1. Introduction
The Qinling–Dabie–Sulu ultrahigh-pressure (UHP) metamorphic belt formed as a result of a Triassic collision between the North China and Yangtze cratons (Zhai et al. Reference Zhai, Cong, Zhang and Wang1994; Hacker et al. Reference Hacker, Ratschbacher, Webb, Ireland, Walker and Dong1998, Reference Hacker, Wallis, Ratschbacher, Grove and Gehrels2006; Liu et al. Reference Liu, Jian, Kroner and Xu2006, Reference Liu, Gu, Rolfo and Chen2011a ; Li et al. Reference Li, Kusky, Zhao, Liu, Zhang, Kopp and Wang2010). Since the discovery of coesite and micro-diamond inclusions in eclogites in the Dabie orogen in Central East China (Okay, Xu & Şengör, Reference Okay, Xu and Şengör1989; Wang, Liou & Mao, Reference Okay, Xu and Şengör1989; Xu et al. Reference Xu, Okay, Ji, Sengör, Su, Liu and Jiang1992), this region has become one of the most important targets for studying UHP metamorphism that documents continental subduction to mantle depths (Hacker et al. Reference Hacker, Ratschbacher, Webb, Ireland, Walker and Dong1998; Jahn et al. Reference Jahn, Wu, Lo and Tsai1999; Chen, Jahn & Wei, Reference Chen, Jahn and Wei2002; Rumble, Liou & Jahn, Reference Rumble, Liou and Jahn2003; Wang et al. Reference Wang, Wyman, Xu, Jian, Zhao, Li, Xu, Ma and He2007; Liu & Liou, Reference Liu and Liou2009; Liou et al. Reference Liou, Ernst, Zhang, Tsujimori and Jahn2009). Further investigations of UHP minerals and exsolution microstructures show that the continental crust was subducted to more than 120 to 200 km, and was rapidly exhumed from mantle depths back to crustal levels (Ye, Cong & Ye, Reference Ye, Cong and Ye2000). Sensitive high-resolution ion microprobe (SHRIMP) zircon U–Pb data and inferred pressure–temperature (P–T) paths of the metamorphic rocks show that UHP metamorphic events occurred between 240 and 230 Ma followed by an amphibolite-facies retrogression between 220 and 200 Ma (Hacker et al. Reference Hacker, Ratschbacher, Webb, Ireland, Walker and Dong1998; Zheng et al. Reference Zheng, Wang, Li and Zhao2002; Liu et al. Reference Liu, Jahn, Liu, Dong and Li2004). This result shows that the protoliths of UHP metamorphic rocks were subducted to mantle depths in the early to middle Triassic, and exhumed to mid-crustal levels in the late Triassic. This conclusion has been confirmed by other geochronological studies, which show that UHP–HP metamorphism took place between 245 and 210 Ma (Hacker et al. Reference Hacker, Ratschbacher, Webb, Ireland, Walker and Dong1998, Reference Hacker, Wallis, McWilliams and Gans2009; Liu et al. Reference Liu, Jahn, Liu, Dong and Li2004, Reference Liu, Jian, Kroner and Xu2006; Zheng et al. Reference Zheng, Wu, Gong, Chen, Tang and Zhao2007 b; Zheng, Chen & Zhao, Reference Zheng, Chen and Zhao2009), and was followed by rapid cooling from peak metamorphic temperatures to 200°C between 210 and 170 Ma (Hacker et al. Reference Hacker, Ratschbacher, Webb, Ireland, Walker and Dong1998; Liu et al. Reference Liu, Jahn, Liu, Dong and Li2004).
Although some degree of melting has taken place during subduction and subsequent exhumation of continental crust in other UHP metamorphic belts (Hermann et al. Reference Hermann, Rubatto, Korsakov and Shatsky2001), the lack of Triassic and Jurassic granites or orogenic magmatism (Tsai et al. Reference Tsai, Lo, Liou and Jahn2000) in the Dabie orogen indicates that significant syn-collisional or subsequent partial melting of subducted continental crust did not happen. In contrast, Cretaceous post-collisional intrusive and extrusive rocks compose 47% of the surface exposure of the Dabie orogen (Hacker et al. Reference Hacker, Ratschbacher, Webb, Ireland, Walker and Dong1998; Ratschbacher et al. Reference Ratschbacker, Hacker, Webb, McWilliams, Ireland, Dong, Calvert, Chateigner and Wenk2000; Bryant et al. Reference Bryant, Ayers, Gao, Miller and Zhang2004; Zhao et al. Reference Zhao, Zheng, Wei, Chen, Liu and Wu2008; Xie, Chen & Cui, Reference Xie, Chen and Cui2010). The timing of emplacement of the intrusive and volcanic rocks suggests that post-collisional melting in the Dabie orogen lagged by 50 to 70 Ma in comparison to other collision orogenic belts such as the Himalayas. Early Cretaceous magmatic activity was widespread throughout eastern China resulting from subduction of the Pacific oceanic plate (Xu, Ma & Zhang, Reference Xu, Ma and Zhang2012; Xu et al. Reference Xu, Ma, Zhang and Ye2013). Although partial melting is a significant process in the evolution of orogens (Brown, Reference Brown2001), the ages of partial melting events in the Dabie orogen are still uncertain.
U–Pb dating and Hf isotope analyses of zircon grains can provide age constraints and new insights into the origin of source rocks of granitic intrusions (Kemp et al. Reference Kemp, Hawkesworth, Paterson and Kinny2006; Zhang et al. Reference Zhang, Zheng, Wu, Zhao, Gao and Wu2006; Zheng et al. Reference Zheng, Zhao, Wu, Zhang, Liu and Wu2006, Reference Zheng, Wu, Gong, Chen, Tang and Zhao2007a , Reference Zheng, Wu, Wu, Zhang, Yuan and Wu2008; Zhao et al. Reference Zhao, Zheng, Wei and Wu2011; Xu, Ma & Zhang, Reference Xu, Ma and Zhang2012; Xu et al. Reference Xu, Ma, Zhang and Ye2013). Thus, these methods are used to test the competing tectonic hypotheses. In this contribution, we report new laser ablation inductively coupled plasma mass spectrometry (LA-ICP-MS) and SHRIMP zircon U–Pb ages, major and trace elements, and zircon Hf isotopic data for granitic intrusions at Tiantangzhai in the North Dabie Complex to unravel the petrogenesis and geodynamic evolution of these intrusions. Specifically, we explain how delamination of the lower continental crust triggered magmatic activity in the North Dabie orogen in the early Cretaceous.
2. Geological setting
The Dabie–Sulu orogen is the eastern part of the Qinling–Dabie orogen (Fig. 1) that formed after Triassic collision between the North China and Yangtze cratons (Cong, Reference Cong1996; Zheng et al. Reference Zheng, Fu, Gong and Li2003; Zhao et al. Reference Zhao, Zheng, Wei and Wu2011). The Dabie Mountains are composed of the following tectonic units (from south to north): (1) the Triassic Yangtze foreland fold and thrust belt; (2) the Southern Dabie HP/UHP metamorphic complex; (3) the Northern Dabie complex unit; and (4) the North Huaiyang greenschist-facies meta-sedimentary unit. All four tectonic units are intruded by voluminous post-collisional Cretaceous granitic rocks and mafic-ultramafic rocks (Jahn et al. Reference Jahn, Wu, Lo and Tsai1999; Ma et al. Reference Ma, Ehlers, Xu, Li and Yang2000; Zhang et al. Reference Zhang, Gao, Zhong, Zhang, Zhang and Hu2002; Chen et al. Reference Chen, Ma, She, Mason, Zhang and Zhang2009). The relative abundance of magmatic rock types is variable in each unit. Anatexis of orogenic lithosphere resulting from Triassic continent–continent collision is used to explain the origin of these igneous rocks (Zhao et al. Reference Zhao, Zheng, Wei and Wu2004, Reference Zhao, Zheng, Wei, Wu, Chen and Jahn2005, Reference Zhao, Zheng, Wei and Wu2007a ,Reference Zhao, Chen, Zheng, Chen and Wub, Reference Zhao, Zheng, Wei, Chen, Liu and Wu2008; Wu et al. Reference Wu, Zheng, Tang, Gong, Zhao and Liu2007; Xie, Chen & Cui, Reference Xie, Chen and Cui2010).

