Introduction
How the environment shapes the ecology of individuals and populations is one of the current key issues of organismal biology (Heatwole Reference Heatwole2009, Schwenk et al. Reference Schwenk, Padilla, Bakken and Full2009). For ectotherms, such as lizards, temperature is particularly important, and their main heat sources are solar radiation, long-wave radiation, conduction with the substrate and convection with the air (Angilletta Reference Angilletta2009, Huey Reference Huey1991, Porter & Gates Reference Porter and Gates1969). The spatial configuration of the thermal landscape deeply affects the performance of lizards (Porter et al. Reference Porter, Mitchell, Beckman and DeWitt1973, Sears & Angilletta Reference Sears and Angilletta2015, Sears et al. Reference Sears, Angilletta, Schuler, Borchert, Dilliplane, Stegman, Rusch and Mitchell2016). Hence many lizards base microhabitat choices on thermal properties (Hertz et al. Reference Hertz, Fleishman and Armsbury1994, Smith & Ballinger Reference Smith and Ballinger2001).
Spatial structure of the thermal habitat affects microhabitat selection of lizards through the distribution of solar radiation (Newbold & MacMahon Reference Newbold and MacMahon2014) and different substrates (Ortega & Pérez-Mellado Reference Ortega and Pérez-Mellado2017, Quirt et al. Reference Quirt, Blouin-Demers, Howes and Lougheed2006). Temporal configuration of the thermal habitat reflects how daily (Castilla & Bauwens Reference Castilla and Bauwens1991, Díaz & Cabezas-Díaz Reference Díaz and Cabezas-Díaz2004), seasonal (Ortega & Pérez-Mellado Reference Ortega and Pérez-Mellado2016) or climatic changes (Doody et al. Reference Doody, Guarino, Georges, Corey, Murray and Ewert2006) modulate their microhabitat selection. Finally, non-thermal traits also influence lizard microhabitat selection, such as social interactions (Allen et al. Reference Allen, Rodríguez, Eifler and Powell2015, Martín & Salvador Reference Martín and Salvador1997, Paterson & Blouin-Demers Reference Paterson and Blouin-Demers2018), morphological adaptations (Jaksić et al. Reference Jaksić, Núnez and Ojeda1980), diet (Blouin-Demers & Weatherhead Reference Blouin-Demers and Weatherhead2001) or protection against predators (Allen et al. Reference Allen, Rodríguez, Eifler and Powell2015, Marshall et al. Reference Marshall, Philpot and Stevens2016). Such is the complexity that approximately 50 variables were identified as forming the niches of lizards (Pianka et al. Reference Pianka, Vitt, Pelegrin, Fitzgerald and Winemiller2017).
Despite the excellent research background about the thermal ecology of desert and tropical lizards (Pianka et al. Reference Pianka, Vitt, Pelegrin, Fitzgerald and Winemiller2017), most research on microhabitat choice is still centred on temperate species (Grbac & Bauwens Reference Grbac and Bauwens2001, Ortega & Pérez-Mellado Reference Ortega and Pérez-Mellado2016, Quirt et al. Reference Quirt, Blouin-Demers, Howes and Lougheed2006). Thus, there is a gap of knowledge in how abiotic traits drive microhabitat selection of tropical lizards (Hertz et al. Reference Hertz, Fleishman and Armsbury1994, Ruibal Reference Ruibal1961). Thermoregulation in tropical environments entails different patterns of costs and benefits, since habitat thermal quality is normally high (Vickers et al. Reference Vickers, Manicom and Schwarzkopf2011). Researchers have drawn attention to the high vulnerability of tropical lizards to climate change (Brusch et al. Reference Brusch, Taylor and Whitfield2016, Huey et al. Reference Huey, Deutsch, Tewksbury, Vitt, Hertz, Pérez and Garland2009, Pontes-da-Silva et al. Reference Pontes-da-Silva, Magnusson, Sinervo, Caetano, Miles, Colli, Diele-Viegas, Fenker, Santos and Werneck2018, Tewksbury et al. Reference Tewksbury, Huey and Deutsch2008), which urges us to understand how the different environmental factors drive their microhabitat selection.
