1. Introduction
Much of the recent work on microbialites has been focused on the identification of the bacteria involved, their calcification and their distribution through time (Riding, Reference Riding2000, Reference Riding, Reitner and Thiel2011). Microbialite accumulations can, however, provide valuable information for environmental interpretation. The importance of the physical environment in controlling the form of microbialite accumulations has been recognized (Carozzi, Reference Carozzi1962; Logan, Rezak & Ginsberg, Reference Logan, Rezak and Ginsburg1964; Playford, Reference Playford1980; Andres & Reid, Reference Andres and Reid2006; Eckman et al. Reference Eckman, Andres, Marinelli, Bowlin, Reid, Aspden and Paterson2008) but studies have been focused on limited ranges of forms and are therefore of limited applicability. Trompette (Reference Trompette1982) proposed a model for the effects of microbial and environmental influences on the development of microbialites, which Andres & Reid (Reference Andres and Reid2006) modified in their study of Bahamian marine microbialite accumulations, where they also noted the influence of macro-biological factors such as grazing. Andres & Reid (Reference Andres and Reid2006) considered the key environmental factors to consist of accommodation space, sediment and hydrodynamics, with macro-biological factors of increased importance where environmental factors were lacking.
The aim of this paper is to describe the wide range of stromatolite forms found within the Middle Old Red Sandstone (MORS) of the Orcadian Basin. Detailed observations made from western Orkney and Caithness are utilized alongside previously described examples (Fannin, Reference Fannin1969; Donovan, Reference Donovan1973, Reference Donovan1975; Janaway & Parnell, Reference Janaway and Parnell1989; Parnell & Janaway, Reference Parnell and Janaway1990; Parnell, Marshall & Astin, Reference Parnell, Marshall and Astin1990) to better understand stromatolite distribution and controls thereof within lacustrine settings and produce a model for their palaeoenvironmental distribution. Comparisons are made with Late Triassic examples from East Greenland and indicate that the model proposed has general applicability to microbialite accumulations in similar lacustrine environments.
2. Setting
The predominantly lacustrine MORS of Northern Scotland was deposited in a number of half-graben basins, collectively termed the Orcadian Basin, which stretched from Inverness, in the south, to Shetland, in the north (Fig. 1). The Orcadian Basin lay between 20° and 30° south of the equator during the deposition of the MORS. Orbitally controlled climatic variance resulted in regular fluctuations in lake level within the basin leading to the deposition of cyclic sedimentary successions (Andrews & Trewin, Reference Andrews and Trewin2010). The lake water was predominantly fresh, as indicated by the paucity of evaporites; however, periods of elevated salinity have been suggested on the basis of δ18O studies (Duncan & Hamilton, Reference Duncan, Hamilton, Fleet, Kelts and Talbot1988). Stromatolites are present throughout the basin; however, the greatest variety of forms occurs in the Lower Stromness Flagstone Formation of western Orkney (N. G. T. Fannin, unpub. Ph.D. thesis, Univ. Reading, 1970), the presence of which was first described by Heddle (Reference Heddle1880). Their abundance in western Orkney is probably the result of low sedimentation rates in a low-relief region of the basin (Andrews & Trewin, Reference Andrews and Trewin2010). A similar, low sedimentation rate, low-relief setting is postulated for stromatolites from the Upper Caithness Flagstone Group in the Thurso area on the north coast of mainland Scotland (Fig. 1) (Parnell, Marshall & Astin, Reference Parnell and Janaway1990; S. D. Andrews, unpub. Ph.D. thesis, Univ. Aberdeen, 2008). Stromatolite accumulations have also been described by Donovan (Reference Donovan1973) from central Caithness at Dirlot Castle where they are seen to coat boulders up to 1.2 m in length that mantle a basement high. Similar accumulations have been described from a number of localities along the north coast of Caithness (Donovan, Reference Donovan1975; Janaway & Parnell, Reference Janaway and Parnell1989).

Figure 1. Generalized Middle Old Red Sandstone palaeogeography and locality map. D – Dirlot; R – Red Point/Isauld Burn; T – Thurso; S – Stromness; Y – Yesnaby; B – Birsay; Ro – Rousay; GGF – Great Glen Fault (modified from Trewin & Thirlwall, Reference Trewin, Thirlwall and Trewin2002).
The stromatolite examples described from the Upper Triassic of East Greenland are found within the Edderfugledal Member (Fleming Fjord Formation), which was deposited within the Jameson Land Basin that stretches from 71°19′ to 72°12′N. Accumulation of the stromatolites described was in a low-relief lacustrine setting similar to that of most of the MORS examples. The Edderfugledal Member consists of cyclic intercalations of grey wave-rippled sandstones, dolomitic mudstones and laminated dark grey mudstones, which have been described in detail by Clemmensen (Reference Clemmensen1978). During the Late Triassic, the Jameson Land Basin lay in the northern arid belt (Kent & Clemmensen, Reference Kent and Clemmensen1996).
3. Occurrence
The sedimentological context of the stromatolites is of great importance in understanding the controls on their form. The occurrences recorded in the MORS of the Orcadian Basin can broadly be divided into those from coincident lake margins, where lake waters lapped on to basement, and those which formed where the lake margin was distant from the basin margin, each having distinct environmental controls.
Examples of the former include those from Dirlot (Donovan, Reference Donovan1973), Isauld Burn Mouth (Donovan, Reference Donovan1975), Red Point in Caithness (Janaway & Parnell, Reference Janaway and Parnell1989) and adjacent to basement islands around Stromness (Fannin, Reference Fannin1969). These localities are characterized by pebble to boulder (up to 1.2 m) breccias which mantle an uneven, knobbly surface with basement knolls that are often steep sided. Clasts are angular and largely of local derivation. The breccias have been interpreted as locally derived scree (Donovan, Reference Donovan1973) which was reworked in a lake shoreline setting. Stromatolite accumulation in these localities is halted by the eventual inundation and overtopping of the basement relief through continued sedimentation.
