Introduction
Myxosporeans are parasitic cnidarians that mainly infect aquatic vertebrates and invertebrates. The involvement of annelids in the life cycle of myxosporeans was first revealed by Wolf and Markiw (Reference Wolf and Markiw1984), upon their discovery that the salmonid-infecting species Myxobolus cerebralis Hofer, 1903 has a life cycle stage that develops in the freshwater oligochaete Tubifex tubifex (Müller, 1774). Since then, several studies have provided direct and indirect evidence for a two-stage life cycle of these parasites, in which a myxosporean stage that develops in a fish alternates with an actinosporean stage that develops in an oligochaete or polychaete (see Eszterbauer et al., Reference Eszterbauer, Atkinson, Diamant, Morris, El-Matbouli, Hartikainen, Okamura, Gruhl and Bartholomew2015). Both life cycle stages are characterized by the production of spores necessary to achieve dissemination and transmission between vertebrate and invertebrate hosts. Spores are multicellular, comprised by two to several external valve cells that form a wall surrounding one to many polar capsules, and one to many sporoplasms. Despite sharing these main morphological features, the myxospores produced in the fish host and the actinospores produced in the annelid host exhibit highly distinct morphotypes (Lom and Dyková, Reference Lom and Dyková2006).
Currently, actinospore morphotypes are divided into ca. 20 collective groups; the differentiation and classification of distinct types within these groups being traditionally based on morphological criteria. The latter include the shape and size of the actinospores’ body and valvular processes, shape and position of the polar capsules, and number of secondary cells in the sporoplasm (Lom et al., Reference Lom, McGeorge, Feist, Morris and Adams1997; Lom and Dyková, Reference Lom and Dyková2006). Actinospores of the aurantiactinomyxon collective group, specifically, are defined as having three stout, semicircularly curved, leaf-like valvular processes attached to an ellipsoidal body with protruding polar capsules at the apex and containing a sporoplasm with many secondary cells (Lom and Dyková, Reference Lom and Dyková2006). In the past few decades, however, the implementation of molecular tools to the study of actinosporeans has revealed that morphological criteria are unreliable for performing differential diagnoses between types (e.g. Hallett et al., Reference Hallett, Atkinson and El-Matbouli2002, Reference Hallett, Atkinson, Erséus and El-Matbouli2004; Eszterbauer et al., Reference Eszterbauer, Marton, Rácz, Letenyei and Molnár2006; Xi et al., Reference Xi, Zhou, Xie, Pan, Yang and Ge2015; Rangel et al., Reference Rangel, Castro, Rocha, Cech, Casal, Azevedo, Székely, Cavaleiro and Santos2016a; Zhao et al., Reference Zhao, Borkhanuddin, Wang, Liu, Cech, Zhai and Székely2016). Congruently, actinospores descriptions are currently based on the combined analysis of morphological and molecular data.
Initially, life cycle studies relied solely on data acquired through means of experimental transmission between vertebrate and invertebrate hosts. The complicated logistics and time-consuming nature of this methodology, however, hinders the achievement of results. Moreover, its reliability has been discredited by the usage of molecular tools, which show that mixed and non-spore forming infections are common and may lead to erroneous associations (Holzer et al., Reference Holzer, Sommerville and Wootten2004; Atkinson and Bartholomew, Reference Atkinson and Bartholomew2009; Marton and Eszterbauer, Reference Marton and Eszterbauer2011; Székely et al., Reference Székely, Borkhanuddin, Cech, Kelemen and Molnár2014). Consequently, life cycle studies now mainly rely on sequencing of the SSU rDNA gene for inferring correspondence between myxosporean and actinosporean stages (see Eszterbauer et al., Reference Eszterbauer, Atkinson, Diamant, Morris, El-Matbouli, Hartikainen, Okamura, Gruhl and Bartholomew2015).
To date, near 60 myxosporean life cycles have been elucidated (see Eszterbauer et al., Reference Eszterbauer, Atkinson, Diamant, Morris, El-Matbouli, Hartikainen, Okamura, Gruhl and Bartholomew2015). Most refer to myxobolid species (Myxobolus, Henneguya and Thelohanellus) that infect fish and oligochaetes in freshwater environments (e.g. Styer et al., Reference Styer, Harrison and Burtle1991; Kent et al., Reference Kent, Whitaker and Margolis1993; Lin et al., Reference Lin, Hanson and Pote1999; Eszterbauer et al., Reference Eszterbauer, Székely, Molnár and Baska2000, Reference Eszterbauer, Marton, Rácz, Letenyei and Molnár2006, Reference Eszterbauer, Atkinson, Diamant, Morris, El-Matbouli, Hartikainen, Okamura, Gruhl and Bartholomew2015; Kallert et al., Reference Kallert, Eszterbauer, El-Matbouli, Erséus and Haas2005; Marton and Eszterbauer, Reference Marton and Eszterbauer2011; Székely et al., Reference Székely, Borkhanuddin, Cech, Kelemen and Molnár2014), with fewer providing information pertaining to the life cycles of myxosporean genera such as Ceratomyxa, Ceratonova, Chloromyxum, Ellipsomyxa, Gadimyxa, Hofferellus, Myxidium, Myxobilatus, Ortholinea, Paramyxidium, Parvicapsula, Sigmomyxa, Sphaerospora and Zschokkella (Grossheider and Körting, Reference Grossheider and Körting1992; Benajiba and Marques, Reference Benajiba and Marques1993; Yokoyama et al., Reference Yokoyama, Ogawa and Wakabayashi1993; Bartholomew et al., Reference Bartholomew, Whipple, Stevens and Fryer1997, Reference Bartholomew, Atkinson and Hallett2006; Holzer et al., Reference Holzer, Sommerville and Wootten2004, Reference Holzer, Sommerville and Wootten2006; Køie et al., Reference Køie, Whipps and Kent2004, Reference Køie, Karlsbakk and Nylund2007, Reference Køie, Karlsbakk and Nylund2008; Atkinson and Bartholomew, Reference Atkinson and Bartholomew2009; Rangel et al., Reference Rangel, Santos, Cech and Székely2009, Reference Rangel, Rocha, Casal, Castro, Severino, Azevedo, Cavaleiro