We aim to disentangle the importance of different aspects of the environment in the microhabitat selection of Neotropical lizards, splitting the effect of heat sources and non-thermal properties of the substrate. We compare two lizard species of different families living in different habitats of central-western Brazil. We expect that lizards would select microhabitats based on their temperatures, prioritizing avoidance of overheating (Vickers et al. Reference Vickers, Manicom and Schwarzkopf2011). We aim to test two sets of hypotheses: (1) about the effects of solar radiation, air temperature and the physical thermal properties of the type of substrate (e.g. conductance, reflectance) on the substrate temperature, and (2) about the effects of solar radiation, air temperature, substrate temperature and the non-thermal properties of substrates in microhabitat selection of lizards. We propose a novel approach that incorporates common statistical tools of habitat selection (i.e. resource selection function, Manly et al. Reference Manly, McDonald, Thomas, McDonald and Erickson2002) into structural equation modelling. We believe our approach should allow disentangling and weighting the importance of different thermal and non-thermal covariates in use of space by lizards.
Species and site studied
Ameivula ocellifera (Spix 1825) is a widespread species complex (Arias et al. Reference Arias, Carvalho, Rodrigues and Zaher2011a) that ranges from north-eastern Brazil to Argentina (Arias et al. Reference Arias, Carvalho, Rodrigues and Zaher2011b). This small teiid (< 100 mm SVL) lives in sandy and hot open habitats (Menezes et al. Reference Menezes, Sluys Van, Fontes and Rocha2011) throughout the South American dry diagonal, which includes caatinga (a xeric semi-arid ecosystem of north-west Brazil), savanna, chaco (a semi-arid lowland ecosystem of central South-America) and coastal sand plain shrub ecosystems. The studied population belongs to a new species (Ameivula sp. 2 of Arias et al. Reference Arias, Recoder, Álvarez, Ethcepare, Quipildor, Lobo and Rodrigues2018) and is called here Ameivula aff. ocellifera. We observed A. aff. ocellifera individuals in an area of the Nhecolândia subregion of Pantanal wetland, at the Nhumirim Ranch, Brazil (18°59′S, 56°39′W). Vegetation is characterized by savanna forest interspersed with grasslands and brackish and freshwater ponds.
Tropidurus oreadicus (Rodrigues 1987) is a small tropidurid (< 100 mm SVL) commonly found in savanna in Brazil, although it also occurs in the southern part of the Amazonian forest (Rocha & Siqueira Reference Rocha and Siqueira2008). This species can be found in several habitat types, but it mainly occurs in rocky sites (Ribeiro et al. Reference Ribeiro, Gomides, Santos and Sousa2008). We observed Tropidurus oreadicus individuals at a savanna located at Experimental Ranch of Universidade Estadual do Mato Grosso do Sul, Brazil (20°26.164’S, 55°39.357’W). Vegetation is composed of savanna forest and some rock outcrops under clearances in the canopy. Both study areas have tropical climate (mean annual temperature of 25ºC) and marked seasonality of rainfall (Bergier & Assine Reference Bergier and Assine2016, Kottek et al. Reference Kottek, Grieser, Beck, Rudolf and Rubel2006).
Methods
We directly observed 43 A. aff. ocellifera and 23 T. oreadicus adult lizards between September and December 2017. For each lizard, we measured four microhabitat variables at the point where it was observed (used microhabitat) and four to eight points (unused microhabitats) at 5 m and 10 m apart (on each main cardinal direction) of the respective used microhabitat. Unused points provide a sample of the microhabitats that are available for each lizard at the exact time and in the surrounding area of the observations, therefore representing true absences of the respective individual (i.e. unselected microhabitats) (Duchesne et al. Reference Duchesne, Fortin and Courbin2010). We sampled lizards on sunny days, although the fact that each used microhabitat is paired with its respective simultaneously unused microhabitats precludes any confounding effect of atmospheric conditions in the results. The comparison between the used and unused microhabitats allows us to understand the importance of different environmental variables for lizard microhabitat selection. For each point (used and unused), we recorded (1) substrate temperature (Ts); (2) air temperature (Ta); (3) type of substrate (rock, dry grass, grass, leaf litter or sand); and (4) exposure to the sun (full sun, filtered sun, shade or cloudy). Ts was measured with an infrared laser thermometer (Benetech® GS320, precision 0.1°C) and Ta was measured at 2 cm above the ground, with a digital thermometer (6802II, precision 0.1°C). Correlation between both thermometers was high (r = 0.970). The study was purely observational regarding animals: no lizard was manipulated or harmed. All procedures are in compliance with the UFMS bioethics committee.
