Introduction
The polished section that contains grains of maletoyvayamite [pronounced: ma-le-toy-va-ya-mite], ideally Au3Se4Te6, comes from the Gaching occurrence of the Maletoyvayam deposit located in the southwestern part of the Koryak Highland of the central Kamchatka volcanic belt, Far East, Russia (60°19′51.87″N, 164°46′25.65″E). The Gaching Au mineralisation in the Maletoyvayam deposit is located in the Eocene–Oligocene central Kamchatka volcanic belt ~1800 km long (Fig. 1). The latter includes a number of other gold–silver epithermal deposits: Ozernovskoe, Aginskoe and Asachi (Goryachev et al., Reference Goryachev, Volkov, Gamyanin, Sidorov, Savva and Okrugin2010). The Maletoyvayam deposit is composed of andesites, tuffs and tuffaceous sandstones (Melkomukov et al., Reference Melkomukov, Razumny, Litvinov and Lopatin2010). The main gangue minerals are quartz, alunite, native sulfur and kaolinite. On the basis of the typomorphic features of mineral assemblages, the Maletoyvayam deposit belongs to high-sulfidation (HS type) epithermal deposits (Okrugin Reference Okrugin2003; Okrugin et al., Reference Okrugin, Andreeva, Yablokova, Okrugina, Chubarov and Ananiev2014; Kalinin et al., Reference Kalinin, Andreeva and Yablokova2012).