Figure 1. (a) Sketch map of part of the Dabie orogen modified from Bryant et al. (Reference Bryant, Ayers, Gao, Miller and Zhang2004), with insert showing the location of this area within the Triassic Qinling–Dabie–Sulu orogenic belt in east-central China (red solid line indicates study area); (b) geological map of the Tiantangzhai intrusive complex showing sample locations and weighted average zircon 206Pb–238U ages; (c) cross-section through the North Dabie country rocks and Tiantangzhai intrusive complex.
A number of geochronological studies show that UHP metamorphism in the Dabie–Sulu orogenic belt occurred in the Triassic (Hacker et al. Reference Hacker, Ratschbacher, Webb, Ireland, Walker and Dong1998; Liu et al. Reference Liu, Jian, Kroner and Xu2006, Reference Liu, Hu, Gao, Feng, Zhong, Qi, Wang, Feng and Yang2011b ). For example, SHRIMP zircon U–Pb dates for UHP metamorphism in the coesite stability field range from 242±2 to 227±2 Ma (Liu et al. Reference Liu, Jian, Kroner and Xu2006; Wu et al. Reference Wu, Zheng, Zhao, Gong, Liu and Wu2006). Zircon U–Pb ages determined by thermal ionization mass and secondary ion mass spectrometries range from 620 to 880 Ma (peak range from 740 to 780 Ma) for the protoliths of most eclogites and granitic gneisses in the Dabie–Sulu orogenic belt (Zheng et al. Reference Zheng, Fu, Gong and Li2003, Reference Zheng, Wu, Chen, Gong, Li and Zhao2004). This result is derived from bimodal magmatism along the northern margin of the South China Block associated with mid-Neoproterozoic rift tectonics (Zheng et al. Reference Zheng, Fu, Gong and Li2003; Zhao et al. Reference Zhao, Zheng, Gao, Wu, Chen, Chen and Wu2006, Reference Zhao, Zheng, Wei and Wu2007a ,Reference Zhao, Chen, Zheng, Chen and Wu b ).
The North Dabie Complex (NDC) is bounded by the Xiaotian–Mozitan fault to the north, the Wuhe–Shuihou fault to the south, the Shangcheng–Macheng fault to the west and the Tancheng–Lujiang fault to the east (Fig. 1a), and is transected by several strike-slip faults. The Tancheng–Lujiang fault is a left-lateral syn-orogenic transform fault formed at 236 Ma and its major strike-slip offset took place in the Cretaceous (132 to 128 Ma). Distributions of the Cretaceous granites generally follow the Tancheng–Lujiang fault, and these two faults are also spatially associated in the study area (Chen, Jahn & Wei, Reference Chen, Jahn and Wei2002; Zhu et al. Reference Zhu, Wang, Liu, Niu, Xie and Li2005, Reference Zhu, Niu, Xie and Wang2010; Wang et al. Reference Wang, Chen, Griffin, O'Reilly, Huang and Li2011 b; Xu, Ma & Zhang, Reference Xu, Ma and Zhang2012; Xu et al. Reference Xu, Ma, Zhang and Ye2013). The NNE-trending Xiaotian–Mozitan fault is the northern boundary of the NDC and formed in the late Jurassic (> 141 Ma). This post-orogenic extensional fault shows that the NDC metamorphic core complex was assembled and uplifted in the early Cretaceous (130 to 120 Ma) (Wang et al. Reference Wang, Wei, Xiang, Zhu, Niu and Xie2010, Reference Wang, Chen, Griffin, O'Reilly, Huang and Li2011a ).
The Tiantangzhai intrusive complex of the NDC covers an area of > 400 km2 (Fig. 1b). This intrusive complex is divided into six sub-intrusions: the Shigujian, Egongbao, Tiantangzhai, Jiuzihe, Bodaofeng and Erwan granites (Fig. 1c). Previous studies (Hacker et al. Reference Hacker, Ratschbacher, Webb, Ireland, Walker and Dong1998; Bryant et al. Reference Bryant, Ayers, Gao, Miller and Zhang2004; Zhao et al. Reference Zhao, Zheng, Wei, Wu, Chen and Jahn2005; Wang et al. Reference Wang, Wyman, Xu, Jian, Zhao, Li, Xu, Ma and He2007) reported zircon ages of 141 Ma, 139 to 131 Ma and 125 Ma for the Tiantangzhai intrusive complex (Fig. 1b). The NNE-trending Shigujian intrusion was emplaced at 141 Ma, and shows the strongest deformation and local migmatization. The Egongbao, Tiantangzhai, Jiuzihe and Erwan weakly deformed intrusions were emplaced between 139 and 131 Ma. Undeformed post-tectonic granites were emplaced after 132 Ma. Gneissic structures of older granites show that the Shigujian intrusion is syn-tectonic and coeval with the formation of the NNE compressional shear-related migmatization. But the younger homogeneous granites are emplaced under an extensional regime in the Dabie orogen (Deng, Wu & Yang, Reference Deng, Wu and Yang2013).
The Shigujian intrusion is a syn-tectonic gneissic granite. The foliation in this gneiss dips at 45 to 70°SE, which is similar to the gneissosity in the surrounding gneisses and the Tancheng–Lujiang fault zone. The Shigujian granitic gneiss shows very strong deformation and local migmatization, and is intruded by the Egongbao granite (Fig. 2a). Field relationships and previous dating (Table 1) show that the Shigujian intrusion is the oldest Cretaceous granite in the study area (Ma et al. Reference Ma, Li, Ehlers, Yang and Wang1998; Xu, Ma & Ye, Reference Xu, Ma and Ye2007; Deng, Wu & Yang, Reference Deng, Wu and Yang2013). Foliation in the Shigujian quartz monzodiorite dips NNE, which is consistent with the ductile shear belt and characteristics of the Tancheng–Lujiang fault zone in the NDC.
Table 1. Geochronological data of the granite in the Tiantangzhai intrusive complex


Figure 2. Field photographs of the Tiantangzhai intrusive complex (contact highlighted by yellow dotted curve). (a) Egongbao granite intruded into Shigujian granite; (b) Egongbao augen monzonitic granite with strong deformation in the NW-trending ductile shear zone and migmatization; (c) no deformation in the centre of the Tiantangzhai granite; (d) a NE-striking left-lateral ductile shear belt in the Jiuzihe granite; (e) homogeneous and fine granular texture of the Bodaofeng granite; (f) two dykes intruded into the Tiantangzhai granite; the earlier is an acidic dyke and the latter is a basic dyke.
The Egongbao gneissic and augen monzonitic granites have an equigranular texture. The NW-trending ductile shear zone deformed this granite. The Egongbao granite underwent local migmatization along the ductile shear zone. However, almost no deformation is found outside the shear zone (Fig. 2b). Previous dating shows that the intrusion was emplaced between 143 and 142 Ma (Wang et al. Reference Wang, Wyman, Xu, Jian, Zhao, Li, Xu, Ma and He2007).