We addressed the study of each species by resource selection function (RSF), which is a function describing the probability of an individual using a certain resource (here, the microhabitats) based on its availability (Manly et al. Reference Manly, McDonald, Thomas, McDonald and Erickson2002). Many analyses can be used to solve a RSF, but it is recommended that data on use and availability of the resource are paired for each individual, in order for the absences of the unused (availability) points to be true absences (Duchesne et al. Reference Duchesne, Fortin and Courbin2010). Previous studies have used paired logistic regression (PLR) or conditional logistic regression (CLR) to solve RSFs related to microhabitat selection by vertebrates (Compton et al. Reference Compton, Rhymer and McCollough2002, Duchesne et al. Reference Duchesne, Fortin and Courbin2010, Ortega & Pérez-Mellado Reference Ortega and Pérez-Mellado2017).
Here we aim to integrate the candidate hypotheses that can disentangle the effect of the environment in microhabitat selection by lizards (Figure 1). To do this, we apply a mixed structural resource selection function (mixed-SRSF) approach, that is, solving the RSFs by means of mixed structural equation modelling (SEM) (Shipley Reference Shipley2016). As said, our data consist of direct observations of the environmental variables in a binomial process of microhabitat used by lizards (scored as 1) and values of these environmental variables at several paired unused points (scored as 0). Thus, including the individual identity as a random factor in SEM we can assess each hypothesis in the same probabilistic selection process. That is, we consider the availability of the environmental conditions for each lizard at the exact moment of sampling, bringing a more mechanistic approach of microhabitat selection.
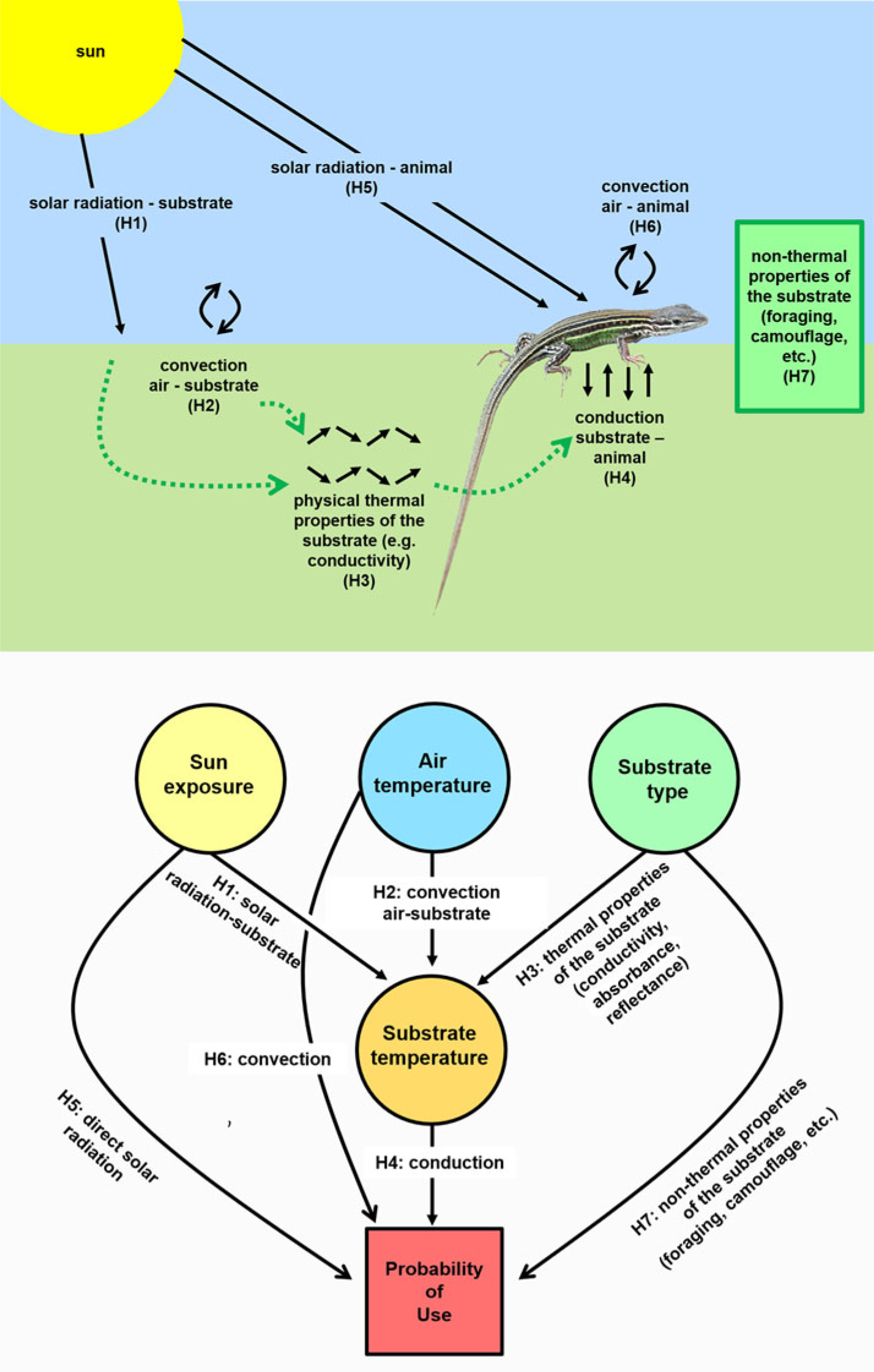
Figure 1. Studied relationships between lizard species and their environments in relation to microhabitat selection: a diagram depicting these relationships in nature (top) and the corresponding structural model used for analysis (bottom). The hypotheses are: (H1) there is a positive direct relationship between solar radiation and substrate