and Santos2017; Karlsbakk and Køie, Reference Karlsbakk and Køie2012). In marine environments, polychaetes appear to be the hosts of choice (Køie et al., Reference Køie, Whipps and Kent2004, Reference Køie, Karlsbakk and Nylund2007, Reference Køie, Karlsbakk and Nylund2008; Rangel et al., Reference Rangel, Santos, Cech and Székely2009, Reference Rangel, Cech, Székely and Santos2011, Reference Rangel, Castro, Rocha, Severino, Casal, Azevedo, Cavaleiro and Santos2016b), with a few known exceptions occurring in estuarine waters, including Portuguese Rivers (Bartholomew et al., Reference Bartholomew, Whipple, Stevens and Fryer1997, Reference Bartholomew, Atkinson and Hallett2006; Rangel et al., Reference Rangel, Rocha, Castro, Severino, Casal, Azevedo, Cavaleiro and Santos2015, Reference Rangel, Rocha, Casal, Castro, Severino, Azevedo, Cavaleiro and Santos2017). Overall, few studies have aimed to investigate myxosporean biodiversity in invertebrate communities of this geographic region, with a total of 20 actinosporean types described from the raabeia (1 type), triactinomyxon (2 types), tetractinomyxon (2 types), sphaeractinomyxon (13 types), synactinomyxon (1 type) and unicapsulaticnomyxon (1 type) collective groups (Székely et al., Reference Székely, Eiras and Eszterbauer2005; Rangel et al., Reference Rangel, Santos, Cech and Székely2009, Reference Rangel, Cech, Székely and Santos2011, Reference Rangel, Rocha, Castro, Severino, Casal, Azevedo, Cavaleiro and Santos2015, Reference Rangel, Castro, Rocha, Cech, Casal, Azevedo, Székely, Cavaleiro and Santos2016a, Reference Rangel, Castro, Rocha, Severino, Casal, Azevedo, Cavaleiro and Santos2016b, Reference Rangel, Rocha, Casal, Castro, Severino, Azevedo, Cavaleiro and Santos2017; Rocha et al., Reference Rocha, Alves, Fernandes, Antunes, Azevedo and Casal2019a, Reference Rocha, Rangel, Castro, Severino, Azevedo, Santos and Casalb). Apart from the Raabeia type of Rocha et al. (Reference Rocha, Alves, Fernandes, Antunes, Azevedo and Casal2019a) and the Synactinomyxon type of Székely et al. (Reference Székely, Eiras and Eszterbauer2005), which were described from the freshwater oligochaetes Ilyodrilus templetoni (Southern, 1909) and Tubifex tubifex, respectively, all the other above-mentioned types were found infecting marine oligochaetes or polychaetes in downstream estuaries. Both the triactinomyxon and tetractinomyxon types have been paired with their respective myxosporean counterparts, through the positive match of SSU rDNA sequences. The triactinomyxon types, one reported from the oligochaete Limnodriloides agnes Habrě, 1967 and the other from an undetermined species of the genus Tectidrilus Erséus, 1982, were molecularly identified as the species Ortholinea auratae Rangel et al., Reference Rangel, Rocha, Castro, Severino, Casal, Azevedo, Cavaleiro and Santos2015 and O. labracis Rangel et al., Reference Rangel, Rocha, Casal, Castro, Severino, Azevedo, Cavaleiro and Santos2017, which parasitize the urinary bladder of gilthead seabream Sparus aurata Linnaeus, 1758 and European seabass Dicentrarchus labrax (Linnaeus, 1758), respectively (Rangel et al., Reference Rangel, Rocha, Castro, Severino, Casal, Azevedo, Cavaleiro and Santos2015, Reference Rangel, Rocha, Casal, Castro, Severino, Azevedo, Cavaleiro and Santos2017). In turn, both tetractinomyxon types were described from marine polychaetes, Hediste diversicolor (Müller, 1776) and an undetermined species of Capitella Blainville, 1828, and molecularly shown to be involved in the life cycle of Ellipsomyxa mugilis (Sitjà-Bobadilla and Alvarez-Pellitero, 1993) from the gall bladder of grey mullets and Sphaerospora dicentrarchi Sitjà-Bobadilla and Alvarez-Pellitero, 1992 from systemic infections in D. labrax, respectively (Rangel et al., Reference Rangel, Santos, Cech and Székely2009, Reference Rangel, Castro, Rocha, Severino, Casal, Azevedo, Cavaleiro and Santos2016b). To our best knowledge, further 17 myxosporean species have been reported from fishes inhabiting Portuguese rivers and estuaries, including Paramyxidium giardi (Cépède, Reference Cépède1906) Freeman and Kristmundsson, Reference Freeman and Kristmundsson2018 (see Eiras, Reference Eiras2016).
The myxosporean species Paramyxidium giardi was originally described as a member of the genus Myxidium, from infections in the kidney of European eel Anguilla anguilla (Linnaeus, 1758) in France (Cépède, Reference Cépède1906). Over time, numerous reports of this species were performed from infections occurring in multiple organs of anguillid eels worldwide (Ishii, Reference Ishii1915; Fujita, Reference Fujita1927; Hine, Reference Hine1975, Reference Hine1978, Reference Hine1980; Copland, Reference Copland1981; Treasurer and Cox, Reference Treasurer and Cox1997), including specimens of A. anguilla from Portuguese estuaries (Ventura and Paperna, Reference Ventura and Paperna1984; Azevedo et al., Reference Azevedo, Lom and Corral1989; Saraiva and Chubb, Reference Saraiva and Chubb1989; Saraiva and Eiras, Reference Saraiva and Eiras1996; Hermida et al., Reference Hermida, Saraiva and Cruz2008). In 1993, Benajiba and Marques Rocha et al., Reference Rocha, Alves, Fernandes, Antunes, Azevedo and Casal2019a identified the actinosporean counterpart of P. giardi as belonging to the aurantiactinomyxon collective group. The authors used cysts from the kidney of infected A. anguilla to experiment infection on samples of Tubifex that were reported to include non-parasitized specimens, having been collected from a previously studied area (presumably in France). Recent phylogenetic analyses corroborate the potential involvement of aurantiactinomyxon, as well as of raabeia and synactinomyxon types, in the life cycle of Paramyxidium spp. (Freeman and Kristmundsson, Reference Freeman and Kristmundsson2018; unpublished results). Nonetheless, molecular data has not been acquired that confirms the association between this genus and any of the above-mentioned collective groups.
