Introduction
People with an autism spectrum disorder (ASD) have difficulties in three core areas, namely social reciprocity, communication and repetitive behaviours or intense preoccupations. Within the spectrum, those affected have a large range of intellectual abilities. People with a normal intelligence quotient (IQ) can be further classified as having Asperger's syndrome (ASP) or high-functioning autism (HFA). Children who have a delay in acquisition of phrase speech have HFA, while those who use phrases before 36 months may have ASP (Gilchrist et al. Reference Gilchrist, Green, Cox, Burton, Rutter and Le Couteur2001; Howlin, Reference Howlin2003). However, there is considerable debate over whether ASP is distinct from HFA (Rinehart et al. Reference Rinehart, Bradshaw, Tonge, Brereton and Bellgrove2002b; Klin & Volkmar, Reference Klin and Volkmar2003).
Using a history of phrase speech acquisition as a marker to distinguish HFA and ASP, we recently reported that distinct patterns of grey-matter abnormalities characterized these subgroups. The HFA and ASP groups had similar impairment in the three core symptom domains mentioned above, but compared with controls, children with HFA had greater left-sided frontal lobe grey-matter deficits than children with ASP; children with ASP had less grey matter in the caudate and thalamus (McAlonan et al. Reference McAlonan, Suckling, Wong, Cheung, Lienenkaemper, Cheung and Chua2008). This finding suggested that maldevelopment of different components of cortico-striatal loop systems may give rise to features of ASP and HFA.
Evidence that autism may involve disruption at a brain-systems level is compelling. We have shown that distributed grey-matter volume abnormalities are associated with a disruption in volumetric correlations across fronto-limbic-striatal and cerebellar systems (McAlonan et al. Reference McAlonan, Cheung, Cheung, Suckling, Lam, Tai, Yip, Murphy and Chua2005). Numerous functional imaging studies show that regional brain activity is desynchronized in autism (Horwitz et al. Reference Horwitz, Rumsey, Grady and Rapoport1988; Just et al. Reference Just, Cherkassky, Keller and Minshew2004, Reference Just, Cherkassky, Keller, Kana and Minshew2007; Koshino et al. Reference Koshino, Carpenter, Minshew, Cherkassky, Keller and Just2005) and these observations are consistent with convincing evidence for anomalous postnatal white-matter development in autism (Herbert et al. Reference Herbert, Ziegler, Makris, Filipek, Kemper, Normandin, Sanders, Kennedy and Caviness2004; Herbert, Reference Herbert2005, Ben Bashat et al. Reference Ben Bashat, Kronfeld-Duenias, Zachor, Ekstein, Hendler, Tarrasch, Even, Levy and Ben Sira2007). Following dramatic brain expansion in very young children with autism (Courchesne, Reference Courchesne2002, Reference Courchesne2004; Courchesne et al. Reference Courchesne, Carper and Akshoomoff2003), we and others have shown that white-matter volume and diffusion tensor imaging indices are significantly lower in older children and adults with autism compared with typically developing controls (McAlonan et al. Reference McAlonan, Daly, Kumari, Critchley, van Amelsvoort, Suckling, Simmons, Sigmundsson, Greenwood, Russell, Schmitz, Happe, Howlin and Murphy2002, Reference McAlonan, Cheung, Cheung, Suckling, Lam, Tai, Yip, Murphy and Chua2005; Barnea-Goraly et al. Reference Barnea-Goraly, Kwon, Menon, Eliez, Lotspeich and Reiss2004; Waiter et al. Reference Waiter, Williams, Murray, Gilchrist, Perrett and Whiten2005; Keller et al. Reference Keller, Kana and Just2007; Sundaram et al. Reference Sundaram, Kumar, Makki, Behen, Chugani and Chugani2008; Cheung et al. Reference Cheung, Chua, Cheung, Khong, Tai, Wong, Ho and McAlonanin press) but whether HFA and ASP affect white matter differently has not been examined.
Therefore we planned a voxel-based study of white-matter volume in ASP and HFA. Based on our previous study of grey-matter abnormalities in ASP and HFA (McAlonan et al. Reference McAlonan, Suckling, Wong, Cheung, Lienenkaemper, Cheung and Chua2008) we hypothesized that similar discrete patterns of abnormalities in white matter would distinguish in children with ASP from those with HFA. Specifically we predicted left-sided frontal abnormalities would predominantly affect the HFA group.
