Introduction
In all vertebrates, oogenesis is a complex process characterized by a series of cellular and molecular events that take place in the nucleus and cytoplasm of the developing oocytes (Monroy et al., Reference Monroy, Parisi and Rosati1983; Sanchez & Villecco 2003). In the ovary, the oocyte and the somatic tissue (follicle cells and theca) form a morphological entity called the ovarian follicle that undergoes structural and functional differentiation (Bachvarova et al., Reference Bachvarova, Manova, Packer, Huang, Besmer, Hsueh and Schomberg1992).
Extensive evidence, both direct and indirect, demonstrates that somatic follicle cells execute multiple and variable functions throughout life and at different stages of gamete and follicle development. Follicle cells have been implicated in a number of gonadotrophin-regulated processes involved in oocyte growth and maturation. These include steroidogenesis (Redshaw, Reference Redshaw1972; Fortune, Reference Fortune1983; Sretarugsa & Wallace, Reference Sretarugsa and Wallace1997; Hernandez et al., Reference Hernandez and Bahr2003; Schoenfelder et al., Reference Schoenfelder, Schams and Einspanier2003), initiation of yolk protein (vitellogenin) uptake (Dumont, Reference Dumont1978), meiotic arrest (Villecco et al., Reference Villecco, Aybar, Sanchez and Sanchez Riera1996) and other processes such as vitelline envelope formation (Dumont and Brummett, Reference Dumont and Brummet1978; Cabada et al., Reference Cabada, Sánchez Riera, Genta, Genta, Sánchez and Barisone1996), RNA transfer (Sanchez Riera et al., 1988; Motta et al., Reference Motta, Filosa and Andreuccetti1995) and organelle transfer (Andreuccetti, 1992; Motta et al., 1995).
In many vertebrates, during the different stages of oogenesis, the follicular is arranged as a single layer of homogeneous cells (Callebaut & Van Nassauw, Reference Callebaut and Van1987). In contrast, the follicular epithelium of previtellogenic oocytes from lizards and snakes is typically polymorphic and multilayered, being characterized by the presence of three distinct cells: small, intermediate and pyriform cells (Filosa, Reference Filosa1973; Andreuccetti et al., Reference Andreuccetti, Limatola and Ghiara1979). The heterogeneity of the granulosa cell population disappears from the vitellogenic oocytes by regression of intermediate and pyriform cells via programmed cell death (Motta et al., Reference Motta, Filosa and Andreuccetti1996, Reference Motta, Tammaro, Cicale, Indolfi, Iodice, Spagnuolo and Filosa2001).
In both anuran and urodele amphibians studied up to now, the follicles cells form a monolayer of homogeneous structure around each developing oocyte that remains unchanged throughout oocyte growth (Kemp, Reference Kemp1956, Wartenberg, Reference Wartenberg and Gusek1960; Hope, Reference Hope, Humphries and Bourne1963, Dumont, Reference Dumont1972; Villecco et al., 1998, Reference Villecco, Aybar, Genta, Sánchez and Sánchez Riera2000).
In the present work we investigate the follicular epithelium of Ceratophrys cranwelli oocytes, the Argentine horned frog. This species was selected because it is capable of effecting rapid oogenesis when it is stimulated by strong transitory rainfall, a factor that seems to be responsible for the activation of the reproductive process (Tinsley et al., Reference Tinsley, Loumont, Kobel, Tinsley and Kobel1996). Moreover, C. cranwelli has a pattern of early morphogenesis significantly different from that of the best-studied amphibian, Xenopus laevis (Purcell & Keller, Reference Purcell and Keller1993).
Materials and Methods
Animals
Ceratophrys cranwelli ovarian follicles from specimens recently collected in the neighborhood of Tucumán (Argentina) during the spring–summer period were used in this study.
Tissue preparation for microscopy
Sexually mature females were anesthetized. After laparoscopy, pieces of ovary were carefully dissected to obtain isolated oocytes at different stages (Villecco, Reference Villecco1998), wrapped in their follicle. Isolated follicles were measured with an eyepiece micrometer in a stereoscopic microscope.
Transmission electron microscopy
For thin sectioning, previtellogenic whole follicles were fixed for 4 h at 4 °C in 25% glutaraldehyde in 0.1% M sodium phosphate (pH 7.4). Follicles were washed twice in phosphate buffer and postfixed in 1% osmium tetroxide in the same buffer at 4 °C overnight. Samples were dehydrated in an ethanol series and embedded in Spurr resin. Sections were prepared with a Potter Blum MTI ultramicrotome, stained with lead citrate and uranyl acetate and examined with a Zeiss EM109 electron microscope.