The stromatolite examples from Orkney (West Mainland and Rousay) and the Thurso area formed in regions where the lake margin was distant from the basin margin and consequently relief was largely subdued. The MORS succession in these regions is dominated by cyclic lacustrine deposits. The cyclic sequences of the Lower Stromness Flagstone Formation, which are similar to those of the Upper Caithness Flagstone Group of the Thurso area, are composed of regular intercalations of sand flat, playa, perennial lake and deep lake facies (Fig. 2) reflecting regular climatically controlled fluctuations in lake level (Andrews & Trewin, Reference Andrews and Trewin2010). Stromatolite accumulations are most common within the perennial lake facies, with a greater frequency of occurrence recorded in the transgressive sequences. Stromatolites are also more rarely recognized within the playa facies. The increased abundances of stromatolites within the transgressive sequences may be accounted for by reduced sedimentation rates resulting from the migration of sediment input points towards the basin margin as a result of rising lake levels. Sedimentation rates have also been interpreted as an important control on microbialite development in Neoarchaean examples recorded in South Africa (Schröder, Beukes & Sumner, Reference Schröder, Beukes and Sumner2009).

Figure 2. A single asymmetric cycle in the Lower Stromness Flagstone Formation, from Skerry of Ness, Stromness, illustrating the common development of stromatolites during the transgressive phase. A summary of the lithological features which characterize the environments identified is also given.
The perennial lake facies consists of silt/very fine sandstone laminae between 1 and 5 mm thick which often contain mudstone rip-up clasts and display loaded bases. Intercalated with the silt/very fine sandstone laminae are grey to dark grey carbonate-rich mudstone laminae which average 10 mm in thickness and commonly weather to a characteristic buff colouration (dolomitic). Syneresis cracks are common with the occurrence of polygonal desiccation cracks being rare. Thicker sandstone beds are common in the regressive sequences but are largely absent in transgressive instances. Oscillation and current rippling are occasionally present in some of the thicker siltstone/sandstone laminae.
The largely fine-grained nature of the perennial lake facies reflects the dominantly low-energy environment of deposition. The mudstone component is interpreted as the settling of fine sediment in a standing body of water with the coarser laminae reflecting under-, inter- and overflow events. Rare rippling within the siltstone/sandstone laminae indicates occasional periods of increased energy, possibly reflecting the lowering of wave base during storm conditions. Falling stage flow during turbidite deposition may also account for some rippling. Abundant syneresis cracks are interpreted as the result of regular fluctuations in the salinity at the sediment–water interface. The rarity of desiccation cracks is indicative of the perennial nature of the lake during the deposition of this facies.
The playa facies is similarly composed of alternations between silt/very fine sandstone and carbonate-rich mudstone/siltstone laminae which reach 1–10 mm and 10–40 mm in thickness, respectively. Syneresis and desiccation cracks are abundant throughout the playa facies, and aligned runnels, up to 0.10 m deep and 0.30 m wide, are also recognized.
Deposition of the playa facies occurred in a predominantly low-energy environment. The mudstone components reflect deposition from suspension within a standing body of water; however, the abundant desiccation horizons indicate regular fluctuations in water level. Variations in salinity are also recorded by the presence of syneresis cracks.
4. Stromatolite microstructure
Despite the variety of forms, only two types of microstructure are identified. The first, and most common type, occurring in the majority of examples, is characterized by its finely laminated (sub-millimetre – 2 mm) nature, which is composed of alternations between micritic and organic-rich laminae. Faint, dark, vertical features up to 100 μm wide and 750 μm long can be discerned within some sections of the micritic laminae (Fig. 3a). These may be microbial filaments but the full morphology cannot be determined. Similar structures were identified by Parnell & Janaway (Reference Parnell and Janaway1990) in microbialites from Stromness, which they interpreted as Girvanella.

Figure 3. Stromatolite microtextures. (a) Photomicrograph (cross-polarized light) illustrating traces of algal filaments preserved in the laterally linked hemispheroids of the sand-cored stromatolite mounds. (b) Photomicrograph of clotted texture and entrained ooid, characteristic of agglutinated stromatolites, from the reefal stromatolite mounds. (c) Polished hand-specimen from the reefal stromatolite mounds illustrating the poorly laminated nature of the agglutinated texture.
The second type is restricted to the reefal stromatolite build-ups, within which the internal structure contains only poorly developed lamination and a predominantly clotted dolomitic microtexture (Fig. 3b) with birdseye fenestrae. The lamination itself is largely defined by subtle variations in the clotted texture and the presence of variably defined organic laminae (Fig. 3c). Some examples also contain detrital material such as sand, intraclasts and ooids (Fig. 3b), which also define the lamination in some instances.
Parnell & Janaway (Reference Parnell and Janaway1990) noted the clotted microstructure of the reefal stromatolites of Stromness as thrombolitic; however, Aitken (Reference Aitken1967) defined a thrombolitic texture to be a macroscopic feature. Riding (Reference Riding2000) suggested that the generation of the clotted microtexture, common in stromatolites, is through the calcification of extracellular polymeric substances (EPS), which microbes produce to form protective and adhesive barriers around the cell. The more crudely developed lamination and the presence of sand, intraclasts and ooids are most closely comparable to the agglutinated stromatolitic microstructure described by Riding (Reference Riding2000), which he recognizes in the modern examples from both Shark Bay, Western Australia, and Lee Stocking Island, the Bahamas. Examples of agglutinated stromatolites in the rock record are rare (Martin, Braga & Riding, Reference Martin, Braga and Riding1993; Braga, Martin & Riding, Reference Braga, Martin and Riding1995; Riding, Reference Riding2000) and therefore the examples described here are an important addition to our knowledge of microbialite forms and their environmental interpretation.