Fig. 1. Volcanic belts of the Kamchatka Peninsula (adapted from Tsukanov, Reference Tsukanov2015), and location of the epithermal deposits.
The vein and disseminated mineralisation in the Maletoyvayam deposit include pyrite, native gold and Au tellurides/selenides in association with Cu sulfosalts in quartzites and quartz–alunite altered rocks. Maletoyvayamite was described originally as an unnamed phase with the composition Au2(Se,S)3Te4 (Tolstykh et al., Reference Tolstykh, Vymazalová, Petrova and Stenin2017, Reference Tolstykh, Vymazalová, Tuhý and Shapovalova2018). It was noted that such a compound has never been found before in any other epithermal deposit.
Both the mineral and its name were approved by the Commission on New Minerals, Nomenclature and Classification of the International Mineralogical Association (IMA2019-021, Tolstykh et al., Reference Tolstykh, Tuhý, Vymazalová, Plášil, Laufek, Kasatkin and Nestola2019b). The name maletoyvayamite (Cyrillic – малетойваямит) is after its type locality, the Maletoyvayam deposit in the Kamchatka peninsula, Russia.
Holotype material with maletoyvayamite (polished section) along with its synthetic analogue (Exp Au2) are deposited in the Central Siberian Geological Museum (CSGM) of VS Sobolev Institute of Geology and Mineralogy of Siberian Branch of the Russian Academy of Sciences (SB RAS), Novosibirsk, Russia, catalogue number V-9/1.
Appearance, physical and optical properties
The individual grains (10–50 μm) of maletoyvayamite in intergrowths with native gold, calaverite, unnamed phases of AuSe–AuTe and Te–Se solid solutions, and sulfosalts (tennantite–tetrahedrite, goldfieldite, watanabeite) were found in a heavy-mineral concentrate. Associated minerals are ‘mustard gold’, complex Au oxides [(Au,Ag)–(Sb,As,Te,S)–O], senarmontite, tripuhyite, rooseveltite, tiemannite, antimonselite and guanajuatite. Associated calaverite has been oxidised in hypergenic conditions into mustard gold mixed with the Fe–Sb oxides (tripuhyite) and goethite or transformed into Au–Sb(Te,Se,S,As) oxides, whereas maletoyvayamite is stable in this environment and not oxidised (Tolstykh et al., Reference Tolstykh, Palyanova, Bobrova and Sidorov2019a). Back-scattered electron images of maletoyvayamite and associated minerals are shown in Fig. 2. Maletoyvayamite is opaque with metallic lustre. Cleavage is good on {010} and {001}, parting not observed. The powder of the synthetic analogue is grey in colour and has a grey streak. The mineral is brittle. The density calculated on the base of the empirical formula is 7.967 g cm–3. In plane-polarised reflected light maletoyvayamite is bluish grey, has strong bireflectance, strong anisotropy and exhibits no internal reflections. Reflectance was measured in air relative to a WTiC standard using a Zeiss 370 spectrophotometer MSP400 TIDAS mounted to Leica microscope with a 50× objective (National Museum in Prague, Czech Republic). Data are given in Table 1 and plotted in Fig. 3.
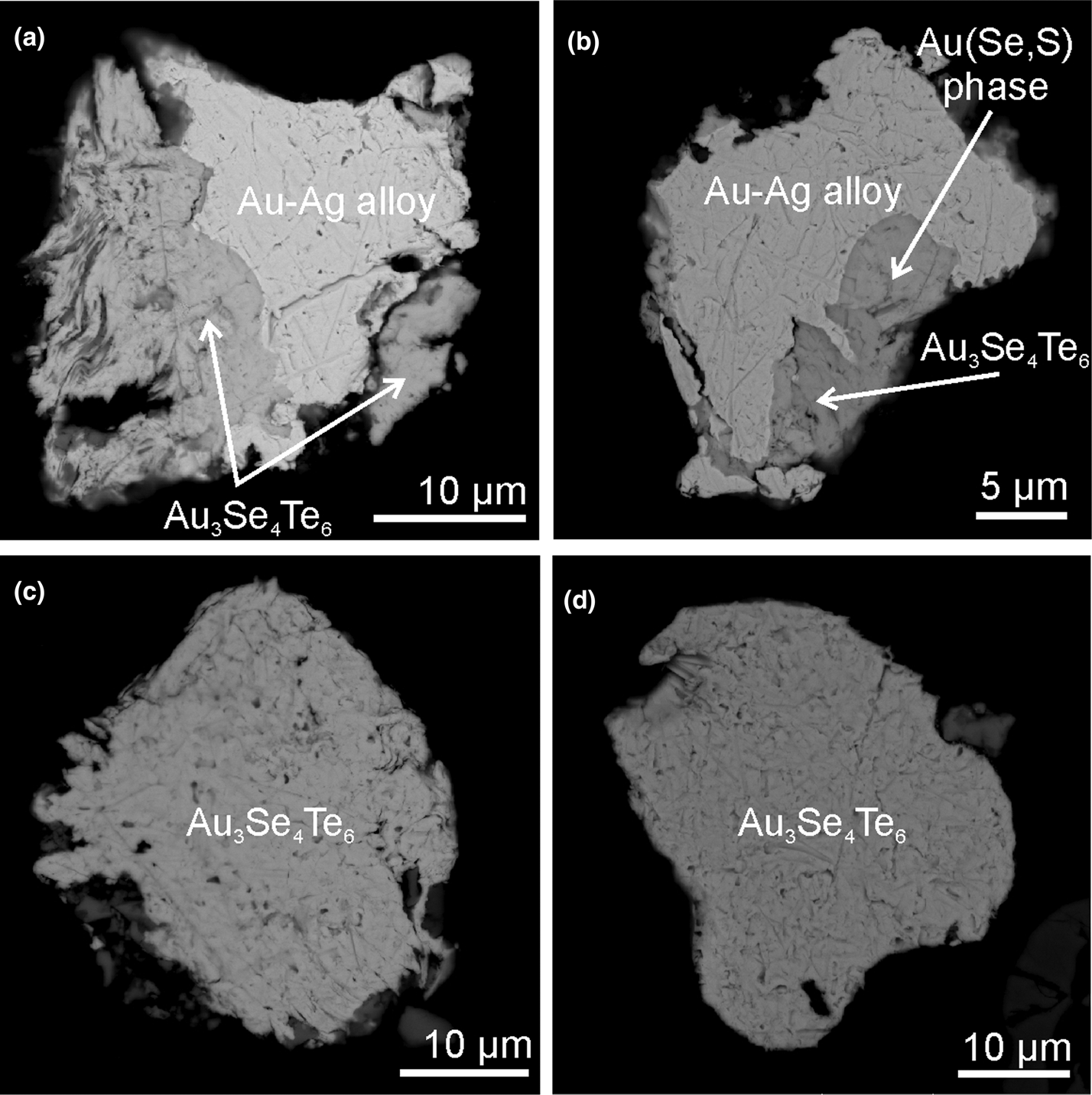
Fig. 2. Back-scattered electron micrographs (FEGSEM) of maletoyvayamite (Au3Se4Te6) intergrown with (a) Au–Ag alloy, (b) Au–Ag alloy and unidentified Au(Se,S) phase and (c,d) individual maletoyvayamite grains which were used for Raman spectroscopy analyses.
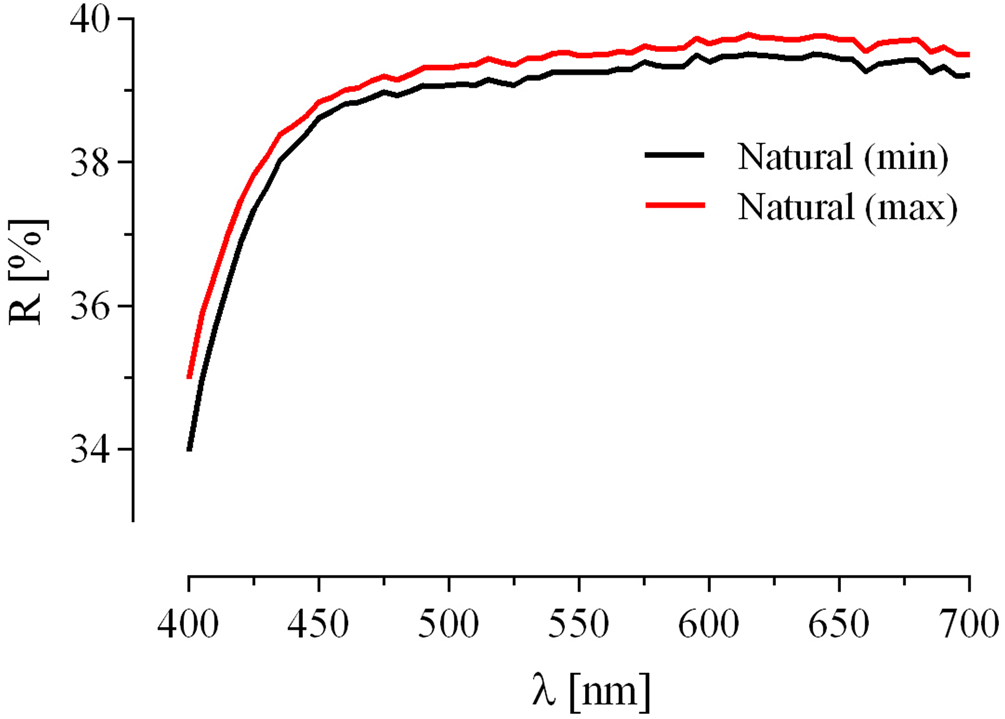
Fig. 3. Reflectance data of maletoyvayamite.
Table 1. Reflectance data of maletoyvayamite.