Radioactive labelling
Pieces of ovary from six animals, containing 10 to 15 previtellogenic follicles each, were incubated in Ringer's solution with antibiotics (25 μm/ml penicillin and 50 μm/ml streptomycin) containing 100 μCi/ml of [3H]-adenosine (specific activity 36.7 Ci/mM; New England Nuclear) in a total volume of 500 μl. The follicles were incubated for 5 h in a moist chamber at 20–25 °C.
For pulse–chase experiments, the follicles were rinsed after 5 h and transferred to 1 mM adenosine solution. The chase periods were 4 h, 9 h and 24 h.
After incubation, follicles were fixed in Ancel & Vintemberger solution (Ancel & Vintemberger, Reference Ancel and Vitemberger1948), dehydrated and embedded in paraffin–celloidin, according to Manes and Nieto (Reference Manes and Nieto1983). Serial sections, 4–6 μm thick, were attached to slides.
Autoradiography
Autoradiography was carried out with Ilford K2 liquid emulsion diluted 1:1 with distilled water. Exposure was carried out for 15 days at 4 °C. Exposed slides were developed with a Kodak D19 developer, washed and fixed with sodium thiosulfate. Dried slides were stained with hematoxylin–eosin and mounted for light microscopic observation. Blanks of non-radioactive slides were also run.
Ultrastructural localization of RNA
Tissue preparation
Previtellogenic follicles oocytes were fixed by immersion in 0.1 M phosphate-buffered 1% glutaraldehyde solution for 2 h at room temperature. After fixation, the follicles were washed in the phosphate buffer, dehydrated in graded ethanol and embedded in L.R. White resin according to the usual procedure. Thin sections were mounted on nickel grids and processed by cytochemical labelling.
Preparation of the RNase–gold complexes
The colloidal gold suspension was prepared according to the method of Frens (Reference Frens1973). Four millilitres of 1% aqueous solution of sodium citrate were added to a boiling aqueous solution of 100 ml of 0.01% tetrachloroauric acid and allowed to boil for 5 min. By this procedure, we obtained a monodispersed colloidal sol, which was composed of gold particles with an average size of 144 in diameter as determined by TEM. It was adjusted to pH 8.5 with 0.2 M K2CO3.
To determine the minimal amount of enzyme necessary for full stabilization of the colloidal gold, a constant volume of colloidal gold (5 ml) was mixed with 0.1 ml of serial dilutions of RNase. After 5 min 0.5 ml of 10% NaCl solution was added and flocculation was judged visually. When flocculation occurs, the red colour of the enzyme–gold complex turns into violet. The minimal amount was found to be 0.005 mg/ml.
For the preparation of the RNase–gold complex, 0.1 to 0.5 mg of RNase dissolved in 0.1 ml H2O was placed in a siliconized tube and 10 ml of the gold suspension (pH 8.5) was added. The mixtures were centrifuged at 25 000 rpm for 30 min at 4 °C. The RNase–gold complex, a dark red sediment, was carefully recovered and resuspended in 3 ml of phosphate-buffered saline (PBS) containing 0.2 mg/ml polyethylene glycol. The pH of the PBS solution was 7.5.
Cytochemical labelling
For cytochemical labelling the grids were first floated, with the tissue sections on the under-surface, for 5 min on a large drop of PBS. In a second step, the sections were incubated on a drop of one of the RNase–gold complexes. This incubation was carried out for various lengths of time from 5 min to over 2 h at 4 °C, at room temperature and at 37 °C. The grids were then thoroughly washed with PBS and rinsed in distilled water. During the entire procedure care was taken to prevent drying of the sections. They were subsequently stained with uranyl acetate and lead citrate and examined with TEM.
In order to determine the specificity of the labelling, the following control was tested. Tissue sections were incubated with the enzyme–gold complex to which the corresponding substrate (RNA 1 mg/ml) was added (Fig. 4B) (see later).