In this study, microscopic and molecular tools are used to describe an aurantiactinomyxon type found infecting the intestinal epithelium of the marine oligochaete Tubificoides pseudogaster (Dahl, 1960) in the lower estuary of the River Minho, Portugal. This type constitutes the first of its collective group to be reported from Portuguese waters, and only the fourth described from a marine oligochaete. Its involvement in the life cycle of Paramyxidium giardi is molecularly inferred by comparison of the newly obtained SSU rDNA sequence to that available in GenBank from Icelandic isolates used in the species re-description.
Materials and methods
Sampling sites and morphological characterization
As part of a two-year myxozoan survey conducted in the River Minho between 2015 and 2016, mud was collected at low tide from the lower estuary, near the village of “Caminha” (41° 52′ N, 08° 50′W). In the laboratory, oligochaetes were collected from the mud and placed individually in 12-well plates containing brackish water (15‰ salinity) and kept at 4 °C. Salinity was chosen according to the value range (15 to 40‰) generally registered for this parameter in the lower estuary throughout the year (see Dias et al., Reference Dias, Morais, Cotter, Antunes and Hoffman2016). All oligochaetes were individually examined using the light microscope for the detection of actinosporean stages in internal tissues and cavities. Developmental stages and free actinospores were examined and photographed using an Olympus BX50 light microscope (Olympus, Japan). Morphometry was determined from fresh material, in accordance to Lom et al. (Reference Lom, McGeorge, Feist, Morris and Adams1997). Measurements include the mean value ± standard deviation (s.d.), range of variation, and number of measured actinospores (n).
DNA extraction, amplification and sequencing
Genomic DNA from infected oligochaetes was extracted using the GenElute™ Mammalian Genomic DNA Miniprep Kit (Sigma-Aldrich, St Louis, USA), following the manufacturer's instructions. The DNA was stored in 50 µL of TE buffer at −20 °C. The SSU rDNA gene of the actinospores was amplified using both universal and myxosporean-specific primers: the 5′-end by pairing the primer 18E (5′-CTG GTT GAT CCT GCC AGT-3′) (Hillis and Dixon, Reference Hillis and Dixon1991) with the primers MyxospecR (5′-CAA CAA GTT GAT AGG GCA GAA-3′) (Fiala, Reference Fiala2006) and MYX4R (5′-CTG ACA GAT CAC TCC ACG AAC-3′) (Hallett and Diamant, Reference Hallett and Diamant2001), and the 3′-end by pairing the primers MyxospecF (5′-TTC TGC CCT ATC AAC TTG TTG-3′) (Fiala, Reference Fiala2006) and MYX4F (5′-GTT CGT GGA GTG ATC TGT CAG-3′) (Rocha et al., Reference Rocha, Casal, Rangel, Castro, Severino, Azevedo and Santos2015) with the primer 18R (5′-CTA CGG AAA CCT TGT TAC G-3′) (Whipps et al., Reference Whipps, Adlard, Bryant, Lester, Findlay and Kent2003). In turn, the 16S mitochondrial DNA (mtDNA) gene of the oligochaete host was amplified using the universal primers 16sar-L (5′-CGC CTG TTT ATC AAA AAC AT-3′) and 16sbr-H (5′-CCG GTC TGA ACT CAG ATC ACG T-3′) (Palumbi et al., Reference Palumbi, Martin, Romano, McMillan, Stice and Grabowski2002). All PCRs were performed in 50 µL reactions using 10 pmol of each primer, 10 nmol of each dNTP, 2.0 mm MgCl2, 5 µL 10× Taq polymerase buffer, 1.5 units Taq DNA polymerase (NZYTech, Lisbon, Portugal), and 3 µL (approximately 100–150 ng) of genomic DNA. The reactions were run on a Hybaid PxE Thermocycler (Thermo Electron Corporation, Milford, Massachusetts), with initial denaturation at 95 °C for 3 min, followed by 35 cycles of 94 °C for 45 s, 53 °C for 45 s, and 72 °C for 90 s. The final elongation step was performed at 72 °C for 7 min. Five-μL aliquots of the PCR products were electrophoresed through a 1% agarose 1× tris-acetate-EDTA buffer (TAE) gel stained with ethidium bromide. PCR products were purified using Puramag™ magnetic beads coated with carboxylic acid groups (MCLAB, San Francisco, California, USA). Sequencing reactions were performed with the same primers used for amplification, using a BigDye Terminator v3.1 Cycle Sequencing Kit from AppliedBiosystems (Thermo Fisher Scientific, Waltham, Massachusetts, EUA), and were run on an ABI3700 DNA analyzer from AppliedBiosystems (Thermo Fisher Scientific, Waltham, Massachusetts, EUA).
Sequence assembly and distance estimation analysis
The SSU rDNA sequence of the parasite was assembled in MEGA7 (Kumar et al., Reference Kumar, Stecher and Tamura2016), by aligning the partial sequences obtained for each PCR fragment. A dataset was generated according to the highest similarity scores obtained using BLAST search, and included all known Paramyxidium spp., as well as closely related sequences of the aurantiactinomyxon, raabeia and synactinomyxon collective groups. All SSU rDNA sequences available for more distantly related aurantiactinomyxon types were also included in the dataset. Sequences were aligned using MAFFT version 7 available online, and distance estimation was calculated in MEGA7, with the p-distance model and all ambiguous positions removed for each sequence pair.
