1. Introduction
Lacustrine carbonates are an important inorganic carbon sink (Dean, Reference Dean1999; Einsele et al. Reference Einsele, Yan and Hinderer2001; Li et al. Reference Li, Wang, Li, Zhou, Zhang and Wang2013) because the dissolution and burial of carbonate are crucial aspects of the global carbon cycle (Archer et al. Reference Archer, Emerson and Reimers1989; Schrag et al. Reference Schrag, Higgins, Macdonald and Johnston2013). Carbonate deposition is sensitive to climate change, thus providing a valuable proxy for palaeoclimate reconstruction (Platt & Wright, Reference Platt and Wright1991; Dean & Megard, Reference Dean and Megard1993; Détriché et al. Reference Détriché, Bréhéret, Karrat, Hinschberger and Macaire2013). As reservoirs or source rocks, lacustrine carbonates further have a considerable petroleum-geology significance (Katz, Reference Katz2001; Thompson et al. Reference Thompson, Stilwell and Hall2015). With the exploration and development of shale oil and gas in recent years (Slatt & Rodriguez, Reference Slatt and Rodriguez2012; Zou et al. Reference Zou, Yang, Cui, Zhu, Hou, Tao, Yuan, Wu, Lin, Wang, Bai and Yao2013; Zhang et al. Reference Zhang, Yuan, Wang, Ge, Huo, Xu, Zhang, Cheng and Jiang2021), studying carbonate-rich shale has become increasingly important (Macquaker & Adams, Reference Macquaker and Adams2003; Loucks & Stephen, Reference Loucks and Stephen2007; Kong et al. Reference Kong, Jiang, Han, Zheng, Zhang, Zhang and Tian2017). The depositional mechanism of lacustrine carbonates remains a topic for active research (Last & Vance, Reference Last and Vance2002; Last & Last, Reference Last and Last2012; Lima & Ros, Reference Lima and Ros2019).
Lacustrine carbonates have various sources, including reworked detrital carbonates, chemical precipitation and biological processes (Talbot & Kelts, Reference Talbot and Kelts1986; Hodell et al. Reference Hodell, Brenner, Kanfoush, Curtis, Stoner, Song, Wu and Whitmore1999; Jiang et al. Reference Jiang, Chen, Qiu, Liang and Ma2007; Singh et al. Reference Singh, Saraswat, Naik and Nigam2017). Carbonate deposition is influenced by many factors, including temperature variations in the water column (Gilbert & Leask, Reference Gilbert and Leask1981; Bassetti et al. Reference Bassetti, Manzi, Lugli, Roveri, Longinelli, Lucchi and Barbieri2004), climate (Détriché et al. Reference Détriché, Bréhéret, Karrat, Hinschberger and Macaire2013; Nelson & Rey, Reference Nelson and Rey2018), water depth (Roeser et al. Reference Roeser, Franz and Litt2016), surface-water plankton (Platt & Wright, Reference Platt and Wright1991; Dean, Reference Dean1999) and water alkalinity (Shapley et al. Reference Shapley, Ito and Donovan2005). Depositional models of lacustrine carbonates have been described in the literature (Platt & Wright, Reference Platt and Wright1991; Gierlowski-Kordesch, Reference Gierlowski-Kordesch, Alonso-Zarza and Tanner2010; Quijada et al. Reference Quijada, Suarez-Gonzalez, Benito and Mas2013; Lettéron et al. Reference Lettéron, Hamon, Fournier, Séranne, Pellenard and Joseph2018). Considerable advances have been made in understanding lacustrine carbonate deposition. However, the varying patterns of lacustrine carbonates and the depositional model based on comparison of the Eocene and Holocene lacustrine carbonates need to be further investigated, in order to deepen understanding of the genetic mechanisms of lacustrine carbonate deposition to reconstruct palaeoenvironmental changes.
The Dongying Depression is a typical representative of faulted basins and is one of the prolific oil-producing basins in eastern China and across Southeast Asia, where more than 30 oilfields have been discovered (Guo et al. Reference Guo, He, Liu, Song, Wang and Shi2010). Lacustrine carbonates concentrate the organic-rich shale of the Eocene Shahejie Formation, which is a source rock and a target for shale oil exploration and development (Liang et al. Reference Liang, Cao, Jiang, Wu, Song and Wang2017; Chen et al. Reference Chen, Jiang, Zhang and Zha2018; Li et al. Reference Li, Chen, Ma, Cao, Li and Jiang2018). Nearly 200 m of continuous lacustrine carbonate-rich cores are accumulated, with a carbonate content reaching 95 wt %. Therefore, the Dongying Depression is an ideal location to study lacustrine carbonate deposition. Carbonate deposition in modern lakes, moreover, provides support for analysing the sedimentary characteristics and controlling factors of carbonate precipitation (Shapley et al. Reference Shapley, Ito and Donovan2005; Stephenson et al. Reference Stephenson, Leng, Michie and Vane2006; Valero-Garcés et al. Reference Valero-Garcés, Morellón, Moreno, Corella, Martín-Puertas, Barreiro, Pérez, Giralt and Mata-Campo2014). Based on the analysis of carbonate mineralogy, petrology, geochemistry, vertical evolution and distribution, this study has two objectives: (1) to provide a depositional model of carbonates in the Eocene Dongying Depression and (2) to compare the Eocene and Holocene lacustrine carbonate deposition.
2. Geological setting
2.a. Geological setting of the Dongying Depression
2.a.1. Structural and stratigraphic features
The Dongying Depression is an asymmetric half-graben situated in the southeastern Bohai Bay Basin in eastern China (Fig. 1a—b; Li & Li, Reference Li and Li2017). It is bordered by the Chengjiazhuang uplift to the north, the Luxi uplift to the southwest, the Guangrao uplift to the southeast, the Qingtuozi uplift to the northeast, and the Binxian and Qingcheng uplifts to the west. The east–west length and north–south width of the depression are c. 90 and 65 km, respectively (Fig. 1c). The depression comprises four oil-bearing sags (Lijin, Boxing, Niuzhuang and Minfeng), a northern steep slope, a central anticline zone and a southern gentle slope (Fig. 1d; Ding et al. Reference Ding, Zhang, Xie, Li, Shi, Zhang, Zhang and Tang2014).