The Tiantangzhai intrusion is composed of weakly deformed, homogeneous granite and granodiorite possessing weak gneissic texture and rare inclusions. This intrusion intrudes into the Jiuzihe granite, and has no ductile deformation fabric (Fig. 2c). This observation indicates that the emplacement of the Tiantangzhai granite postdates the intrusion of the Jiuzihe granite and formation of the NE-trending, left-lateral ductile shear zone. Zircon U–Pb ages in porphyritic granite and mylonites are 132.3±1 and 127.9±0.8 Ma, respectively (Xu, Ma & Ye, Reference Xu, Ma and Ye2007).
The Jiuzihe granite consists of banded and porphyritic monzonitic domains, where the banded granite is more widespread than the porphyritic monzonitic domains. The Jiuzihe granite has weakly developed foliation that dips SE at the edge of the intrusion. However, almost no deformation is found in the centre of the Jiuzihe granite. After or during the emplacement of the Jiuzihe granite, the NE-trending sinistral ductile shear belt significantly deformed the granite and is associated with its migmatization (Fig. 2d).
The Erwan quartz diorite has an inequigranular texture. One granite sample has yielded a zircon U–Pb age of 131±4 Ma.
The Bodaofeng granite is the youngest Cretaceous intrusion in the area, which cuts across all granites and gneisses in the area (Fig. 2e). This granite, without any deformation and inclusions, shows large-scale crustal extension in East China. One monzonitic granite sample has yielded a zircon U–Pb age of 132±2 Ma. Two kinds of dykes intruded into the Tiantangzhai granite and Dabie gneiss. The older dyke is felsic in composition whereas the younger one is a mafic dyke (Fig. 2f).
In summary, field relationships and zircon U–Pb dating show that the late Mesozoic magmatic intrusion sequences progressed from the Shigujian granite through the main body of the Cretaceous granites (e.g. Egongbao, Tiantangzhai, Jiuzihe and Erwan granites) to the latest Bodaofeng granite.
3. Petrography and sampling
The Shigujian granite is composed of K-feldspar (25%) and plagioclase (35%). The Egongbao granite to monzonite is characterized by coarse granular (1 cm) phenocrysts of K-feldspar (1 to 5%). The Tiantangzhai granite and granodiorite have an equigranular texture. Both of these rocks contain coarse granular (0.6 to 2.0 cm) K-feldspar phenocrysts, fine- to medium-grained (0.05 to 0.35 cm) K-feldspar, plagioclase, quartz, biotite, hornblende, minor albite and accessory minerals such as titanite, magnetite and zircon (Fig. 3). K-feldspar phenocrysts are distributed unevenly in the intrusion, and their modal abundance ranges from 1 to 30%. The Jiuzihe granite has a similar texture to that of the Tiantangzhai granite and granodiorite. This granite consists of plagioclase (25 to 35%), quartz (20 to 30%), K-feldspar (29 to 35%), biotite (3 to 8%) and accessory minerals such as titanite, magnetite, apatite and zircon. The Erwan quartz diorite has an inequigranular texture containing K-feldspar (5 to 10%), plagioclase (59 to 62%), quartz (9 to 15%), hornblende (3 to 5%), biotite (7 to 21%) and accessory minerals such as magnetite (~1%), titanite (~3%), apatite (0.5%) and zircon (<1%). The later Bodaofeng granite shows a homogeneous and fine granular texture consisting of K-feldspar (32–44%), quartz (20%), plagioclase (20 to 35%), biotite (18%) and accessory minerals (magnetite, titanite and zircon).

Figure 3. Photomicrographs of the Tiantangzhai intrusive complex. Qz – quartz; FK – K-feldspar; Pl – plagioclase; Bi – biotite.
For this study, eight granite samples (DT07-2, DT16, DT18, DT20-1, DT20-2, DT22, DT31 and DT36) were collected in the NDC (Fig. 1b). These samples were used for the integrated study including cathodoluminescence (CL) imaging for interpreting zircon internal structures and LA-ICP-MS and SHRIMP for U–Pb dating. Three granite samples (DT07-2, DT20-1 and DT31) were used for zircon Hf isotope analyses. Twenty granite and four orthogneiss samples were analysed for the major and trace elements. Global Positioning System locations for the samples are listed in Table 2.
Table 2. GPS locations for samples of the Tiantangzhai intrusive complex and Dabie gneiss

Pl – plagioclase; Kfs – K-feldspar; Hb – hornblende; Qz – quartz; Bi – biotite; Ttn – titanite; Mt – magnetite; Zrn – zircon; Ap – apatite.
4. Analytical methods
4.a. Whole-rock major and trace element analyses
The analyses of major elements were conducted at the Analytical Institute of the Hubei Bureau of Geology and Mineral Resources using a Regaku 3080E XRF spectrometer. Trace element analyses were performed using the LA-ICP-MS at the State Key Laboratory of Geological Processes and Mineral Resources in China University of Geosciences, China. Regular settings of the laser source, mass spectrometer and standardizing procedures were described in detail by Liu et al. (Reference Liu, Hu, Gao, Gunther, Xu, Gao and Chen2008). The results are listed in Table 3.
Table 3. Major (wt%) and trace (ppm) element concentrations for the Tiantangzhai intrusive complex and orthogneiss in the NDC

Major element compositions of minerals were measured with a JEOL JXA 8100 electron microprobe; trace element analyses were conducted using the laser ablation ICP-MS at State Key Laboratory of Geological Processes and Mineral Resources in China University of Geosciences, China.
4.b. Zircon U–Pb dating analysis
LA-ICP-MS U–Pb analyses were performed in the China University of Geosciences at Wuhan. The technique was described in detail by Liu et al. (Reference Liu, Hu, Gao, Gunther, Xu, Gao and Chen2008). A GeoLas 200M LA system equipped with a 193 nm ArF-excimer laser was used with the ELAN6100 DRC ICP-MS. Helium was used as the carrier gas to enhance the transport efficiency of the ablated material. Helium carrier gas inside the ablation cell was mixed with argon before entering the ICP to maintain stable and optimum excitation conditions. The spot diameter for each analysis was 32 μm, and data were collected during 60 s of ablation after approximately 20 s of background counting. All measurements were performed using zircon 91500 as the reference standard with a recommended 206Pb–238U age of 1065.4±0.6 Ma. Common Pb correction was made using the EXCEL spreadsheet ComPbCorr#3_151 (Andersen, Reference Andersen2002). U–Pb ages were calculated using the ISOPLOT program (Ludwig, Reference Ludwig2001). The results are listed in Table 4 (with 2σ errors of the mean for isotopic ratios and calculated ages).
Table 4. LA-ICP-MS and SHRIMP zircon U–Pb isotope data for zircons from the Egongbao, Tiantangzhai, Jiuzihe, Erwan and Bodaofeng granites in the NDC

DT16 (JZH-3)* using SHRIMP zircon U–Pb dating
SHRIMP U–Pb analyses were performed using SHRIMP II at the Beijing SHRIMP Centre in the Chinese Academy of Geological Sciences. Instrumental conditions and data acquisition were performed according to those described by Williams (Reference Williams1998). Intensity of the primary O2-ion beam was 9 nA. Spot sizes were ~30 μm at 150 s raster before isotopic analysis. Five scans of the mass stations were made for each age determination, and reference zircon TEMORA (417 Ma) was analysed after every fourth analysis. Common Pb was corrected using the measured 204Pb. All data were processed using the SQUID and ISOPLOT programs of Ludwig (Reference Ludwig2001). The results are listed in Table 4 (errors are reported with 2σ uncertainties).