Results
Myxozoan survey, prevalence of infection and host identification
During this study, 577 oligochaetes were isolated from the mud collected in the lower estuary of the River Minho. Myxozoan infections were determined in a total of 9 specimens, in all of which it was possible to observe mature actinospores alongside younger developmental stages. Five specimens displayed infection in the coelomic cavity by members of the sphaeractinomyxon collective group. The remaining 4 specimens displayed actinosporean development in the intestinal epithelium: two by a type of the aurantiactinomyxon collective group, and the other two by a type of the synactinomyxon collective group. The description of the aurantiactinomyxon type is presented here; descriptions of the remaining types will be published elsewhere.
All 9 oligochaete specimens displaying myxozoan infection were identified as belonging to the marine species Tubificoides pseudogaster (Dahl, 1960). Identification was based on combined morphological features and molecular data of the 16S mitochondrial DNA (mtDNA). Infected oligochaetes looked alike, having similar dimensions, bifid setae and a body tegument devoid of papillae. Sequences of the 16S mtDNA were 98–100% similar to those available for the lineage II of this species, as reported by Kvist et al. (Reference Kvist, Sarkar and Erséus2010).
Characterization of the novel Aurantiactinomyxon type (Cnidaria, Myxozoa)
Description: Developmental stages of the gametogamy and sporogony phases developing in the intestinal epithelium (Fig. 1). Youngest developmental stages observed were binucleated cells (Fig. 2A). The latter divided twice to produce a set of four cells that form the initial pansporocyst, comprised by two somatic cells surrounding two generative inner cells (Fig. 2B and C). Three successive mitotic divisions and one meiotic division of the generative cells produced a final set of 16 haploid cells within the pansporocysts (Fig. 2D–F). At the end of gametogamy, these cells united two by two to form 8 diploid zygotes (Fig. 2G). Subsequent mitotic divisions of the zygotes originated 8 immature actinospores, each comprised by a sporoplasmic cell connected to three valvogenic cells and three capsulogenic cells. The valvogenic cells surrounded the capsulogenic cells and appeared positioned centrally, while corresponding sporoplasmic cells leaned against the pansporocyst's wall. Sporoplasmic cells increased in size and multiplied the number of secondary cells prior to migrating into the involucre formed by the valvogenic cells (Fig. 2H–I). At the end of sporogony, 8 mature actinospores were observed within each pansporocyst. Mature actinospores subspherical, 14.4 ± 0.6 (13.6–15.9) µm long (n = 25) and 12.7 ± 0.7 (11.3–13.3) µm wide (n = 25), entirely embraced by 3 equally sized valvular processes, leaf-like with rounded tips, 22.4 ± 2.4 (18.1–27.6) µm long (n = 47) and 15.5 ± 0.9 (13.3–17.0) µm wide at the base (n = 25). Three equally sized spherical polar capsules protruding from the anterior end of the actinospores, 2.6 ± 0.3 (1.9–3.5) µm in diameter (n = 53), each containing a polar tubule exhibiting 4 to 5 coils (Figs 2J–L and 3). Sporoplasm containing an undetermined number of secondary cells.

Fig. 1. Light micrographs showing pansporocysts of the new type of aurantiactinomyxon developing in the intestinal epithelium of the marine oligochaete Tubificoides pseudogaster in the lower estuary of the River Minho.
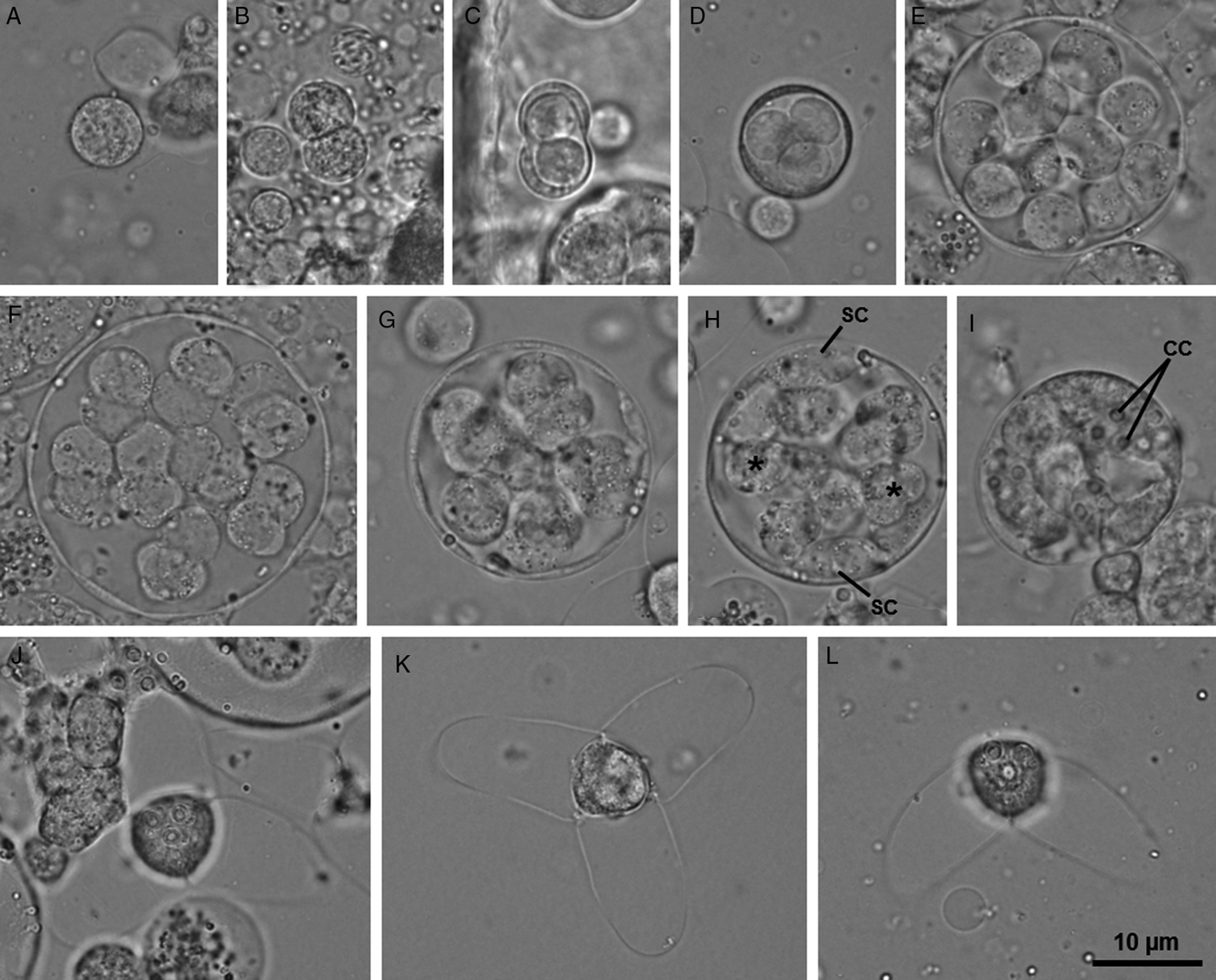
Fig. 2. Light micrographs of some developmental stages of the new type of aurantiactinomyxon found infecting the marine oligochaete Tubificoides pseudogaster in the lower estuary of the River Minho. (A–F) Gametogamy phase: (A) Binucleated cell; (B) Binucleated cell in cellular division; (C) Initial pansporocyst with two involucre cells and two inner cells; (D–F) Pansporocysts with four, 14 and 16 inner cells, respectively. (G–I) Sporogony phase: (G) Pansporocyst with 8 zygotes undergoing cellular divisions; (H) Pansporocyst showing the valvogenic and capsulogenic cells located centrally (*), while the sporoplasmic cells (SC) lean against the pansporocyst wall; (I) Pansporocyst containing 8 immature actinospores. Notice the capsulogenic cells; (J, K) Mature actinospores in apical view, evidencing the 3 spherical polar capsules protruding from the anterior end, and the rounded tips of the 3 equally sized leaf-like valvular processes; (L) Mature actinospore as observed in lateral view.

