Introduction
The Blue Lizard mine in Red Canyon, Utah, USA, has proven to be a remarkable source of new minerals, especially sodium uranyl sulfates. Lussierite, Na10[(UO2)(SO4)4](SO4)2(H2O)3, is the 13th new sodium uranyl sulfate mineral to be described from the mine and several more potentially new sodium uranyl sulfate minerals are currently under study. The astounding diversity and relatively high structural complexity of sodium uranyl sulfate minerals has been highlighted recently by Gurzhiy and Plášil (Reference Gurzhiy and Plášil2019). A large number of stable combinatorial linkages of uranyl and sulfate tetrahedra are possible, with the topological arrangements appearing to be strongly affected by at least three parameters: pH (Plášil et al., Reference Plášil, Kampf, Kasatkin and Marty2014); cation content; and water content. In general, sodium uranyl sulfate minerals follow the same structure topology trends as do other uranyl minerals (Lussier et al., Reference Lussier, Lopez and Hawthorne2016), where uranyl polyhedra preferentially polymerise into extended structures via linkages of their equatorial vertices, most often forming infinite chain or infinite sheet topologies. However, cluster topologies, such as that found in lussierite, are relatively abundant among the sodium uranyl sulfate minerals, for reasons that are not completely clear. Understanding the hierarchical arrangements of these structures and how conditions of formation influence the crystallised topologies is important to understanding the crystal–chemical nature of U–S systems, and for uranyl mineralogy as a whole.
Lussierite (/lu: 'si: ei ait/) is named in honour of Canadian mineralogist Aaron J. Lussier (born 1980). Dr. Lussier received his Ph.D. in mineralogy and crystallography from the University of Manitoba in 2012, studying zonation in tourmaline from granitic pegmatites and the occurrence of tetrahedrally coordinated aluminium and boron in tourmaline (Lussier, Reference Lussier2012). Dr. Lussier held a Post-Doctoral Research Fellowship at the University of Notre Dame from 2012 to 2015, investigating the crystal–chemistry of actinide elements with support from the Natural Sciences and Engineering Research Council (NSERC). He is currently a Research Scientist at the Canadian Museum of Nature and Councilor of the Mineralogical Association of Canada since 2017. Dr. Lussier has been involved in the descriptions of four new minerals, fluor-elbaite (Bosi et al., Reference Bosi, Andreozzi, Skogby, Lussier, Abdu and Hawthorne2013), ferro-ferri-nybøite (Lussier et al., Reference Lussier, Hawthorne, Abdu, Ball, Tait, Back, Steede, Taylor and McDonald2014), maruyamaite (Hawthorne et al., Reference Hawthorne, Lussier, Ball, Henry, Shimizu, Ogasawara and Ota2016) and shinkolobweite (Olds et al., Reference Olds, Lussier, Oliver, Petříček, Plášil, Kampf, Burns, Dembowski, Carlson and Steele2017). He has authored or co-authored several articles that advance our understanding of actinide mineralogy and crystal chemistry, and was senior author of a comprehensive review of the structures of uranyl compounds: “A revised and expanded structure hierarchy of natural and synthetic hexavalent uranium compounds” (Lussier et al., Reference Lussier, Lopez and Hawthorne2016). Dr. Lussier has given permission for this mineral to be named in his honour.
The new mineral and name were approved by the Commission on New Minerals, Nomenclature and Classification of the International Mineralogical Association (IMA2018-101, Kampf et al., Reference Kampf, Olds, Plášil, Nash and Marty2018). The description is based on one holotype and two cotype specimens deposited in the collections of the Natural History Museum of Los Angeles County, 900 Exposition Boulevard, Los Angeles, CA 90007, USA, catalogue numbers 73518 (holotype), 73519 (cotype) and 73520 (cotype).
Occurrence
Lussierite was found underground in the Blue Lizard mine (37°33′26″N, 110°17′44″W), Red Canyon, White Canyon District, San Juan County, Utah, USA. The mine is ~72 km west of the town of Blanding, Utah, and ~22 km southeast of Good Hope Bay on Lake Powell. The following information on the mine and its geology is taken largely from Chenoweth (Reference Chenoweth1993).
The uranium deposits in Red Canyon occur within the Shinarump member of the Upper Triassic Chinle Formation, in channels incised into the reddish-brown siltstones of the underlying Lower Triassic Moenkopi Formation. The Shinarump member consists of medium- to coarse-grained sandstone, conglomeratic sandstone beds and thick siltstone lenses. Ore minerals were deposited as replacements of wood and other organic material and as disseminations in the enclosing sandstone. Since the mine closed in 1978, oxidation of primary ores in the humid underground environment has produced a variety of secondary minerals, mainly sulfates, as efflorescent crusts on the surfaces of mine walls.
Lussierite is a rare mineral in the secondary mineral assemblages of the Blue Lizard mine. It occurs on a thick crust of gypsum overlaying matrix comprised mostly of subhedral to euhedral, equant quartz crystals that are recrystallised counterparts of the original grains of the sandstone. Other secondary phases found in close association with lussierite are belakovskiite, ferrinatrite, halite, ivsite, metavoltine, thénardite and at least three other potentially new minerals. Lussierite is the 19th new mineral to be described from the Blue Lizard mine, all within the last 6 years (cf. Kampf et al., Reference Kampf, Plášil, Kasatkin, Marty and Čejka2017), and numerous more new phases await characterisation.
Physical and optical properties
Lussierite crystals are prisms or blades, elongate on [001] and sometimes flattened on {010}, up to ~0.5 mm long. Prisms tend to occur in sprays (Fig. 1) or randomly scattered individuals; blades tend to occur in parallel growths (Fig. 2). Crystals exhibit the prism forms {010}, {110} and {$\bar{1}\bar{1}$0}, and have angled, wedge-like terminations composed of a series of {
$\bar{1}$k1} forms: {
$\bar{1}$11}, {
$\bar{1}$31}, {
$\bar{1}$51}, {
$\bar{1}$71} and {
$\bar{1}$·17·1} (Fig. 3). Twinning was not observed visually, but the structure refinement indicates twinning by merohedry, as is typical of noncentrosymmetric crystals.
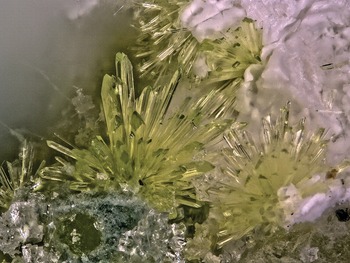
Fig. 1. Sprays of greenish yellow lussierite prisms with opaque white thénardite. The field of view is 1.7 mm across.