4.c. Zircon Lu–Hf isotope analysis
In situ zircon Lu–Hf isotopic measurements were obtained using the Nu Plasma high-resolution multi-collector ICP-MS, which is equipped with a 193 nm ArF laser at the Northwest University in Xi'an. The analyses were performed with a spot size of 44 μm, 10 Hz repetition rate and 100 mJ pulse−1 laser power. Interference of 176Lu on 176Hf was corrected by measuring the intensity of the interference-free 175Lu using the recommended 176Lu/175Lu ratio of 0.02669 to calculate 176Lu/177Hf. Isobaric interference of 176Yb on 176Hf was corrected by using a recommended 176Yb/172Yb ratio of 0.5886 (Chu, Taylor & Chavagnac, Reference Chu, Taylor and Chavagnac2002) to calculate 176Hf/177Hf ratios. Zircon 91500 was used as the reference standard (Blichert-Toft & Albarede, Reference Blichert-Toft and Albarede1997). Details of the operating conditions and data reduction were described by (Yuan et al. Reference Yuan, Gao, Liu, Li, Gunther and Wu2004). To characterize the source of protoliths, initial Hf isotope ratios were calculated based on the chondritic reservoir at the time of magma crystallization that corresponds to the age of zircon growth from the magma (Zheng et al. Reference Zheng, Zhao, Wu, Zhang, Liu and Wu2006). A decay constant of 176Lu 1.865 × 10−11 yr−1 (Scherer, Münker & Mezger, Reference Scherer, Münker and Mezger2001) and chondritic ratios of 176Hf/177Hf (0.282785) and 176Lu/177Hf (0.0336) were used. Two-stage Hf model ages (T DM2) were calculated by assuming a mean 176Lu/177Hf value of 0.015 for the average continental crust (Griffin et al. Reference Griffin, Wang, Jackson, Pearson, O'Reilly, Xu and Zhou2002). The results are presented in Table 5.
Table 5. LA-MC-ICP-MS zircon Lu–Hf isotope data for the Tiantangzhai intrusive complex

5. Results
5.a. Major and trace elements
The Tiantangzhai Complex shows a minor variation in major and trace element abundances (Table 3). Major element compositions are clustered very closely on variation diagrams (Fig. 4). The SiO2 contents are between 68.7 and 75.0 wt%. The contents of Al2O3, MgO, FeO, TiO2, CaO and P2O5 show negative correlations with SiO2. In contrast, the K2O and Na2O contents in orthogneiss show positive correlations. On the Na2O+K2O versus SiO2 diagram (Fig. 4a), the Tiantangzhai intrusions plot in the granite and granodiorite fields. These rocks contain unusually low abundances of CaO (<3.5 wt%), TiO2 (<0.5 wt%), MgO (<2% wt%), FeOt (<3 wt%) and relatively low Sr, but are highly enriched in K2O (>3.2 wt%), Rb (65 to 222 ppm), Ba (587 to 2388 ppm) and Sr/Y (5.7–622) (Table 3). These rocks are metaluminous to weakly peraluminous (A/CNK = 0.89–1.05; Fig. 4b), and are high-K calc-alkaline to shoshonite in composition (Fig. 4d). On the Na2O–K2O diagram (Fig. 4c), the majority of the samples plot in the field of A-type granite, but the Shigujian samples plot in the field of I-type granite.

Figure 4. (a) Na2O+K2O versus SiO2 diagram of the Cretaceous granites in the Tiantangzhai area; (b) Al2O3/(Na2O+K2O) versus Al2O3/(CaO+Na2O+K2O) diagram; (c) Na2O versus K2O diagram; (d) K2O versus SiO2 diagram.
All granitic intrusions in the NDC are characterized by light rare earth element (LREE)-enriched patterns and negative Nb, Ta, P and Ti anomalies (Fig. 5). These rocks display superchondritic (La/Yb)N (13–87) and (Gd/Yb)N (1.43–5.50) ratios. The Tiantangzhai, Jiuzihe, Egongbao and Erwan granites do not have significant Eu anomalies (Fig. 5a), whereas the Dabie Complex and Shigujian granite show positive Eu anomalies (Fig. 5b). The Bodaofeng granite is characterized by variable negative Eu anomalies (Fig. 5c). Both the Tiantangzhai and Dabie complexes show strong negative Nb, Ta, P and Ti anomalies and pronounced positive Rb, Th, K and La (and LREE) anomalies on extended trace element diagrams (Fig. 5d). The Shigujian granite plots in the adakite field, but the majority of other granites fall in the normal andesite–dacite–rhyolite field on the (La/Yb)N versus YbN and Sr/Y versus Y diagrams (Fig. 6a, b).

Figure 5. (a, b, c) Chondrite-normalized REE patterns for the Tiantangzhai intrusive complex and NDC country rocks showing highly fractionated REE patterns; (a) shows no Eu anomalies; (b) shows positive Eu anomalies; (c) shows pronounced negative Eu anomalies. (d) Primitive mantle (PM)-normalized spidergrams for the Tiantangzhai intrusive complex showing high abundances of Rb, Th, K and La (LREEs), and relative depletion of Nb, Ta, P and Ti. Primitive mantle-normalizing values from Sun & McDonough (Reference Sun, McDonough, Saunders and Norry1989).

Figure 6. (a) LaN/YbN versus YbN diagram; normalizing values from Drummond & Defant (Reference Drummond and Defant1990); all the samples are located in the adakite field. (b) Sr/Y versus Y diagram; normalizing values from Defant et al. (Reference Defant, Xu, Kepezhinskas, Wang, Zhang and Xiao2002); the Shigujian granite and part of the Tiantangzhai Complex are located in the adakite field. Ol – olivine; Pl – plagioclase; Cpx – clinopyroxene; Opx – orthopyroxene.
5.b. Zircon U–Pb ages
Eight LA-ICP-MS and SHRIMP U–Pb analyses were performed on samples from five early granites (Egongbao, Tiantangzhai, Jiuzihe, Erwan and Bodaofeng granite: EGB-1, TTZ-1, TTZ-2, JZH-1, JZH-2, JZH-3, EW-1, BDF-1). The zircons are mostly prismatic with well-developed pyramidal faces. Cathodoluminescence images of the zircons clearly display oscillatory zoning in most crystals and the presence of old cores in some zircons (Fig. 7). The results of the LA-ICP-MS and SHRIMP U–Pb zircon analyses are given in Table 4 and are illustrated on a concordia plot in Figure 8. The zircons have high Th/U ratios of 0.11–2.87, which are typical of an igneous origin (Wu & Zheng, Reference Wu and Zheng2004). The U–Pb ages plot close to the concordia curve with apparent 206Pb–238U ages ranging from 111±3 to 151±2 Ma, displaying two peaks at 137 and 125 Ma. These ages suggest that zircon crystallization is related to the partial melting events between 137 and 125 Ma. Three metamorphic zircon analyses have yielded ages of 202±3 to 228±2 Ma, indicating a record of HP–UHP metamorphism. The large age span probably reflects re-melting during the emplacement processes of the younger granites. Scatter in ages might have resulted from Pb loss during later metamorphic events. The four oldest 206Pb–238U ages yield a weighted mean age of 804±6 to 812±7 Ma; these Neoproterozoic ages are interpreted as the ages of protolith formation.