Fig. 3. Schematic drawing depicting a mature actinospore of the new type of aurantiactinomyxon found infecting the marine oligochaete Tubificoides pseudogaster in the lower estuary of the River Minho, as observed in lateral (A) and apical (B) view.
Type host: Tubificoides pseudogaster (Dahl, 1960) (Annelida, Oligochaeta).
Type locality: The lower estuary of the River Minho, near “Caminha” (41° 52′ N, 08° 50′ W), Portugal.
Site of infection: The intestinal epithelium.
Prevalence: 0.3% (2 infected in a total of 577 oligochaetes examined).
Deposition of material: Series of phototypes deposited together with a representative DNA sample in the Type Material Collection of the Laboratory of Animal Pathology, Interdisciplinary Centre of Marine and Environmental Research, Porto, Portugal, reference CIIMAR 2019.44.
Molecular data: One SSU rDNA gene sequence with a total of 2027 bp, deposited in GenBank with the accession no. MK635346. The latter is representative of two identical sequences that were separately obtained from the actinosporean developmental stages in the intestinal epithelium of two infected oligochaetes.
Remarks: Morphometry was determined from mature actinospores observed in both infected hosts. Morphometric variation between individual measurements was not recorded. Comparison to the ca. 39 known aurantiactinomyxon types without available molecular data revealed some morphometric similarity to the actinospores of the Aurantiactinomyxon type of McGeorge et al. (Reference McGeorge, Sommerville and Wootten1997), Aurantiactinomyxon type of Xiao and Desser (Reference Xiao and Desser1998) and Aurantiactinomyxon type 2 of Özer et al. (Reference Özer, Wootten and Shinn2002). Nonetheless, these types parasitize different oligochaete hosts in freshwater habitats of distant geographic locations (Canada and Scotland), further differing from the one in study in specific morphological aspects of the actinospores. Both the actinospores of the Aurantiactinomyxon type of McGeorge et al. (Reference McGeorge, Sommerville and Wootten1997) and of the Aurantiactinomyxon type 2 of Özer et al. (Reference Özer, Wootten and Shinn2002) are spherical instead of subspherical, with the first further having thinner valvular processes, and the second being generally bigger. In turn, the Aurantiactinomyxon type of Xiao and Desser (Reference Xiao and Desser1998) has pyriform polar capsules instead of spherical, and a slightly smaller actinospore body. It should be noted that no gross similarity was determined in relation to the three marine aurantiactinomyxon types reported in the literature (Hallett et al., Reference Hallett, Erséus, Lester and Morton1997), all of which are without available molecular data. In turn, morphological comparison to the freshwater Aurantiactinomyxon type of Benajiba and Marques (Reference Benajiba and Marques1993), previously reported to be the life cycle counterpart of P. giardi, was strongly hampered by the paucity of morphometric data given in its original description. Molecular comparison could be performed only in relation to 20 freshwater types with SSU rDNA sequences available from either their original descriptions, follow-up studies, or from the myxosporean species determined to be their life cycle counterparts through experimental transmission. The results showed significant genetic differences of the SSU rDNA sequences in all cases (ranging from 1.4% to as high as 24.8%). In turn, a high similarity value (99.4%) was obtained in relation to the sequence of Paramyxidium giardi available in GenBank (MH414925) from infections in the kidney of European eel A. anguilla. These two sequences differ in a total of 9 nucleotide positions over 2020 bp. Overall, the parasite was more related to other members of the genus Paramyxidium, as well as to actinosporean types known to be phylogenetically associated with the latter, namely raabeia, synactinomyxon and aurantiactinomyxon (similarity values ranged from 98.6% to as low as 81.6%). All other myxosporean sequences available in GenBank shared less than 80% similarity with the new Aurantiactinomyxon type.