TUNEL assay
In situ TUNEL analyses were performed with ovarian fragments using a commercial assay kit (Apoptosis Detection System, Fluorescein, Promega). Serial section of ovary, 4–6 μm thick, were attached to glass microscope slides. The sections were deparaffined, rehydrated, washed with a hypotonic solution (0.85% sodium chloride) and air dried. Slides were fixed in 4% formaldehyde solution in PBS, pH 7.4. The samples were washed by immersing the slides in PBS for 5 min at room temperature and then washed twice with PBS. Sections were permeabilized with proteinase k (20 μg/ml) for 20 min at room temperature, washed twice with PBS and incubated with TUNEL reagents according to manufacturer's protocols. Two slides each were used for negative and positive controls before incubation with TUNEL. Negative-control slides were incubated as describe above, but without the addition of TdT. Positive-control slides were preincubated in the presence of DNase I for 15 min at 37 °C (100 ng/ml) and then washed twice in PBS before incubation.
After the TUNEL reactions were complete, the slides were washed in PBS, sealed under coverslip with nail polish and examined using an Ilford HP5 (ASA 400) fluorescence microscope equipped with a standard filter set for visualization of green fluorescence of fluorescein isothiocyanate-12-dUTP-labelled-DNA (fragmented DNA) within the apoptotic follicle cells.
Results
Follicular epithelium of early previtellogenic oocytes
The ultrastructural analyses of early previtellogenic follicles show a follicular epithelium made up of a single layer of squamous cells forming a continuous monolayer that surrounds the oocyte. Adjacent cells exhibit superposition among them on the marginal zone. The plasma membrane of the follicular cells is in close apposition to the oocyte membrane and they have a clear cytoplasm (Fig. 1A, B).

Figure 1 Electron micrographs of C. cranwelli early previtollogenic follicles showing the follicle cell epithelium. (A) Perpendicular section of the follicular epithelium. Notice that adjacent follicle cell (fc) overlap and plasma membranes of oocyte and follicle cell are in apposition (arrows). Scale bar represent 0.4 μm. (B) The cytoplasm is featureless, presenting few ribosomes and RER. The marginal zone shows follicle cells (fc) superposition. Scale bar represents 0.3 μm; t: theca, Oo: oocyte. (C) Tangential section showing the nucleus (n) and nucleolus (nu) of follicle cells; M (mitochondria). Scale bar represents 1.4 μm.
In superficial follicular sections, follicle cells show a polygonal shape, with a large round nucleus and electron-lucent cytoplasm (clear cells). The nucleus has a prominent nucleolus and highly dispersed chromatin and is surrounded by a thin layer of cytoplasm with few ribosomes and cisternae of rough endoplasmic reticulum and mitochondria (Fig. 1C).
Follicular epithelium of mid-previtellogenic oocytes
The ultrastructural analyses of the follicular epithelium of mid-previtellogenic follicles show a squamous epithelium similar to that of early previtellogenic follicles. However, this epithelium is formed by two types of cells, one similar to clear cells and the other characterized by electron dense cytoplasm filled with free ribosomes (dark cells) (Fig. 2A–C, E). Dark cells can have a length of over 30 μm in perpendicular section (Fig. 2D).
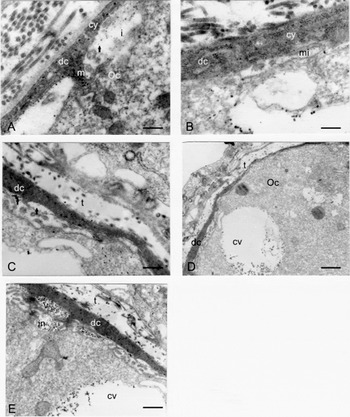
Figure 2 Electron micrograph of a C. cranwelli mid-previtellogenic follicle showing the follicle cell epithelium and the interface with the oocyte cortex. (A) Dark follicle cell (dc) with electron dense cytoplasm (cy) and a macrovilli (m) that contacts the oocyte (Oo) plasma membrane. The interface (i) shows few particles (arrow). Scale bar represents 0.3 μm. (B) Dark follicle cell cytoplasm (cy) with abundant free ribosomes. The interface shows a microvilli with microfilaments (mi). Scale bar represents 0.25 μm. (C) Dark follicle cell with vesicle in the apical surface (arrow). Scale bar represents 0.35 μm. (D) Long dark follicle cell (dc) surrounding the oocyte (Oo). The oocyte cortex displays cortical vesicles (cv). Scale bar represents 1.1 μm. (E) Dark follicle cell (dc) with a vesicle full of particles at the apical surface (v); the oocyte plasma membrane has an invagination with the same particulate material (in). The oocyte cortex also displays a cortical vesicle with the same particulate material (cv). Scale bar represents 0.7 μm; t: theca.
