Experimental investigations have further demonstrated that the processes of liquid immiscibility of the felsic magmas also contributed to the potassium-rich affinity, as Na2O dissolves more favourably than K2O in the volatiles during the magmatic evolution in the magma chamber (Veksler & Thomas, Reference Veksler and Thomas2002; Veksler, Reference Veksler2004; Samuel et al. Reference Samuel, Moussa and Azer2007). The explosive eruption of volcanoes with a high volatile content naturally causes the removal of a great part of Na2O in melts, as well as K2O contents to a lesser degree (Samuel et al. Reference Samuel, Moussa and Azer2007). It is noteworthy that most reported potassic or/and ultrapotassic rhyolites are always associated with abundant pyroclastic rocks in the field outcrops (Fig. 4k, l); examples are the Wadi El-Mahash and Gebel El-Homra ultrapotassic rhyolites in Sinai (Samuel et al. Reference Samuel, Moussa and Azer2007), the Tanggula Pass ultrapotassic rhyolites in southern Qiangtang (Chen et al. Reference Chen, Shi, Fan, Zou, Liu, Huang, Gong, Yi and Wu2016), and the Lhasa ultrapotassic rhyolites in southern Tibet (Ding et al. Reference Ding, Zhang, Dong, Yan, Lin and Jiang2015). These characteristics suggest a crucial role for the accumulation of gases, vapours or volatiles. In this case, the remaining alumina would import more potassium into the magma chamber, forming more alkaline feldspars owing to the lack of sodium, as well as the increase in normative corundum content. Based on a calculation of the CIPW norms, these rhyolites in the Kulun region with potassic affinity presented in this study have high contents of normative corundum, further supporting this hypothesis. Calculation results show that these rhyolite samples from the Kulun region have normative corundum values ranging from 5.35 to 11.18 vol. % and 7.49 to 15.70 wt %, respectively. Therefore the potassic affinity of these rhyolites from the Kulun region is dominantly ascribed to liquid immiscibility of felsic magma, as subsequent fractional crystallization and removal of plagioclase were limited.
5.b. The late Palaeozoic geodynamic evolution of the eastern Palaeo-Asian Ocean
The Solonker–Changchun suture zone has been considered as the locus for the final disappearance of the eastern Palaeo-Asian Ocean between the NCC and the northern micro-continental blocks (combined NE China blocks; Sengör et al. Reference Sengör, Natal’in and Burtman1993; Badarch et al. Reference Badarch, Cunningham and Windley2002; Li, Reference Li2006; Zhou, J. B. et al. Reference Zhou, Wilde, Zhao, Zhang, Wang and Zeng2010, Reference Zhou, Wilde, Zhao and Han2018; Wilde, Reference Wilde2015; Eizenhöfer & Zhao, Reference Eizenhöfer and Zhao2018; Chai et al. Reference Chai, Ma, Santosh, Hao, Luo, Fan, Gao, Zong, Mao and Wang2020). However, the proposed hypotheses favoured that the suturing took place in the Middle Devonian to Carboniferous (Tang, Reference Tang1990; Xu et al. Reference Xu, Charvet, Chen, Zhao and Shi2013, Reference Xu, Zhao, Wang, Liao, Luo, Bao and Zhou2015; Zhao et al. Reference Zhao, Chen, Xu, Faure, Shi and Choulet2013, Reference Zhao, Appel, Xu and Sukhbaatar2020), early Permian (Zhang et al. Reference Zhang, Xue, Yuan, Ma and Wilde2012) or late Permian–Middle Triassic (Sengör et al. Reference Sengör, Natal’in and Burtman1993; Xiao et al. Reference Xiao, Windley, Hao and Zhai2003, Reference Xiao, Windley, Sun, Li, Huang, Han, Yuan, Sun and Chen2015; Yu et al. Reference Yu, Ge, Yang, Zhao, Zhang and Su2014; Eizenhöfer et al. Reference Eizenhöfer, Zhao, Zhang and Sun2014; Wilde, Reference Wilde2015; Yuan et al. Reference Yuan, Zhang, Xue, Lu and Zong2016; Guo et al. Reference Guo, Li, Fan, Li, Zhao and Huang2016; Liu, J. et al. Reference Liu, Liu, Li, Zhao, Wang, Peng, Yang and Dou2016, Reference Liu, Wang, Liu, Liu and Lai2020; Huang et al. Reference Huang, Yan, Piper, Zhang, Yi, Yu and Zhou2018; Eizenhöfer & Zhao, Reference Eizenhöfer and Zhao2018; Song et al. Reference Song, Han, Gao, Geng, Li, Meng, Han, Zhong, Li, Du, Yan and Liu2018; Jing et al. Reference Jing, Ge, Dong, Yang, Ji, Bi, Zhou