5. MORS stromatolite forms
5.a. Clast-coating stromatolites
Clast-coating stromatolites have been described from Caithness and Orkney (Fannin, Reference Fannin1969; Donovan, Reference Donovan1973, Reference Donovan1975; Janaway & Parnell, Reference Janaway and Parnell1989) within basement-mantling breccias that have been interpreted as wave-reworked scree deposits (Fig. 4). Donovan (Reference Donovan1973) described three stages in their development based on examples from Dirlot in central Caithness: an initial massive ferroan dolomite coating (0.1–1 mm) followed by flat laminated stromatolitic lamination (up to 12 mm) which is in turn succeeded by the accumulation of digitate or laterally linked hemispheroid stromatolite forms completing a coating up to 80 mm thick (Fig. 4). The thickest and most fully developed stromatolite coatings are recorded on larger boulders reflecting their greater stability. However, the development of fully circumferential coatings to the clasts reflects their regular overturn during high-energy conditions at the lake margin. Such conditions also resulted in erosion of the coatings, in some instances producing stromatolite clasts up to 150 mm long which in turn developed stromatolite coatings, but more regularly resulting in angular fragments which were preserved as the matrix to the basement-mantling breccias, alongside locally derived angular pebbles. Stromatolite coatings have also been recorded adhering directly to the basement at Isauld Burn (Donovan, Reference Donovan1975). The clast-coating stromatolites described from elsewhere in Caithness and Orkney display a broadly similar suite of features to those at Dirlot but tend to be less fully developed, such as those at Red Point where clast coatings are of sub-millimetre thicknesses (Janaway & Parnell, Reference Janaway and Parnell1989).

Figure 4. (a) Reconstruction of the setting of the clast-coating stromatolite at Dirlot, Caithness. (b) Circumferential clast coatings within the basement-mantling breccias. (c) Well-developed digitate clast-coating stromatolite forms.
The overturning of cobble-grade clasts indicates periodic exposure to high-energy conditions during the formation of the stromatolite coatings; however, the poorly sorted nature of these deposits mitigates against regular reworking. The relief of the basement knolls on which the clast-coating stromatolites formed resulted in negligible sediment supply, which was favourable for microbialite growth. The cessation of microbialite accumulation in these settings is commonly marked by the inundation of the basement topography with sandstones of fluvial origin or sandy lacustrine flagstones as seen at Dirlot.
5.b. Stromatolite sheets
Stromatolite sheets have been recorded in the Lower Stromness Flagstone Group of Western Mainland, Orkney, and in the Upper Caithness Flagstone Group in Thurso. The facies within which they are recorded consists of massive dolomitic mudstones with intercalations of minor millimetre-scale sandstone laminae. The absence of desiccation cracks suggests a perennial lacustrine setting (Fig. 2); however, stromatolite colonization of the sediment surface is likely to have inhibited the formation of such features. No evidence for wave action is recorded in association with the stromatolite sheets, so a predominantly low-energy environment can be inferred.
The stromatolite sheets form extensive horizons up to 10 mm thick. The microbial laminae are composed of a thin (1–2 mm) organic component and associated micritic and carbonate-rich mudstone components. Deformation of the stromatolitic lamina and associated deformation of the underlying sediment is common with the generation of domal features, downturned folds and fractures (Fig. 5a). Sediment-filled pustular features, involving thicker mat developments, have been recognized in stromatolite sheet deposits within the Lower Stromness Flagstone Group of Yesnaby (Fig. 5e). The fill of such features tends to be of a coarser nature. The overlying sediment is largely draped over the underlying stromatolitic laminae. The surface texture of the stromatolitic sheets is largely smooth with some examples displaying fine, shallow fracturing. Circular holes, 2–5 mm in diameter, with upturned margins (Fig. 5b) are also recorded on the well-exposed surface of stromatolitic sheets within sinuous runnels in Hesta Geo, Birsay (Fig. 5d). The runnels are commonly filled with partially brecciated algal material but those areas of mat which have not been reworked provide excellent detail of the mat surfaces.