Fig. 1. Location map, structural units and sedimentary facies in the Dongying Depression. (a–b) Location of the Dongying Depression. (c) Sedimentary facies distribution of the Es4s sub-member (Tian & Jiang, Reference Tian and Jiang2009). Micropalaeontological analysis suggests that the depth range of shallow lake is 0–15 m and the depth range of semi-deep lake to deep lake is 20–30 m (Su et al. Reference Su, Ding, Jiang, Hu, Meng and Chen2012). (d) Structural cross-section (for the location, see (c)).
The Dongying Depression hosts Palaeogene, Neogene and Quaternary strata, among which the Palaeogene and Neogene act as the most important basin-filling strata and exhibit a maximum thickness of c. 7000 m. The Palaeogene strata include the Kongdian, Shahejie (Es) and Dongying formations from bottom to top (Fig. 2). The Es Formation can be divided into four members (Es1–Es4; Fig. 2; Yang et al. Reference Yang, Cao, Friis, Wang and Zhou2018). The upper member of Es4 (Es4s) shale is carbonate-rich and was selected for this study.

Fig. 2. Stratigraphy of the Dongying Depression (Ma et al. Reference Ma, Fan, Lu, Liu, Hao, Xie, Liu, Peng, Du and Hu2016; Liang et al. Reference Liang, Jiang, Cao, Wu, Wang and Fang2018 b).
2.a.2. Sedimentary facies
The Dongying Depression started to expand from the Palaeocene, and the tectonic evolution of the Cenozoic includes three stages: a rifting, a fault–depression conversion and a post-rifting thermal subsidence stage (Fig. 2). The Es4s sub-member was deposited during the rifting stage with a rapid subsidence (∼70 m Ma−1; Feng et al. Reference Feng, Li and Lu2013; Fig. 2). A semi-deep lake is widely developed in the central area of the basin (Fig. 1c; Tian & Jiang, Reference Tian and Jiang2009; Liang et al. Reference Liang, Jiang, Cao, Wu, Wang and Fang2018 b). Organic-rich shale c. 200 m thick is developed in the central area of the Dongying Depression (Fig. 2; Guo et al. Reference Guo, He, Liu, Song, Wang and Shi2010; Zhang et al. Reference Zhang, Jiang, Liang, Wu, Xian and Li2016). Fan delta and nearshore subaqueous fan are developed along the northern steep slope, and beach-bars are distributed along the southern gentle slope of the Dongying Depression (Fig. 1c; Tian & Jiang, Reference Tian and Jiang2009). Sr/Ba and B/Ga ratios suggest that the Dongying Depression is a saline lake of the Es4s sub-member (Liang et al. Reference Liang, Jiang, Cao, Wu, Wang and Fang2018 b).
2.b. Geological setting of four Holocene lakes
The Qinghai and Barkol lakes in China, the Oro Lake in Canada and the Montcortès Lake in Spain are four Holocene lakes which are comparable to the Dongying Depression and are saline lakes. The sediments in these lakes are rich in carbonates, and the vertical evolution of carbonate deposition is comparable to that of the Dongying Depression.
Qinghai Lake is the largest saline lake in China, spanning an area of 4400 km2 (Fig. 3a—b; Xu et al. Reference Xu, Ai, Tan and An2006; Liu et al. Reference Liu, Zhang, Zhao, Wang, An and Liu2018). The average water depth is 20 m, with a maximum depth of 27 m (Xu et al. Reference Xu, Liu, An, Hou, Dong and Liu2010). The total organic carbon (TOC) content ranges from 2.25 to 6.80 wt % (average 3.40 wt %; Xu et al. Reference Xu, Ai, Tan and An2006). The carbonate content of surface sediments ranges from 30 to 66 wt % (Qian & Song, Reference Qian and Song2010; Liu et al. Reference Liu, Zhang, Zhao, Wang, An and Liu2018). It is composed mainly of aragonite and calcite with multiple sources (Qian & Song, Reference Qian and Song2010; Liu et al. Reference Liu, Zhang, Zhao, Wang, An and Liu2018). The clay minerals are mainly illite, followed by chlorite, with minor content of montmorillonite and kaolinite (Zeng et al. Reference Zeng, Song, An, Chang and Li2014). The content and morphology of carbonate and clay minerals in Qinghai Lake are comparable with those in the Dongying Depression, whereas the terrigenous clastic content is lower. Barkol Lake is a saline lake located in a graben in Xinjiang, China (Fig. 3c–d; Zhong et al. Reference Zhong, Pen, Xue, Ouyang, Tang and Cao2012). The carbonate content of surface sediment ranges from 6 to 60 wt % (Li et al. Reference Li, Lv, Tao and An2008; Zhao et al. Reference Zhao, Lv, An, Tao, Zheng and Dong2010). The Oro lake is located in Canada and is a small saline lake (0.5 km2 and ∼30 parts per thousand salinity) with a relatively shallow water body (mean depth 3 m; maximum depth:6 m; Fig. 3e–f; Last & Vance, Reference Last and Vance2002). Carbonate deposition is dominated by aragonite, calcite and dolomite (Last & Vance, Reference Last and Vance2002). The Montcortès Lake is located in Spain and is one of the deepest karst lakes in the Iberian Peninsula (Valero-Garcés et al. Reference Valero-Garcés, Morellón, Moreno, Corella, Martín-Puertas, Barreiro, Pérez, Giralt and Mata-Campo2014). Montcortès Lake is meromictic, and its water bodies lack mixing, yielding the formation of a permanently anoxic monimolimnion below 18 m in summer and 20 m in winter (water depth up to 30 m; Fig. 3g–h; Corella et al. Reference Corella, Moreno, Morellón, Rull, Giralt, Rico, Pérez-Sanz and Valero-Garcés2011, Reference Corella, Brauer, Mangili, Rull, Vegas-Vilarrúbia, Morellón and Valero-Garcés2012; Valero-Garcés et al. Reference Valero-Garcés, Morellón, Moreno, Corella, Martín-Puertas, Barreiro, Pérez, Giralt and Mata-Campo2014). Calcite laminae textures are developed (4.77–0.26 m) in distal facies, reflecting seasonal changes in limnological conditions (Valero-Garcés et al. Reference Valero-Garcés, Morellón, Moreno, Corella, Martín-Puertas, Barreiro, Pérez, Giralt and Mata-Campo2014).