Fig. 2. Lussierite blades in incandescent (left) and 365 nm ultraviolet (right) illumination. The field of view is 0.84 mm across.

Fig. 3. Crystal drawing of lussierite; clinographic projection in non-standard orientation.
The mineral is light greenish yellow with a white streak and fluoresces bright cyan under 365 nm ultraviolet illumination (Fig. 2). Crystals are transparent and have a vitreous lustre. The tenacity is brittle, the Mohs hardness is 2½ (based on scratch tests), the fracture is irregular and no cleavage was observed. The density measured by flotation in methylene iodide–toluene is 2.87(2) g cm–3. The calculated density is 2.907 g cm–3 for the empirical formula and 2.912 g cm–3 for the ideal formula. At room temperature, the mineral is easily soluble in H2O.
Optically, lussierite is biaxial (+), with α = 1.493(1), β = 1.505(1) and γ = 1.518(1) (measured in white light). The 2V measured directly on a spindle stage is 88(1)°; the calculated 2V is 88.4°. Dispersion is r > v, moderate. The mineral is pleochroic: X = colourless, Y and Z = green yellow; X < Y ≈ Z. The optical orientation is X = b, Z ∧ a = 44° in obtuse β. The Gladstone–Dale compatibility, 1 – (K P/K C), is –0.001 (superior) based on the empirical formula using k(UO3) = 0.118, as provided by Mandarino (Reference Mandarino1976).
Raman spectroscopy
Raman spectroscopy was conducted on a Horiba XploRA PLUS both with 532 nm and 785 nm diode lasers. Some fluorescence was observed with the 532 nm laser, but it was relatively minor, so this spectrum is reported. The spectrum was recorded from 4000 to 100 cm–1 (Fig. 4) using a 50 µm laser slit, 1800 gr/mm diffraction grating, 100× (0.9 N.A.) objective and 15.2 mW laser power at the sample, with a beam diameter of ~1.2 µm.