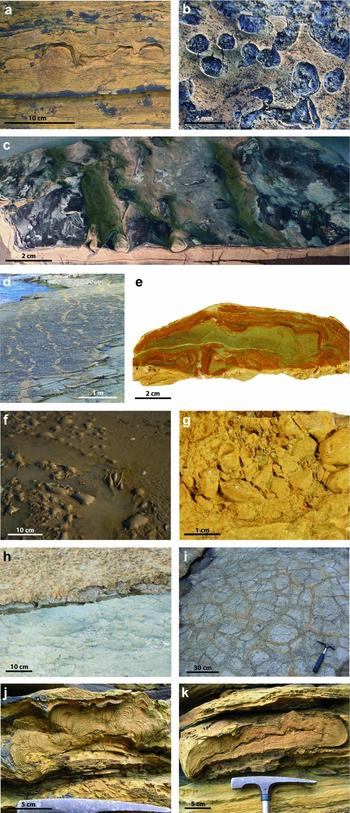
Figure 5. Mesoscale stromatolite textures and forms. (a) Single thread stromatolite sheet in the Lower Stromness Flagstone Formation, Skerry of Ness, Stromness. (b) Surface detail of well-exposed stromatolite sheets, Hesta Geo, Birsay. (c) Ruckled surface texture of stromatolite sheets in the Triassic Edderfugledal Member of East Greenland. (d) Sinuous runnels in the Lower Stromness Flagstone Formation of Hesta Geo, Birsay. (e) Polished section of a sediment-filled pustular feature from a stromatolite interval within the Lower Stromness Flagstone Formation, Yesnaby. (f) Pustular microbial mat in a fresh meltwater pool in a back beach setting, Vega Sund, East Greenland. (g) Subtle weathering of dolomitic mudstones reveals the presence of proto-oncoids in the Lower Stromness Flagstone Formation, Skerry of Ness, Stromness. (h) Erosion of microbially bound sediment, generating pebble-sized intraclasts at a channel margin in the sand flats west of Norret, Mestersvig, East Greenland. (i) Desiccation crack fringing stromatolites developed along the upturned margins of desiccation polygons within the Upper Stromness Flagstone Formation, Quoynalonga Ness, Rousay. (j) A domal stromatolite mound in the Lower Stromness Flagstone Formation, Skerry of Ness, Stromness. (k) Jelly-roll structure in the Lower Stromness Flagstone Formation, Skerry of Ness, Stromness.
The stromatolitic sheets represent microbial colonization of a soft substrate which led to the stabilization of the sediment surface. Interlaminations of dolomitic mudstone, sometimes infilling convolutions, reflect periods of sedimentation before re-colonization of the sediment surface. The buckling of the mats to form domal features and in some cases the associated desiccation suggests that the mats periodically underwent exposure; however, the well-developed lamination within the mats suggests that exposure was not regular (Hoffman, Reference Hoffman and Walter1976). The nature of these deformation features suggests that the mats were not subject to early lithification. Although flexible and strong during formation, it seems likely that these mats would become dry, brittle and possibly weakly lithified if exposed to prolonged desiccation, potentially contributing to the formation of stromatolite chip breccias seen in association with other forms. Minor deformation of the overlying sediment is likely to be related to compaction. Deformation of the underlying sediment may have resulted from contraction of the microbial material when exposed. Sediment is likely to have been drawn upwards into the domal features during this process. Where filled with coarser material as in the example described from Yesnaby it seems likely that these features contained a void into which, following rupturing, sediment was washed. Similar processes have been observed in modern examples from Carbla, Shark Bay, Western Australia and in back beach areas of Vega Sund, East Greenland (Fig. 5f).
Microbial mats are found in many modern environments and largely prefer shallow-water conditions with restricted sediment supply (Davies, Reference Davies, Logan, Davies, Read and Cebulski1970). Schieber (Reference Schieber1998) suggested that modern examples can withstand strong currents owing to their cohesive nature; however, Hoffman (Reference Hoffman and Walter1976) recognized that mats tended to be characteristic of embayment environments where generally quiescent conditions predominate. Observations made of modern microbial mat developments in the Kong Oscar Fjord and Vega Sund regions of East Greenland concur with those of Hoffman (Reference Hoffman and Walter1976) from Western Australia, with mats restricted to the upper portions of intertidal areas in sheltered embayments where the regularity of sediment reworking is low. In the modern East Greenland examples the mats themselves are several millimetres thick but are easily ruptured and torn and would be unlikely to survive in high-energy settings. Gas filled pustules up to 150 mm in diameter are also recorded which, when ruptured, do trap sediment (Fig. 5f). Davies (Reference Davies, Logan, Davies, Read and Cebulski1970) reported pustules in convoluted microbial mats from the Gladstone Embayment of Shark Bay, Western Australia, and noted that the pustules formed as loci for gas escape from the underlying sediment, which in turn filled the pustules (Davies, Reference Davies, Logan, Davies, Read and Cebulski1970, fig. 8c). Such a process could be envisaged for the sediment-filled pustules recorded from the thicker stromatolitic sheet developments described from Yesnaby (Fig. 5e); however, such a mechanism seems unlikely in the thinner stromatolite sheets where the pustule would be likely to collapse during such a process. The substrate and the thickness of the mat development are likely to be controlling factors in which process is prevalent.
5.c. Proto-oncoids
Mudstone beds composed of rounded dolomitic clasts up to small pebble grade (Fig. 5g) are recorded in the perennial lake facies (Fig. 2) of the Lower Stromness Flagstone Group but are rare. Internally, a clotted texture is recognized, and in some instances the margins of the clasts are of a darker colour with some degree of preferential development on one side of the clasts. The matrix is composed of silt and sand grains, some of which have undergone replacement with carbonate.
The darker colouration around the margins of the clasts is interpreted as an indication of increased organic content, the most likely origin of which would be microbial. Some examples have a more complete coating of the darker, organic-rich, ‘microbial’ material suggesting the rolling of the clasts creating proto-oncoids. The more common one-sided coating may indicate that microbial growth occurred previous to reworking, as part of a microbial mat, or that following initial reworking the clasts were then not remobilized. Given their well-rounded nature, it seems that the former is more likely.
Similar deposits have been recognized in the Gladstone Embayment of Shark Bay (Davies Reference Davies, Logan, Davies, Read and Cebulski1970, fig. 16b) where the desiccation of pustular microbial mats has led to the formation of algal balls, which were then blown into depressions and deposited. Erosion of mats, binding very fine sand and silt on modern intertidal flats, along the margins of small-scale channels observed in East Greenland, results in the generation of algal fragments (Fig. 5h), commonly with sediment still adhering to the underside. Preservation of these fragments would result in clasts within which the relict upper side would contain elevated levels of organic material.