Method
Participants
Ninety-one, right-handed children aged 6–16 years with an IQ>70 (estimated using the vocabulary subset of the Wechsler Intelligence Scale for Children) participated in the study. Grey-matter volumetric analysis of data from 88 of these children has already been reported (McAlonan et al. Reference McAlonan, Suckling, Wong, Cheung, Lienenkaemper, Cheung and Chua2008). Exclusion criteria were co-morbid psychiatric (e.g. mood disorder or attention deficit hyperactivity disorder) or medical conditions (e.g. epilepsy) requiring intervention, history of head injury, or genetic disorder associated with autism (e.g. tuberous sclerosis or fragile X syndrome). Thirty-six were non-medicated children with an independent clinical diagnosis of ASD. Following the same definition as previous neuroimaging studies (Kwon et al. Reference Kwon, Ow, Pedatella, Lotspeich and Reiss2004; Lotspeich et al. Reference Lotspeich, Kwon, Schumann, Fryer, Goodlin-Jones, Buonocore, Lammers, Amaral and Reiss2004; McAlonan et al. Reference McAlonan, Suckling, Wong, Cheung, Lienenkaemper, Cheung and Chua2008), 18 children (three females) with phrase speech before the age of 36 months comprised the ASP group. Eighteen (three females) with a history of delayed phrase speech formed the HFA group. As shown in Table 1 the children did not differ in diagnostic algorithm scores of the Autism Diagnostic Interview, Revised (ADI-R; Western Psychological Services, Los Angeles, CA, USA). Fifty-five typically developing control children (eight females) were recruited from local schools and screened for major psychiatric illness using the Diagnostic Interview Schedule for Children for DSM-IV. They did not differ in mean age or verbal IQ (see Table 1), maternal language or ethnicity from the autism spectrum group. Every child's parent gave informed consent for the protocol approved by the University of Hong Kong Faculty of Medicine Research Ethics Committee, and each child gave their assent.
Table 1. Group characteristics

s.d., Standard deviation; ADIA, social interaction subscale of the Autism Diagnostic Interview, Revised (ADI-R; Western Psychological Services, Los Angeles, CA, USA); ASP, Asperger's syndrome (n=18); HFA, high-functioning autism (n=18); ADIB, communication subscale of the ADI-R; ADIC, repetitive behaviours subscale of the ADI-R; ASD, autism spectrum disorder, combined group (n=36); VIQ, pro-rated verbal intelligence quotient.
Magnetic resonance imaging and analysis
An interleaved dual-echo fast-spin echo (FSE) sequence [repetition time=3000 ms, echo times, TE1=20 ms (proton density weighted images) and TE2=100 ms (T2-weighted images)] was used to collect whole-brain data on a GE Signa 1.5 T system (General Electric, Milwaukee, WI, USA). Images were aligned to the anterior commissure–posterior commissure (AC–PC) line, with contiguous slices 0.859 mm in-plane and 3 mm thick. Each scan was screened by a consultant radiologist to exclude clinically