Fig. 4. The baseline-corrected Raman spectrum of lussierite recorded with a 532 nm laser.
A broad band of weak intensity in the range ~3600–3200 cm–1 is attributed to the stretching vibrations of H2O molecules. According to the correlation given by Libowitzky (Reference Libowitzky1999), the approximate O–H···O hydrogen bond lengths range between 3.2 and 2.8 Å. There are a few broad weak bands located between ~1700–1450 cm–1, in the region expected for the ν2 (δ) bending vibrations of H2O molecules; however, we conclude that the observed features are more likely to be due to fluorescence because they were not observed in the spectrum recorded using the 785 nm diode laser.
Several past studies of uranyl sulfates informed our assignments of bands to specific Raman modes (cf. Plášil et al., Reference Plášil, Buixaderas, Čejka, Sejkora, Jelička and Novk2010; Čejka, Reference Čejka, Burns and Finch1999). In the lussierite spectrum, the split, triply degenerate ν3 (SO4)2– antisymmetric stretching vibrations occur as weaker bands at 1210 (with a shoulder at 1195), 1180, 1150 and 1130 cm–1. Weak bands at 1070 and 1040 cm–1 can be also related to ν3 (SO4)2–; the increased number of observed bands related to antisymmetric stretching vibrations is due to the fact that there are six symmetrically non-equivalent SO4 tetrahedra in the structure. The medium-strong bands at 998 and 989 cm–1 are unambiguously assignable to the ν1 (SO4)2– symmetric stretching vibrations. The weak band at 933 cm–1 is related with the ν3 (UO2)2+ antisymmetric stretching vibrations. The ν1 (UO2)2+ symmetric stretching vibration is present as a very strong band at 813 cm–1. Bartlett and Cooney (Reference Bartlett and Cooney1989) provided an empirical relationship to derive the approximate U–OUr bond lengths from the band positions assigned to the (UO2)2+ stretching vibrations, which gives 1.80 Å (ν1) and 1.76 Å (ν3), in accordance with U–OUr bond lengths from the X-ray data (1.77 Å and 1.78 Å).
Weak bands observed at 651 (with a shoulder at 645) and 620 cm–1 (with a shoulder at 627) are attributed to the split, triply degenerate ν4 (δ) (SO4)2– bending vibrations, and those at 500, 459, 450 and 427 cm–1 to the split doubly degenerate ν2 (δ) (SO4)2– bending vibrations. A weak band at 374 cm–1 is either due to ν (U–Oeq) stretching vibrations or Na–O stretches (e.g. Volkovich et al., Reference Volkovich, Griffiths, Fray and Fields1998; Plášil et al., Reference Plášil, Buixaderas, Čejka, Sejkora, Jelička and Novk2010; Kampf et al., Reference Kampf, Plášil, Kasatkin and Marty2015). Bands at 267 and 225 cm–1 arise from ν2 (δ) U–O–U bending modes. The bands at the lowest frequencies are attributable to further unassigned phonons.
Composition
Chemical analyses (6 points on 3 crystals) were performed using a Cameca SX-50 electron microprobe operating at an accelerating voltage of 15 kV, with a beam current of 10 nA and 20 µm spot diameter. Matrix effects were accounted for using the PAP correction routine (Pouchou and Pichoir, Reference Pouchou, Pichoir and Armstrong1985). The sample exhibited substantial damage from the electron beam. The phase is very susceptible to Na migration under the electron beam. Efforts to correct for this with very short count times or translating the sample under the beam during analysis, as well as zero-time corrections were only partially successful. Analyses exhibited large variations in Na content, always yielding values significantly below those indicated by the structure refinement, which was consistent with stoichiometric Na (see below). Consequently, we have used the calculated Na content corresponding to 10 Na atoms per formula unit (apfu). U and S exhibited time-dependent ingrowth during analysis for which a correction was applied. No other elements (including N) were detected by energy- or wavelength-dispersive spectroscopy. Due to the limited amount of material available, the H2O content could not be measured and is instead calculated by stoichiometry based on 6 S and 29 O apfu as indicated by the structure. The loss of loosely bound H2O under vacuum apparently results in higher concentrations for the remaining constituents than are to be expected for the fully hydrated phase and is probably the cause of the high analytical total. Analytical data are given in Table 1. The empirical formula (calculated on the basis of 29 O apfu) is Na10(U0.99O2)(S1.00O4)6·3H2O (+0.06 H for charge balance). The ideal formula is Na10[(UO2)(SO4)4](SO4)2(H2O)3, which requires Na2O 27.42, UO3 25.31, SO3 42.50, H2O 4.78, total 100 wt.%.
Table 1. Chemical composition of lussierite.