5.d. Desiccation crack fringing stromatolites
Of relatively minor occurrence in the Lower and Upper Stromness Flagstone groups of Orkney and the Upper Caithness Flagstone Group on the north coast of Caithness are desiccation crack fringing stromatolites (Fig. 5i). These are largely found within the playa facies (Fig. 2) but reflect growth during periods of flooding of the playa surface.
These stromatolite accumulations are restricted to the upturned margins of desiccation cracks where they form dolomitic coatings up to 20 mm thick and 10 mm wide. Fragments of the stromatolite material are commonly present within the desiccation crack fill.
Following inundation of the playa surface, the upturned edges of desiccation cracks provided areas for the preferential development of stromatolites owing to relatively increased levels of sunlight available. The preservation of these stromatolites may have been further enhanced by the elevated pH of water within the restricted setting of the desiccation cracks themselves, aiding in the precipitation of carbonate.
5.e. Domal mounds
Stromatolite mounds are often numerous on individual surfaces within the Lower Stromness Flagstone Group of Orkney and have also been described from the Mey Sub-Group in the Upper Caithness Flagstone Group on the north coast of Caithness (Parnell, Marshall & Astin, Reference Parnell, Marshall and Astin1990). As with a number of the stromatolite forms described here, the domal mounds are commonly developed within the perennial lake facies (Fig. 2) during the initial transgressive phases of the cycles outlined above. The mounds reach 0.05 m in height, up to 0.5 m diameter and are composed of spaced, laterally linked domes which in some examples bifurcate and tighten, generating a fanned morphology (Fig. 5j). In some examples the domes are linked by stromatolite sheets. Dolomitic mudstone laminae are intercalated with the stromatolitic laminae and thicken to form the cores of the domes where significant tightening has occurred. Planar, stratiform accumulations which are gently domed have also been recognized laterally from the more clearly developed domal mounds. Deformation of these planar accumulations has led to the generation of jelly-roll structures, on the top of which laterally linked stromatolite domes have accumulated (Fig. 5k).
The development of domal stromatolite mounds is interpreted to have originated through the perpetuation of relief generated on microbial mats. Erosion and the development of pustular features provided preferred areas for continued algal accumulation and the development of stromatolite mounds. Elevated areas provided greater availability of sunlight and an extended window for microbial accumulation before sedimentation could curtail growth. Periodic storm events were probably responsible for the disruption of the thicker algal accumulations, resulting in the generation of jelly-roll structures formed through the overturning of algal accumulations. The buckle folding recorded indicates that the stromatolites where strong but flexible during their formation.
Hoffman (Reference Hoffman and Walter1976) suggested that the development of microbialite mounds represents an increase in exposure to a higher energy environment. In the examples described above evidence is seen for erosion in the generation of initial topographic highs, which formed loci for preferential microbialite accumulation. This is consistent with increased energy in this setting, as is the presence of jelly-roll structures. Parallels can also be drawn with the algal accumulations described by Carozzi (Reference Carozzi1962) from the shores of the Great Salt Lake, Utah, which he concluded reproduced and exaggerated the sedimentary features generated around the lake margin. Carozzi (Reference Carozzi1962), moving lakeward, noted a zone of isolated mounds followed by composite rings and flat-topped mounds, tongue-like festooned ridges and finally sub-parallel festooned ridges (Fig. 6). The stromatolite mounds described here would lie at the shoreward margin of such a system; however, the relatively sporadic occurrence and the limited lateral persistence of the outcrop does not allow the microbialite forms to be traced laterally at any one horizon.

Figure 6. Zonation of algal accumulations in the shore zone of the Great Salt Lake, Utah (modified from Carozzi, Reference Carozzi1962). Cross-section A–A′ can be compared to Figure 7, which illustrates the aligned stromatolitic mound developments from the Lower Stromness Flagstone Group at Skerry of Ness, Stromness.
5.f. Aligned mounds/runnels
Aligned mounds/runnels are identified in the Lower Stromness Flagstone Group of Orkney at Skerry of Ness (Fig. 7), Stromness and Birsay (Fig. 5d) where they are found within the perennial lake facies (Fig. 2). The stromatolite mounds are similar in their basic form to those described above; however, in this instance they form well-defined ridges (Fig. 7) 0.2–1.2 m wide and up to 0.1 m high, often consisting of two well-defined ridges and an inter-ridge platform which are aligned at 90° to the palaeoshoreline. Between the largely linear stromatolite ridges are runnels. The runnels vary between 0.5 and 1 m wide and are either filled with dark finely laminated mudstones which drape the runnel margins (Fig. 7) or brecciated algal material.

Figure 7. Aligned stromatolitic mound developments (Skerry of Ness, Stromness) stabilizing the margins of large-scale runnels. Compass clinometer for scale is 0.1 m long. Inter-ridge platform areas resemble those described by Carozzi (Reference Carozzi1962) from the Great Salt Lake, Utah.
The coarse nature of the runnel fill algal breccias is indicative of the elevated energy involved in the generation of these stromatolite accumulations. Again, an erosional origin is suggested, with initial stabilization of the sediment surface through microbial mat development and subsequent erosion resulting in the generation of runnels as a consequence of wave action. Fannin (Reference Fannin1969) also suggested that the form of the runnels may be a function of the palaeoslope on which they formed. The more favourable conditions for continued microbialite growth were thus generated on the now elevated ridges, the continued stabilization of which resulted in focused erosion within the runnels. Increased sediment transport within the runnels may have contributed to the growth of the ridge edges through sediment trapping resulting in the generation of a lower inter-ridge platform. The increased erosion responsible for the creation of the runnels and aligned stromatolite mounds suggests a further increase in energy compared with the environment in which the stromatolite mounds formed. This suggests a more exposed position on the lake shore.