Fig. 3. Geological settings of four modern lakes. (ab) Contour map of water depth and TOC content in Qinghai Lake, respectively, modified from Liu et al. (Reference Liu, Liu, Wang, An and Cao2017) and Huang (Reference Huang1981). (c) Location of Barkol Lake, modified from Li et al. (Reference Li, Lv, Tao and An2008). (d) Satellite image of Barkol Lake. (e) Location and bathymetry map of Oro Lake, modified from Last & Vance (Reference Last and Vance2002). (f) Satellite image of Oro Lake. (g) Location and bathymetry map of Montcortès Lake, modified from Valero-Garcés et al. (Reference Valero-Garcés, Morellón, Moreno, Corella, Martín-Puertas, Barreiro, Pérez, Giralt and Mata-Campo2014). (h) Satellite image of Montcortès Lake.
3. Methods
3.a. Data
The data include a composite core of 465 m from wells FY1, LY1 and NY1 in the Dongying Depression (Fig. 1). X-ray diffraction (XRD) was performed on 377 samples, and thin-section observations were performed on 193 samples. Field-emission scanning electron microscopy (FESEM) was performed on 58 samples, X-ray fluorescence (XRF) and inductively coupled plasma – mass spectrometry (ICP-MS) were conducted on 138 samples, and Rock-Eval pyrolysis was performed on 93 samples. All measurements were performed at the Geological Science Research Institute of Shengli Oilfield, Sinopec, except for the FESEM observation, which was performed at the Experimental Research Center of the Petroleum Geology Institute (Wuxi), Sinopec.
Data on mineralogy, petrology and organic geochemistry of the four Holocene lakes were collected from the literature. The chronology of sediment sequence in the four Holocene lakes is derived from radiocarbon ages obtained from accelerator mass spectrometry (Last & Vance, Reference Last and Vance2002; Li et al. Reference Li, Lv, Tao and An2008; An et al. Reference An, Colman, Zhou, Li, Brown, Timothy Jull, Cai, Huang, Lu, Chang, Song, Sun, Xu, Liu, Jin, Liu, Cheng, Liu, Ai, Li, Liu, Yan, Shi, Wang, Wu, Qiang, Dong, Lu and Xu2012; Valero-Garcés et al. Reference Valero-Garcés, Morellón, Moreno, Corella, Martín-Puertas, Barreiro, Pérez, Giralt and Mata-Campo2014). The above data are integrated and discussed with the data from Dongying Depression to analyse the depositional characteristics of lacustrine carbonates.
3.b. XRD and petrology
Mineral and petrological characteristics were obtained using XRD analysis, thin-sections and FESEM observations. Approximately 150 g of fresh rock was pulverized to obtain a sediment fraction finer than 200-mesh size. The XRD analysis was performed on the powdered samples using an X’Pert-Multipurpose Diffractometer. Thin-sections were observed using a polarizing Zeiss microscope (Axio Scope A1). The FESEM observation samples were gold-coated and observed using a HITACHI S-4800 system.
3.c. TOC and Rock-Eval pyrolysis
Analysis of the organic geochemistry characteristics included the TOC, free hydrocarbons (S1, mg HC/g rock), hydrocarbons cracked from kerogen (S2, mg HC/g rock), chloroform bitumen ‘A’, maximum yield temperature of pyrolysate (T max) and hydrogen index (HI). The TOC content was measured using a CS344 carbon–sulfur detector (Leco Company, US). The Rock-Eval pyrolysis was conducted using the Rock-Eval 6 analyser.
3.d. Assessment of the depositional conditions
The depositional conditions of the Es4s shale were studied based on the analyses of mineralogy, petrology, organic characteristics and inorganic geochemical elements. XRF was performed to determine the major elements (i.e. Na and Al). Trace elements (i.e. Ti, Sr, Ba, V and Ni) were determined via ICP-MS (JY38S).
Titanium (Ti) was used as an indicator for detrital influx (Murphy et al. Reference Murphy, Sageman, Hollander, Lyons and Brett2000; Tribovillard et al. Reference Tribovillard, Algeo, Lyons and Riboulleau2006). Relatively low Na/Al ratios are interpreted as intense rainfall and chemical weathering under a warm and humid climate, and conversely indicate predominantly mechanical weathering under arid and cold climate conditions (Aplin, Reference Aplin, Manning, Hall and Hughes1993). Therefore, Na/Al ratios can be used as an indicator of the palaeoclimate and strength of chemical weathering. The Sr/Ba ratio can be used as a proxy for water-body salinity because these are positively proportional to each other (Liang et al. Reference Liang, Jiang, Cao, Wu, Wang and Fang2018 b). Biogenic Ba (Babio) is used as a proxy for effective palaeoproductivity and correlates positively to palaeoproductivity (Tribovillard et al. Reference Tribovillard, Riboulleau, Lyons and Baudin2004). It is usually calculated using the equation Babio = Batot − (Altot × Ba/Alalu) = Batot − (Altot × 0.0075), where Batot and Altot refer to the total Ba content and total Al content, respectively (Tribovillard et al. Reference Tribovillard, Riboulleau, Lyons and Baudin2004). Ba/Alalu indicates the Ba/Al ratios of aluminosilicate detritus in crustal rocks, which is commonly set to 0.0075 (Dymond et al. Reference Dymond, Suess and Lyle1992). Vanadium (V) tends to be fixed in sediments with organic compounds, and nickel (Ni) frequently precipitates as sulphides under anoxic conditions (Arthur & Sageman, Reference Arthur and Sageman1994). The V/(V + Ni) ratio positively correlates to reducibility (Arthur & Sageman, Reference Arthur and Sageman1994; Algeo & Ingall, Reference Algeo and Ingall2007). In a strongly reducing environment, uranium (U) exists in the form of insoluble U4+, enhancing the enrichment of U in sediments, whereas it exists in the form of soluble U6+ under oxidizing conditions. Thorium (Th) enrichment is not affected by the redox conditions of water bodies. Th/U ratio is a good indicator of redox conditions of bottom water, and correlates negatively to reducibility. (Davies & Elliot, Reference Davies and Elliot1996; Zhang et al. Reference Zhang, Jiang, Liang, Wu, Xian and Li2016).
4. Results
4.a. Mineralogy of the Es4s shale in the Dongying Depression
The Es4s sub-member predominantly comprises carbonate, clay minerals and quartz (Fig. 4a). Subordinate pyrite and feldspar were observed. The carbonate content (ranging from 3 to 95 wt % with an average of 51 wt %) mainly comprises calcite (ranging from 1 to 78 wt % with an average of 38 wt %) and dolomite (ranging from 1 to 93 wt % with an average of 14 wt %). The clay mineral content ranges from 2 to 57 wt % (average 16 wt %), with few samples exceeding 50 wt %. The clay minerals are mainly illite, followed by an illite–smectite mixed layer with trace chlorite. The quartz content ranges from 2 to 60 wt % with an average of 24 wt %. The clay mineral content and quartz content both show negative correlations with carbonate content (Fig. 4b–c).