S.D. – standard deviation; * based on the structure.
X-ray crystallography and structure refinement
Powder X-ray studies were carried out using a Rigaku R-Axis Rapid II curved imaging plate microdiffractometer, with monochromatic MoKα radiation. A Gandolfi-like motion on the φ and ω axes was used to randomise the samples and observed d values and intensities were derived by profile fitting using JADE 2010 software (Materials Data, Inc.). The observed powder data for lussierite, presented in Table 2, show good agreement with the pattern calculated from the structure refinement. The unit-cell parameters refined from the powder data using JADE 2010 with whole pattern fitting are a = 9.3134(4), b = 28.7501(11), c = 9.6346(7) Å, β = 93.442(7)° and V = 2575.1(2) Å3.
Table 2. Powder X-ray data (d in Å) for lussierite. Only calculated lines with I ≥ 3 are listed.

Single-crystal X-ray studies were done using the same diffractometer and radiation used for the powder studies. The Rigaku CrystalClear software package was used for processing the structure data, including the application of an empirical absorption correction using the multi-scan method with ABSCOR (Higashi, Reference Higashi2001). The structure was solved in space group Cc using SIR2011 (Burla et al., Reference Burla, Caliandro, Camalli, Carrozzini, Cascarano, Giacovazzo, Mallamo, Mazzone, Polidori and Spagna2012). SHELXL-2016 (Sheldrick, Reference Sheldrick2015) was used for the refinement of the structure. The structure was noted to be the same as that determined for synthetic Na10[(UO2)(SO4)4](SO4)2·3H2O by Burns and Hayden (Reference Burns and Hayden2002). Consequently, equivalent atoms were assigned the same numbers in the lussierite structure. Both structures exhibit merohedral twinning, but have different dominant twin components. All non-hydrogen atoms were successfully refined with full occupancies and anisotropic displacement parameters. Because the electron microprobe analysis provided much lower than stoichiometric Na, an attempt was made to refine the occupancies of the Na sites; however, all refined to very close to full occupancy and did not improve the R factor. Difference-Fourier maps failed to reveal possible H sites. Data collection and refinement details are given in Table 3, atom coordinates and equivalent isotropic displacement parameters in Table 4, anisotropic displacement parameters in Table 5, selected bond distances in Table 6 and a bond-valence analysis in Table 7. The crystallographic information files have been deposited with the Principal Editor of Mineralogical Magazine and are available as Supplementary material (see below).
Table 3. Data collection and structure refinement details for lussierite.

R int = Σ|F o2–F o2(mean)|/Σ[F o2]. GoF = S = {Σ[w(F o2–F c2)2]/(n–p)}1/2. R 1 = Σ||F o|–|F c||/Σ|F o|. wR 2 = {Σ[w(F o2–F c2)2]/Σ[w(F o2)2]}1/2; w = 1/[σ2(F o2)+(aP)2+bP] where a is 0.0225, b is 0 and P is [2F c2+Max(F o2,0)]/3.
Table 4. Atom coordinates and equivalent isotropic displacement parameters (Å2) for lussierite.