Discussion
The majority of known actinosporean stages have been described based solely on morphological criteria. The implementation of molecular tools to the study of myxosporeans, however, has revealed that species having morphologically similar actinospores may be only distantly related based on SSU rDNA sequence data. In the other way around, species having actinospores with divergent morphology may be closely related (Hallett et al., Reference Hallett, Atkinson and El-Matbouli2002, Reference Hallett, Atkinson, Erséus and El-Matbouli2004; Eszterbauer et al., Reference Eszterbauer, Marton, Rácz, Letenyei and Molnár2006; Xi et al., Reference Xi, Zhou, Xie, Pan, Yang and Ge2015; Rangel et al., Reference Rangel, Castro, Rocha, Cech, Casal, Azevedo, Székely, Cavaleiro and Santos2016a; Zhao et al., Reference Zhao, Borkhanuddin, Wang, Liu, Cech, Zhai and Székely2016). In the case of aurantiactinomyxon, for instance, previous studies showed that distinct morphotypes can, in fact, share the same genotype (see Hallett et al., Reference Hallett, Atkinson and El-Matbouli2002; Eszterbauer et al., Reference Eszterbauer, Marton, Rácz, Letenyei and Molnár2006; Zhao et al., Reference Zhao, Borkhanuddin, Wang, Liu, Cech, Zhai and Székely2016). Consequently, the morphological characterization of actinospores is important but not enough for the description of new types, making the acquisition of molecular data indispensable for the identification of actinosporean stages. Our study strengthens this contention, given that without the use of molecular data, the Aurantiactinomyxon type described here could easily have been misidentified with the Aurantiactinomyxon type 1 of Negredo and Mulcahy (Reference Negredo and Mulcahy2001), as these types share a strong morphological resemblance (Negredo and Mulcahy, Reference Negredo and Mulcahy2001; Negredo et al., Reference Negredo, Dillane and Mulcahy2003). Despite morphological comparison to types without molecular data having revealed significant similarity to the Aurantiactinomyxon type of McGeorge et al. (Reference McGeorge, Sommerville and Wootten1997), Aurantiactinomyxon type of Xiao and Desser (Reference Xiao and Desser1998) and Aurantiactinomyxon type 2 of Özer et al. (Reference Özer, Wootten and Shinn2002), correspondence to any of these types could never be established with confidence, given their different hosts, habitats and geographical origins. In addition to the known unreliability of the usage of morphological criteria for type differentiation (see Hallett et al., Reference Hallett, Atkinson and El-Matbouli2002; Eszterbauer et al., Reference Eszterbauer, Marton, Rácz, Letenyei and Molnár2006; Zhao et al., Reference Zhao, Borkhanuddin, Wang, Liu, Cech, Zhai and Székely2016), aurantiactinomyxon appear to have strict host specificity, considering that no type has been molecularly proven to infect more than a single annelid species. Thus, we suggest that extensive morphological comparisons to all types that are without molecular data and have distinct oligochaete hosts are unnecessary. Actinospores of new isolates should only be identified either by DNA match or through a comprehensive morphological and biological comparison to known types sharing the same annelid host, regardless of there being high morphological similarity to other types.
Morphological features and genetic data identified the host species of the new Aurantiactinomyxon type as being Tubificoides pseudogaster. This annelid species is known to host several types of sphaeractinomyxon in Portuguese estuaries (this study; Rangel et al., Reference Rangel, Castro, Rocha, Cech, Casal, Azevedo, Székely, Cavaleiro and Santos2016a) and is further reported here to host infection by a member of the synactinomyxon collective group. Despite this significant biodiversity, concomitant infections were never observed in individual specimens. This is congruent with the very low infection rates commonly reported in wild oligochaete populations (e.g. Hallett et al., Reference Hallett, Erséus, O'Donoghue and Lester2001; Negredo and Mulcahy, Reference Negredo and Mulcahy2001; Atkinson and Bartholomew, Reference Atkinson and Bartholomew2009). In the same manner, the low value of individual prevalence of infection determined in this study (0.3%) agrees with those previously reported for aurantiactinomyxon types (e.g. Xiao and Desser, Reference Xiao and Desser1998; Xi et al., Reference Xi, Zhou, Xie, Pan, Yang and Ge2015; Zhao et al., Reference Zhao, Borkhanuddin, Wang, Liu, Cech, Zhai and Székely2016; Milanin et al., Reference Milanin, Atkinson, Silva, Alves, Maia and Adriano2017). Actinospore development was further demonstrated to follow the pattern typically reported for members of the aurantiactinomyxon collective group; development within the host was asynchronous, but sporogony within the pansporocysts was synchronous. Multiple layers of pansporocysts were present in the intestinal epithelium, partially protruding into the coelomic cavity (e.g. Marcucci et al., Reference Marcucci, Caffara and Goretti2009; Zhao et al., Reference Zhao, Zhai, Liu, Wang and Gu2017).
Almost all aurantiactinomyxon types reported in the literature infect oligochaete species belonging to the family Naididae Ehrenberg, 1828 [currently includes the members of the former Tubificidae (Erséus et al., Reference Erséus, Wetzel and Gustavsson2008)], be it in freshwater or marine environments. Aurantiactinomyxon pavinsis constitutes the only exception, having been widely reported to infect the freshwater lumbriculid Stylodrilus heringianus Claparède, 1862 (see Marques, Reference Marques1984; Oumouna et al., Reference Oumouna, Hallett, Hoffmann and El-Matbouli2003; Holzer et al., Reference Holzer, Sommerville and Wootten2004; Marcucci et al., Reference Marcucci, Caffara and Goretti2009). A few types have their oligochaete hosts identified only up to the genus- or family-level, but all infect naidids (see Marques, Reference Marques1984; Grossheider and Körting, Reference Grossheider and Körting1992; Benajiba and Marques, Reference Benajiba and Marques1993), while the Aurantiactinomyxon types 7 and 11 of El-Mansy et al. (Reference El-Mansy, Székely and Molnár1998), Aurantiactinomyxon type 1 of Oumouna et al. (Reference Oumouna, Hallett, Hoffmann and El-Matbouli2003) and Aurantiactinomyxon type 1 of Hallett et al. (Reference Hallett, Atkinson, Erséus and El-Matbouli2006) constitute the only members of the collective group that lack host information (El-Mansy et al., Reference El-Mansy, Székely and Molnár1998; Oumouna et al., Reference Oumouna, Hallett, Hoffmann and El-Matbouli2003; Hallett et al., Reference Hallett, Atkinson, Erséus and El-Matbouli2006). Up until this study, only the three aurantiactinomyxon types described by Hallett et al. (Reference Hallett, Erséus, Lester and Morton1997) were known to occur in the marine environment, parasitizing the naidids Limnodriloides toloensis Erséus, 1984, Pacifidrilus vanus (Erséus, 1984) and P. darvelli (Erséus, 1984). The species Tubificoides pseudogaster is, therefore, identified here as another member of the family Naididae serving as host for the aurantiactinomyxon collective group in marine environments.