Fig. 4. Ternary diagram of the mineral composition (a) and crossplots of carbonate content vs clay mineral content (b) and quartz content (c) of the Es4s sub-member in the Dongying Depression.
Three major carbonate forms occur, including micritic calcite/dolomite, granular calcite and columnar calcite. The micritic calcite/dolomite is made up with light laminae ranging in thickness from 60 to 400 μm (Fig. 5a–b), interlaced with dark laminae comprising clay minerals and trace organic matter (OM). The micritic calcite recrystallizes to granular calcite, which recrystallizes to columnar calcite as the degree of recrystallization increases (Fig. 5c–f; Liu et al. Reference Liu, Zhang, Song, Zhang, Hao, Xie, Xu and Liu2017; Bai et al. Reference Bai, Yu, Liu, Xie, Han, Zhang, Ye and Ge2018; Liang et al. Reference Liang, Cao, Liu, Jiang, Wu and Hao2018a). Granular calcite has a large particle size (5–20 μm in diameter) and can form light laminae alone or be distributed at the bottom of the micritic calcite laminae (Fig. 5c–d). The laminae comprising columnar calcite are bright and appear alternately with clay lamina enriched in OM (Fig. 5e). The maximum growth orientation of the columnar calcite particles is perpendicular to the laminae deposition plane. In addition, two subordinate forms of carbonate, including calcareous shell fragments and reworked detrital calcite, occur occasionally (Fig. 5g). The clay minerals are mostly mixed with OM to form dark laminae (Fig. 5b–e). The quartz particles have a sub-angular shape showing the characteristics of a terrigenous origin (Fig. 5h).
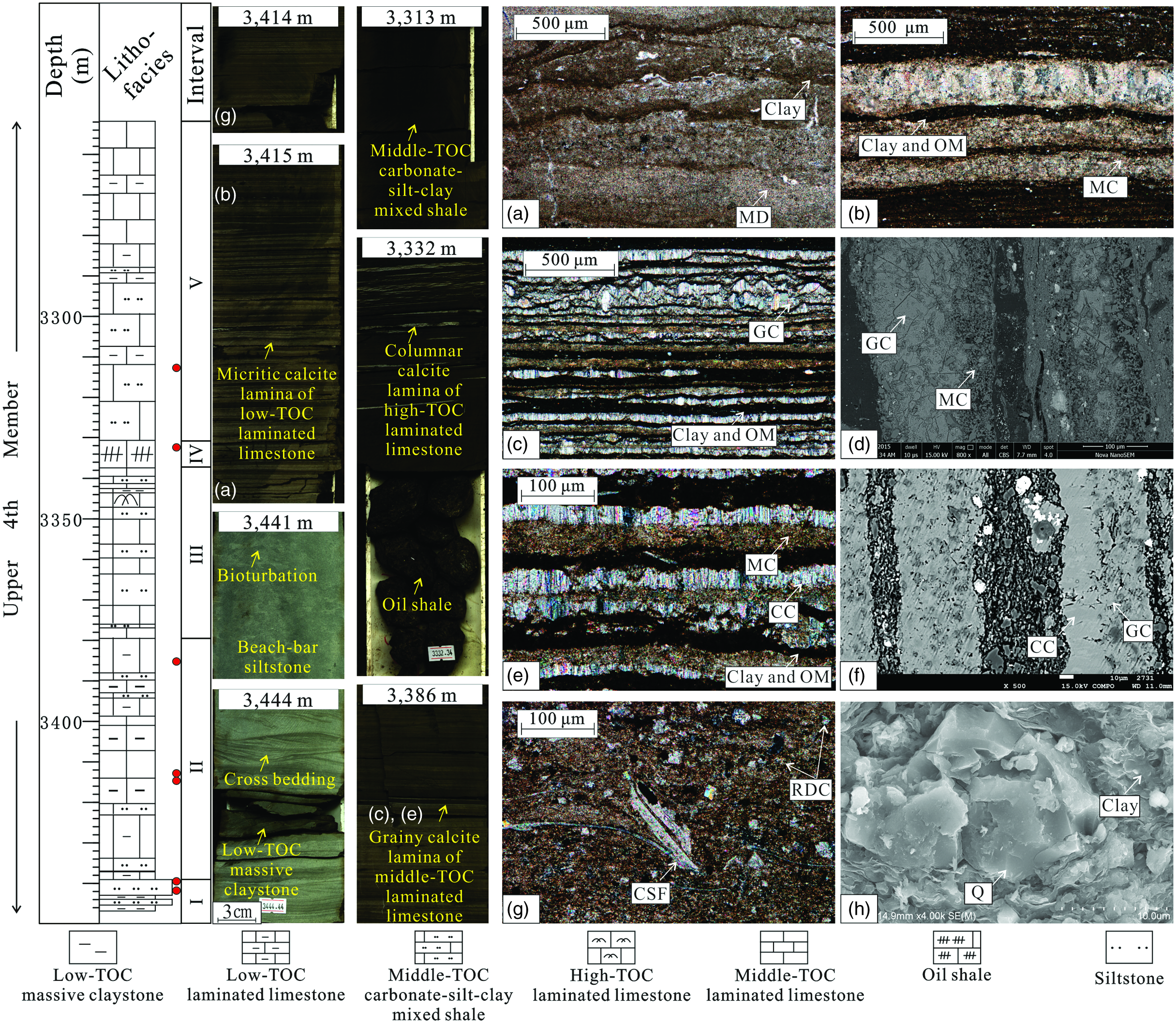
Fig. 5. Macroscopic and microscopic observations of minerals and lithofacies of the Es4s sub-member in the Dongying Depression. The location of samples in the core is marked by the red circle. (a) Light laminae comprise micritic dolomite (MD), Well FY1, 3415 m. (b) Micritic calcite (MC) in low-TOC laminated limestone, Well FY1, 3415 m. (c) Granular calcite (GC) constitutes light laminae, Well FY1, 3386 m. (d) Recrystallization from MC to GC, Well NY1 (Fig. 1c), 3453 m. (e) High-TOC laminated limestone; light laminae comprise columnar calcite (CC), and dark laminae comprise clay minerals and OM, Well FY1, 3386 m. (f) Recrystallization from GC to CC, Well NY1 (Fig. 1C), 3442 m. (g) Reworked detrital calcite (RDC) particles with certain abrasion and a diameter between 5 and 20 μm form massive bedding together with calcareous shell fragments (CSF) and clay minerals, Well FY1, 3414 m. (h) FESEM image showing quartz (Q) surrounded by clay minerals, Well FY1, 3308 m.
4.b. Geochemistry characteristics of the Es4s shale in the Dongying Depression
The Na/Al ratio varies between 0.08 and 0.29 (average 0.14), and the Babio content ranges from 58.1 to 845.8 µg g−1 (average 433.7 µg g−1). The Ti content ranges from 0.05 % to 0.55 %, with an average of 0.21 %, and the Sr/Ba ratio is high, in the range 1.27–12.80 (average 4.30). The V/(V + Ni) ratio ranges from 0.30 to 0.99, with an average of 0.75 (Fig. 6a). The Th/U ratio ranges from 0.35 to 5.85 (average 1.50), and its vertical variation trend is opposite to the vertical variation trend of the V/(V + Ni) ratio and TOC content (Fig. 6a).