temperature (Ts); (H2) there is a positive direct relationship between the air temperature (Ta) and Ts; (H3) there is an effect of the physical properties of the substrates on their temperatures (H1–H3 are the control hypotheses about the effects of the different heat sources and the thermal properties of the substrates on the substrate temperature); (H4) the Ts influences the probability of use of a certain microhabitat; (H5) solar radiation influences the probability of use of a certain microhabitat; (H6) Ta influences the probability of use of a certain microhabitat; (H7) the non-thermal properties of the substrates (e.g. food availability, predation pressure, potential for camouflage, suitability for lizard locomotion) influence the probability of use of a certain microhabitat (H4–H7 are the hypotheses of interest on how the studied environmental attributes shape the microhabitat selection of lizards).
The advantage of the mixed-SRSF over previous approaches is that it allows disentangling the effects of the different components of the environment in the selection process. In our case, we firstly isolate the thermal properties of the substrates in order to control for their effects on microhabitat selection by lizards (Figure 1). To do this, we established three control hypotheses about the thermal properties of substrates: (H1) exposure to the sun will directly affect substrate temperature (Ts), with lower temperatures in the shade, milder in filtered sun and higher under full sun; (H2) air temperature (Ta) will positively affect the Ts; and (H3) different types of substrates, due to their physical thermal properties (e.g. conductance, reflectance) will show different Ts (Figure 1). We know that these thermal relationships are well studied by biophysical mechanistic models (Kearney et al. Reference Kearney, Isaac and Porter2014a, Reference Kearney, Shamakhy, Tingley, Karoly, Hoffmann, Briggs and Porter2014b; Porter & Gates Reference Porter and Gates1969, Porter et al. Reference Porter, Mitchell, Beckman and DeWitt1973). Nonetheless, it is important to control for these effects within our own empirical data to better understand how these variables affect the microhabitat selection of lizards.