The values required by the Commission on Ore Mineralogy are given in bold.
Synthetic analogue
Single-crystal X-ray studies of (natural) maletoyvayamite could not be carried out because the crystals tested were too poor. Therefore, a synthetic Au3Se4Te6 phase was prepared and subsequently used for a crystal structure analysis. The X-ray diffraction data obtained from the natural material (the largest grain of size 20–50 μm) were not sufficient to solve the crystal structure.
Synthetic Au3Se4Te6 was prepared using the evacuated silica glass tube method in the Laboratory of Experimental Mineralogy of the Czech Geological Survey in Prague. Gold (99.95%; additionally, refined at the laboratory using a digestion–precipitation technique), tellurium (99.999%) and selenium (99.999%) were used as starting materials for the synthesis. The evacuated silica-glass tube with its charge was sealed and heated at 400°C. Three times during the experiment, after cooling by a cold-water bath, the charge was ground into powder in acetone using an agate mortar, and thoroughly mixed to homogenise. The pulverised charge was sealed in an evacuated silica-glass tube again and heated at 400°C for 4 months. Finally, the experimental product was quenched rapidly in a cold-water bath.
Chemical composition
The polished sections prepared from both the natural and experimental samples were also examined using an electron probe microanalyser (EPMA, CAMECA SX-100) equipped with wavelength dispersion spectrometers (WDS). Quantitative data and chemical analysis were obtained using 15 kV acceleration voltage and 10 nA beam current (diameter: 1–2 μm). Standards were pure metals (Au and Te), Bi2Se3 (Se) and FeS2 (S). No other elements with atomic numbers higher than 8 were detected. The EPMA results for maletoyvayamite, both natural and synthetic are given in Table 2. The empirical formula, calculated for the mean composition (n = 16; measured on nine different grains) on the basis of 13 atoms per formula unit (apfu) is Au2.90(Se3.52S0.44)Σ3.96Te6.14 for natural maletoyvayamite, the ideal chemical formula is Au3Se4Te6 which requires Au 35.34, Se 18.88, Te 45.78, total 100 wt.%.
Table 2. Chemical composition of maletoyvayamite (wt.%) and its synthetic analogue.