Near the apical surface in the cytoplasm, the dark cells have vesicles containing two types of particles: one about 15–25 nm and the other 25–50 nm (Fig. 2E). The vesicles are separated from the interface by a thin discontinuous cytoplasm. Follicle cells exhibit long processes called macrovilli that extend from the apical surface and make contact with the developing oocyte (Fig. 2A, E). Because of the development of macrovilli and vesicles, the follicle cells appear arched over the oocyte. The oocyte surface displays cytoplasmic projections called microvilli (Fig. 2E).
At the interface, particles identical to those of the dark cell vesicles can be observed between the macrovilli and the microvilli (Fig. 2E). At this stage the oocyte has endocytic invaginations that incorporate these particles, forming peripherally arranged cortical vesicles ranging from about 2 to 3 μm (Fig. 2B, D, E). In vitellogenic oocytes, these vesicles full of particles and membranes are present in the oocyte cortex (Fig. 3).

Figure 3 Electron micrograph of a C. cranwelli vitellogenic follicle. The oocyte (Oo) cortex shows a cortical vesicle (cv) with numerous particles; ve: vitelline envelope. Scale bar represents 1.3 μm.
For examination of RNA at the ultrastructural level, thin sections of previtellogenic oocyte were incubated with the RNase–gold complex. Gold particles are present inside the cortical vesicles that contain the particulate material (Fig. 4A).

Figure 4 RNA localization by RNase–gold complex. (A) Electron micrograph of a mid-previtellogenic follicle incubated with RNase–gold complex showing a cortical vesicle (cv) with particles labelled inside it in the oocyte cortex. (B) Section of the control incubated with RNase–gold complex + RNA. No particles are present inside the cortical vesicle. Scale bar represent 0.7 μm; fc: follicle cell.
Pulse–chase experiments
To find out whether the follicular cells from mid-previtellogenic follicles are actively involved in the RNA synthesis, especially in the polyadenylate RNA, follicles were incubated with [3H]-adenosine. In autoradiographs of a previtellogenic follicle incubated with the precursor for 5 h, intense radioactive labelling is present in the follicle cells, mainly in the nucleus and dense cluster of silver grains appear mainly in the nucleolus of the oocyte germinal vesicle Fig. 5A).

Figure 5 Pulse–chase experiments. Autoradiography sections of mid-previtellogenic follicles of C. cranwelli incubated for 5 h in [3H]-adenosine and then transferred for 4 h; 9 h and 24 h to 1 mM adenosine solution. (A) Follicle section incubated for 5 h in [3H]-adenosine, showing intense radioactive labelling in follicle cell nucleus (arrow) and oocyte nucleus (n) and nucleolus (nu). Scale bar represent 80 μm. (B) Follicle section incubated for 4 h in 1 mM adenosine solution. Radioactive labelling can be seen in the nucleus (n) and cytoplasm (cy) of the follicle cell Scale bar 20 μm. (C) Follicle section incubated for 9 h in 1 mM adenosine solution. The radioactive labelling can be seen in the interface between the follicular epithelium and the oocyte (i). Scale bar 20 μm. (D) Follicle section incubated for 24 h in 1 mM adenosine solution showing a decrease in silver deposition in the follicle cell and an accumulation of silver grain on the oocyte cortex. Scale bar represent 20 μm; fc: follicle cell.
We carried out pulse–chase experiments to study the movement of the newly synthesized RNA. After a 5 h pulse with [3H]-adenosine, previtellogenic follicles were transferred to fresh medium containing unlabelled adenosine and incubation was continued for 4 h, 9 h and 24 h. Figure 5B–D illustrates the distribution of the radioactive RNA after the different chase periods. As shown in Fig. 5B, the material labelled after 4 h of incubation was almost entirely chased into the cytoplasm of the follicle cells and after 9 h it was found between the follicle cells and the oocyte (Fig. 5C). After 24 h there was a decrease in silver deposition in the follicular cells and an accumulation of silver grains on the oocyte cortex (Fig. 5D).