Then we advance by testing the effect of Ts (H4), direct solar radiation (H5) and air convection (H6) on microhabitat selection of lizards (Figure 1). The direction of the predicted effect of the H4 and H5 will depend on the environmental temperatures; if they are below their thermal preferences, lizards will select microhabitats with higher solar exposure and/or warmer conditions, and vice versa. Finally, we tested the effect of substrate type (H7) on microhabitat selection, which will be related to non-thermal substrate properties (that can be associated to camouflage, prey availability, access to shelter or escape paths, or other ecologically relevant traits). It is worth noting that covariates such as exposure to the sun, air temperature and substrate type can drive microhabitat selection of lizards via direct effects (H5, H6 and H7) and indirect effects, by changes in Ts (H1, H2 and H3) that, in turn, can cause changes in microhabitat selection (H1, H2, and H3 via H4) (Figure 1).
In addition, direct, indirect and total effects can also be assessed, that is: the direct effect of Ta on the probability of use of the microhabitat is the coefficient estimated in H6, the indirect effect is given by the product between H2 and H4; therefore the total effect of Ta on microhabitat selection follows H6 + H2*H4 (Figure 1) (Shipley Reference Shipley2016). The SEM was fitted in R routine (version 3.2.3) using the piecewiseSEM package (Holst & Budtz-Jørgensen Reference Holst and Budtz-Jørgensen2013). Goodness of fit for mixed-SRSF measures the discrepancy between predicted and observed covariance matrices in structural equation modelling, combining P-values of the conditional independence tests in the C of Fischer statistic (Holst & Budtz-Jørgensen Reference Holst and Budtz-Jørgensen2013).
Results
Environmental temperatures were quite similar at the used and unused points for both species (Table 1); detailed descriptive statistics are provided in Appendices 1–3. We first assessed the control hypotheses on how solar radiation, air convection and the physical thermal properties of the substrates drive Ts (H1–H3). The mixed-SRSF revealed that Ts was simultaneously affected by solar radiation on the substrate (H1) (increasing in sunny microhabitats to approximately double the decreases in shade), air convection with the substrate (H2) (positive effect in Ts, resulting the main driver of Ts in both studied areas, but more important for T. oreadicus) and the physical thermal properties of the substrate type (H3) (where the leaf litter was hotter and the rock was colder than other substrates for A. aff. ocellifera and T. oreadicus, respectively) (Figures 2 and 3).
Table 1. Air (Ta) and substrate (Ts) temperatures measured at the microhabitats used by lizards of two species (Ameivula aff. ocellifera and Tropidurus oreadicus) in Brazil and their paired available (unused) microhabitats. All temperatures are reported in °C


Figure 2. Path analysis model predicting the probability of presence of Ameivula aff. ocellifera lizards of the Pantanal of Nhêcolandia (Brazil) depending on the studied environmental variables. The solid arrows represent significant effects on the probability of use (that is, selection of a certain microhabitat). The dashed arrow represents a not significant result. The standardized path coefficients (β) represent the relative strength of the corresponding effect. AIC = 2002.3, log-likelihood of model = −985.15, Chi-squared (comparing the observed covariances with those predicted by the model) = 0.016, P = 0.898.

Figure 3. Path analysis model predicting the probability of presence of Tropidurus oreadicus lizards of Aquidauana (Brazil) depending on the studied environmental variables. The solid arrows represent significant effects in the probability of use (that is, selection of a certain microhabitat). The dashed arrow represents a not significant result. The standardized path coefficients (β) represent the relative strength of the corresponding effect. AIC = 502.75, log-likelihood of model = −235.37, Chi-squared (comparing the observed covariances with those predicted by the model) = 2.087, P = 0.148.