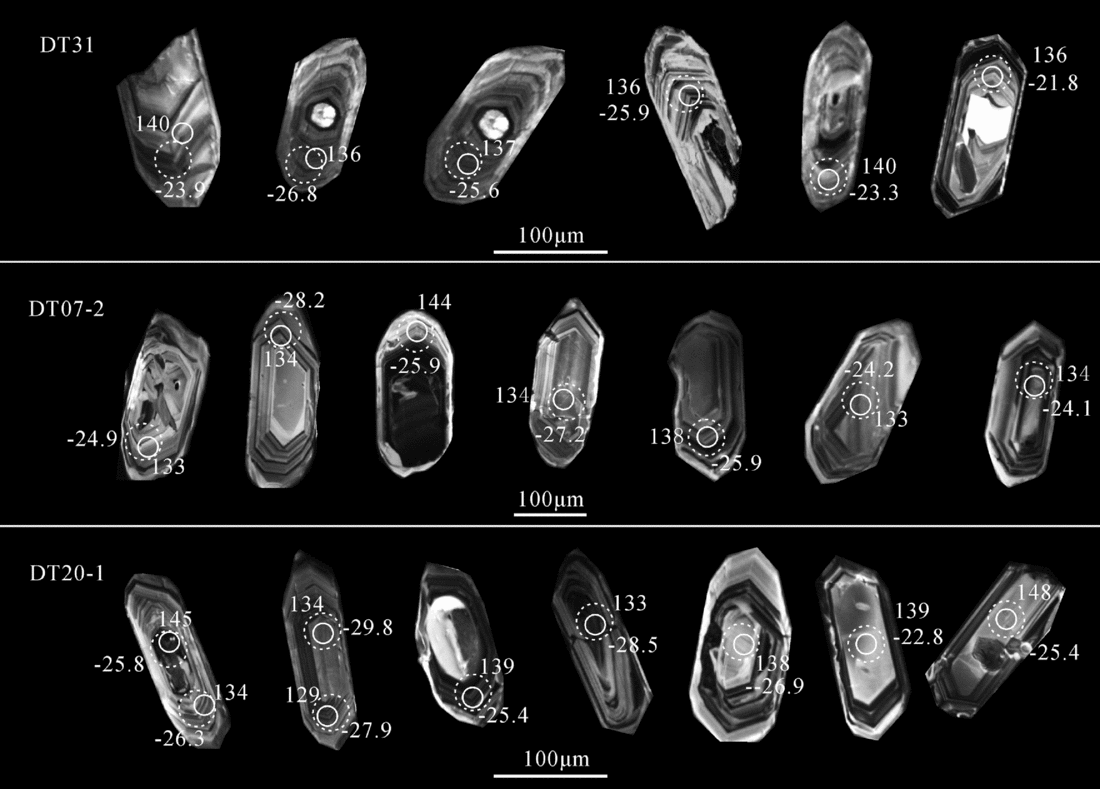
Figure 7. CL images of zircon grains with initial Hf isotope ratios as εHf(t) and 206Pb–238U ages for the Tiantangzhai, Jiuzihe and Egongbao granites.

Figure 8. Zircon U–Pb concordia diagrams for the Tiantangzhai intrusive complex in the NDC. (a) Egongbao granite using LA-ICP-MS dating; (b, c) Tiantangzhai granite using LA-ICP-MS dating; (d, e, f) Jiuzihe granite, with (f) using SHRIMP dating; (g) Erwan granite using LA-ICP-MS dating; (h) Bodaofeng granite using LA-ICP-MS dating.
One sample was collected from the Egongbao granite (DT31). Thirteen analyses of zircon grains from DT31 (EGB-1) have yielded 206Pb–238U ages ranging between 132 and 148 Ma, with a weighted mean age of 139±3 Ma (MSWD = 2) (Table 4; Fig. 8a).
Samples DT07-2 and DT18 were collected from the Tiantangzhai granite. Twenty analyses of zircon grains from sample DT07-2 (TTZ-1) have yielded 206Pb–238U ages ranging between 127 and 145 Ma, with a weighted mean age of 135±2 Ma (MSWD = 6) (Table 4; Fig. 8b). One zircon core from sample DT07-2 also gives a discordant age of 201.8±2.6 Ma. Fourteen analyses of zircon grains from sample DT18 (TTZ-2) have yielded 206Pb–238U ages ranging from 125 to 150 Ma, with a weighted mean age of 138±4 Ma (MSWD = 4.6) (Table 4; Fig. 8c), providing the best estimate for the crystallization age of the Tiantangzhai granite. Four zircons from the metamorphic zircon cores have yielded coherent 206Pb–238U ages between 224 and 228 Ma, and between 804 and 812 Ma, which are interpreted as representing the best age estimate of the source of the Tiantangzhai granite. The emplacement age of the Tiantangzhai granite is estimated to be between 135 and 138 Ma from these two samples.
Three samples were collected from the Jiuzihe granite (DT20-1, DT20-2 and DT16). Twenty analyses of zircon grains from DT20-1 (JZH-1) have yielded 206Pb–238U ages ranging between 125 and 148 Ma, with a weighted mean age of 136±3 Ma (MSWD = 10) (Table 4; Fig. 8d). Nine analyses of zircon grains from DT20-2 (JZH-2) have yielded 206Pb–238U ages ranging between 128 and 151 Ma, with a weighted mean age of 134±6 Ma (MSWD = 9) (Table 4; Fig. 8e). Eight SHRIMP analyses of zircons from DT16 (JZH-3) provide 206Pb–238U ages between 131 and 138 Ma, with a weighted mean age of 135±2 Ma (MSWD = 0.86) (Table 4; Fig. 8f), which is interpreted as the crystallization age of the Jiuzihe granite. Given the combination of the three samples, the emplacement age of the Jiuzihe granite ranges from 134 to 136 Ma.
Twelve analyses of zircon grains from sample DT22 (EW-1) from the Erwan intrusion have yielded 206Pb–238U ages ranging between 111 and 138 Ma, with a weighted mean age of 131±4 Ma (MSWD = 6) (Table 4; Fig. 8g), which is the crystallization age of the Erwan granite. The thin rims on two zircon grains have also yielded 206Pb–238U ages of 111 and 117 Ma. The rim age is similar to the crystallization ages (116 to 118 Ma) of the late Baimajian, Zhuboyuan and Yunfengding granites in the NDC (Bryant, et al. Reference Bryant, Ayers, Gao, Miller and Zhang2004; Wang, et al. Reference Wang, Wyman, Xu, Jian, Zhao, Li, Xu, Ma and He2007; Zhang, et al. Reference Zheng, Wang, Li and Zhao2002). The age range indicates that late magmatism might have caused the recrystallization of the rims of some zircons in the Erwan granite.
Fifteen analyses of zircon from sample DT36 (BDF-1) have yielded a 206Pb–238U age range between 127 and 143 Ma, with a weighted mean age of 132±2 Ma (MSWD = 2) (Table 4; Fig. 8h), which is interpreted as the crystallization age of the Bodaofeng granite.
5.c. Zircon Lu–Hf isotopes
The zircon grains in all samples dated through the LA-ICP-MS U–Pb method were analysed for Lu–Hf isotopes. Twenty Lu–Hf analyses were obtained for the 18 zircon grains from sample DT31 (Table 5; Fig. 7). The 176Hf/177Hf ratios in sample DT31 range from 0.281929 to 0.282070, corresponding to εHf(t) values of −26.8 to −21.8 at t = 139 Ma. All samples have a weighted mean εHf(t) value of −24.8±0.6 (MSWD = 3.6) (Fig. 9a), and have yielded a weighted average two-stage Hf model age (T DM2) of 2748±34 Ma (MSWD = 0.9) (Fig. 9b).

Figure 9. Histograms of εHf(t) values and two-stage Hf model ages (T DM2) for zircons from the Egongbao, Tiantangzhai and Jiuzihe granites. All the analyses of zircons are negative.
The 176Hf/177Hf ratios in 14 zircon grains from sample DT07-2 range from 0.281861 to 0.282009, corresponding to εHf(t) values of −29.3 to −24.1 at t = 135 Ma, with a weighted mean εHf(t) value of −26.2±0.7 (MSWD = 5.3) (Fig. 9c). These grains have yielded a weighted average T DM2 value of 2843±43 Ma (MSWD = 1.3) (Fig. 9d).