Follicular epithelium in late previtellogenic oocytes
The monolayered epithelium in late previtellogenic follicles is formed by dark and clear cells. The dark cells partly overlap the clear cells and extend between them and the basal membrane of the epithelium (Fig. 6A, B). However, there are several regressing dark cells that present different morphological alterations characteristic of the apoptotic process. The apoptotic dark cells can be observed more clearly in a tangential than in a transverse section of the epithelium. These cells show an irregularly outlined nucleus, a large nucleolus and condensations of chromatin accumulating at the nuclear rim. The nuclear membrane in some regions is swollen. The cytoplasm contains organelles but these appear less abundant than in mid-previtellogenesis (Fig. 7A).

Figure 6 Electron micrograph of a C. cranwelli late previtellogenic follicle showing the follicular epithelium and the interface with the oocyte cortex. (A) The epithelium displays clear (cl) and dark cells (dc). At the periphery the dark cell body extends over the clear cell. Arrow shows chromatin near the clear cell. In the interface (i) fibrillar material, the precursor of the vitelline envelope, begins to appear. Scale bar represent 0.5 μm (B) Dark cell with vesicles containing fibrillar material (asterisk) and a large vacuole with smooth endoplasmic reticulum (va). The oocyte cortex has cortical vesicles (cv) and several apoptotic bodies (arrow). Scale bar represents 2 μm.
Other dark cells present a more advanced stage of degeneration; there is loss of contact with adjacent cells, the cytoplasm is shrunken and the cell is surrounded by a large empty space. The nucleus presents extensive lobes with condensed chromatin and fragments of lobes near the nucleus (Fig. 7B). The cytoplasm of these cells contains a large number of vacuoles of various sizes, some of them with remains of chromatin or dif-ferent materials and clumps of electron dense material as well as multivesicular bodies (Fig. 7C). Dark cell cytoplasm can also be seen between clear cells in a tangential section of the follicular epithelium (Fig. 7D).

Figure 7 Electron micrograph of C. cranwelli late previtellogenic follicle showing tangential section of follicular epithelium. (A) Epithelial dark cell in early stage of regression with an irregular nucleus (n) and several patches of condensed chromatin accumulated at the nuclear membrane (asterisks). The cytoplasm (cy) contains few free ribosomes. Scale bar represents 1.4 μm. (B) Dark cell in late stage of degeneration showing a lobed nucleus (n) with highly condensed chromatin. Cytoplasm shrinkage produces loss of contact between adjacent cells. Note the presence of empty intercellular spaces (asterisk). Scale bar represents 1.4 μm. (C) Cytoplasm (cy) of apoptotic cell showing numerous vesicles, some of them with chromatin (white arrow); clumps of electron dense material (black arrow) and multivesicular bodies (mb) Scale bar represents 1.4 μm. (D) Two clear cells and between them the cytoplasm of dark cell with numerous apoptotic bodies (white arrows) and vesicles (v). Scale bar represents 0.5 μm.
On a perpendicular section in the interface, a packet of chromatin can be observed near the clear cells, which probably take up this material from the dark cells. Apoptotic bodies are clearly visible in the oocyte cortex. One or several masses of chromatin are present inside the apoptotic bodies (Fig. 6A, B).
To demonstrate nuclear DNA fragmentation, thin ovary sections were treated with the Apoptosis Detection System. Figure 8 shows no apoptotic cells in the mid-previtellogenesis stage. The first positive signal can be detected at late previtellogenesis.

Figure 8 Light micrographs of previtellogenic oocytes double labelled with TUNEL and DAPI. (A) Early previtellogenic follicle; no signal of apoptosis is evident at the follicle cell epithelium (white arrow). Late previtellogenic follicle; positive signal is evident at the follicle cell epithelium (empty arrow). (B) The same micrograph showing the follicle cell epithelium nuclei counterstained with DAPI. Scale bar represents 30 μm.
Discussion
Our results demonstrate for the first time that the fol-licular epithelium of the amphibian C. cranwelli undergoes relevant modifications during the previtellogenic period of oogenesis. In the early previtellogenesis the epithelium consists of a single layer of squamous polygonal cells with clear cytoplasm. Interestingly, the boundaries of the epithelial cells are overlapping. This cytological characteristic would allow the epithelium to extend and the cells to spread over the surface of the oocyte as follicle growth goes on. The ultrastructural analysis showed that the plasma membrane of follicle cells is in close apposition to the plasma membrane of the oocyte. The interface shows no signs of an exchange of material between them.