significant abnormalities. Extracerebral tissues were removed (Suckling et al. Reference Suckling, Brammer, Lingford-Hughes and Bullmore1999a) and brain tissue segmented into grey and white matter, cerebrospinal fluid and a fourth class including dura, vessels and other extraneous tissues (Suckling et al. Reference Suckling, Sigmundsson, Greenwood and Bullmore1999b). The current protocol yielded superior grey/white differentiation compared with high-resolution T1 scans, as demonstrated previously (see fig. 1 a in Chua et al. Reference Chua, Cheung, Cheung, Tsang, Chen, Wong, Cheung, Yip, Tai, Suckling and McAlonan2007). The white-matter images were mapped into the standard space of Talairach & Tornoux (Reference Talairach and Tournoux1988) by minimizing the sum-of-square intensity difference of each proton density image to a group-specific template image (McAlonan et al. Reference McAlonan, Cheung, Cheung, Suckling, Lam, Tai, Yip, Murphy and Chua2005) and smoothed with a 4.4 mm kernel. The main effect of diagnostic group was estimated at each voxel by regression of a general linear model using BAMM software (Brain Analysis Morphological Mapping version 2.5; University of Cambridge, Cambridge, UK; http://www-bmu.psychiatry.cam.ac.uk/BAMM/index.html) on a SPARC workstation (Sun Microsystems Europe Inc., Camberley, Surrey, UK) as previously described (McAlonan et al. Reference McAlonan, Daly, Kumari, Critchley, van Amelsvoort, Suckling, Simmons, Sigmundsson, Greenwood, Russell, Schmitz, Happe, Howlin and Murphy2002, Reference McAlonan, Cheung, Cheung, Suckling, Lam, Tai, Yip, Murphy and Chua2005, Reference McAlonan, Cheung, Cheung, Chua, Murphy, Suckling, Tai, Yip, Leung and Ho2007; Chua et al. Reference Chua, Cheung, Cheung, Tsang, Chen, Wong, Cheung, Yip, Tai, Suckling and McAlonan2007). The between-group structural differences (spatial extent statistics) at each intracerebral voxel were assessed using non-parametric methods. This involved randomly reassigning group membership to generate 10 permutated white-matter maps, thereby sampling the null hypothesis that group differences occur by chance (Bullmore et al. Reference Bullmore, Suckling, Overmeyer, Rabe-Hesketh, Taylor and Brammer1999). The statistical thresholds were corrected for multiple comparisons by controlling the ‘family-wise error rate’ and results reported where the number of false-positive clusters (FPC) expected under the null-hypothesis <1.
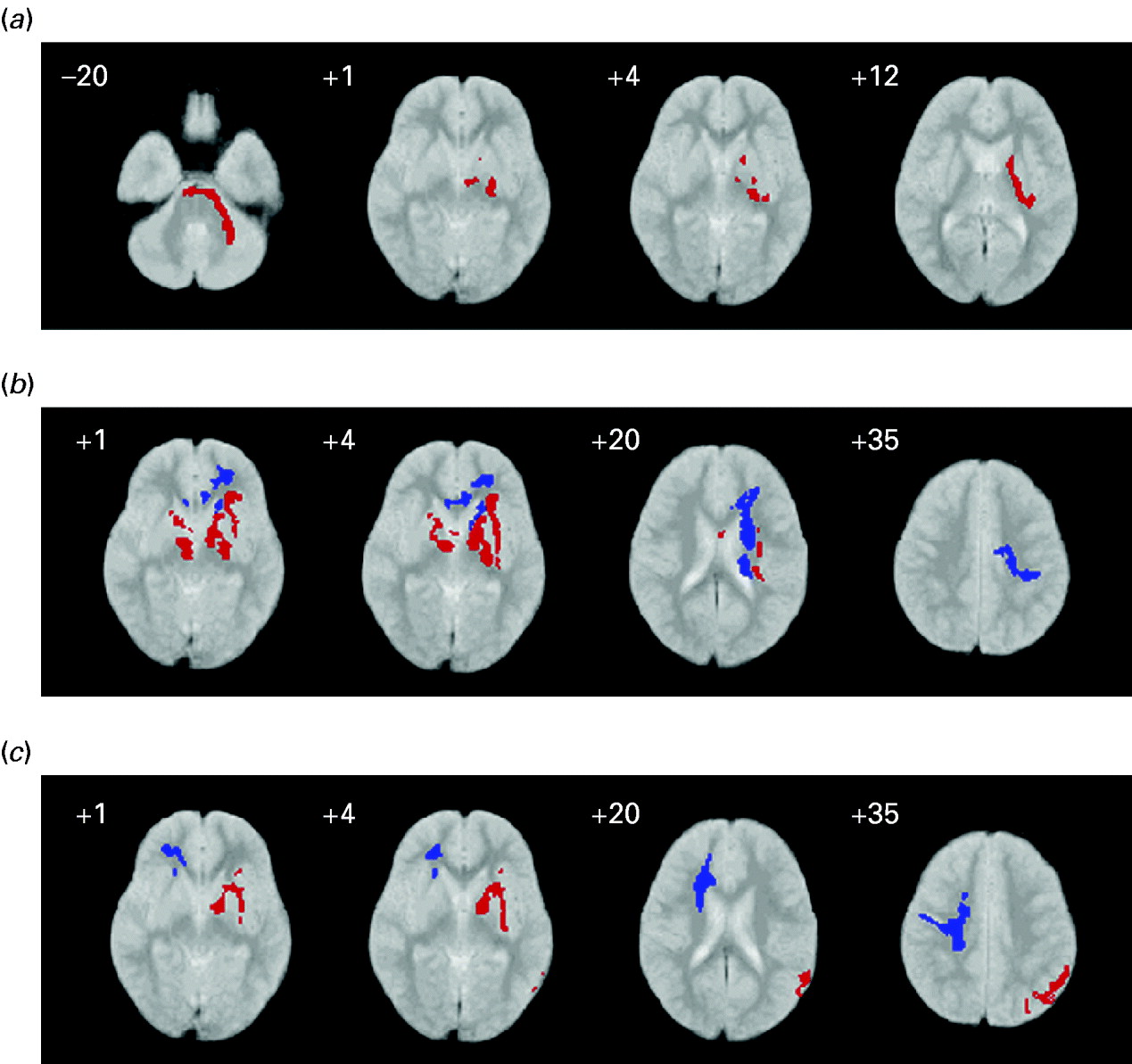
Fig. 1. White-matter volume in children with autism spectrum compared with controls: (a) high-functioning autism (HFA) relative to Asperger's syndrome (ASP); (b) HFA relative to controls; (c) ASP relative to controls. Red indicates relative volume excess; blue indicates relative volume deficit. The right side of the brain is shown on the left side of each panel. The z coordinate for each axial slice in standard space (Talairach & Tournoux, Reference Talairach and Tournoux1988) is given in mm.