and Xing2020 a,b; Chai et al. Reference Chai, Ma, Santosh, Hao, Luo, Fan, Gao, Zong, Mao and Wang2020) periods. Important insights into the tectonic evolution of the eastern CAOB, which formed in response to the subduction, accretion and final closure of the Palaeo-Asian Ocean, are provided by the early Permian magmatic rocks in the Kulun region (Cao et al. Reference Cao, Xu, Pei, Wang, Wang and Wang2013; Xu et al. Reference Xu, Charvet, Chen, Zhao and Shi2013, Reference Xu, Zhao, Wang, Liao, Luo, Bao and Zhou2015; Yu et al. Reference Yu, Ge, Yang, Zhao, Zhang and Su2014; Wilde, Reference Wilde2015; Xiao et al. Reference Xiao, Windley, Sun, Li, Huang, Han, Yuan, Sun and Chen2015; Liu, J. et al. Reference Liu, Liu, Li, Zhao, Wang, Peng, Yang and Dou2016, Reference Liu, Wang, Liu, Liu and Lai2020; Song et al. Reference Song, Han, Gao, Geng, Li, Meng, Han, Zhong, Li, Du, Yan and Liu2018; Jing et al. Reference Jing, Ge, Dong, Yang, Ji, Bi, Zhou and Xing2020 a; Chai et al. Reference Chai, Ma, Santosh, Hao, Luo, Fan, Gao, Zong, Mao and Wang2020).
The early Permian igneous rocks in the Kulun region are predominantly andesites, rhyolites, and diorite porphyrites. They belong to the high-K calc-alkaline to shoshonite series, and show enrichments in LREEs and LILEs, and depletions in HREEs (Nb, Ta and Ti) and HFSEs. These geochemical characteristics are similar to the volcanic rocks formed in an active continental margin environment analogous to the modern Andes (Pearce, Reference Pearce and Thorpe1982; Pitcher, Reference Pitcher and Hsu1983; Xiao et al. Reference Xiao, Windley, Hao and Zhai2003). Furthermore, Th/Ta ratios have been used to discriminate active continental margins from oceanic arcs based on immobile elements (Gorton & Schandl, Reference Gorton and Schandl2000). These studied intermediate-acidic volcanic rocks have relatively high Th/Ta ratios and display an affinity with active continental margins (Fig. 11a). The same conclusion is also verified in the Hf–(Rb/30)–(Ta*3) discrimination diagram, as most intermediate-acidic volcanic rock samples from the Kulun region plot into the area of volcanic arc granites (VAG; Fig. 11b). These results, combined with previously published data, identify an E–W-trending calc-alkaline magmatic belt from early Carboniferous to Early Triassic times (347–250 Ma) along the Solonker–Changchun suture zone (Li, Reference Li2006; Wu et al. Reference Wu, Sun, Ge, Zhang, Grant, Wilde and Jahn2011; Li et al. Reference Li, Wilde, He, Jiang, Liu and Zhao2014, Reference Li, Wilde, Wang, Xiao and Guo2016; Guo et al. Reference Guo, Li, Fan, Li, Zhao and Huang2016; Yuan et al. Reference Yuan, Zhang, Xue, Lu and Zong2016; Song et al. Reference Song, Han, Gao, Geng, Li, Meng, Han, Zhong, Li, Du, Yan and Liu2018; Liu, J. et al. Reference Liu, Wang, Liu, Liu and Lai2020; Jing et al. Reference Jing, Ge, Dong, Yang, Ji, Bi, Zhou and Xing2020 a,b). The magmatic belt was believed to be the product of the Andean-type active continental margin environments in response to southward subduction of the eastern Palaeo-Asian Ocean (Fig. 1b; Li, Reference Li2006; Zhou et al. Reference Zhou, Wilde, Zhao, Zhang, Wang and Zeng2010; Wu et al. Reference Wu, Sun, Ge, Zhang, Grant, Wilde and Jahn2011; Wilde, Reference Wilde2015; Eizenhöfer & Zhao, Reference Eizenhöfer and Zhao2018; Zhou, Z. B. et al. Reference Zhou, Wilde, Zhao and Han2018). Magmatic activities were prolonged, lasting for ∼100 Myr from 347 Ma to 250 Ma, and cannot be related to continental rifting or a post-collision extension, but is coincident with the subduction-related arc magmatism caused by the southward subduction of the eastern Palaeo-Asian Ocean slab (Fig. 1b; online Supplementary Material Table S1). High-magnesium andesites with ages of c. 250 Ma have been identified recently in the Kaiyuan and Linxi regions along the Solonker–Changchun suture zone, further demonstrating that the eastern Palaeo-Asian Ocean existed until Early Triassic time (Liu et al. Reference Liu, Wang, Wang, He, Zong, Gao, Hu and Gong2012; Yuan et al. Reference Yuan, Zhang, Xue, Lu and Zong2016).