Then, we focus on the effects of conduction with the substrate (H4), direct solar radiation on the animal (H5), air-convection with the animal (H6) and the non-thermal properties of the substrate type (H7) on microhabitat selection (Figures 2 and 3). Conduction with the substrate (H4) and convection of air around the animal (H6) were not significant in microhabitat selection by either species. This means that a gradient of substrate or air temperature (within the range studied here) over the same substrate, as well as exposure to solar radiation, failed to exert any change in the use of space of the two lizard species. Direct solar radiation (H5) affected microhabitat selection of both species: A. aff. ocellifera avoided full sun and T. oreadicus avoided both the full sun and the shade (with the same strength). The non-thermal properties of the substrate type (H7) also affected the probability of use by both species: A. aff. ocellifera selected sand over dry grass, grass and leaf litter (Figure 2), while T. oreadicus selected rock and log over soil and leaf litter (Figure 3). The comparison among standardized coefficients estimated in mixed-SRSF suggested both solar radiation and non-thermal properties of substrate had similar importance in microhabitat selection for the two species, with a slightly larger relevance of non-thermal properties of substrate type (β substrate-sand = 0.25 versus β sun exposure-sun = −0.22 and β substrate-rock = 0.32 versus β sun exposure-shade = −0.23). Mixed-SRSF presented adequate adjustment for both species (A. aff. ocellifera: Fisher’s C = 0.31, df = 2; P = 0.86 for A. aff. ocellifera and Fisher’s C = 3.98, df = 2; P = 0.13 for T. oreadicus).
Discussion
The mixed-SRSF approach allowed us to disentangle the thermal and non-thermal effects of the environment on lizard microhabitat selection. Among the thermal mechanisms underlying microhabitat selection, we were able to split the effects of conduction with the substrate, direct solar radiation and convection with the surrounding air. We were also able to quantify how solar radiation and air convection on substrate, as well as the physical thermal properties of the type of substrate, regulate the conduction between the animal and the substrate. Besides this, we were able to separate the effect of the non-thermal properties of the substrate from the other attributes. The approach also allowed us to compare the intensity of both the direct and indirect effects on microhabitat selection. Although we were able to disentangle the thermal effects of the microhabitat, the mechanisms underlying the non-thermal properties of the substrate remain hidden in our sampling and model design. We propose to include other variables in future studies, aiming to understand how animals interact with the non-thermal component of the environment (such as food resources, cryptic properties of the substrates, predation pressure or availability of shelter). While adding more explicative variables, the remaining unexplained variability attributed to the effect of type of substrate on the probability of microhabitat selection (analogous to our H7) (Figure 1) will be split, allowing us to quantify the part of variability due to the type of substrate that we could not explain in this study. The flexibility of our approach to add more direct and indirect candidate drivers of microhabitat selection provides an advance on the mechanistic understanding of animal movement.
Solar radiation, rather than convection or conduction, was the main thermal driver of microhabitat selection of lizards. Ameivula aff. ocellifera avoided the sun, and selected filtered sun or shaded areas, while T. oreadicus avoided both sunny and shaded areas (with the same strength), only selecting microhabitats with filtered sun. Solar energy increases towards the equator, and it can be the most important heat source – and also potential stressor – for tropical animals (Da Silva et al. Reference Da Silva, Maia and Macedo-Costa2014). Direct convection with air and conduction from the substrate were not modulating the microhabitat selection of the two studied Neotropical lizards. The preferred temperatures of the same population of T. oreadicus (unpubl. data) go from 32.9°C to 36.4°C, and the interquartile range of the substrate and air temperatures of the microhabitats (used or available) was ~30.5–35.5°C. Preferred temperatures of A. aff. ocellifera are unknown, but their active body temperatures usually range between 37°C and 40°C (Menezes et al. Reference Menezes, Sluys Van, Fontes and Rocha2011, Mesquita & Colli Reference Mesquita and Colli2003). Thus, it seems that the good thermal quality of