Fig. 6. Vertical evolution (a) and relatively low carbonate content interval (b) of the Es4s sub-member in Well FY1 in the Dongying Depression.
The TOC content ranges from 0.11 to 9.05 wt % (average 2.21 wt %; Fig. 6a). The S1 values range between 0.02 and 6.04 mg g−1 (average 2.48 mg g−1), and the S2 values range from 0.01 to 41.08 mg g−1 (average 6.81 mg g−1; Fig. 6a). The chloroform bitumen ‘A’ content is primarily between 0.28 and 0.97, with an average of 0.58. The T max value ranges from 384 °C to 461 °C (average 447 °C), and the HI value ranges from 9.09 to 453.92 mg HC (hydrocarbons)/g TOC (average 296.04 mg HC/g TOC; Fig. 6a). Based on the HI and T max, the OM types are classified and dominated by type-II and type-II–III kerogen, with a few samples characterized by type-III–II and type-III kerogen. The vertical variation trends of S1, S2, chloroform bitumen ‘A’, T max and HI are similar to that of TOC content (Fig. 6a).
4.c. Petrology of the Es4s shale in the Dongying Depression
According to mineral compositions, TOC content (low TOC: TOC < 2 wt %; middle TOC: 2 wt % < TOC < 4 wt %; high TOC: TOC > 4 wt %) and sedimentary structures, seven lithofacies are identified, namely: (i) grey-blue siltstone; (ii) grey-blue low-TOC massive claystone; (iii) low-TOC laminated limestone; (iv) middle-TOC laminated limestone; (v) high-TOC laminated limestone; (vi) middle-TOC carbonate–silt–clay mixed shale (middle-TOC MS); and (vii) oil shale (Fig. 5). The grey-blue siltstone is characterized by the development of cross-bedding, massive bedding, ripple bedding and bioturbation. The siltstone is developed with a certain degree of hydrodynamism and may be of beach-bar or delta front deposition. The grey-blue low-TOC massive claystone, along with siltstone, only develops at the bottom of the Es4s sub-member with high clay mineral content (greater than 50 wt %). Based on the aforementioned characteristics of mineral compositions, texture, sedimentary structures, TOC content, lithofacies and sedimentary facies distribution (Fig. 1c), the co-development of these two types of lithofacies indicates a nearshore area. Low-TOC laminated limestone, middle-TOC laminated limestone and high-TOC laminated limestone are commonly carbonate-rich (greater than 50 wt %) lithofacies and are characterized by well-developed laminae suggesting a weak influence of terrigenous input and hydrodynamism. The light laminae of these three lithofacies comprise micritic, granular and columnar calcite, respectively. The dark laminae mainly contain clay minerals and OM. With the increase in TOC content, the core colour of these three lithofacies darkens from grey to black, suggesting a gradual deepening of the water body. Middle-TOC MS is developed in the upper part of the Es4s sub-member and differs from middle-TOC laminated limestone by a relative increase in terrigenous material (e.g. quartz and feldspar), indicating a shallow environment. The average content of quartz and feldspar is 32 wt %. The average content of carbonate decreases to 42 wt %. The clay mineral content ranges from 11 to 35 wt % (average 22 wt %). Oil shale is mainly developed in deep lake, suggested by the black colour in the core, high content of TOC (greater than 4 wt %), and lack of terrigenous material.
4.d. Vertical sedimentary evolution in the Dongying Depression
Based on mineral composition, lithofacies and geochemical data, five intervals (I, II, III, IV and V) are determined from the bottom to the top of the Es4s sub-member.
Interval I (3447–3439 m). The lithofacies are dominated by low-TOC massive claystone and siltstone with cross-bedding and intense bioturbation (Fig. 5). The carbonate content and TOC content are the lowest (averages 12 and 0.11 wt %, respectively; Figs 6a and 7) in the entire Es4s sub-member. In contrast, the Th/U ratio is high and increases upward. The aforementioned characteristics suggest that sediments influenced by the terrigenous input in this interval were deposited in the nearshore area (Jiang et al. Reference Jiang, Liu, Zhang, Su and Jiang2011).

Fig. 7. Contents of carbonate (a) and TOC (b) in five intervals of the Es4s sub-member in the Dongying Depression.
Interval II (3439–3380 m). A large amount of carbonate developed in this interval (Figs 6a and 7a). Although the carbonate content frequently fluctuates, it mostly exceeds 50 wt % (Fig. 6a). The average contents of calcite and dolomite reach a maximum. The content of quartz and clay minerals is low (Fig. 6a). The lithofacies is dominated by low-TOC laminated limestone, interlaced with middle-TOC laminated limestone and middle-TOC MS. The content of TOC (average 2.25 wt %) as well as pyrite and the V/(V + Ni) ratio (average 0.93) increases (Figs 6a and 7b), which are all positively proportional to reducibility; these are accompanied by a drastic decrease in Th/U ratio compared with that of interval I. These characteristics suggest that the water body deepened.
Interval III (3380–3337 m). The carbonate content greatly fluctuates, ranging from 3 to 94 wt %, with an average of 48 wt % (Figs 6a and 7a). Low-carbonate-content samples appear at 3356–3359 and 3364–3372 m. The average contents of calcite and dolomite decrease to 33 % and 15 %, respectively. The dominant lithofacies changes from low-TOC laminated limestone of shallow lake facies in interval II to middle-TOC MS and middle/high-TOC laminated limestone of semi-deep lake facies in interval III. The high content of TOC and pyrite, as well as the V/(V + Ni) ratio (ranging from 0.89 to 1.00, average 0.96), suggest that the water body continued to deepen.
Interval IV (3337–3332 m). The carbonate content considerably decreases, ranging from 3 to 49 wt %, with an average of 24 wt % (Fig. 7b). The average calcite and dolomite contents decrease to their lowest value (19 and 6 wt %, respectively). The TOC content is the highest (maximum 9.05 wt %, average 3.97 wt %) in the entire Es4s sub-member, and oil shale of deep-lake facies is developed. The V/(V + Ni) ratio and pyrite content reach a maximum, indicating the deepest water level (Fig. 6b). These characteristics suggest that the carbonate content decreases to a minimum in the deep lake.
Interval V (3332–3252 m). The carbonate content increases again (Fig. 6a), ranging from 5 to 95 wt % (average 52 wt %). The average values of calcite and dolomite increase to 44 and 8 wt %, respectively. The dominant lithofacies changes from middle-TOC MS to low-TOC laminated limestone. The Th/U ratio starts to increase, and the TOC content (ranging from 1.29 to 3.79 wt %, average 2.08 wt %), V/(V + Ni) ratio (ranging from 0.31 to 0.98, average 0.63) and pyrite content are all lower than those in interval IV, suggesting a shallowing trend of the water body.
4.e. Distribution of carbonate and lithofacies in the Dongying Depression
Based on the seismic data, well log data and core observations, the Es4s sub-member is interpreted as one entire third-order sequence (Fig. 8, SQ1; Tian & Jiang, Reference Tian and Jiang2009; Zahid et al. Reference Zahid, Chunmei, Lin, Gluyas, Jones, Zhang, Munawar and Ma2016), including the lowstand system tract (LST), transgressive system tract (TST) and highstand system tract (HST). According to the cross-section within the sequence-stratigraphic framework, the distributions of carbonate content and lithofacies were analysed (Fig. 8). Affected by strong terrigenous input, the carbonate content of interval I (LST) is low. Conglomerates are developed in the northern steep slope (Fig. 8, Well S54; Li et al. Reference Li, Yang, Wang, Zhang, Luo, Liu, Zhang and Luo2019), and siltstone and low-TOC massive claystone with a small amount of gypseous claystone are developed in the central area of the basin (Fig. 8, Well NY1). Throughout interval II (early TST), the dominant lithofacies is sandstone and claystone in the northern basin edge, whose lateral distribution is limited and changes rapidly. The central area of the basin is far away from the sediment input. The lithofacies is dominated by low-TOC laminated limestone with a few thin layers of middle-TOC MS (Fig. 8, Well FY1). Variations in the lithofacies association and high values of TOC, pyrite and V/(V + Ni) ratio indicate that the water body deepens during interval III. The dominant lithofacies changes from siliciclastics in the northern basin edge to middle-TOC MS and middle-TOC laminated limestone toward the centre of the basin. During interval IV (late TST), with the deepening of the water body, the carbonate content considerably decreases in the central basin (Fig. 8; Well LY1, FY1 and NY1). However, the carbonate content increases in the low-TOC laminated limestone of the north basin (Fig. 8, Well B666). Sandstone (Fig. 8, Well S54), claystone, low-TOC laminated limestone (Fig. 8, Well B666), middle-TOC MS (Fig. 8, Well LY1) and oil shale (Fig. 8, Well FY1 and NY1) are successively developed from the edge to the centre of the basin as the water body deepens. In interval V (HST), the change in carbonate content and lithofacies from the edge to the centre of the basin is similar to interval III.

