The degree of heat treatment of raw milk affects the texture of the corresponding acid milk gels, and it positively correlates with the ease of formation and gel strength. When milk is heated at ≥80°C, α-lactalbumin (α-La) and β-lactoglobulin (β-Lg) of the whey protein (WP) form disulfide (SS) bonds with κ-casein on the surface of casein micelles to yield κ-casein–WP complexes that subsequently dissociate from these micelles (Guyomarc'h et al., Reference Guyomarc'h, Dalgleish and Law2003, Reference Guyomarc'h, Renan, Chatriot, Gamerre and Famelart2007, Reference Guyomarc'h, Violleau, Surel and Famelart2010; Vasbinder et al., Reference Vasbinder, Alting and de Kruif2003) and then interact with them to enhance acid coagulation (Donato et al., Reference Donato, Alexander and Dalgleish2007; Morand et al., Reference Morand, Guyomarc'h and Famelart2011). In contrast, the dissociation of κ-casein from casein micelles lowers their ζ-potential and increases their surface hydrophobicity, thus increasing the strength of the curd and that of the interactions between micelles (Oka et al., Reference Oka, Ono, Ohara, Noguchi and Takano2018). Notably, the strength of acid milk gels increases when milk is heated in the presence of WP (Cheng et al., Reference Cheng, Augustin and Clarke2000; Sodini et al., Reference Sodini, Mattas and Tong2006; Delikanli and Ozcan, Reference Delikanli and Ozcan2014) and is influenced by the extent of κ-casein dissociation (Mohomud et al., Reference Mohomud, Katsuno and Nishizu2017).
WP is obtained as a by-product of cheese production after numerous steps including membrane treatment, heat sterilization, and spray drying; these steps affect the functional properties of the by-product (Morr and Foegeding, Reference Morr and Foegeding1990; Huffman, Reference Huffman1996), with the strongest effect exerted by thermal denaturation (Hollar et al., Reference Hollar, Parris and Cockley1995). Heating of aqueous WP solutions yields gels (Hiller and Cheeseman, Reference Hiller and Cheeseman1979) due to thermal denaturation of WP because dry heat sterilization affects heat-induced gelation (Mangino et al., Reference Mangino, Liao, Harper, Morr and Zadow1987). Upon heating, α-La and β-Lg or β-Lg and β-Lg form SS bonds to produce aggregates (Hollar et al., Reference Hollar, Parris and Cockley1995; Ikeuchi et al., Reference Ikeuchi, Kato, Sugai, Fujino and Kuwajima1998; Schokker et al., Reference Schokker, Singh and Creamer2000) that are assumed to affect the heat-induced gelation of WP. Kinekawa and Kitabatake (Reference Kinekawa and Kitabatake1995) reported that heat-denatured WP soluble aggregates form more elastic gels than non-denatured WP. On the contrary, dry-heat-treated WP forms weaker gels than non-treated WP (Rector et al., Reference Rector, Matsudomi and Kinsella1991). This suggests that the thermal denaturation of WP may also affect the formation of acid milk gels. Karam et al. (Reference Karam, Hosri, Hussain, Barbar, Gaian and Scher2017) reported that the dry denaturation of WP during storage affects the characteristics of the corresponding acid milk gels and that acid milk gels prepared by adding dry-heat-denatured (103°C for 2 h) WP to milk exhibit a lower gelation rate and strength than those prepared from non-denatured WP. In contrast, Vasbinder et al. (Reference Vasbinder, Valde and de Kruif2004) reported that WP-soluble aggregates heated at 68°C for 2 h increased the strength of acid milk gel and play an important role in gel properties. Several previous studies report on the relationship between the properties of WP added to milk and the strength of acid milk gel. However, few studies have explored the effect of heat treatment after adding WP to milk. As previously stated, Cheng et al. (Reference Cheng, Augustin and Clarke2000) investigated the effect of WP addition during heating, but there are no known experiments on the differing denaturation degree of WP.
Therefore, in this study, the effect of adding WP with different degrees of denaturation to milk on the strength of acid milk gel was investigated. In our previous studies, we clarified the influence of κ-casein dissociation on the strength of acid milk gels. Following on form this, here the relationships between the dissociation of κ-casein from casein micelles and the strength of the acid milk gels due to the addition of denatured WP are further investigated.