the habitat would make it unnecessary for lizards to prioritize conduction from the substrate on their microhabitat decisions, as could be the case of temperate lizards (Grbac & Bauwens Reference Grbac and Bauwens2001, Ortega & Pérez-Mellado Reference Ortega and Pérez-Mellado2016, Reference Ortega and Pérez-Mellado2017; Quirt et al. Reference Quirt, Blouin-Demers, Howes and Lougheed2006). This result is in contrast with what we know about temperate lizards, for whom environmental temperatures – and mainly the temperature of the substrate – play an important role in microhabitat selection. Convection, however, could be important in windy conditions (Maia-Carneiro et al. Reference Maia-Carneiro, Dorigo and Rocha2012, Ortega et al. Reference Ortega, Mencía and Pérez-Mellado2017). As solar radiation is stronger in the tropics, our results suggest that lizards could be prioritizing avoidance of overheating, as suggested for thermoregulation of tropical lizards (Vickers et al. Reference Vickers, Manicom and Schwarzkopf2011). While the temperatures offered by the different microhabitats are not excessively high for any species, direct solar radiation can rapidly increase the body temperature of lizards (Belliure & Carrascal Reference Belliure and Carrascal2002, Carrascal et al. Reference Carrascal, López, Martín and Salvador1992), which could probably entail risk of overheating for both species (Kearney et al. Reference Kearney, Shine and Porter2009, Tewksbury et al. Reference Tewksbury, Huey and Deutsch2008). Thus, the availability of enough vegetation to provide microhabitats with filtered sun seems important for the conservation of these Neotropical lizards. Our approach allows comparing the relative importance of the different heat sources on microhabitat selection of lizards from different habitats (e.g. deserts, rock outcrops, deciduous forests and rain forests), different times of activity (diurnal vs nocturnal) and different climates (temperate vs tropical areas). Such comparisons will provide us with a good mechanistic understanding of how lizards exploit the different heat sources.
The non-thermal properties of the substrates were the other main component mediating microhabitat selection by the studied lizard species. These properties can be related to locomotion, availability of shelter, food and/or nest sites, or to the presence of competitors, parasites and/or predators (Pianka et al. Reference Pianka, Vitt, Pelegrin, Fitzgerald and Winemiller2017). Although we were not able to split the effect of these non-thermal variables of the substrates, we were able to quantify their relative importance compared with the thermal properties of the microhabitats. Hence, we showed that A. aff. ocellifera acted indifferently to grass (dry or fresh) and leaf litter, and clearly selected sand, while T. oreadicus reacted randomly to soil and leaf litter, selecting logs and rocks instead. Furthermore, we can state that the selection of these habitats by each species is due to non-thermal properties (Figures 2 and 3). The selection of sandy substrates (based on their non-thermal traits) is somehow more important for A. aff. ocellifera than is the avoidance of direct solar radiation (standardized coefficients: 0.25 vs 0.22, respectively, Figure 2). For this species, we believe that availability of food resources and avoidance of intraspecific competition are the main traits that make sand attractive to lizards (Mesquita & Colli Reference Mesquita and Colli2003). For T. oreadicus, the selection of rocky substrates (logs were only observed twice) seems substantially more important than the avoidance of sunny and shaded areas (0.32 vs ≈ 0.22, respectively). This tropidurid mainly relies on sit-and-wait hunting, which is associated with the necessity to be cryptic with its surroundings (Pianka et al. Reference Pianka, Vitt, Pelegrin, Fitzgerald and Winemiller2017). The fact that rocky substrates probably provide food, camouflage and shelter to T. oreadicus lizards could explain why this substrate is attractive to them, once we know that it is not because of its thermal properties. A recent study found that Aspidoscelis sexlineata lizards select the substrate that facilitates the best locomotor performance (Sathe & Husak Reference Sathe and Husak2018). Following the proposed niche ordination (Pianka et al. Reference Pianka, Vitt, Pelegrin, Fitzgerald and Winemiller2017), we believe that including food, shelter, structural traits of substrates affecting lizard locomotion, camouflage and availability of nest sites to our approach promote an understanding of the contributions of different mechanisms to lizard microhabitat selection.