X-ray crystallography
Single-crystal diffraction study of synthetic analogue Au3Se4Te6
As the natural crystals of maletoyvayamite proved to be unsuitable for crystal structure analysis, the relevant structural investigations were performed on a synthetic analogue Au3Se4Te6. Only a powder X-ray diffraction pattern of natural maletoyvayamite was collected (see below). Single-crystal X-ray studies on synthetic analogue Au3Se4Te6 were carried out using a Rigaku SuperNova single-crystal diffractometer with Atlas S2 CCD detector and monochromatised MoKα radiation from the microfocus X-ray tube. The crystal structure was solved from single-crystal data using the program SHELXT (Sheldrick, Reference Sheldrick2015), and subsequently refined by the full-matrix least-squares of the JANA2006 program (Petříček et al., Reference Petříček, Dušek and Palatinus2014). All atoms were refined with anisotropic displacement parameters; R 1 = 0.0375 for 2042 unique reflections with I > 3σ(I) and 119 refined parameters. Crystallographic details and details for the structure refinement can be found in Table 3, atom coordinates and displacement parameters in Table 4 and selected interatomic distances in Table 5. The crystallographic information files have been deposited with the Principal Editor of Mineralogical Magazine and are available as Supplementary material (see below).
Table 3. Single-crystal data collection and structure-refinement details for synthetic Au3Se4Te6.

Table 4. Atom coordinates, anisotropic and equivalent displacement parameters (Å2) for synthetic Au3Se4Te6.

Table 5. Selected bond distances (Å) for synthetic Au3Se4Te6.

Powder X-ray diffraction study of natural maletoyvayamite and its synthetic analogue Au3Se4Te6
The micro-powder X-ray diffraction data on a natural crystal of maletoyvayamite were acquired using a Rigaku-Oxford Diffraction Supernova diffractometer equipped with an X-ray micro-source (MoKα) and a 200K Dectris detector on a grain with an average radius of 0.04 mm. The sample-to-detector distance was 68 mm. The data were measured over 0–360° in φ with an exposure time of 60 s per frame. A complete list of observed d values and relative intensities is reported in Table 6. The refined unit-cell parameters are: a = 8.901(2), b = 9.0451(14), c = 9.265(4) Å, α = 97.66(3)°, β = 106.70(2)°, γ = 101.399(14)° and V = 685.9(4) Å3.
Table 6. Comparison of powder XRD patterns (d in Å) of maletoyvayamite and its synthetic analogue Au3Se4Te6.

a Obtained by the pseudoGandolfi method, micro-source MoKα radiation.
b Collected in Bragg–Brentano geometry, CuKα radiation.
The powder X-ray diffraction data of the synthetic material were collected using Bruker D8 Advance diffractometer equipped with LynxEye XE detector in Bragg–Brentano geometry and using CuKα radiation. The data were collected in the range from 6° to 110°2θ with a step size of 0.015°2θ. Considering the low symmetry of the maletoyvayamite and resulting strong degree of reflections overlap in powder diffraction patterns, the unit-cell parameters were calculated using the Rietveld method in the Topas 5 program (Bruker AXS, Reference Bruker2014). The structure model obtained from single-crystal study (see above) was used as the starting structure model in a subsequent Rietveld refinement of synthetic Au3Se4Te6. The refined parameters include those describing crystal size, preferred orientation along [010] and unit-cell parameters. No fractional coordinates and displacement parameters were refined. The refinement converged to the agreement factor R wp 13.89% and yielded refined unit-cell parameters: a = 8.9835(3), b = 9.1129(2), c = 9.3090(3) Å, α = 97.29(1)°, β = 107.11(1)°, γ = 101.73(1)°, and V = 698.81(6) Å3.
A comparison of powder X-ray diffraction data between maletoyvayamite and its synthetic analogue is shown in Table 6. The obtained unit-cell parameters are compared in Table 7. The positions and intensities of diffractions from a fragment of natural material extracted from the polished sections match very well with the diffraction pattern of synthetic Au3Se4Te6 and consequently demonstrates the structural identity between natural material and its synthetic analogue. The structural identity was further supported by Raman spectroscopy (see below). A few discrepancies in positions of diffractions might be due to the slightly different compositions of natural maletoyvayamite and its synthetic analogue. The minor difference in intensities might be due to using different diffraction methods (PseudoGandolfi versus Bragg–Brentano), radiations (MoKα versus CuKα) and preferred orientation observed in the diffraction pattern from Bragg–Brentano geometry.
Table 7. Comparison between unit-cell parameters obtained by powder XRD performed on maletoyvayamite, and powder and single-crystal XRD on its synthetic analogue.