Comparable structures have been observed in modern examples from both lacustrine and marine settings. The aligned stromatolite ridges described here closely resemble the lakeward reaches of the system described by Carozzi (Reference Carozzi1962) from the Great Salt Lake, Utah, despite the disparity between the internal structure of the algal accumulations described by Carozzi and the stromatolites described here. Carozzi (Reference Carozzi1962) suggested that the aligned algal accumulations he described reproduce and exaggerate features characteristic of the lake margin environment. In this case, runnel systems provided the basis for the microbialite form generated. Similar structures, termed ‘ridge and rill’, have been described from Shark Bay, Western Australia (Logan, Reference Logan1981). Logan (Reference Logan1981) recognized that the rills were initiated through localized wave and tidal scours. Where such features cut through existing microbial mats, algal growth and sediment trapping continued on the higher ridges but the rills focused currents, inhibiting algal growth. Logan (Reference Logan1981) also noted that in some instances initial ‘ridge and rill’ forms produced by wave and tidal processes were only later colonized by algae, leading to the stabilization and perpetuation of the ‘ridge and rill’ structures.
5.g. Sand-cored mounds
Examples of sand-cored stromatolite mounds are relatively limited but have been identified in the Lower Stromness Flagstone Group of Orkney in the Stromness region. The sediments which form the substrate for the development of the sand-cored mounds reflect a shore zone setting with the overlying strata recording increasing water depths resulting in a deep lake environment (e.g. Fig. 2). Therefore, the stromatolites can be interpreted as forming during a period of lake transgression.
The sandstone cores reach 1 m in height, are 1–2 m in diameter and are coated with laterally linked hemispheroidal columnar stromatolites up to 0.2 m thick, with individual columns between 10 and 40 mm wide (Fig. 8a, b). Bedding is recognized within the sandstone cores, which contain oscillation and current ripples. The stromatolitic coating is replaced near the base of the mound, where the margins are vertical, with a breccia apron predominantly consisting of brecciated microbial material up to pebble size. Fine to medium-grained sand, rare ooids and pebbles are also recognized in the breccia aprons. In some instances, further stromatolite accumulations also overlie the breccia aprons.

Figure 8. Sand-cored stromatolite accumulations. (a) Lower Stromness Flagstone Formation, Skerry of Ness, Stromness (metre stick for scale, see Figure 9a(3) for a cross-section). (b) Detail of flanking debris aprons and the laterally linked hemispheroidal nature of the stromatolites in (a). (c) Sand-cored stromatolite from the Triassic Edderfugledal Member of East Greenland. Hammer for scale is 0.3 m long.
The development of breccia aprons, including clasts up to pebble grade, is indicative of a high-energy setting, and is therefore also consistent with structures identified in the underlying shore zone facies (oscillation and current ripples, see Fig. 2). Erosional processes played an important role in the formation of the sand-cored mounds, as is evidenced by the steep-sided nature of the sand cores and the truncation of bedding planes. Continued erosion of the stromatolites themselves during the development of the mounds is also indicated by the predominance of stromatolitic material within the fringing breccia aprons. Fannin (Reference Fannin1969) first recognized the influence of erosion in the formation of the sand-cored stromatolite mounds of Orkney but did not propose a more detailed mechanism for their formation.
Initiation of the sand-cored mound development is interpreted to have resulted from the initial stabilization of the sediment surface by microbial mat development followed by erosion, resulting in the generation of patchy microbial mats occupying areas of higher relief (Fig. 9a). Continued erosion of less-extensively colonized areas and continued microbial accumulation on those, now higher relief, areas resulted in the perpetuation and exaggeration of relief. The relief generated would have focused erosion around the base of the microbialite mounds generating further relief and resulting in the generation of the breccia aprons. The well-developed laterally linked hemispheroidal form of the stromatolites involved in the sand-cored stromatolite mounds suggests a lack of exposure and therefore a perennial lacustrine setting for accumulation (Hoffman, Reference Hoffman and Walter1976). The transition to the overlying deep lake is indicative of a general deepening in the environment, which is consistent with reduced periods of exposure in a more perennial lacustrine setting.

Figure 9. Models for the formation of sand-cored stromatolite mounds (a) and reefal stromatolite build-ups (b).
Examples of modern high-relief columnar microbialite accumulations described from Shark Bay by Hoffman (Reference Hoffman and Walter1976) occur at exposed headlands, which would be exposed to similarly elevated energy conditions as those inferred in the formation of the sand-cored microbialite mounds described here. Such a headland position could be envisaged for the Stromness examples given their proximity to the basal unconformity and the related basement high to the north (Parnell, Reference Parnell, Marshall and Astin1990).
5.h. Reefal stromatolite build-ups
Reefal agglutinated stromatolite build-ups are recorded from the Lower Stromness Flagstone Group in Orkney in the Stromness area. The reefal stromatolite build-ups occur close to the basal unconformity and their occurrence may be linked to areas of pre-existing relief. The stromatolites are underlain by playa facies and overlain by perennial lake facies. The presence of coarse-grained alluvial facies within the section attests to the proximity of the basement high.
Reefal stromatolite build-ups consist of numerous stacked stromatolite bodies which form large-scale reefs. Underlying the reef, subtle doming of the sediment is present (Fig. 10). The succeeding stromatolite bodies are up to 0.5 m thick and 1.5 m in diameter, often with an upwardly radiating lower portion and domed upper portion (Fig. 10). As discussed in Section 4, the reefal stromatolite build-ups differ from the others described in their agglutinated microstructure. Around the margins of the stromatolite accumulations are breccia aprons similar to those described from the sand-cored stromatolite domes consisting of fragmented algal material, dolomitic mudstone intraclasts, fine to medium sand, rare pebbles and ooids. Successive microbialite bodies and related breccia aprons accumulate in a stacked arrangement forming a reef structure with up to 1.5 m of relief (Fig. 10).