Fourteen Lu–Hf analyses were performed on nine zircon grains from sample DT20-1 (Table 5; Fig. 7). The nine zircon grains in sample DT20-1 have 176Hf/177Hf ratios between 0.281887 and 0.282042, corresponding to εHf(t) values of −28.5 to −22.8 at t = 136 Ma. The other 13 analyses, excluding analysis #8 that has abnormally high εHf(t) values, have yielded a weighted mean εHf(t) value of −26.6±0.8 (MSWD = 5.1) (Fig. 9e), corresponding to T DM2 value of 2864±40 Ma (MSWD = 1.1) (Fig. 9f).
6. Discussion
6.a. Constraints on the source rocks and Triassic metamorphism
Several hypotheses have been proposed to explain the formation of the NDC, including the following: (1) a Palaeozoic magmatic arc that formed in the hanging wall of the North China Block (Zhai et al. Reference Zhai, Cong, Zhang and Wang1994); (2) a magmatic complex that was produced almost entirely through post-collisional extensional tectonic processes in the early Cretaceous (Hacker et al. Reference Hacker, Ratschbacher, Webb, Ireland, Walker and Dong1998); (3) a part of the subducted South China Block that experienced UHP metamorphism (Tsai et al. Reference Tsai, Lo, Liou and Jahn2000; Zheng et al. Reference Zheng, Zhou, Wu and Xie2005); and (4) an extension of the South China Block that was unaffected by Triassic UHP metamorphism (Bryant et al. Reference Bryant, Ayers, Gao, Miller and Zhang2004). The available data in the literature suggest that the Dabie granites originated from the Yangtze Craton (Hacker et al. Reference Hacker, Ratschbacher, Webb, Ireland, Walker and Dong1998; Bryant et al. Reference Bryant, Ayers, Gao, Miller and Zhang2004; Zheng et al. Reference Zheng, Wu, Chen, Gong, Li and Zhao2004; Wang et al. Reference Wang, Wyman, Xu, Jian, Zhao, Li, Xu, Ma and He2007; Wu et al. Reference Wu, Zheng, Tang, Gong, Zhao and Liu2007; Zhao et al. Reference Zhao, Zheng, Wei, Chen, Liu and Wu2008).
Two zircon cores from the sample TTZ-2 have given consistent U–Pb ages between 812 and 804 Ma (Table 4), with high Th/U ratios ranging from 0.87 to 1.03. The inherited cores show oscillatory or planar zoning, suggesting that these cores are magmatic zircons and that the source rocks of the cores experienced different degrees of solid-stage recrystallization. These ages are accordingly interpreted to represent the time of the granite and gneiss protolith formation. Similar zircon U–Pb ages (e.g. 800 to 700 Ma) were previously acquired for the orthogneisses and eclogites of the NDC and UHP metamorphic rocks in the Dabie orogen (Hacker et al. Reference Hacker, Ratschbacher, Webb, Ireland, Walker and Dong1998; Zheng et al. Reference Zheng, Wu, Chen, Gong, Li and Zhao2004; Zhao et al. Reference Zhao, Zheng, Wei, Chen, Liu and Wu2008, Reference Zhao, Zheng, Wei and Wu2011; Xie, Chen & Cui, Reference Xie, Chen and Cui2010). These ages are also consistent with the timing of the magmatic activity in the margins and interiors of the Yangtze Craton (Xiao et al. Reference Xiao, Zhang, Ni, Xiang and Liu2007). Therefore, the protoliths of the granites in this study are the products of magmatism during Neoproterozoic extension of the Yangtze Craton (Zheng et al. Reference Zheng, Wu, Gong, Chen, Tang and Zhao2007 b, Reference Zheng, Wu, Wu, Zhang, Yuan and Wu2008; Zhao et al. Reference Zhao, Zheng, Wei, Chen, Liu and Wu2008).
Zircon U–Pb dating is crucial in deciphering the protolith age for metamorphosed granites. Hafnium isotope analyses can be used to provide further constraints on the origin and nature of the protoliths (Zheng et al. Reference Zheng, Zhao, Wu, Zhang, Liu and Wu2006; Wu et al. Reference Wu, Zheng, Tang, Gong, Zhao and Liu2007). Three samples (DT07-2, DT20-1 and DT31) have yielded εHf(t) values of −26.2±0.7, −26.6±0.8 and −24.8±0.6 (Fig. 9), respectively, which are consistent with the partial melting of ancient continental crust. However, significant variations in the εHf(t) values and T DM2 ages suggest multiple sources for the ancient crust (Fig. 9). These results are consistent with the whole-rock Nd and Hf isotope data obtained from these rocks (Wu et al. Reference Wu, Zheng, Tang, Gong, Zhao and Liu2007; Xu, Ye & Ma, Reference Xu, Ye and Ma2008; Zhao et al. Reference Zhao, Zheng, Wei, Chen, Liu and Wu2008). Zircons in samples DT07-2, DT20-1 and DT31 have given T DM2 ages of 2843±43, 2864±40 and 2748±34 Ma, respectively, which suggest that the zircon protoliths formed through the reworking of the 2.7 to 2.8 Ga Neoarchaean continental crust (Fig. 9). Wu et al. (Reference Wu, Zheng, Tang, Gong, Zhao and Liu2007) also reported similar T DM2 ages (2.5 Ga) for the migmatitic gneisses in the NDC. Proterozoic Nd model ages (1.8 to 2.3 Ga) were also obtained from the Zhuboyuan granites and gneisses in the NDC (Ma et al. Reference Ma, Ehlers, Xu, Li and Yang2000; Chen, Jahn & Wei, Reference Chen, Jahn and Wei2002; Bryant et al. Reference Bryant, Ayers, Gao, Miller and Zhang2004; Wang et al. Reference Wang, Wyman, Xu, Jian, Zhao, Li, Xu, Ma and He2007; Xu, Ma & Ye, Reference Xu, Ma and Ye2007).
The Dabie orogen is the eastern extension of the Qinling–Dabie orogen that resulted from the continental collision between the Yangtze and the North China cratons in the Triassic (Hacker et al. Reference Hacker, Ratschbacher, Webb, Ireland, Walker and Dong1998; Zheng et al. Reference Zheng, Zhou, Wu and Xie2005). Several tectonothermal events are recorded by the zircon U–Pb ages, and by the Sm–Nd, Rb–Sr, Ar–Ar and Lu–Hf isotopic data. Liu, Li & Xu (Reference Xu, Ma and Ye2007 ) and Liu et al. (Reference Liu, Li, Gu, Xu and Chen2007) suggested that the zircon U–Pb ages of 218±3 Ma from a gneiss sample and 212±10 Ma from an eclogite in the NDC represent the time of UHP metamorphism. Three inherited zircon cores in sample TTZ-1 and TTZ-2 have yielded coherent 206Pb–238U ages between 228 and 202 Ma. The Th/U ratios in these zircons are in the range of 0.05–0.96 (Table 4). The low Th/U ratios suggest that the overgrowth is not of a magmatic origin but resulted from fluid precipitation, which indicates that the granite protoliths experienced Triassic metamorphism (Wu & Zheng, Reference Wu and Zheng2004).