To date, few other actinosporean types have been described from marine oligochaetes and all to parasitize naidids. These marine oligochaete-infecting types mostly belong to the sphaeractinomyxon collective group, with few representatives belonging to endocapsa and triactinomyxon. The 13 distinct types of sphaeractinomyxon recently reported from Portuguese estuaries are hosted by T. pseudogaster, its congener T. insularis (Stephenson, 1923), and Limnodriloides agnes Hrabĕ, 1967 (see Rangel et al., Reference Rangel, Castro, Rocha, Severino, Casal, Azevedo, Cavaleiro and Santos2016b; Rocha et al., Reference Rocha, Rangel, Castro, Severino, Azevedo, Santos and Casal2019b). Worldwide, the remaining known marine sphaeractinomyxon types were also reported from naidids belonging to the genera Ainudrilus Finogenova, 1982, Aktedrilus Knöllner, 1935, Bathydrilus Cook, 1970, Doliodrilus Erséus, 1984, Heronidrilus Erséus and Jamieson, 1981, Limnodriloides Pierantoni, 1903 and Thalassodrilides Brinkhurst and Baker, 1979 (Hallett et al., Reference Hallett, Erséus, Lester and Morton1997, Reference Hallett, O'Donoghue and Lester1998, Reference Hallett, Erséus, O'Donoghue and Lester2001; Hallett and Lester, Reference Hallett and Lester1999). Similarly, the three marine types included within the endocapsa collective group were reported from naidids of the genera Heronidrilus, Heterodrilus Pierantoni, 1902 and Thalassodrilides (Hallett et al., Reference Hallett, Erséus and Lester1999, Reference Hallett, Erséus, O'Donoghue and Lester2001). Lastly, to our best knowledge there are 5 marine triactinomyxon types described in the literature from naidids of the subfamily Limnodriloidinae Erséus, 1982 (including Limnodriloides and Tectidrilus spp.) (see Roubal et al., Reference Roubal, Hallett and Lester1997; Hallett et al., Reference Hallett, Erséus, O'Donoghue and Lester2001; Rangel et al., Reference Rangel, Rocha, Castro, Severino, Casal, Azevedo, Cavaleiro and Santos2015, Reference Rangel, Rocha, Casal, Castro, Severino, Azevedo, Cavaleiro and Santos2017). Overall, this suggests that the family Naididae played a preponderant role in the establishment and evolution of myxosporeans in invertebrate communities of estuarine and marine habitats.
Recently, a comprehensive co-phylogenetic study by Holzer et al. (Reference Holzer, Bartošová-Sojková, Born-Torrijos, Lövy, Hartigan and Fiala2018) evidenced that oligochaetes and polychaetes are the most ancient hosts of myxosporeans. As such, it can be assumed that the composition, ecology, spatial distribution and susceptibility of these hosts is crucial for myxosporean evolution and diversification. The Naididae is probably the most diverse and cosmopolitan oligochaete family, being present in all biogeographic regions, including sub-Antarctic islands (Timm and Martin, Reference Timm, Martin, Thorp and Rogers2015). Its involvement in myxosporean settlement and evolution, therefore, most likely relates to the high availability of its species in different aquatic habitats worldwide. Conjectures to other potential factors possibly influencing the reported range of myxosporean infections in naidids are difficult to be performed based on the limited information currently available for annelid-myxosporean interactions, especially in marine habitats. Future research should, therefore, aim to provide insight into the host-, parasite-, and environmental-related factors that influence myxosporean infection in annelid hosts. The acquisition of further information on this subject will, most certainly, prove to be relevant in improving our knowledge of myxosporean evolution.