Materials and methods
Materials
Unmodified milk, that is, raw milk obtained from Holstein cows raised in the Tokyo University of Agriculture Fuji Farm (Fujinomiya City, Shizuoka Prefecture), was used. The milk fat was separated and removed using a cream separator at 40°C to prepare undenatured skimmed milk. Fonterra Co-operative Group Ltd. (New Zealand) product WPI895 (protein 93.9 wt%, fat 0.3 wt%, carbohydrate 0.4 wt%, minerals 1.5 wt%) was used as the whey protein isolate (WPI), and κ-casein (≥70%, lyophilized powder) isolated from bovine milk (Sigma-Aldrich, St. Louis, MO) was employed for the calibration curve.
Heat treatment of the milk protein
Undenatured skimmed milk was heated at 80°C for 30 min in a thermostatically controlled water bath to prepare heated skimmed milk. The denatured WPI solution was made by reconstituting WPI powder in deionized water with up to 2.5% (wt/wt) total solid content. The solution was heated at 80°C for 3, 6, 9, or 12 min in a thermostatically controlled water bath and then freeze-dried to prepare the WPI with different denaturation degrees. Undenatured skimmed milk was supplemented with the WPI of different denaturation degrees to a content of 0.56 wt% (equivalent to the amount of WP normally present in milk) and heated at 80°C for 30 min. Afterward, the heated mixtures of the WPI-skim milk were cooled to room temperature to prepare WPI-containing heated skimmed milk.
Determination of whey protein nitrogen index
WP denaturation degree was evaluated using the whey protein nitrogen index (mg/g) in accordance with the method of Kuramoto et al. (Reference Kuramoto, Jenness, Coulter and Choi1959). The WPI samples (2 g) were submitted to the salting-out process with excess sodium chloride, filtration, and addition of hydrochloric acid and supersaturated sodium chloride solution. Transmittance readings were then performed at 420 nm.
Sodium dodecyl sulfate-polyacrylamide gel electrophoresis (SDS-PAGE)
The effects of heating time on the WP properties were evaluated by 15% SDS-PAGE (reduction/non reduction), which was performed using the Laemmli method with or without 2-mercaptoethanol as a reducing agent, Precision Plus Protein™ Unstained Protein Standards (Bio-Rad Laboratories, Hercules, CA) as markers, and Coomassie Brilliant Blue as a protein staining agent. An image of the gel after electrophoresis was captured using Chemi Doc™ (Bio-Rad), and band intensity was measured using Quantity One one-dimensional gel analysis software (Bio-Rad).
Preparation and strength measurement of acid milk gel
The method for preparing the acid milk gel and the measurement of physical properties were measured by the same method as previously reported (Oka et al., Reference Oka, Ono, Ohara, Noguchi and Takano2018). Acid milk gel was prepared by acidification with glucono-δ-lactone (GDL; Kanto Chemical, Tokyo). After adding GDL to milk sample to a concentration of 1.84%, 20 ml of the mixture was placed in a 50 ml plastic tube (inner diameter: 28 mm) and left at 37°C for 24 h. The gel reached a pH of approximately 4.2 after around 24 h of incubation. The strength at break (N) of these gels was measured by compression testing using the Instron Universal Testing Machine (Model 5564; Instron Corp., Norwood, MA, USA) with a 7 mm-diameter plunger at a compression speed of 0.8 mm/s.
Gel filtration chromatography
The effect of heating time on the molecular weight of WP was analyzed by gel filtration chromatography (GFC). The heated WPI samples were diluted to a concentration of 2 mg/ml with 50 mM phosphate buffer containing 0.3 M NaCl (pH 6.6), and 50 μl of the obtained solution was injected into a KW-804SB (diameter 1.2 cm × 27 cm, Shodex, Japan) column equilibrated with the same buffer and eluted at a flow rate of 0.3 ml/min. Peak areas were determined using Chromat-Pro (Runtime Corp., Tokyo, Japan) software to calculate the relative content of each protein and the heated complex. The WPI and κ-casein samples were mixed at amounts present in milk, and then dissolved in 50 mM phosphate buffer (pH 6.6), heated at 80°C for 30 min, and analyzed by GFC to probe the heating-induced formation of κ-casein–WPI complexes.
Quantitation of κ-casein
The extent of κ-casein dissociation was quantified by reversed-phase high-performance liquid chromatography using the method of Bonfatti et al. (Reference Bonfatti, Grigoletto, Cecchinato, Gallo and Carnier2008). The sample preparations were prepared according to the method proposed by Bobe et al. (Reference Bobe, Beitz, Freeman and Lindberg1998) A 50 μl aliquot of the prepared sample was loaded on a ZORBAX 300SB-C8 column (Agilent Technologies, Santa Clara, CA, USA) and eluted at 0.5 ml/min using a gradient of 0.1 wt% aqueous TFA → 0.1 wt% TFA in acetonitrile. The detection was performed at a wavelength of 214 nm. Peak areas were determined by fitting to a calibration curve constructed using a standard solution, and the ratio of κ-casein content in the whey fraction to that in milk was defined as the extent of κ-casein dissociation (%).