Structure description
The crystal structure of maletoyvayamite contains three Au, six Te and four Se atoms in the asymmetric unit (Fig. 4). The structure is relatively simple and resembles a molecular structure. The basic structural unit is a molecular cluster with [Au6Se8Te12] composition and can be described as a distorted cube with Se atoms at vertices. Au atoms occupy centres of the faces of the cube and Te atoms are placed at the midpoints of Se–Se edges. Each unit cell contains one cluster. Au atoms show a square planar coordination by four Te atoms, as is typical for Au in d 8 configuration. Whereas Au1 atom shows four Te at almost equal distances of 2.68 Å, Au2 and Au3 atoms show two shorter (2.68 Å) and slightly longer (2.75 Å) Te contacts. This tiny difference in Au–Te bonds causes the slight distortion of the [Au6Se8Te12] clusters from the ideal cubic shape. Se atoms, forming corners of the [Au6Se8Te12] clusters, show three short Te contacts at distances ranging from 2.641(2) to 2.951(2) Å. The distortion of the cluster is mirrored by the values of Au–Se–Au angles ranging from 84.74(6)° to 88.56(6)° instead of being 90° for an ideal cube. The Se and Te atoms have acentric environments implying stereoactivity of lone electron pairs.

Fig. 4. Crystal structure of Au3Se4Te6 showing the [Au6Se8Te12] cluster. The [AuTe4] square is emphasised (blue shading) for clarity.
The [Au6Se8Te12] clusters are linked in the [100] direction (Fig. 5) via weak Se–Se (3.39 Å) and Au–Au (3.41 Å) interactions. The linkage in approximate [010] and [001] directions is achieved through weak Se–Se (3.37 Å), Au–Te (3.36), Au–Se (3.69 Å), Te–Te (3.69 Å) and Te–Se (3.77 Å) interactions. It is interesting to note that Au atoms form an octahedron within the [Au6Se8Te12] cluster with Au–Au distances ranging from 3.79 to 3.95 Å (Fig. 5). These distances are shorter than the Au–Au contacts between the [Au6Se8Te12] clusters (see above), however they indicate a certain degree of weak interactions.