There were three contrasts: (1) HFA and ASP; (2) HFA and controls; (3) ASP and controls.
Regional white-matter volumes in any of the autism spectrum groups were defined as greater or less, relative to the control group. White-matter volumes in HFA were described relative to ASP. The effect size of each contrast was examined using partial η2 measures in spss (version 16.0; SPSS Inc., Chicago, IL, USA).
Global white-matter volumes were not different across groups: control 463.8 (s.d.=19.8) ml; ASP 459.0 (s.d.=28.6) ml; HFA 465.2 (s.d.=19.7) ml.
Regional white-matter volumes
HFA compared with ASP
Compared with ASP, children with HFA had significantly greater white-matter volume around the left basal ganglia (internal and external capsules), thalamus and cerebellum (see Fig. 1 and Table 2).
Table 2. White-matter differences in autism spectrum

s.d., Standard deviation; ASP, Asperger's syndrome; HFA, high-functioning autism; FOF, fronto-orbital fasciculus.
a A sample Talaraich coordinate (x, y, z) is given for the approximate centre of each cluster. The three-dimensional clusters are not confined to these areas, nor are they all encompassing.
HFA compared with control
Children with HFA also had significantly more white matter than controls in bilateral subcortical circuits including the internal and external capsule. However, they had significantly less white matter in left frontal pathways corresponding to the orbitofrontal fasiculus and extending to the genu of the corpus callosum and basal ganglia. The lower the volume of white matter in the left frontal lobe, the older the age in months that the child with HFA acquired phrase speech, although this relationship did not reach significance (Spearman's ρ=−0.34, p=0.09) (see Fig. 1 and Table 2).
ASP compared with control
Children with ASP had significantly more white matter in subcortical circuits and the parietal lobe of the left hemisphere when compared with controls. However, they had significantly less white matter in the right frontal lobe than controls especially around the orbitofrontal fasiculus and right genu of the corpus callosum (see Fig. 1 and Table 2).
Discussion
Our main finding was that intellectually able children with ASDs subgrouped on the basis of their history of language acquisition had distinct patterns of white-matter abnormalities. Children with ASP had predominantly right-sided white-matter deficits compared with controls. Children with HFA had greater white-matter deficits in the left hemisphere. Both groups had larger white-matter volumes in deep white-matter regions around the basal ganglia regions compared with controls. These white-matter volumes were significantly greater in HFA than ASP. These findings extend our previous study of grey matter in ASP and HFA (McAlonan et al. Reference McAlonan, Suckling, Wong, Cheung, Lienenkaemper, Cheung and Chua2008) and carry the important implication that the autism spectrum maps onto a spectrum of brain abnormalities.