Fig. 11. Diagrams of (a) Th/Ta versus Yb (Gorton & Schandl, Reference Gorton and Schandl2000), and (b) Hf–(Rb/30)–(Ta*3) ternary variation diagram (Harris et al. Reference Harris, Pearce, Tindle, Coward and Ries1986) for the late Palaeozoic magmatic rocks in the Kulun region along the Solonker–Changchun suture zone. Abbreviations: VAG – volcanic arc granites; WPG – within-plate granites; syn-COLG – syn-collisional granites; POG – post-collisional granites; MORBs – mid-ocean ridge basalts. The data for Permian granites along the Solonker–Changchun suture zone are from Jing et al. (Reference Jing, Ge, Dong, Yang, Ji, Bi, Zhou and Xing2020 a,b and references therein). Typical potassic rhyolite data are from Samuel et al. (Reference Samuel, Moussa and Azer2007), Hu et al. (Reference Hu, Li, Wang, Xie and Wu2013), Ding et al. (Reference Ding, Zhang, Dong, Yan, Lin and Jiang2015) and Chen et al. (Reference Chen, Shi, Fan, Zou, Liu, Huang, Gong, Yi and Wu2016).
In addition, models that propose an Early–Middle Devonian age for the final closure of the eastern Palaeo-Asian Ocean (Xu et al. Reference Xu, Charvet, Chen, Zhao and Shi2013) cannot explain the occurrence of middle Permian radiolarians and conodonts in the northern Solonker–Changchun suture zone (Wang, Reference Wang1997). The amalgamation of the Angara and Cathaysian floras along the Solonker–Changchun suture zone in late Permian time (Deng et al. Reference Deng, Wan and Yang2009), and the occurrence of marine pelagic facies in the middle Permian Zhesi Formation and mixed marine-terrestrial facies in the upper Permian Linxi Formation in the southeastern CAOB support a latest Permian–Early Triassic age for the final closure of the eastern Palaeo-Asian Ocean (Shang, Reference Shang2004). Furthermore, the latest researches on regional metamorphism in the southeastern CAOB carried out by Li et al. (Reference Li, Yu, Guo and Xu2021) revealed a medium-pressure metamorphism in the eastern Songnen Block, and the metamorphic conditions were under lower-amphibolite-facies with P–T conditions of 5.5–7.1 kbar and 592–630 °C. The medium-pressure metamorphism occurred in late Permian time (260–251 Ma), which was associated with the collision between the combined NE China blocks and the NCC in response to the final closure of the Palaeo-Asian Ocean. We thus support those models advocating the final closure of the eastern Palaeo-Asian Ocean occurring in latest late Permian–Early Triassic times.