Fig. 8. Lithofacies cross-section of the Es4s sub-member in the Dongying Depression. See Figure 1c for the location of the cross-section.
Based on the aforementioned details, carbonates hardly precipitate in the nearshore area, where sedimentation is dominated by terrigenous inputs. However, the carbonate content is high in the shallow lake, where the influence of terrigenous input is reduced and the lithofacies is dominated by low-TOC laminated limestone. As the water body deepens, the carbonate content gradually decreases and the deposition mainly comprises middle-TOC laminated limestone and high-TOC laminated limestone. The carbonate content is minimal in the deep lake, where the lithofacies is dominated by oil shale.
5. Discussion
5.a. A comparison of the Eocene and Holocene lacustrine carbonate deposition
5.a.1. Vertical evolution of lacustrine carbonates
The varying patterns of carbonates (VPC) and sedimentary environments of four modern lakes are investigated based on their lithofacies, mineral content, TOC content and grain size.
Hole 1F was drilled in the deposition centre of the southwestern sub-basin of Qinghai Lake (An et al. Reference An, Colman, Zhou, Li, Brown, Timothy Jull, Cai, Huang, Lu, Chang, Song, Sun, Xu, Liu, Jin, Liu, Cheng, Liu, Ai, Li, Liu, Yan, Shi, Wang, Wu, Qiang, Dong, Lu and Xu2012). We divided the sediments retrieved from hole 1F into four intervals in this study: I, II, III and IV (Fig. 9). The sediments of interval I (18.61–6.68 m) change from khaki fine sand to silt and silty mud. The carbonate content is low, whereas the quartz content is obviously higher than in the other intervals. The TOC flux content is less than 1 g cm−2 kyr−1. The mean grain size of this interval is the largest, ranging from 6 to 75 μm (average 36 μm). The low TOC flux content and large grains of sediments suggest that the water body is shallow in this interval (Roeser et al. Reference Roeser, Franz and Litt2016), which may be affected by the arid and cold climate during the last glacial episode (Yu & Kelts, Reference Yu and Kelts2002; An et al. Reference An, Colman, Zhou, Li, Brown, Timothy Jull, Cai, Huang, Lu, Chang, Song, Sun, Xu, Liu, Jin, Liu, Cheng, Liu, Ai, Li, Liu, Yan, Shi, Wang, Wu, Qiang, Dong, Lu and Xu2012). In interval II (6.68–5.22 m), grey silty mud is well developed. The carbonate content abruptly increases (c. 50 wt %) as a result of aragonite precipitation. The TOC flux content considerably increases to 3 g cm−2 kyr−1, which indicates a deepening water body, combined with the variation in lithology and grain size. The sediments in Qinghai Lake are relatively coarse, and the water body is shallower with stronger terrigenous clastic input compared with the Dongying Depression. The VPC and variation in water body in intervals I and II (18.61–5.22 m) in Qinghai Lake are similar to those of intervals I and II recorded in the Dongying Depression (Figs 6 and 9). The sediments of interval III (5.22–3.78 m) change from light-grey silty mud to grey silty mud, with decreasing grain size. Compared with interval II, even though the content of aragonite and dolomite increases in interval III, the total carbonate content decreases. The TOC flux content remains at a stable, high value (c. 3 g cm−2 kyr−1). Black-grey mud is dominant in interval IV (3.78–0 m). Both quartz content and mean grain size are low (c. 10 wt % and 16 μm, respectively). The water depth is at the maximum, and the carbonate content continues to decrease and then stabilizes, with a high TOC flux content. The VPC in intervals III and IV are similar to those of intervals III and IV in the Dongying Depression, both of which show a deepening water body, decreased carbonate content and smaller sediment grain sizes compared with those of intervals I and II.

Fig. 9. Vertical evolution of Holocene deposition in hole 1F in Qinghai Lake, modified from Qian & Song (Reference Qian and Song2010) and An et al. (Reference An, Colman, Zhou, Li, Brown, Timothy Jull, Cai, Huang, Lu, Chang, Song, Sun, Xu, Liu, Jin, Liu, Cheng, Liu, Ai, Li, Liu, Yan, Shi, Wang, Wu, Qiang, Dong, Lu and Xu2012).
Similar VPC are developed in Barkol Lake and Oro Lake (Figs 10 and 11), the sediments of which are divided into five and three intervals in this study, respectively. The carbonate content is low in interval I due to the presence of sandy/gypseous sediments. The amount of carbonate increases abruptly in interval II and decreases in interval III with a deepening water body, as inferred by an enrichment in OM. The carbonate content remains low in interval IV with the deepest water body, as indicated by the minimum sediment grain size, while it increases again in interval V.