Total white-matter tissue volumes were not significantly different in the autism spectrum groups or controls. This may be initially surprising given that brain volumes are dramatically larger in autism in early life (Carper et al. Reference Carper, Moses, Tigue and Courchesne2002; Courchesne, Reference Courchesne2002, Reference Courchesne2004; Hazlett et al. Reference Hazlett, Poe, Gerig, Smith, Provenzale, Ross, Gilmore and Piven2005). Larger cerebral white-matter volumes in children with autism persist through age 7–11 years (Herbert et al. Reference Herbert, Ziegler, Deutsch, O'Brien, Lange, Bakardjiev, Hodgson, Adrien, Steele, Makris, Kennedy, Harris and Caviness2003), especially in radiate white matter (Herbert et al. Reference Herbert, Ziegler, Makris, Filipek, Kemper, Normandin, Sanders, Kennedy and Caviness2004). With increasing age, these volume increases abate (Courchesne, Reference Courchesne2004; Herbert, Reference Herbert2005). The participants in our study were aged up to 16 years and this older age group may explain why we detected only regional differences, not global volume differences in those with autism. Our findings implicate pathways corresponding to deeper white-matter tracts such as the fronto-occipital fasciculus and internal capsule in autism spectrum. These systems have recently been shown to continue to mature well into late adolescence and young adulthood (Lebel et al. Reference Lebel, Walker, Leemans, Phillips and Beaulieu2008), and this slower maturation may make such tracts vulnerable to damage for a longer period. However, the distinctive involvement of the left and right hemisphere in HFA and ASP, respectively, indicates that the developmental pressures on white matter in subgroups of children in autism spectrum may not fully coincide.
White-matter deficits in the corpus callosum have previously been reported in autism. For example, young children with ASD have disproportionately smaller corpus callosum volumes than typically developing controls (Boger-Megiddo et al. Reference Boger-Megiddo, Shaw, Friedman, Sparks, Artru, Giedd, Dawson and Dager2006), although in older groups the deficits may be more posterior (Egaas et al. Reference Egaas, Courchesne and Saitoh1995; Waiter et al. Reference Waiter, Williams, Murray, Gilchrist, Perrett and Whiten2005). Still others have reported that the size of the genu of the corpus callosum is highly correlated with the extent of fronto-parietal asynchrony during executive function tasks (Just et al. Reference Just, Cherkassky, Keller, Kana and Minshew2007). In general, the literature is not completely clear (for a review, see Brambilla et al. Reference Brambilla, Hardan, di Nemi, Perez, Soares and Barale2003). The current study indicates that the corpus callosum findings depend upon the nature of the ASD samples examined, as we found that lower corpus callosum volumes were predominantly right-sided in HFA and left-sided in ASP.
In frontal regions also, children with ASP had mainly right-sided deficits; those with HFA had left-sided deficits. We found the same pattern when we examined grey-matter differences in many of these children in an earlier study; the HFA group had left-sided frontal grey-matter deficits relative to controls (McAlonan et al. Reference McAlonan, Suckling, Wong, Cheung, Lienenkaemper, Cheung and Chua2008). In that study the extent of grey matter decrease in the HFA group was correlated with their delay in language acquisition. In the current study the correlation between age (in months) of phrase language acquisition in the HFA group and white-matter volume in the left frontal lobe was less striking, but consistent. This backs the possibility that brain structure in autism at least partly reflects the developmental language difference distinguishing subgroups.