Nowadays, the recognition of myxosporean life cycles is mainly based on DNA match between myxosporean and actinosporean counterparts. This molecular approach for establishing life cycle connections relies on the typically significant interspecific variability and limited intraspecific variability of the SSU rDNA gene of myxosporeans (Køie et al., Reference Køie, Whipps and Kent2004; Atkinson and Bartholomew, Reference Atkinson and Bartholomew2009). In some cases, however, it can be difficult to distinguish between different species and different geographic genotypes of one single species. Molecularly inferred life cycles range from 99.4% to 100% similarity between the SSU rDNA sequences of myxosporean and actinosporean counterparts (see Eszterbauer et al., Reference Eszterbauer, Atkinson, Diamant, Morris, El-Matbouli, Hartikainen, Okamura, Gruhl and Bartholomew2015). These values agree with the intraspecific variability generally accepted for myxosporeans, which can range between 0–3.6%, but is usually lower than 1% (Atkinson et al., Reference Atkinson, Bartošová-Sojková, Whipps, Bartholomew, Okamura, Gruhl and Bartholomew2015 and references therein). In this study, comparative molecular analysis of the SSU rDNA gene revealed the Aurantiactinomyxon type infecting T. pseudogaster sharing 99.4% of similarity with P. giardi, thus inferring its involvement in the life cycle of this myxosporean pathogen. The low genetic difference obtained (0.6%) is most likely representative of intraspecific variability due to the different geographic origin of the biological material, as previously suggested in the life cycle studies of Myxidium truttae Léger, 1930, Myxobolus cultus Yokoyama et al., 1995 and Thelohanellus kitauei Egusa and Nakajima, 1981 (see Holzer et al., Reference Holzer, Sommerville and Wootten2004; Eszterbauer et al., Reference Eszterbauer, Marton, Rácz, Letenyei and Molnár2006; Xi et al., Reference Xi, Zhang, Xie, Pan, Xu and Ge2013; Zhao et al., Reference Zhao, Borkhanuddin, Wang, Liu, Cech, Zhai and Székely2016). In these cases, the emergence of genotypically different subspecies was hypothesized to be the outcome of geographic isolation, with subsequent evolutionary adaptation to distinct environmental factors, and possibly different invertebrate hosts. In order to confirm our assumption of intraspecific variability between geographic isolates of P. giardi, a myxozoan survey on A. anguilla from the River Minho is being prepared and will aim to acquire molecular information from myxospores. This will allow to verify if genetic variation also occurs within a given geographic location and/or between life cycle counterparts. In this context, acquiring information from molecular markers other than the SSU rDNA gene may prove necessary in order to either confirm or refute the observations of relatedness performed here. Similarly, it would be interesting to sample marine oligochaetes from Icelandic Rivers where infections by P. giardi are known to occur.
The information thus far available for myxosporean life cycles demonstrates that there is no obvious correspondence between actinosporean and myxosporean morphotypes. Accordingly, phylogenetic analyses show most collective groups spanning distinct myxosporean suborders, as is the case of aurantiactinomyxon (e.g. Rocha et al., Reference Rocha, Rangel, Castro, Severino, Azevedo, Santos and Casal2019b). Members of aurantiactinomyxon have been implicated in the life cycles of species belonging to the genera Chloromyxum, Henneguya, Hoferellus, Myxobolus, Myxidium and Thelohanellus (Styer et al., Reference Styer, Harrison and Burtle1991; Grossheider and Körting, Reference Grossheider and Körting1992; Benajiba and Marques, Reference Benajiba and Marques1993; Lin et al., Reference Lin, Hanson and Pote1999; Holzer et al., Reference Holzer, Sommerville and Wootten2004; Eszterbauer et al., Reference Eszterbauer, Marton, Rácz, Letenyei and Molnár2006; Molnár et al., Reference Molnár, Marton, Székely and Eszterbauer2010). Our results further broaden the array of genera associated with the aurantiactinomyxon collective group, by molecularly inferring the involvement of the type described here in the life cycle of Paramyxidium giardi. This finding is corroborated by the experimental transmission study performed by Benajiba and Marques (Reference Benajiba and Marques1993), who also identified the actinosporean counterpart of P. giardi as an aurantiactinomyxon type, but developing in a freshwater tubificid. Accordingly, Freeman and Kristmundsson (Reference Freeman and Kristmundsson2018) suggested freshwater oligochaetes as the most probable annelid hosts of Paramyxidium spp., considering that known fish hosts [European eel and Indo-Pacific tarpon Megalops cyprinoides (Broussonet, 1782)], were sampled from freshwater habitats. Nonetheless, both these species are migratory fish that spawn at sea producing leptocephalic larvae that migrate into inland waters to grow. Taking into account that a significant portion of their lives is spent in fresh- and brackish waters, it is possible that infection is acquired from marine oligochaetes inhabiting estuarine habitats, as our results suggest in the case of P. giardi. During our myxozoan survey, molecular evidence was also found for the development of a single type of sphaeractinomyxon in both a freshwater oligochaete species of the genus Potamothrix Vejdovský and Mrázek, 1903 and T. pseudogaster, suggesting that the myxosporean counterpart of this type uses a fish host that is not entirely restricted to freshwater, most probably a grey mullet (unpublished results). Congruently, the possibility of P. giardi having acquired both marine and freshwater oligochaetes as hosts cannot be disregarded, considering that the ecology of the European eel allows it to contact with intrinsically distinct invertebrate communities throughout its life.
The genus Paramyxidium Freeman and Kristmundsson, Reference Freeman and Kristmundsson2018 encompasses species that, whilst being morphologically similar to Myxidium, differ by having histozoic development in various tissues of fish from Elopomorpha (see Freeman and Kristmundsson, Reference Freeman and Kristmundsson2018). The European eel is the sole representative of Elopomorpha in Portuguese waters. Thus far, it has been recognized to host four myxosporean species in this geographic location, including P. giardi from infections that occur in multiple organs (Ventura and Paperna, Reference Ventura and Paperna1984; Azevedo et al., Reference Azevedo, Lom and Corral1989; Saraiva and Chubb, Reference Saraiva and Chubb1989; Saraiva and Eiras, Reference Saraiva and Eiras1996; Hermida et al., Reference Hermida, Saraiva and Cruz2008). The study by Freeman and Kristmundsson (Reference Freeman and Kristmundsson2018), however, showed that P. giardi is not systemic; while myxospore morphology is conserved and, therefore, unreliable for identification at the species-level, the site of infection appears to be an important diagnostic feature for the group. As such, it is expected that the myxozoan survey of A. anguilla in Portuguese Rivers will lead to the discovery of other Paramyxidium spp.
Author ORCIDs
S. Rocha, 0000-0001-6230-6142
Acknowledgements
The authors acknowledge Professor Eduardo Rocha and the Laboratory of Histology of the Institute of Biomedical Sciences, University of Porto, for the usage of the Olympus BX50 light microscope, as well as Miguel Pereira for his iconographic assistance.
Financial support
This work was financially supported by the Foundation for Science and Technology (Lisbon, Portugal) within the scope of the Ph.D. fellowship grant attributed to S. Rocha (SFRH/BD/92661/2013) through the programme QREN-POPH/FSE; and the Eng° António de Almeida Foundation (Porto, Portugal).
Conflicts of interest
None.
Ethical standards
Not applicable.