Fig. 10. Holocene deposition in hole 2F in Barkol Lake, modified from Li et al. (Reference Li, Lv, Tao and An2008).

Fig. 11. Content and texture characteristics of sediments in OR2 in Oro Lake, modified from Last & Vance (Reference Last and Vance2002).
The VPC, which are comparable to the Dongying Depression, appear in the sediments of Montcortès Lake (Figs 3g and 12). The water body gradually shallows (intervals III–V) after a short deepening (intervals I and II), which is influenced by the basin morphology, hydrologic fluctuations and climate (Valero-Garcés et al. Reference Valero-Garcés, Morellón, Moreno, Corella, Martín-Puertas, Barreiro, Pérez, Giralt and Mata-Campo2014). The water body then shallows again (interval VII) after a slight deepening (interval VI). Correspondingly, the carbonate content is low in intervals I and II, and then gradually increases in intervals III–V. The amount of carbonate decreases in interval V and slightly increases in intervals VI and VII before decreasing again. Although the sequence of sediments differs from the Dongying Depression, the correspondence between carbonate deposition and sedimentary environment is consistent.

Fig. 12. Contents of sediments in 1A and 4A in Montcortès Lake, modified from Valero-Garcés et al. (Reference Valero-Garcés, Morellón, Moreno, Corella, Martín-Puertas, Barreiro, Pérez, Giralt and Mata-Campo2014).
5.a.2. Distribution of lacustrine carbonates
The evolution of carbonate content from the basin edge to the centre in the north–south and southeast–northwest directions in Barkol Lake is illustrated in Figure 13 (Zhao et al. Reference Zhao, Lv, An, Tao, Zheng and Dong2010). Taking the transect BB′ as an example, the carbonate content increases from 21 wt % at BB′1 to 32 % at BB′7 and decreases to 19 wt % at BB′9 at the basin centre (Fig. 13b). The lateral distribution of the Holocene deposition shows that the carbonate content gradually increases to a maximum from the edge of the basin to its centre and then decreases at the deepest water zone, which is consistent with VPC in the Dongying Depression (Figs 6a and 8).

Fig. 13. Distribution of the Holocene lacustrine carbonates in AA′ (a) and BB′ (b) in Barkol Lake, modified from Zhao et al. (Reference Zhao, Lv, An, Tao, Zheng and Dong2010).
5.b. Genetic mechanisms of variation in carbonate
According to the VPC and sedimentary environment, four distinct lake stages are determined: terrigenous clastic / gypsum-rich stage (T/GRS), carbonate-rich stage (CRS), carbonate-decreasing stage (CDS) and carbonate-poor stage (CPS), corresponding to intervals I, II, III and IV, respectively.
T/GRS (Interval I): The terrigenous input is strong, as indicated by the high content of Ti, which enhances the enrichment of silt, hindering carbonate precipitation (Figs 8, 14 and 15; Bomou et al. Reference Bomou, Adatte, Tantawy, Mort, Fleitmann, Huang and Föllmi2013; Montero-Serrano et al. Reference Montero-Serrano, Föllmi, Adatte, Spangenberg, Tribovillard, Fantasia and Suan2015). Simultaneously, gypsum is developed by evaporation under an arid climate condition in some areas lacking terrigenous clastic (Fig. 8, Well NY1; Liang et al. Reference Liang, Jiang, Cao, Wu, Wang and Fang2018 b).

Fig. 14. Controlling factors of carbonate deposition in the Es4s sub-member in Well FY1.