Fig. 5. System of weak bond interactions between [Au6Se8Te12] clusters in the crystal structure of Au3Se4Te6. Green, yellow, red and blue dashed lines represent Se–Se, Au–Au, Te–Te and Au–Te weak interactions between clusters, respectively. Violet dashed lines indicate weak Au–Au interactions within in one of [Au6Se8Te12] cluster.
The crystal structure of maletoyvayamite represents a unique structure type; no exact structural analogues among minerals are known. It is a molecular structure and hence it is structurally very different from the well-known gold tellurides (e.g. Bindi et al., Reference Bindi, Paar and Lepore2018 and references therein). The presence of molecular clusters in the maletoyvayamite structure resembles crystal structures of realgar, AsS, though that structure contains As4S4 molecules (Mullen and Nowacki, Reference Mullen and Nowacki1972) and hence is structurally very different from maletoyvayamite. The structure of sinnerite [Cu6As4S9] (Bindi et al., Reference Bindi, Nestola and Makovicky2013) is metrically close to maletoyvayamite, however it contains an As4S12 molecular cluster composed of four AsS3 coordination pyramids. The crystal structure of the chemically related synthetic phase α-AuSe can be described as packing of infinitively long AuSe molecules parallel to the b axis (Rabenau and Schulz, Reference Rabenau and Schulz1976). These molecules are connected by weak Au–Au (3.22 Å) and Se–Se (3.35–3.73 Å) interactions. However, a linear coordination of Au atoms observed in α-AuSe was not observed in the maletoyvayamite structure. Similar to the [Au6Se8Te12] unit is the [Re6Se8(OH)6] cluster observed in the structure of the K4[Re6Se8(OH)6].5H2O synthetic phase (Brylev Reference Brylev2013), however it lacks the atoms positioned at the midpoints of edges of the Se8 cube (i.e Te atoms in maletoyvayamite).
Raman spectroscopy
Raman spectroscopy was applied to four grains of maletoyvayamite and compared with its synthetic analogue Au3Se4Te6. The Raman spectra of natural and synthetic Au3Se4Te6 are shown in Fig. 6. Raman spectra were obtained in back-reflecting geometry using an S&I MonoVista CRS+ Raman microspectrometer (spectrometer SP2750i, Princeton Instruments; grating 1800 gr./mm) equipped with a Peltier-cooled Andor iDus-416 CCD detector (size 2000 × 256 pixels, pixel size 15 × 15 μm) and Cobolt Samba 100 mW Nd–YAG laser source (nominal wavelength λ = 532 nm) installed at the Czech Academy of Sciences, Institute of Geology, Prague, Czech Republic. Laser power on the sample was measured with a Coherent LaserCheck pencil-style power meter as 1.8 mW. An upright microscope Olympus BX-51 WI with a 100× magnifying objective was used to direct the laser beam on the sample and to collect the Raman signal. An exposure time of 10 s with 25 accumulations and a single-frame correction for cosmic radiation was used to acquire the spectra. The wavelength scale was calibrated with an IntelliCal Hg-Ne-Ar lamp and Raman band positions were checked against those of silicon and polystyrene standards. The obtained Raman spectra of natural and synthetic Au3Se4Te6 show four discernible absorption bands at the following values: 101, 137, 158 and 178 cm–1 (Fig. 6).

Fig. 6. Raman spectrum of maletoyvayamite in comparison with its synthetic analogue Au3Se4Te6.
The Raman spectra of maletoyvayamite and its synthetic analogue Au3Se4Te6 (Fig. 6) are identical and further supports the structural identity between synthetic and natural material.
Genetic implications
The Gaching ore occurrence of the Maletoyvayam deposit belongs to the HS (high sulfidation) type of epithermal deposits. Gold and Se-rich minerals are characteristic for this ore association that implies special conditions for their formation: an abundant source of Au and Se, and a strongly oxidising environment (pH = 0–2). Therefore, under these conditions Au selenides can be formed. Maletoyvayamite, Au3Se4Te6, in intergrowths with Au–Ag alloys, Au tellurides, and other unnamed Au–Se–Te compounds are related to the main stage of ore mineralisation which is stable at 250°C in a log$f_{{\rm S}{\rm e}_ 2}$ range of −12.4 and −5.7 (Tolstykh et al., Reference Tolstykh, Vymazalová, Tuhý and Shapovalova2018). A further increase of
$f_{{\rm O}_ 2}$ leads to oxidation of Au tellurides to Au,Ag(Sb,As,Te,S) oxides and formation of mustard gold (Tolstykh et al., Reference Tolstykh, Tuhý, Vymazalová, Plášil, Laufek, Kasatkin and Nestola2019b).
Supplementary material
To view supplementary material for this article, please visit https://doi.org/10.1180/mgm.2019.81.
Acknowledgements
The authors acknowledge Ritsuro Miyawaki, Chairman of the IMA–CNMNC and its members for helpful comments on the submitted data. The studies of minerals were carried out within the framework of the state assignment of the VS Sobolev Institute of Geology and Mineralogy of SB RAS. The authors would like to thank Vladimir Korolyuk and Mikhail Khlestov for assisting during analytical procedures, Luboš Vrtiška (National Museum in Prague) for reflectance measurements, Roman Skála (Institute of Geology AS CR, v.v.i.) for Raman measurements and Zuzana Korbelová (Institute of Geology AS CR, v.v.i.) for EPMA. This research was supported by the Grant Agency of the Czech Republic (project No. 18-15390S), by Russian Foundation for Basic Research (RFBR No. 19-05-00316) and by the Ministry of Education, Youth and Sports National sustainability program I of the Czech Republic (project No. LO1603). Additional support came from the Ministry of Education and Science of the Russian Federation (project no. 14.Y26.31.0018). The authors would like to thank Peter Leverett and one anonymous reviewer for the comments and the Principal Editor Stuart Mills.