Statistical analysis
All results are expressed as an average of three replicates of individual experiments. The statistically significant differences (P < 0.01) were determined using the Tukey–Kramer multiple comparison adjustment method.
Results and discussion
Determination of the denaturation degree of whey protein
Aqueous WPI solutions heated at 80°C for 0, 3, 6, 9, 12, and 15 min presented WP nitrogen index of 10.3, 10.3, 7.4, 3.6, 2.5, and 0.9, respectively (online Supplementary Figure S1). The results of non-reducing SDS-PAGE are shown in Figure 1, and the intensity of the obtained band is shown beside each band in Figure 1. With an increase in heating time, the band intensity of α-La and β-Lg weakened, and bands appeared in the high-molecular-weight region around 250 kDa (Aggregate Ⅰ) after 6 min. After 9 min, bands at ≥250 kDa (Aggregate Ⅱ) at the top of the gel were detected and became more intense with time (online Supplementary Fig. S2). In the reducing SDS-PAGE, bands in the high-molecular-weight region were not detected, and the band pattern did not change compared with that of the unheated WPI. Heating the α-La/β-Lg mixtures results in the formation of aggregates held together by SS bonds and hydrophobic interactions (Hollar et al., Reference Hollar, Parris and Cockley1995; Ikeuchi et al., Reference Ikeuchi, Kato, Sugai, Fujino and Kuwajima1998; Schokker et al., Reference Schokker, Singh and Creamer2000). With the increase in heating time, the band intensity of α-La and β-Lg was reported to weaken, and the intensity of the band in the high-molecular-weight region became strong, suggesting that the number of aggregates of both proteins was different. From the above results, it was confirmed that WPIs with different degrees of denaturation or protein aggregates were obtained.

Fig. 1. SDS-PAGE (15%) of the whey protein with different denaturation degrees. M; molecular weight standard (Precision Plus Protein™ Unstained Protein Standards, Bio-Rad Laboratories, Hercules, CA). The number in brackets shows the intensity of the stained band (heating 0 min → heating 12 min).
Effect of whey protein denaturation degree on the strength of acid milk gels
Acid milk gels were prepared by adding WPI with different denaturation degrees to undenatured skimmed milk, and this showed that the WPI denaturation degree did not noticeably affect the strength of gels prepared without heating (Fig. 2). However, with heating, this effect was noticeable, with strengths of 110 ± 3.6 mN (non-addition WPI, means ± sd), 247 ± 1.1 mN (+non-denatured WPI), 249 ± 29.7 mN (+WPI denatured for 3 min), 203 ± 14.2 mN (+WPI denatured for 6 min), 141 ± 24.5 mN (+WPI denatured for 9 min), and 150 ± 15.3 mN (+WPI denatured for 12 min) (Fig. 2). As a result, the gel strength decreased as the degree of denaturation of the added WPI increased. Vasbinder et al. (Reference Vasbinder, Valde and de Kruif2004) reported that heated WP-soluble aggregates increase the strength of acid milk gels. Their results are different from those of the present study because in their study, the acid milk gel was prepared without heat treatment after WP-soluble aggregates were added to the heated milk. Thus, the curd strength increased upon the addition of WPI to the undenatured skim milk during heating, and it negatively correlated with the WPI denaturation degree.

Fig. 2. Effects of the denaturation degree of whey protein isolate (WPI) on the strength of acid milk gels prepared with glucono-δ-lactone. The error bars represent the standard deviation of three measurements. a−bMeans within a row with different superscripts differ significantly (Tukey–Kramer test, P < 0.01). Unheated = sample with the WPI added to skimmed milk and not heated. Heated = sample with the WPI added to skimmed milk and heated at 80°C for 30 min.
The extent of hydrophobicity of the casein micellar surface due to the κ-casein dissociation in heated milk affects the strength of acid milk gels (Oka et al., Reference Oka, Ono, Ohara, Noguchi and Takano2018), and the gel strength and κ-casein dissociation increases with WPI addition (Mohomud et al., Reference Mohomud, Katsuno and Nishizu2017). The dissociation of κ-CN is usually promoted by changes in pH during heating (Anema, Reference Anema2007). However, there was no change of pH due to the addition of denatured WPI. Therefore, it was concluded that the extent of dissociation of κ-casein influences the gel strength with the addition of WPI with variable denaturation degrees.