Evidence from other sources echoes this relationship between language ability and brain lateralization in autism spectrum. Right-sided asymmetries in children with developmental language delay or autism have been recorded (Herbert et al. Reference Herbert, Harris, Adrien, Ziegler, Makris, Kennedy, Lange, Chabris, Bakardjiev, Hodgson, Takeoka, Tager-Flusberg and Caviness2002, Reference Herbert, Ziegler, Deutsch, O'Brien, Kennedy, Filipek, Bakardjiev, Hodgson, Takeoka, Makris and Caviness2005) and children with autism who have severe language impairment have a significant decrease in serotonin synthesis in the left hemisphere relative to the right (Chandana et al. Reference Chandana, Behen, Juhasz, Muzik, Rothermel, Mangner, Chakraborty, Chugani and Chugani2005). Similarly, in language-impaired children with autism the normal asymmetry in Broca's area (left inferior frontal lobe) is reversed – i.e. right frontal lobe volumes are greater than left (De Fosse et al. Reference De Fosse, Hodge, Makris, Kennedy, Caviness, McGrath, Steele, Ziegler, Herbert, Frazier, Tager-Flusberg and Harris2004). Additional support for distinct patterns of lateralization comes from a careful dissection of executive function performance in HFA and ASP, showing left-sided abnormalities in HFA (Rinehart et al. Reference Rinehart, Bradshaw, Brereton and Tonge2002a). In contrast, the social interaction and motor symptoms of ASP have been compared with ‘non-verbal language disorder’, which is thought to arise from right-hemisphere dysfunction (Gunter et al. Reference Gunter, Ghaziuddin and Ellis2002). In 1999, Ellis & Gunter hypothesized that this apparent right-hemispheric dysfunction seen in ASP could be a disorder of white-matter development (Ellis & Gunter, Reference Ellis and Gunter1999). The results from the present study appear to agree with their prediction, but because we did not examine behavioural features outside the diagnostic triad, this interpretation of our results is tentative.
In our study of grey-matter differences in these children, we observed that both ASP and HFA groups had significant abnormalities in white matter associated with the basal ganglia and thalamus (McAlonan et al. Reference McAlonan, Suckling, Wong, Cheung, Lienenkaemper, Cheung and Chua2008). Basal ganglia pathways carry afferent information from the entire cerebral cortex for integration and output to motor and thalamic targets (Utter & Basso, Reference Utter and Basso2008). Of particular relevance to autism are limbic circuits through the basal ganglia and thalamus which interconnect social brain areas strongly associated with autism, including the amygdala and fusiform gyrus (Baron-Cohen et al. Reference Baron-Cohen, Ring, Bullmore, Wheelwright, Ashwin and Williams2000; Schultz, Reference Schultz2005), superior temporal sulcus (Zilbovicius et al. Reference Zilbovicius, Meresse, Chabane, Brunelle, Samson and Boddaert2006), together with the medial prefrontal lobe (Happe et al. Reference Happe, Ehlers, Fletcher, Frith, Johansson, Gillberg, Dolan, Frackowiak and Frith1996; Castelli et al. Reference Castelli, Frith, Happe and Frith2002). The concentration of white-matter abnormalities noted fits with a functional disturbance of basal ganglia–thalamo–cortical loop systems which coordinate social behaviours in autism. Moreover, since the basal ganglia are a major motor output structure, the incidence of neuromotor symptoms in ASD (Vilensky et al. Reference Vilensky, Damasio and Maurer1981; Rinehart et al. Reference Rinehart, Tonge, Bradshaw, Iansek, Enticott and Johnson2006b; Freitag et al. Reference Freitag, Kleser, Schneider and von Gontard2007, Loh et al. Reference Loh, Soman, Brian, Bryson, Roberts, Szatmari, Smith and Zwaigenbaum2007) also fits with theories of pathology in these circuits (Damasio & Maurer, Reference Damasio and Maurer1978; Vilensky et al. Reference Vilensky, Damasio and Maurer1981).
The extent of basal ganglia abnormalities was greatest in the HFA group. Children with HFA also had significantly more white matter than ASP in the left cerebellum. Given the key role of the basal ganglia as a limbic–motor interface (Alexander et al. Reference Alexander, Crutcher and DeLong1990; Utter & Basso, Reference Utter and Basso2008), and its connections with the cerebellum, we speculate that these anatomical differences may have something to do with the distinct motor output phenomena ascribed to each group. Clumsiness may be a feature of ASP, while children with autism may have postural abnormalities (Rinehart et al. Reference Rinehart, Bellgrove, Tonge, Brereton, Howells-Rankin and Bradshaw2006a, c). In a fractionation of movement planning and execution, Rinehart's group reported a quantitatively greater impairment in motor preparation in children with HFA compared with those with ASP. The authors suggested that the particular motor phenotypes in HFA and ASP could represent a downstream effect of this ‘quantitative dissociation’ in motor planning (Rinehart et al. Reference Rinehart, Bellgrove, Tonge, Brereton, Howells-Rankin and Bradshaw2006a).