6.b. Timing of partial melting in the early Cretaceous
Much of East Asia experienced crustal extension and large-scale magmatism in the early Cretaceous (Zhao et al. Reference Zhao, Zheng, Wei, Wu, Chen and Jahn2005, Reference Zhao, Zheng, Wei and Wu2007b ; Zheng, Chen & Zhao, Reference Zheng, Chen and Zhao2009). Most zircon U–Pb ages for the granites in the NDC fall between 140 and 100 Ma (Hacker et al. Reference Hacker, Ratschbacher, Webb, Ireland, Walker and Dong1998; Bryant et al. Reference Bryant, Ayers, Gao, Miller and Zhang2004; Ma et al. Reference Ma, Yang, Ming and Ling2004; Wang et al. Reference Wang, Wyman, Xu, Jian, Zhao, Li, Xu, Ma and He2007; Xu, Ma & Ye, Reference Xu, Ma and Ye2007; Zhao et al. Reference Zhao, Zheng, Wei, Chen, Liu and Wu2008; Xie, Chen & Cui, Reference Xie, Chen and Cui2010). The formation of the Cretaceous granites is related to extension of the thickened crust (Xie, Chen & Cui, Reference Xie, Chen and Cui2010). Investigations of the migmatites in the NDC documented two peaks at 139±1 and 123±1 Ma (Wu et al. Reference Wu, Zheng, Tang, Gong, Zhao and Liu2007). The spread of ages can be attributed to the presence of melt generation throughout this time interval, whereas the two age peaks may suggest that two major episodes of zircon crystallization are associated with partial melting events (Wu et al. Reference Wu, Zheng, Tang, Gong, Zhao and Liu2007).
The samples from eight granite intrusions analysed for this study have yielded U–Pb ages ranging from 111±3 to 151±2 Ma, and display two peaks at 137±1 and 125±1 Ma (Table 4; Fig. 8). The 137±1 Ma peak can be interpreted as the initiation of the partial melting, whereas the second peak might represent the climax of the magma generation in the Dabie orogen. The Shigujian granite (141±2.3 Ma) is the oldest intrusive rock, which is a syn-tectonic intrusion associated with the NE-trending shear zone (Deng, Wu & Yang, Reference Deng, Wu and Yang2013). The granite is strongly deformed and has a high-K adakitic geochemical characteristic (Fig. 4) and a positive Eu anomaly (Fig. 5b). The fractionated REE patterns (La/Sm)N (3.65–8.85) and (Gd/Yb)N (1.43–5.50) in this intrusion are consistent with garnet- and amphibolite-bearing residue in the source, whereas the positive Eu anomalies indicate that the plagioclase was consumed during melt extraction. This intrusion has probably resulted from the partial melting of over-thickened (>50 km) lower continental crust. The emplacement of the Shigujian granite resulted from SE-trending tensional stress. The emplacement suggests that partial melting commenced after 141 Ma, which also constrains the onset of extensional tectonism in this region to be after 141 Ma (Deng, Wu & Yang, Reference Deng, Wu and Yang2013). Field observations and geochemical studies on the Tiantangzhai, Jiuzihe and Erwan granites indicate that the partial melting event was coeval with the widespread post-collision magmatism. This event appears to have resulted from the gravitational collapse and delamination of the orogenic lithosphere beneath the Dabie orogen. The youngest Bodaofeng granite (132 Ma) does not have adakitic geochemical signatures, suggesting that the granite originated from shallower depths (c. 35 km) through partial melting of the lower continental crust. The rims of two zircon grains from the Erwan granite (DT22) have yielded ages between 111 and 117 Ma. The geological and petrographic characteristics of the Erwan granite are similar to those of granitic dykes in the NDC, which implies the termination of the gravitational collapse and delamination beneath the Dabie orogen. Voluminous igneous intrusions were emplaced in a short period of time (<15 Ma) after the tectonic regime changed from compression to extension, which corresponds to a large extensional tectonic event in eastern China (Xu, Ma & Ye, Reference Xu, Ma and Ye2007).
6.c. Magma emplacement mechanisms
Orogenic evolution generally comprises a period of crustal thickening followed by the extension and thinning of the previously thickened crust (Brown, Reference Brown2001; Vanderhaeghe & Teyssier, Reference Vanderhaeghe and Teyssie2001). Eastern China (e.g. the Liaodong Peninsula, Xingmeng Orogenic Belt, Yanshan–Liaoxi area, Jiaodong Peninsula and eastern Yangtze Craton) has long been recognized as having undergone lithospheric thinning in the Mesozoic through delamination (Zhai et al. Reference Zhai, Windley, Kusky and Meng2007). The formation of migmatites and granites in the Dabie orogen was related to the extension and thinning of thickened crust formed during the Triassic collision (Wu et al. Reference Wu, Zheng, Tang, Gong, Zhao and Liu2007). Detailed zircon U–Pb dating and petrologic experimental studies on the Dabie orogen HP–UHP metamorphic rocks suggest that the HP–UHP metamorphism occurred between 245 and 210 Ma. The UHP metamorphism appears to have taken place between 245 and 222 Ma, lasting c. 20 Ma, whereas the amphibolite-facies retrograde metamorphism and related magmatism occurred between 222 and 210 Ma (Hacker et al. Reference Hacker, Wallis, McWilliams and Gans2009). ‘Rapid cooling’ between 210 and 170 Ma followed (Hacker et al. Reference Hacker, Ratschbacher, Webb, Ireland, Walker and Dong1998; Liu et al. Reference Liu, Jahn, Liu, Dong and Li2004; Wang et al. Reference Wang, Wyman, Xu, Jian, Zhao, Li, Xu, Ma and He2007).
A quiet time period existed from the rapid cooling at 170 Ma to the numerous Cretaceous volcanic eruption events at 141 Ma. The voluminous granitic magmatism in the Dabie orogen may have occurred in response to the lithospheric delamination and foundering during the early Cretaceous (Huang et al. Reference Huang, Li, Dong, Li, Chen, Wang and Yang2007; Wang et al. Reference Wang, Wyman, Xu, Jian, Zhao, Li, Xu, Ma and He2007; Wu et al. Reference Wu, Zheng, Tang, Gong, Zhao and Liu2007; Zhao et al. Reference Zhao, Zheng, Wei and Wu2011).
The geochemical and isotopic characteristics of the Tiantangzhai Complex in the NDC suggest that different types of granites were derived from different crustal sources. The emplacement ages of the Cretaceous granites in the NDC display a negative correlation with the SiO2 contents (Fig. 10). The SiO2 content increases from about 64 to 74 wt% from the Shigujian granite to the Bodaofeng granite. The increase in SiO2 content indicates the source rocks changed from basic to acidic through time. The emplacement process of the granites shows the foundering of the thickened crust and the reduction of the magma chamber depth, which implies that the order of the intrusion is consistent with the delamination and collapse processes. The interpreted tectonic model is illustrated in Figure 11.

Figure 10. SiO2 versus 206Pb–238U age diagram of the Tiantangzhai intrusive complex from the NDC.

Figure 11. A simplified model for the structure of the Dabie orogen and the magmatic mechanism of the early Cretaceous granites. Modified from Wang et al. (Reference Wang, Wyman, Xu, Jian, Zhao, Li, Xu, Ma and He2007, Reference Wang, Xiang, Zhu and Jiang2011b ). (a) Partial melting of the eclogite of thickened crust and lithospheric mantle under compression from the southeast during 150–141 Ma, forming the Shigujian syn-tectonic granite. (b) Doming and partial melting of the lower crust under an extensional tectonic regime in response to hot asthenosphere upwelling, possibly resulting from delamination of the lower lithospheric mantle during 137–125 Ma, forming voluminous granitoid plutons. (c) Successive upwelling of hot asthenosphere leads to partial melting of the middle–lower crust under an extensional tectonic regime during 117–111 Ma, forming acidic dykes and basic dykes. NHY – North Huaiyang belt; NDC – North Dabie Complex; SDC – South Dabie Complex; LCC – lower continental crust.