During the mid-previtellogenic stage, two types of cells develop in the follicular epithelium: dark cells and clear cells. The dark cells appear sequentially as the stage progresses. Dark cells display ultrastructural features indicative of great synthetic activity, including large amounts of free ribosomes, glycogen, a rough endoplasmic reticulum and particle-filled vesicles. Until now, cells similar to dark cells had been described only in the avian granulosa epithelium (Callebaut, Reference Callebaut1991).
To clarify the role of the dark cells during the growth of previtellogenic oocytes, we performed ultrastructural and autoradiographic studies. Experiments with tritiated adenosine demonstrate that the follicular cells are actively involved in RNA synthesis. Moreover, pulse–chase experiments showed the migration of the radioactive granules to the oocyte, suggesting a transfer of RNA. In the oocyte cortex, vesicles containing ribonucleoproteins appear, as shown by the RNase–gold complexes. The proposed flow of material toward the oocyte is strongly supported by the ultrastructural presence of the same vesicles with the same components in vitellogenic oocytes.
Throughout the previtellogenesis stage amphibian oocytes accumulate RNAs for their subsequent use in early embryogenesis (Davidson, Reference Davidson1986; Kloc et al., Reference Kloc, Dougherty, Bilinski, Chan, Brey, King, Patrick and Etkin2002). Thus, an important characteristic of previtellogenic oocytes is the very active synthesis because of rRNA being synthesized on amplified rDNA sequences and of heterogeneous RNA being synthesized on lampbrush chromosomes. Our findings demonstrated that during mid-previtellogenesis, dark follicle cells add RNA to the oocyte cytoplasm; thus, cortical vesicles containing RNA appear in the oocyte cortex. This transfer is similar to that observed in insects and other invertebrates, where nurse cells support oocyte development by supplying nutrients, RNAs, proteins and organelles (Bonhag, Reference Bonhag1958; King & Devine, Reference King and Devine1958; Berry, Reference Berry and Browder1985; Robinson et al., Reference Robinson, Kant and Cooley1994; Nezis et al., Reference Nezis, Vassilis, Mpakou, Stravopodis, Papassideri, Mammali and Margaritis2003). The same process was observed in lizards between the pyriform cells and the oocyte (Andreuccetti, Reference Andreuccetti1992).
The presence of cortical vesicles in C. cranwelli oocytes would be interpreted as an evolutionary mechanism that provides enough RNA to allow rapid oocyte development, considering that the level of RNA in the oocyte is correlated with the speed of embryonic development, as suggest by Del Pino (Reference Del Pino1989).
In this context, C.cranwelli previtellogenic oocytes contain high levels of RNAs not only because of gene amplification that produces multiple nucleoli, but also of the RNAs from the follicle cells present in the cortical vesicles. High RNA levels ensure a rapid development in view of the fact that this species must adapt to certain weather conditions (storms) for egg deposition to occur.
Surprisingly, as previtellogenesis progresses, a new remodelling of the follicular epithelium takes place and at the end of this stage a degeneration of dark follicle cells has evidently occurred. Our findings demonstrated that this process is associated with programmed cell death.
Degenerating cells showed the typical features of apoptosis: the condensation of chromatin around the nuclear perimeter, cell shrinkage, membrane blebbing and release of apoptotic bodies. The remodelling of the follicular epithelium was also observed in other vertebrates like the lizard Podarcis sicula, where the regression of the epithelium was observed by morphological and biochemical analyses (Motta et al., Reference Motta, Filosa and Andreuccetti1996). Moreover, the same cellular mechanism was described in granulose cells in mammals (Hughes et al., Reference Hughes and Gorospe1991). These findings raise the interesting possibility that programmed cell death could be a potential mechanism underlying the follicular epithelium of vertebrate oogenesis.
The apoptotic bodies released from regressing dark cells are apparently engulfed by the oocyte and can be seen in the oocyte cortex. However, the functional significance of the apoptotic bodies inside the oocyte is unknown at present.
Our ultrastructural results evidence that during the previtellogenesis stage in the C. cranwelli oocyte there is a reorganization of the follicular epithelium that takes place as the oocyte grows.
Acknowledgements
This work was supported by grants to S. Sánchez from Consejo Nacional de Investigaciones Científicas y Técnicas (CONICET) and Consejo de Investigaciones de la Universidad Nacional de Tucumán (CIUNT). The authors thank Mr Enrique Dozetos for his photographic assistance and Mrs Virginia Méndez for her proofreading.