Table 5. Anisotropic displacement parameters for lussierite.

Table 6. Selected bond distances (Å) for lussierite.

Table 7. Bond-valence analysis for lussierite. Values are expressed in valence units.*

* All bond valence parameters are from Gagné and Hawthorne (Reference Gagné and Hawthorne2015). Hydrogen bonds are not included. Note that O27, O28 and O29 are O atoms of H2O groups.
During the course of checking numerous lussierite crystals, one crystal examined provided the cell: a = 9.3141(7), b = 86.264(5), c = 9.6317(7) Å and β = 93.391(7)°. The structure of this crystal, also solved in space group Cc, indicates it to be the 3M polytype: lussierite-3M. The structure refinement was significantly poorer than that noted above (only the U atoms could be refined anisotropically). Notably, the powder X-ray diffraction pattern calculated from this structure fits the observed lussierite powder X-ray diffraction pattern equally well.
Description of the structure
The U site in the structure of lussierite is surrounded by seven O atom sites forming a squat pentagonal bipyramid. This is a typical coordination for U6+ in which the two short apical bonds of the bipyramid constitute the uranyl group (cf. Burns, Reference Burns2005). The two apical O atoms of the bipyramid (OUr) form short bonds with the U, and this unit comprises the UO22+ uranyl group. The five equatorial O atoms (Oeq) complete the U coordination and link to four different SO4 tetrahedra in the structure. Three of these SO4 tetrahedra (S1, S2 and S3) share single equatorial O atoms, while one (S6) shares two of its O atoms with the U1 bipyramid, thereby linking along a polyhedral edge. Such a bidentate linkage between a UO7 pentagonal bipyramid and a SO4 tetrahedron has been previously reported in the Na uranyl sulfate minerals klaprothite, péligotite and ottohahnite, which also occur at the Blue Lizard mine (Kampf et al., Reference Kampf, Plášil, Kasatkin, Marty and Čejka2017). The [(UO2)(SO4)4]6– uranyl sulfate cluster (Fig. 5) is the fundamental building block (FBB) in the structure of lussierite. It is also the FBB in the structures of klaprothite and péligotite; while in the structure of ottohahnite, four of these uranyl sulfate clusters are combined through shared SO4 tetrahedra to form a larger [(UO2)4(SO4)10]12– cluster.

Fig. 5. The [(UO2)(SO4)4]6– uranyl sulfate cluster in the structure of lussierite.
The uranyl sulfate clusters occur in layers parallel to {010} and are linked through a complex network of bonds involving 10 different Na+ cations, two isolated SO4 tetrahedra (S4 and S5) and three H2O groups (Fig. 6). Na–O coordination varies from 5 to 8. Isolated SO4 tetrahedra are rare in the structures of uranyl sulfates; however, they have been observed, for example, in belakovskiite, which contains [(UO2)(SO4)4(H2O)]6–FBBs (with monodentate U–S linkage) (Kampf et al., Reference Kampf, Plášil, Kasatkin and Marty2014).

Fig. 6. The crystal structure of lussierite. The unit cell is indicated by dashed lines. The Na atoms are shown as small turquoise spheres (labelled with small numbers). The O atoms of H2O groups are shown as white spheres (labelled with large numbers). Na–O bonds are drawn as sticks.
Acknowledgements
Reviewer Igor V. Pekov, Structures Editor Peter Leverett and an anonymous reviewer are thanked for their constructive comments on the manuscript. A portion of this study was funded by the John Jago Trelawney Endowment to the Mineral Sciences Department of the Natural History Museum of Los Angeles County. This research was also financially supported by the Czech Science Foundation (project GACR 17–09161S to JP).
Supplementary material
To view supplementary material for this article, please visit https://doi.org/10.1180/mgm.2019.34.