Fig. 15. Depositional model of the Es4s sub-member in the Dongying Depression.
CRS (Interval II): The terrigenous input greatly decreases, reducing the dilution effect of terrigenous materials and providing suitable conditions for carbonate precipitation. Based on the evaporative setting in interval I, a decreasing Na/Al ratio and a high Sr/Ba ratio indicate a warm climate and salinized water body in interval II. Such depositional conditions favour the chemical precipitation of calcite (Last & Vance, Reference Last and Vance2002; Romero-Viana et al. Reference Romero-Viana, Julia, Camacho, Vicente and Miracle2008). The increasing Babio content indicates an enhancement in primary productivity, and the OM changes from type-III–II kerogen to type-II kerogen, suggesting an increase in planktonic algae content (Fig. 6a; Tribovillard et al. Reference Tribovillard, Riboulleau, Lyons and Baudin2004; Liu & Wang, Reference Liu and Wang2013). Planktonic algae use free CO2 and HCO3− for photosynthesis, forming CO32− (Fig. 15; Felfoldy, Reference Felfoldy1960; Han et al. Reference Han, Yu, Zhao, Zhao, Tucker and Yan2018) and secreting calcium carbonate (Robbins & Blaekwelder, Reference Robbins and Blaekwelder1992; Hodell et al. Reference Hodell, Sehelske, Fahnenstiel and Robbins1998; Singh et al. Reference Singh, Saraswat, Naik and Nigam2017). Favourable chemical conditions of water body and biological processes together contribute to carbonate enrichment in this interval, corresponding to chemically and biochemically derived carbonate, respectively. This enrichment process of carbonate can be indirectly suggested by the negative correlations of carbonate content with the terrigenous clay minerals and quartz, as shown in Figure b–c.
CDS (Interval III): The lower ratios of Na/Al, Sr/Ba and Th/U indicate that the climate was warmer and wetter than during interval II, which brings a large volume of fresh water into the lake, leading to a further deepening of the water body as well as a decrease in salinity (Fig. 14). These conditions are not conducive to the chemical precipitation of carbonate (Romero-Viana et al. Reference Romero-Viana, Julia, Camacho, Vicente and Miracle2008). However, in this interval, milder temperatures enhance the lake productivity, and the OM is dominated by type-II kerogen with more planktonic algae (Fig. 6a), which favour the biochemical precipitation of carbonate (Roeser et al. Reference Roeser, Franz and Litt2016). Compared with interval II, even though biochemically derived carbonate increases, chemically derived carbonate decreases, leading to a decrease in total carbonate.
CPS (Interval IV): The warmest and wettest climate leads to high primary productivity, strong freshwater input and low salinity, inferred from the lowest ratios of Na/Al and Sr/Ba and the highest ratio of V/(V + Ni). The chemical precipitation of carbonate continues to decrease. In addition, a thermal stratification of the water body can be formed under this climate condition (Wagner & Adrian, Reference Wagner and Adrian2011), reducing the mixing of water and material between the upper and lower layers in the lake (O’Reilly et al. Reference O’Reilly, Alin, Plisnier, Cohen and McKee2003; Stainsby et al. Reference Stainsby, Winter, Jarjanazi, Paterson, Evans and Young2011). The dissolved oxygen in the surface water does not easily penetrate to the bottom of the lake, resulting in a long-term anoxic condition that favours OM enrichment (Jankowski et al. Reference Jankowski, Livingstone, Bührer, Forster and Niederhauser2006). The degradation of OM at the water bottom produces CO2 and organic acid, affording a decrease in water pH and carbonate dissolution (Fig. 15; Emerson & Berder, Reference Emerson and Berder1981; Milliman et al. Reference Milliman, Troy, Balch, Adams, Li and Mackenzie1999; Mercedes-Martín et al. Reference Mercedes-Martín, Rogerson, Brasier, Vonhof, Prior, Fellows, Reijmer, Billing and Pedley2016). A relatively reduced carbonate supply and increased carbonate dissolution lead to the formation of the CPS.
Interval V: A considerably decreased V/(V + Ni) ratio and increased Th/U ratio indicate a reduction in the water depth. The salinity of the water body is similar to that of interval III (Fig. 14). The OM is dominated by type-II and type-II–III kerogen and is associated with relatively high primary productivity. Due to the effects of biology and biochemistry, carbonate is relatively enriched again.
A lake is a complex and varied dynamic system, in which detrital influx, climate, primary productivity and salinity all influence carbonate precipitation and dissolution, resulting in a variation in carbonate.
5.c. Formation conditions of VPC developed in this study
As demonstrated by the analysis of the Holocene carbonate deposition in modern lakes, the development of VPC in the Dongying Depression requires certain conditions, including but not limited to the following. (1) Sufficient carbonate from the weathering of the carbonate-rich provenance and calcareous organisms. (2) A particular water depth to ensure that light can penetrate for photosynthesis to facilitate carbonate precipitation, and to limit the effect of wind mixing, i.e. free from the exchange of the upper and lower water bodies (Schubel & Lowenstein, Reference Schubel and Lowenstein1997; Quijada et al. Reference Quijada, Suarez-Gonzalez, Benito and Mas2013). For example, the maximum water depth is only c. 4–5 m in interval III at the Oro Lake (Fig. 11, Last & Vance, Reference Last and Vance2002) and the carbonate content decreases mildly in the carbonate-decreasing stage. The carbonate-poor stage (CPS) is absent with the lack of sufficient water depth, which is different from the Dongying Depression. (3) Appropriate salinity is helpful to the formation of the carbonate-rich stage. For example, in the hypersaline Dead Sea, the carbonate precipitates mainly during a period of high water level, whereas during a period of shallow water the salinity increases, and gypsum and halite become the dominant precipitating minerals (Golan et al. Reference Golan, Lazar, Wurgaft, Lensky, Ganor and Gavrieli2017). (4) A closed lake will increase salinity and promote the formation of thermodynamic stratification, which result in an anoxic condition at the lake bottom (Liang et al. Reference Liang, Jiang, Cao, Wu, Wang and Fang2018b) that is helpful for OM enrichment. As mentioned above, the degradation of abundant OM acts as a crucial factor for the development of CPS in lakes. When the integrated conditions of the lake are appropriate, VPC similar to those in the Dongying Depression can appear in lakes Qinghai, Barkol and Montcortès.
5.d. Influence of carbonate variation
Organic matter enrichment, which is important to petroleum resources, is different in the distinct lake stages. The average TOC content is 2.3 wt % in the carbonate-rich stage (CRS), whereas it is 2.1 wt % in the carbonate-decreasing stage (CDS), with a higher average value of S1 + S2 (Fig. 16). The content of TOC, S1 + S2, and bitumen ‘A’ is highest in the carbonate-poor stage (CPS). The heterogeneous distribution of organic geochemical parameters is attributable to the different sedimentary conditions of the lake stages. The primary productivity is low, and oxygen content is relatively high, leading to poor conditions for OM preservation in the CRS (Figs 14 and 15), which makes OM accumulation difficult (Schieber, Reference Schieber2011). Both primary productivity and reducibility increase in the CDS. The proportion of type-II kerogen increases from 50 % in the CRS to 80 %, and the hydrocarbon generation efficiency of OM increases, resulting in more OM turning into S1 and S2 (Fig. 16). The primary productivity is high, and the preservation conditions for OM are favourable, with low oxygen content in the CPS (Stow et al. Reference Stow, Hue and Bertrand2001). This is the optimal interval for OM enrichment. In addition, carbonate deposition, which influences the heterogeneous enrichment of OM, further affects the variation in carbonate crystal forms (from micritic to sparry calcite) as a result of organic–inorganic interactions during the thermal evolution of OM, particularly in the lamina-developed lithofacies (Liang et al. Reference Liang, Cao, Liu, Jiang, Wu and Hao2018 a).

Fig. 16. The parameters of the CRS, CDS and CPS of the Es4s sub-member in the Dongying Depression.
6. Conclusions
Four evolutionary stages were identified in the Eocene carbonate-rich Dongying Depression (China). During the terrigenous clastic / gypsum-rich stage, carbonates hardly precipitate owing to the influence of silty/gypseous sediments. During the carbonate-rich stage, large amounts of chemically and biochemically derived carbonate precipitate as the water body deepens. During the carbonate-decreasing stage, the continuous deepening and reduced salinity of the water body affected by a warm and humid climate yield a decrease in chemically derived carbonate. During the carbonate-poor stage, the degradation of organic matter promotes carbonate dissolution in the deep part of the lake. Similar varying patterns of carbonates (VPC) are observed in four Holocene lakes (the Qinghai Lake and Barkol Lake in China, Oro Lake in Canada, and Montcortès Lake in Spain). The development of VPC in the Dongying Depression requires certain conditions, including but not limited to a sufficient carbonate source, a particular water depth, an appropriate salinity and a relatively closed lake. A depositional model of carbonates in the Dongying Depression is developed based on the comparison of VPC in ancient and modern lakes. The findings of this study deepen the understanding of the diversity and genetic mechanisms of lacustrine carbonates.
Acknowledgements
The results discussed in this paper were supported by the Natural Science Foundation of Shandong Province (No. ZR2019BD042), the National Natural Science Foundation of China (No. 42072164, No. U1762217, No. 41902134 and No. 42172165), the Taishan Scholars Program (No. TSQN201812030), the Fundamental Research Funds for the Central Universities (19CX07003A) and the China Postdoctoral Science Foundation Grant (No. 2019M652435 and No. 2020T130384). Our deepest gratitude goes to Editor-in-Chief Peter Clift, Associate Editor J. Le Goff, reviewer C. Ian and an anonymous reviewer, and Editor Susie Cox and Susie Bloor for their excellent work and thoughtful suggestions that have helped to improve this manuscript considerably.
Declaration of Interest
None.