Figure 10. Reefal stromatolite build-up in the Lower Stromness Flagstone Formation, Skerry of Ness, Stromness. The notebook (circled) is 0.2 m long. See Figure 3c for detail of the internal structure.
Accumulation of the reefal stromatolite build-ups was initiated by the generation of relief through the differential accretion of algal material and trapped sediment. The relief generated provided conditions suitable for the growth of the stromatolite. The agglutinated microstructure, including clasts up to granule and small pebble size is indicative of a high-energy environment and that the microbialite surface must have been soft and adhesive. The poorly developed simple convex lamination is interpreted to reflect regular exposure, resulting in fragmentation and reworking of any microbial lamination which developed. Breccia aprons, also suggestive of high-energy conditions, formed around the margins of the stromatolites, widening the area suitable for further microbialite growth. Successive generations of growth led to the development of large-scale reef features (Fig. 9b). The reefal stromatolites are therefore interpreted to reflect accumulation in exposed, possibly basement-controlled headlands, but in a shallower water environment than that of the sand-cored stromatolites. Comparable forms with a similar internal structure have been described from exposed lower to upper intertidal settings of Shark Bay by Hoffman (Reference Hoffman and Walter1976).
6. Late Triassic stromatolite forms of East Greenland
Remarkably similar stromatolite forms to those described from the MORS of Northern Scotland have been recognized from the Late Triassic Edderfugledal Member in East Greenland. These include stromatolite sheets, aligned mounds and sand-cored stromatolite mounds, which are briefly described below.
Stromatolite sheets identified in the Edderfugledal Member occur in perennial lake facies closely resembling those of the Lower Stromness Flagstone Formation. The fine-grained nature of the sediments and the absence of ripples suggest a low-energy setting. The stromatolite sheets consist of laterally extensive sheets which are 10–20 mm thick and display a smooth to ruckled appearance (Fig. 5c). Lamination is similar to that described in the MORS examples, but within the hollows of the ruckled surface, dolomitic mud drapes are commonly observed, preceding continued microbialite accumulation.
Aligned mounds/runnels in the Edderfugledal Member show close similarities to those described from the MORS, and occur in similar facies reflecting a perennial lake setting. Increased energy is indicated by the erosive nature of the runnels and their coarse fill of fragmented stromatolite material. The ridges recognized are up to 0.8 m wide, are sediment cored, display up to 0.2 m relief and are separated by runnels up to 0.3 m wide. The coating stromatolites are similar to those described from the MORS examples and thin into the runnels. The runnel fill is commonly composed of an edgewise breccia of stromatolite fragments or laminated dolomitic mudstones. In some instances re-activation of the ridge and runnel systems results in the more resistant edgewise breccias, which previously filled the runnels, forming the core of the ridges.
Within the Edderfugledal Member, sediment-cored stromatolite mounds form features with up to 0.4 m of relief (Fig. 8c) which are often fringed by breccia aprons consisting of microbialite fragments, ooids and oncoids. The sediment cores commonly contain bedding which is truncated towards the stromatolite covering. In contrast to the MORS examples, the sediment cores are composed of bedded calcareous mudstone and the microbialites are more laminar with only minor hemispheroidal development (Fig. 8c). However, shore zone facies are found associated with these stromatolite forms, a feature consistent with their generation in a high-energy setting. The stromatolites themselves are largely composed of smooth carbonate laminations 0.5–4 mm thick, with occasional examples containing quartz grains and gypsum.
The broad similarity of the forms and occurrence of stromatolites observed in the Edderfugledal Member to those described from the MORS of Scotland suggests that this range of structures and the controls on their development may be expected in similar lacustrine settings.
7. Discussion
It is widely accepted that the form of microbialite accumulations reflects the influence of the physical environment and microbial activity (Logan, Rezak & Ginsberg, Reference Logan, Rezak and Ginsburg1964; Paul & Peryt, Reference Paul and Peryt2000; Allwood et al. Reference Allwood, Walter, Burch and Kamber2007). Logan, Rezak & Ginsberg (Reference Logan, Rezak and Ginsburg1964) recognized the importance of sedimentation, erosion, regularity of wetting and desiccation and the sediment cohesion, resulting from microbialite growth, as important factors in controlling microbialite development. The strong influence of remnant topography on palaeoenvironments in the development of the Archaean Strelley Pool Chert stromatolites in Western Australia was described by Allwood et al. (Reference Allwood, Walter, Burch and Kamber2007). However, although the stromatolite facies associations presented in this example show environmental control, within the individual facies associations rapid lateral changes are difficult to account for through purely environmental controls, suggesting a significant biological role. Only subtle traces of the original algae/bacteria are recognized within the examples studied here, in the form of faint vertical filaments up to 100 μm wide and 500 μm in length preserved within the micritic component of the microbial lamination (Fig. 3a), restricting investigation of biological controls. Furthermore, most of the variation in the broad range of macro forms recognized can be accounted for by environmental factors.