Multi-stage early Cretaceous granitoids are closely associated with the evolution of the continental crust in the NDC. Figure 11a depicts the thickening of the NDC and eclogitization of its root. Wu et al. (Reference Wu, Zheng, Tang, Gong, Zhao and Liu2007) suggested that the transition of the tectonic regime from compression to extension occurred at c. 145 Ma. The Shigujian intrusion is a syn-tectonic, high Sr/Y granite (HSG). Anisotropy of magnetic susceptibility (AMS) data show that most magnetic foliations dip steeply to the SE; 85% of sampling points dip between 40° to 90°. The anisotropy degree (P) is between 1.065 and 1.532 and the shape parameter (T) is between 0.005 and 0.694. The Flinn diagram of the magnetic fabrics shows that the value of K is less than 1. The AMS of the Shigujian intrusion suggests that the pluton was emplaced and deformed under a SE–NW compressional stress regime. The tectonic regime of the Dabie orogen changed from compression to extension after 141 Ma (see Deng, Wu & Yang, Reference Deng, Wu and Yang2013 for detailed discussion). The HSG partial melting of the thickening lower continental crust implies that the HSGs were equilibrated with eclogitized residues. This model is also supported by previous studies (Xu, Ma & Zhang, Reference Xu, Ma and Zhang2012; Xu et al. Reference Xu, Ma, Zhang and Ye2013) on high Sr/Y granitoids in the Tiantangzhai area. Therefore, we suggest that the syn-tectonic granites are the product of the partial melting of the thickened lower continental crust with eclogitized residues under a contractional tectonic regime, and the granite ascended along the NNE-trending ductile shear zone. The thickened lower continental crust remained stable (>141 Ma) until the late Mesozoic anatexis and magmatism occurred widely across the orogen.
The intrusion of widespread voluminous granites may have been related to the transition to an extensional tectonic regime and the thinning of the thickened crust in the Dabie orogen. Figure 11b shows the foundering mechanism of the lower continental crust and the extensional collapse of the orogen. The tectonic regime changed to extension along with foundering of the over-thickened lithospheric root of the Dabie orogen as well as the subsequent upwelling of the hot asthenosphere. The input of heat from the hot asthenosphere into the lower continental crust further caused the partial melting of the crust. The partial melting of the thickened lower continental crust generated most of the Cretaceous granitoids (137 to 125 Ma) (Wang et al. Reference Wang, Wyman, Xu, Jian, Zhao, Li, Xu, Ma and He2007; Xu, Ma & Zhang, Reference Xu, Ma and Zhang2012; Xu et al. Reference Xu, Ma, Zhang and Ye2013). The types of granites range from low-Mg adakitic (Xu, Ma & Zhang, Reference Xu, Ma and Zhang2012; Xu et al. Reference Xu, Ma, Zhang and Ye2013; Deng, Wu & Yang, Reference Deng, Wu and Yang2013) to high-Mg adakitic granites (Wang et al. Reference Wang, Wyman, Xu, Jian, Zhao, Li, Xu, Ma and He2007; this study) to normal granites (Zhao et al. Reference Zhao, Zheng, Wei and Wu2004; Xu, Ma & Ye, Reference Xu, Ma and Ye2007) depending on the extent of delamination. Therefore, the thickened crust of the NDC had become normal or thin, following the large-scale granitic magmatism between 140 and 120 Ma.
The Dabie orogen became normal thickness crust (35 km) again following the large-scale delamination of the lithospheric mantle and the removal of the orogenic root. Further mantle upwelling as well as the foundering of eclogite restites could have caused the extensive magma emplacement (Fig. 11c). Wang et al. (Reference Wang, Wyman, Xu, Jian, Zhao, Li, Xu, Ma and He2007) reported a range of zircon U–Pb ages between 118 and 105 Ma for the late-tectonic granitic dykes. The analyses of two zircon grain rims from sample EW-1 have given ages between 117 and 111 Ma, which are comparable to the age of the granitic dykes in the NDC. This age range implies the continued upwelling of the hot asthenosphere and the delamination of the eclogitic lower crust; the middle and lower crust were also heated and began to melt to generate the late granitic magmas (Chen, Jahn & Wei, Reference Chen, Jahn and Wei2002; Bryant et al. Reference Bryant, Ayers, Gao, Miller and Zhang2004; Wang et al. Reference Wang, Wyman, Xu, Jian, Zhao, Li, Xu, Ma and He2007). The emplacement of the granites and mafic-ultramafic rocks marks the end of the delamination beneath the Dabie orogen.
Four mechanisms have been proposed to account for the early Cretaceous magmatism in the Dabie orogen: (1) Triassic collision between the Yangtze and North China cratons (e.g. Gao, Qiu & Ling, Reference Gao, Qiu and Ling2002); (2) rapid mantle convection below the collision-thickened orogen (Wu et al. Reference Wu, Zheng, Tang, Gong, Zhao and Liu2007; Zhao et al. Reference Zhao, Chen, Zheng, Chen and Wu2007 b); (3) large-scale strike-slip motion along the Tanlu fault (Xu, Ma & Zhang, Reference Xu, Ma and Zhang2012; Xu et al. Reference Xu, Ma, Zhang and Ye2013); and (4) subduction of the Pacific plate beneath eastern China (Hacker et al. Reference Hacker, Ratschbacher, Webb, Ireland, Walker and Dong1998; Ratschbacher et al. Reference Ratschbacker, Hacker, Webb, McWilliams, Ireland, Dong, Calvert, Chateigner and Wenk2000; Wu et al. Reference Wu, Zheng, Tang, Gong, Zhao and Liu2007). We suggest that the partial melting of the lower continental crust was driven by a westward subduction of the Pacific plate beneath the Eurasian continent (Hacker et al. Reference Hacker, Ratschbacher, Webb, Ireland, Walker and Dong1998; Ratschbacher et al. Reference Ratschbacker, Hacker, Webb, McWilliams, Ireland, Dong, Calvert, Chateigner and Wenk2000; Wu et al. Reference Wu, Lin, Wilde, Zhang and Yang2005, Reference Wu, Zheng, Tang, Gong, Zhao and Liu2007). The direction of the Pacific plate's subduction beneath the Eurasian plate changed from northwest to west during the late Jurassic to the early Cretaceous transition (Zhao et al. Reference Zhao, Chen, Zheng, Chen and Wu2007 b). In summary, we propose that the change of the subduction angle and stress release triggered the delamination and foundering of the over-thickened continental crust of the Dabie orogen.
7. Conclusions
-
(1) The sequence of magmatic intrusions in the Tiantangzhai granitic suites in the Dabie orogen range from the oldest Shigujian through the Egongbao, Tiantangzhai, Jiuzihe and Erwan to the youngest Bodaofeng.
-
(2) The Shigujian granite (141±2.3 Ma) is a syn-tectonic intrusion originating from the partial melting of the thickened lower continental crust with eclogitized residues under a compressional tectonic regime. Magmas of the granite ascended along the NNE-trending ductile shear zone. The age (141 Ma) of the granite indicates the maximum time that the tectonic regime changed from contraction to extension.
-
(3) The early Cretaceous (151 to 111 Ma) granites have two zircon age peaks: 137±1 and 125±1 Ma. The 137 Ma peak indicates a partial melting event following the change in the tectonic regime from contraction to extension, whereas the 125 Ma peak represents the climax of the magma formation event in the Dabie orogen. The non-adakite granites and the mafic-ultramafic rocks (117 to 111 Ma) indicate the termination of the delamination processes beneath the Dabie orogen.
-
(4) The change in subduction angle of the Pacific plate beneath the Eurasian plate in eastern China might have triggered the delamination and foundering of the over-thickened continental crust of the Dabie orogen.
Acknowledgements
We appreciate the assistance of Yongsheng Liu and Zhaochu Hu with LA-ICP-MS zircon U–Pb dating and trace element analysis, and Dunyi Liu of the Beijing SHRIMP centre for SHRIMP dating. We also gratefully thank Mengning Dai of the Northwest University for their assistance with zircon Hf isotope analysis. This study was supported by funds from the Natural Science Foundation of China (40972137, 41172189, 91014002).