Effect of the extent of κ-casein dissociation on the strength of acid milk gels
Reversed-phase high-performance liquid chromatography was used to quantify the extent of κ-casein dissociation with the addition of WPI as well as to determine its relationship with the strength of acid milk gel. The κ-casein dissociation in WPI-free heated skimmed milk was determined as 25.7%. This dissociation is the effect of the WP present in the skimmed milk. For heated skimmed milk containing WPI, the extent of κ-casein dissociation from casein micelles was 42.9% in the +non-denatured WPI, 41.4% in the +WPI heated for 3 min, 39.1% in the +WPI heated for 6 min, 31.8% in the WPI heated for 9 min, and 30.6% in the WPI heated for 12 min (Table 1). Thus, the extent of κ-casein dissociation was revealed to be negatively correlated with the WPI denaturation degree and strongly positively correlated (R = 0.9815; P = 0.005) with gel strength. These results indicate that the extent of κ-casein dissociation is related to the difference in the strength of acid milk gels prepared using WPI with different degrees of denaturation, as reported previously (Oka et al., Reference Oka, Ono, Ohara, Noguchi and Takano2018). The dissociation of κ-casein occurs by the formation of an SS bond with β-Lg (Guyomarc'h et al., Reference Guyomarc'h, Dalgleish and Law2003, Reference Guyomarc'h, Renan, Chatriot, Gamerre and Famelart2007, Reference Guyomarc'h, Violleau, Surel and Famelart2010; Vasbinder et al., Reference Vasbinder, Alting and de Kruif2003). It was inferred that β-Lg suppresses binding to κ-casein owing to aggregate formation by heating (Fig. 1).
Table 1. Extent of κ-casein dissociation from casein micelles observed upon the 30 min heating of a variable-denaturation-degree whey protein isolate (WPI)–skimmed milk mixture at 80°C

a−fMeans within a row with different superscripts differ significantly (Tukey–Kramer test, P < 0.01). The values are presented as mean ± sd of three independent experiments.
Confirmation of κ-casein–WPI complex formation
As WPI denaturation degree was negatively correlated with the extent of κ-casein dissociation, factors contributing to the decrease in the latter parameter were further investigated. As κ-casein dissociation is triggered by the formation of SS bonds with β-Lg, the formation of κ-casein–WPI complexes by GFC was analyzed. For the WPI-only samples, two peaks were detected at retention times of 24.15 and 25.02 min. Based on the difference in molecular weight, the retention time of 24.15 min was considered to reflect β-Lg and that of 25.02 min was considered to reflect α-La (Fig. 3). A new peak (retention time = 15.38 min) appeared for the sample heated for 6 min and was ascribed to a high-molecular-weight product formed via the formation of SS bridges between α-La and β-Lg, as confirmed by SDS-PAGE. The relative contents of this high-molecular-weight product calculated from the peak area were 6.9, 17.9, and 23% at heating times of 6, 9, and 12 min, respectively, indicating that the formation of this high-molecular-weight product was positively correlated with the heating time, as confirmed by SDS-PAGE.

Fig. 3. Effect of heating time at 80°C on whey protein (WP) molecular weight.
Subsequently, GFC was used to analyze samples obtained by mixing and heating κ-casein and WPI with different degrees of denaturation, and a new peak was detected at a retention time of 12.99 min (Fig. 4). Considering a peak was observed at a retention time of 14.11 min for κ-casein alone, the peak at retention time 12.99 min was ascribed to a complex of WPI and κ-casein rather than to κ-casein itself. However, as the peaks of κ-casein and the κ-casein–WPI complex partially overlapped and could not be clearly separated, vertical splitting was then performed using Chromato-PRO software to determine the corresponding area ratio. The result revealed that the peak of the κ-casein–WPI complex had relative contents of 67.4% (WPI heating time = 0 min), 56.7% (3 min), 50.4% (6 min), 43.4% (9 min), and 40.0% (12 min), i.e., the content of the κ-casein–WPI complex decreased with increasing heating time. Conversely, the relative content of κ-casein was 0% (WPI heating time = 0 min), 11.8% (3 min), 17.7% (6 min), 23.7% (9 min), and 28.4% (12 min); that is, the residual amount of κ-casein increased with the increase in heating time.