In temperate areas, lizard assemblages usually contain fewer species than in the tropics (Hillebrand Reference Hillebrand2004, Huey et al. Reference Huey, Deutsch, Tewksbury, Vitt, Hertz, Pérez and Garland2009). In addition, spatial thermal heterogeneity is high, which makes shuttling between microhabitats at different temperatures an important thermoregulation mechanism (Grbac & Bauwens Reference Grbac and Bauwens2001, Ortega & Pérez-Mellado Reference Ortega and Pérez-Mellado2016, Quirt et al. Reference Quirt, Blouin-Demers, Howes and Lougheed2006). However, tropical lizard communities are usually formed of many species that share generally more thermally homogeneous habitats (Addo-Bediako et al. Reference Addo-Bediako, Chown and Gaston2000, Janzen Reference Janzen1967). Thus, tropical lizards tend to be microhabitat specialists (Brusch et al. Reference Brusch, Taylor and Whitfield2016), which is totally in tune with our findings. The fact that Neotropical lizards are more specialized to a type of substrate or microhabitat makes them more vulnerable to modification of their habitats, such as changes in land use, fragmentation or climate change (Brusch et al. Reference Brusch, Taylor and Whitfield2016, Huey et al. Reference Huey, Deutsch, Tewksbury, Vitt, Hertz, Pérez and Garland2009). Our results highlight the importance of understanding the role of the different environmental variables in the habitat selection of tropical lizards, which is probably different than in the temperate areas, and the importance of doing it soon, since many tropical populations, as those studied here, are probably highly vulnerable in a future of climate change.
In short, we disentangled which components of the environment influence microhabitat selection of two Neotropical lizard species. Substrate temperature was mainly determined by solar radiation and air temperature, while the intrinsic physical properties of the substrate types showed a lesser contribution. Surprisingly, the substrate and air temperatures did not influence the microhabitat decision of lizards. On the contrary, direct solar radiation and the non-thermal properties of the substrates were the main drivers of microhabitat selection in both species. Results were highly consistent for both species and reinforce the idea of tropical lizards being microhabitat specialists, from which the selection of environmental temperatures (air and substrate) could be less important than exposure to the sun or other non-thermal features of their habitats.
Author ORCID
Zaida Ortega https://orcid.org/0000-0002-8167-1652
Acknowledgements
The staff of the Fazenda Nhumirim, Embrapa-Pantanal, Walfrido Thomas and Fernando Paiva helped with logistics, and Taynara Leão, Liliana Piatti and Evander Sanches helped with data collection on the Pantanal. The staff of the Universidade Estadual of Mato Grosso do Sul (UEMS), and particularly Afranio J.S. Soares, helped with logistics on Aquidauana. Arlindo F. Béda kindly helped us to find the populations of T. oreadicus. Harold Heatwole helped with careful revision of the text.
Financial support
This work was supported by ‘Fundação de apoio ao desenvolvimento do ensino, ciência e tecnologia’ of the Mato Grosso do Sul state [Fundect #0247/2014], Coordenação de Aperfeiçoamento de Pessoal de Nível Superior (CAPES), the Programa de Pós-graduação em Ecologia e Conservação (PPGEC) and the Programa de Pós-graduação em Biologia Animal (PPGBA). Postdoctoral fellowships of Programa Nacional de pós-doutorado (PNPD/CAPES) supported Z.O. and A.M. A doctoral fellowship from CAPES supported P.S. and a MSc fellowship from Fundect supported K.M.
Appendix 1
Mean (± SE, N) substrate temperatures (Ts) and air temperatures (Ta) of the substrates on which Ameivula aff. ocellifera were observed (used) and those available in the studied habitat at the Pantanal of Nhecolândia (Brazil). Temperatures are in °C.

Appendix 2
Mean (± SE, N) substrate temperatures (Ts) and air temperatures (Ta) of the substrates on which Tropidurus oreadicus were observed (used) and those available in the studied habitat at Aquidauana (Brazil). Temperatures are in °C.

Appendix 3
Mean (± SE, N) substrate temperatures (Ts) and air temperatures (Ta) of the different conditions of exposure to light (full sun, filtered sun, shade and cloudy) at which Ameivula aff. ocellifera and Tropidurus oreadicus lizards were observed (used) and those of available microhabitats at both studied areas at Mato Grosso do Sul (Brazil). Temperatures are in °C.