In our study, children with ASP had greater white-matter volumes than controls around the inferior parietal lobule in the left hemisphere. Recently Nordahl et al. completed an elegant study of cortical folding abnormalities in autism spectrum (Nordahl et al. Reference Nordahl, Dierker, Mostafavi, Schumann, Rivera, Amaral and Van Essen2007). They reported that children with ASP had the greatest sulcal depth differences from typically developing controls in the left intraparietal sulcus of children (Nordahl et al. Reference Nordahl, Simon, Zierhut, Solomon, Rogers and Amaral2008). This convergence of morphometric abnormalities to the left parietal lobe in two distinct structural studies is striking. Moreover, in a functional magnetic resonance imaging study of working memory, Koshino et al. (Reference Koshino, Carpenter, Minshew, Cherkassky, Keller and Just2005) noted that individuals with autism showed greater activation in the left extrastriate cortex, near the cluster of higher white-matter volume observed here in the ASP group (Koshino et al. Reference Koshino, Carpenter, Minshew, Cherkassky, Keller and Just2005). The authors interpreted this spurious posterior activation in ASD as due to a greater reliance on lower-order visual analysis (Koshino et al. Reference Koshino, Carpenter, Minshew, Cherkassky, Keller and Just2005). Although the ASD sample in their study was described as high functioning, the language history of the participants was not explicitly determined. It is feasible that this complementary collection of data points to some compensatory reorganization of white matter in the left parietal lobe in ASP, but this idea needs much further investigation.
An important limitation of our study was that we did not directly examine how white-matter abnormalities relate to the behavioural features of individuals with HFA and ASP outside the diagnostic triad (e.g. motor symptoms, executive functioning, etc). However, a key feature of our study was that HFA and ASP groups had no difference in their diagnostic scores on the ADI-R. Thus, the differences in white matter that we observed, taking history of language acquisition as the only discriminative marker, are quite dramatic. We would emphasize that language was solely used as a discriminatory marker to define subgroups in our study. We do not believe that the history of language acquisition can fully explain the results. It is much more likely that the white-matter systems involved in HFA and ASP are associated with a constellation of features, including executive function, motor skills and language which potentially separate HFA from ASP.
Another limitation of our study was that subdividing the ASD sample lowered numbers and hence statistical power. However, we observed sizable effect sizes in each subgroup contrast, and take this to indicate that more homogeneous subject groupings may be key to a better understanding of the autism spectrum. In focusing on HFA and ASP we excluded the majority of children with autism who have learning disability. Therefore we cannot extrapolate our findings more generally. It will be important to consider to what extent the brain determinants of autistic children with low IQ are distinct from these intellectually able samples. In this investigation we included children and adolescents only. Of note, we conducted a study of adults with ASP some years ago (McAlonan et al. Reference McAlonan, Daly, Kumari, Critchley, van Amelsvoort, Suckling, Simmons, Sigmundsson, Greenwood, Russell, Schmitz, Happe, Howlin and Murphy2002). In that study we found white-matter excesses in the left basal ganglia similar to those reported here. This suggests that some of the abnormalities present in childhood are persistent and could therefore contribute to the pervasive nature of ASD. The plan is to follow-up the present cohort to determine whether there are longitudinal differences in ageing in ASP and HFA.
In conclusion, we used the history of language acquisition as a marker for HFA and ASP. We found that ASDs have heterogeneous effects on white-matter systems. This has important implications for the causal mechanisms underlying HFA and ASP. The present study, along with our previous assessment of grey-matter abnormalities in ASP and HFA, indicates that the autism spectrum cannot be described along a single dimension of severity. We believe our work should encourage further research into the heterogeneous nature of autism, focusing as much on individual differences as shared pathologies.
Acknowledgements
The autism research programme in the Department of Psychiatry, University of Hong Kong is supported by a donation from ING Asia/Pacific and a University of Hong Kong grant. We thank Ms Michelle Deng for her help with image preparation.
Declaration of Interest
None.