The absence of a wide range of microbialite forms in modern lakes (Moore, Knott & Stanley, Reference Moore, Knott and Stanley1984; Kazmierczak & Kempe, Reference Kazmierczak and Kempe2006; Gischler, Gibson & Oschmann, Reference Gischler, Gibson and Oschmann2008) limits comparisons with those forms described from the Orcadian Basin. Therefore, the widely studied Shark Bay microbialites (Davies, Reference Davies, Logan, Davies, Read and Cebulski1970; Hoffman, Reference Hoffman and Walter1976; Logan, Reference Logan1981; Riding, Reference Riding2000; Reid et al. Reference Reid, James, Macintyre, Dupraz and Burne2003) are incorporated alongside examples from the Great Salt Lake, southwest USA (Carozzi, Reference Carozzi1962), to aid the construction of a model for describing the distribution of the lacustrine forms documented here. Although the Orcadian lake was not tidal, small-scale fluctuations in lake level caused by climatic and short-term meteorological variance would have led to areas of repeated exposure similar to an intertidal zone.
Hoffman (Reference Hoffman and Walter1976) recognized significant variation in the form of the microbial accumulations of Shark Bay, which he attributed to variations in the intensity of wave attack. Stratiform mats are well developed in lower energy embayments, with nodular forms present in slightly higher energy bights and columnar forms developed on the exposed headlands. The physiography and prevailing climatic conditions in which the microbialites accumulate exert the primary controls on the energy gradient of the environment and therefore the distribution of the various microbialite forms. Hoffman (Reference Hoffman and Walter1976) attributed variations in the internal structure of the microbial accumulations to the regularity of exposure, which controlled the microbes present within the stromatolites. Within the upper intertidal zone, pustular mats were prevalent and therefore microbial accumulations have an irregular, poorly laminated/massive texture; however, in the lower intertidal zone, smooth mat textures are developed which when involved in columnar accumulations result in simple convex laminae. The sublittoral microbial accumulations are characterized by colloform developments.
The environmental interpretation of the microbialite forms identified has allowed the construction of a palaeoenvironmental model (Fig. 11). The stromatolite sheets described here are interpreted as mat deposits which formed in low-energy environments with limited erosion such as embayments (Fig. 11), similar to those described from Shark Bay (Hoffman, Reference Hoffman and Walter1976). Sedimentary structures associated with these forms are consistent with a low-energy environment. Periodic exposure and formation of gas through biodegradation resulted in the minor disruption of the mats and generation of pustular textures. Proto-oncoids are interpreted as reworked fragments of the stromatolite sheets. The formation of domal mounds reflects preferential growth on elevated areas, generated through erosive processes or pustule formation, and therefore represent increased energy and a more exposed, lakeward position of formation. Carozzi (Reference Carozzi1962) noted a transition from isolated mounds to ridges and runnels in proximal to distal positions within the Great Salt Lake, Utah. The clearly erosional origin of the aligned mounds and runnels described here suggests that a similar interpretation can be made (Fig. 11).

Figure 11. Palaeoenvironmental reconstruction, illustrating the relative positioning of the various forms of stromatolite accumulations from the Lower Stromness Flagstone Group, with reference to the lake shore.
The coarse-grained nature of the mantling breccia aprons present around the sand-cored stromatolite mounds and reefal build-ups is indicative of further increased energy in the environment of formation as are the associated facies. The high-relief nature of these forms is interpreted as indicative of an exposed headland setting for formation. Further evidence for such a setting is the proximity of these forms to a basement high (≈ 15 m). The poorly developed internal structure of the reefal stromatolite build-ups can be compared to those from intertidal zones in Shark Bay described by Hoffman (Reference Hoffman and Walter1976), resulting from episodic exposure, and therefore are interpreted to reflect formation in a shallower, more ephemeral setting. Hoffman (Reference Hoffman and Walter1976) also noted that better developed laterally linked hemispheroids are commonly a feature associated with a lack of exposure and therefore the sand-cored stromatolite mounds are interpreted as forming in deeper, or more perennial lacustrine settings (Fig. 11). This is further evidenced by the upward transition to deep lake facies above the occurrence described.
Interestingly, Fannin (Reference Fannin1969) only recorded the larger scale, sand-cored and reefal, microbialite accumulations from the Point of Ness, and no further examples have been identified in Orkney. Given the dependence of the Shark Bay examples on their position relative to embayments and headlands, it may be that the palaeorelief imposed by the basement produced conditions suitable for the wide variety of microbialite forms identified. Following continued sedimentation, the inherited basin margin relief may have become subdued to the point that fewer niches were available for the development of such varied stromatolite forms. Prior to the development of the suite of forms described above, clast-coating stromatolites formed on exposed sediment-starved, basin coincident, lake margins.
8. Conclusions
The most significant controls on the form of the stromatolite accumulations described here are erosion and sedimentation, which are primarily a function of water depth, which is in turn controlled by climatic and tectonic factors. Feedbacks occur through sediment cohesion, generated by microbialite growth, and the related focus of erosional currents.
We describe eight distinct microbialite forms and the environmental controls on their formation. The identification of forms characteristic of low-energy, sheltered settings to those of higher energy exposed settings and their spatial distribution has provided the basis for a palaeoenvironmental model for the formation of the stromatolites identified in the MORS of Northern Scotland. A closely comparable suite of stromatolite forms from the Late Triassic of East Greenland and modern examples is consistent with this model. We suggest that this palaeoenvironmental model is widely applicable to stromatolitic accumulations in similar lacustrine settings through large portions of the Phanerozoic.
Acknowledgements
Fieldwork undertaken by SDA was supported by the Inverness Field Club and the Carnegie Trust. Triassic and modern microbialites described from East Greenland were examined while undertaking fieldwork with CASP. NHT visited Shark Bay many years ago under the expert guidance of Brian Logan. We thank Robert Riding and Susan Marriott for constructive and helpful reviews of the original manuscript.