Abundant palaeomagnetic data obtained over the past few decades have provided additional constraints on the geodynamic evolution of the eastern Palaeo-Asian Ocean during the Permian–Triassic period. For example, Zhang et al. (Reference Zhang, Huang, Zhao, Meert, Zhang, Liang, Bai and Zhou2018) proposed that the final closure of the eastern Palaeo-Asian Ocean occurred in late Permian–Early Triassic times (ca. 250 Ma), as no significant latitudinal difference between the southeastern CAOB blocks was indicated by palaeomagnetic data from Permian volcanic rocks (the Sanmianjing, Elitu and Dashizhai formations) (Huang et al. Reference Huang, Yan, Piper, Zhang, Yi, Yu and Zhou2018). In addition, Zhang et al. (Reference Zhang, Huang, Zhao, Meert, Williams, Zhao and Zhou2021) further reported two robust palaeomagnetic results from 320–280 Ma volcanic strata in the South Mongolia–Xing’an Belt, and the results indicate that the northward motion of North China and Mongolia paralleled Laurussia from late Carboniferous–early Permian times. Quantization results show the N–S width of the Palaeo-Asian Ocean in the east-central segment was ca. 2700 km during late Carboniferous to early Permian times. It is therefore suggested the early Permian magmatic rocks in the Kulun region were formed in an active continental margin setting influenced by the southward subduction of the eastern Palaeo-Asian Ocean. The widespread occurrence of the latest Early–Middle Triassic syn-collisional granites such as the Dayushan pluton (Sun et al. Reference Sun, Wu, Zhang and Gao2004), Dongfeng granite (Xin et al. Reference Xin, Ren, Peng and Sun2011) and Baicaoping porphyrite (Zhang et al. Reference Zhang, Wu, Wilde, Zhai, Lu and Sun2004) along the Solonker–Changchun suture zone also suggests a prolonged contraction (Jing et al. Reference Jing, Yang, Ge, Dong, Ji, Bi, Zhou and Xing2022). Thus, we prefer that the eastern Palaeo-Asian Ocean likely closed during Early–Middle Triassic time (Fig. 12; Xiao et al. Reference Xiao, Windley, Hao and Zhai2003; Li, Reference Li2006; Wu et al. Reference Wu, Sun, Ge, Zhang, Grant, Wilde and Jahn2011; Cao et al. Reference Cao, Xu, Pei, Wang, Wang and Wang2013; Eizenhöfer et al. Reference Eizenhöfer, Zhao, Zhang and Sun2014; Li et al. Reference Li, Wilde, He, Jiang, Liu and Zhao2014, Reference Li, Wilde, Wang, Xiao and Guo2016; Wilde, Reference Wilde2015; Yuan et al. Reference Yuan, Zhang, Xue, Lu and Zong2016; Huang et al. Reference Huang, Yan, Piper, Zhang, Yi, Yu and Zhou2018; Song et al. Reference Song, Han, Gao, Geng, Li, Meng, Han, Zhong, Li, Du, Yan and Liu2018; Eizenhöfer & Zhao, Reference Eizenhöfer and Zhao2018; Liu, J. et al. Reference Liu, Wang, Liu, Liu and Lai2020; Jing et al. Reference Jing, Ge, Dong, Yang, Ji, Bi, Zhou and Xing2020 a,b; Zhao et al. Reference Zhao, Appel, Xu and Sukhbaatar2020).

Fig. 12. (a) Palaeogeographic reconstruction model of the East Asian blocks and Gondwana, Laurussia and Siberia during the early Permian period (Ren et al. Reference Ren, Zhang, Gao, Zhao, Wu, Yang and Li2020). Abbreviations: NQT – North Qiangtang Terrane; SQT – South Qiangtang Terrane; SI – Sibumasu Block; IC – Indochina Block; SIB – Siberia Block; TM – Tarim Block; QD – Qaidam Block; NCB – North China Block; SCB – South China Block; XSB – Xilinhot–Songliao Block; MOB – Mongolia Block; JB – Jiamusi–Bureya Block. (b) Schematic models for the early Permian tectonic evolution of the eastern Palaeo-Asian Ocean.
6. Conclusions
Based on geochronological and geochemical studies, early Permian arc magmatic suites with a crystallization age of ca. 275 Ma have been identified in the Kulun region of the southeastern CAOB. The diorite porphyrites and andesites show crustal affinities and originated by partial melting of amphibolitic lower crust at a crustal depth of 30–40 km. The rhyolites show potassic affinity and heterogeneous zircon Hf isotopic signatures, with predominately negative values. These characteristics suggest a mixed source dominated by recycled older crust mixed with minor juvenile crustal materials. The potassic affinity of these rhyolites from the Kulun region is dominantly ascribed to liquid immiscibility of felsic magma, as subsequent fractional crystallization and removal of plagioclase were limited. Identification of this early Permian magmatic association and coeval arc-style magmatic rocks provides evidence of an active continental margin linked to the southward subduction of the Palaeo-Asian oceanic slab.
Supplementary material
To view supplementary material for this article, please visit https://doi.org/10.1017/S0016756822000358
Acknowledgements
We appreciate the staff (Li Su, Yu-jie Hao and Wen-qing Li) of China University of Geosciences (Beijing) and Jilin University for their support and help during the analyses of U–Pb isotopes. We are sincerely grateful to the staff (Jun-hui Bi and Hong-ying Zhou) of the Institute of Geology and Mineral Resources in Tianjin, because of their help, support and care during the process of the zircon Hf isotopic analyses. We also appreciate Sarah Sherlock for helpful comments in his handling of this manuscript, and we thank two anonymous reviewers for their detailed, constructive comments that have greatly improved the manuscript. This manuscript is subsidized by the National Natural Science Foundation of China (41872056).