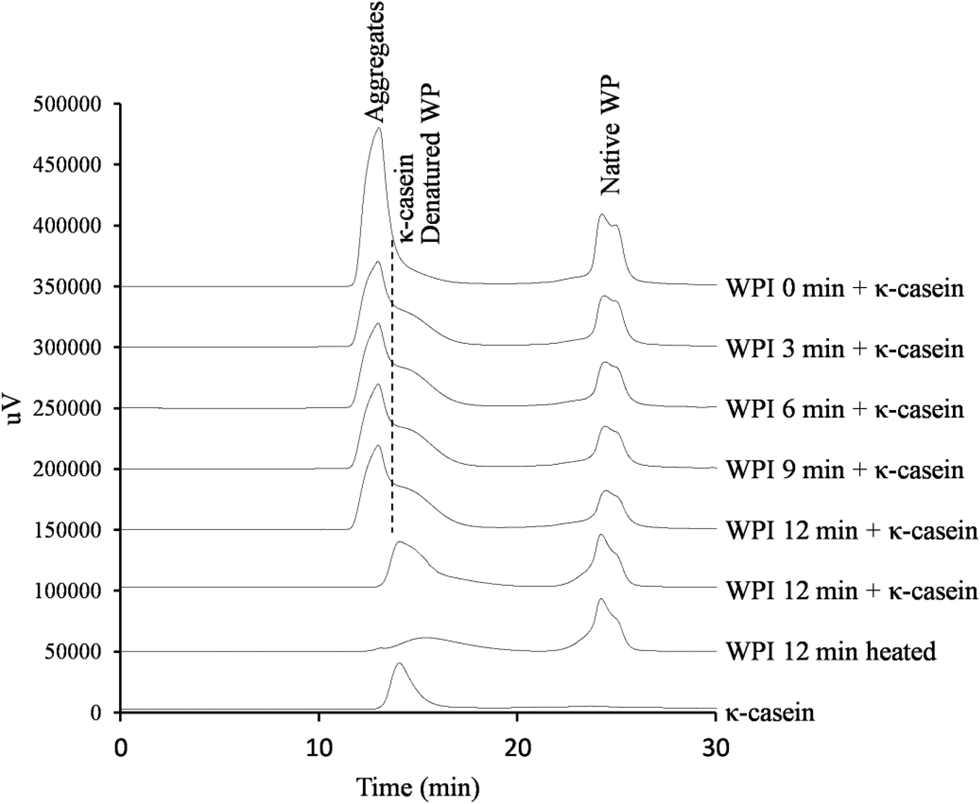
Fig. 4. Effect of whey protein (WP) denaturation degree on the molecular weight of heated κ-casein–WPI mixtures. The + symbol denotes the heated mixture of κ-casein and WPI.
The above results demonstrate that bonding with κ-casein is less likely to occur when α-La and β-Lg undergo heat-induced aggregation. β-Lg has a highly reactive free SH group (Considine et al., Reference Considine, Patel, Singh and Creamer2005), and therefore, it easily forms SS bonds with κ-casein (Elfagm and Wheelock, Reference Elfagm and Wheelock1978). Thus, if β-Lg forms SS bonds with α-La in advance upon heating, the amount of highly reactive free SH groups decreases, which complicates the formation of SS bridges between β-Lg and κ-casein. Under these circumstances, the extent of κ-casein dissociation from casein micelles is low, and the strength of the acid milk gel decreases. Lucy et al. (Reference Lucey, Michelle, Harjinder and Peater1998) reported that denatured WPs are involved in the characteristic differences among milk acid gels through the formation of intermolecular disulfide bonds with casein micelles. Given this, it was considered that the decrease in the binding amount of β-Lg and κ-casein due to the denaturation of WP affects not only the dissociation of κ-casein but also the binding of WP and casein micelles. From these insights, it was inferred that the degree of denaturation of the added WP is a factor that causes a decrease in acid milk gel strength.
In conclusion, the strength of acid milk gels increased with the supplementation of milk with the WPI and heating, and it negatively correlated with WPI denaturation degree. This behavior can be explained by considering the dissociation of κ-casein from casein micelles. The binding of β-Lg to α-La due to the thermal denaturation of WPI suppressed the binding of β-Lg to κ-casein and thus reduced the amount of κ-casein–WPI complexes. Given that binding to β-Lg induces the dissociation of κ-casein, the bonding between β-Lg and α-La inhibited the formation of bonds with κ-casein, and thus, hindered its dissociation and reduced the strength of acid milk gels.
Supplementary material
The supplementary material for this article can be found at https://doi.org/10.1017/S0022029922000103