Introduction
Nitric oxide (NO) is primarily synthesized by the action of nitric oxide synthase (NOS), which converts arginine into citrulline and NO in an NADPH- and O2-dependent manner. Three isoforms of this enzyme provide a wide range of concentration and temporal NO profiles in mammals. Two of these isoforms are constitutive (NOS1 or neuronal nNOS and NOS3 or endothelial eNOS), while the third is inducible (NOS2 or iNOS) (Stuehr et al., Reference Stuehr, Santolini, Wang, Wei and Adak2004). However, the specific involvement of the NOS/NO system in events that regulate bovine oocyte maturation has not been entirely elucidated (Viana et al., Reference Viana, Caldas-Bussiere, Matta, Faes, Paes de Carvalho and Quirino2007; Matta et al., Reference Matta, Caldas-Bussiere, Viana, Faes, Paes de Carvalho, Dias and Quirino2009; Schwarz et al., Reference Schwarz, Pires, Mesquita, Chiaratti and Leal2014; Botigelli et al., Reference Botigelli, Schwarz, Zaffalon, Del Collado, Castro, Fernandes and Leal2017; Dubeibe et al., Reference Dubeibe, Caldas-Bussiere, Maciel, Sampaio, Quirino, Gonçalves, De Cesaro, Faes and Paes de Carvalho2017).
In in vitro conventional oocyte culture systems, the inhibition of NOS activity by l-NAME, an analogue of arginine that inhibits NO production (Matta et al., Reference Matta, Caldas-Bussiere, Viana and Quirino2002; Schwarz et al., Reference Schwarz, Pires, de Bem, Adona and Leal2010), or aminoguanidine, a specific inhibitor of the iNOS (Matta et al., Reference Matta, Caldas-Bussiere, Viana, Faes, Paes de Carvalho, Dias and Quirino2009), blocks meiotic resumption and progression to metaphase II (MII). Therefore, these observations suggest that the NOS/NO system regulates meiosis in cattle (Matta et al., Reference Matta, Caldas-Bussiere, Viana and Quirino2002, Reference Matta, Caldas-Bussiere, Viana, Faes, Paes de Carvalho, Dias and Quirino2009; Schwarz et al., Reference Schwarz, Pires, de Bem, Adona and Leal2010). Nevertheless, the effects of NO during in vitro oocyte maturation are concentration based, i.e. using NO donors at different concentration, nuclear maturation may be inhibited [10–3 M sodium nitroprusside (SNP), Viana et al., Reference Viana, Caldas-Bussiere, Matta, Faes, Paes de Carvalho and Quirino2007; and 10–5 M and 10–3 M snitroso-N-acetyl-1,1-penicillamine (SNAP), Schwarz et al., Reference Schwarz, Pires, Adona, De Bem and Leal2008] or cytoplasmic maturation stimulated (10–5 M SNP; Viana et al., Reference Viana, Caldas-Bussiere, Matta, Faes, Paes de Carvalho and Quirino2007, Reference Viana, Caldas-Bussiere, Paes de Carvalho, Dias, Faes, Lanes, Quirino and Escocard2010, Reference Viana, Caldas-Bussiere, Paes de Carvalho, Dias, Lanes and Quirino2011).
In the same way, the effects of the NOS/NO system in tissues may be toxic or protective depending on their activity, concentration, type, and degree of cellular differentiation (Basini et al., Reference Basini, Baratta, Ponderato, Bussolati and Tamanini1998). Specifically, NO produced endogenously in follicles regulates cell viability (Faes et al., Reference Faes, Caldas-Bussiere, Viana, Dias, Costa and Escocard2009; Tripathi et al., Reference Tripathi, Khatun, Pandey, Mishra, Chaube, Shrivastav and Chaube2009; Zamberlam et al., Reference Zamberlam, Portela, de Oliveira, Gonçalves and Price2011) and plays a role in the production of steroids by granulosa cells (Basini et al., Reference Basini, Baratta, Ponderato, Bussolati and Tamanini1998; Faes et al., Reference Faes, Caldas-Bussiere, Viana, Dias, Costa and Escocard2009). Therefore, in some circumstances, NO acts as a local modulator of follicular cell activity.
The presence of follicular wall cells in culture, especially theca cells, partially blocks nuclear maturation in bovine cumulus–oocyte complexes (COCs) (Richard and Sirard, Reference Richard and Sirard1996), even in the presence of follicle-stimulating hormone (FSH) (Van Tol and Bevers, Reference Van Tol and Bevers1998) and luteinizing hormone (LH) (De Cesaro et al., Reference De Cesaro, Trois, Gutierrez, Siqueira, Rigo, Glanzner, Oliveira and Gonçalves2013). Therefore, a model involving the culture of COCs with follicular wall hemisections (HSs) has been used to evaluate the factors and interactions that regulate oocyte nuclear maturation and the competency of follicular cells (De Cesaro et al., Reference De Cesaro, Trois, Gutierrez, Siqueira, Rigo, Glanzner, Oliveira and Gonçalves2013; Dubeibe et al., Reference Dubeibe, Caldas-Bussiere, Maciel, Sampaio, Quirino, Gonçalves, De Cesaro, Faes and Paes de Carvalho2017).
Despite the current efforts in understanding nuclear maturation of bovine oocytes, there is a lack of knowledge on signalling events that regulate meiosis resumption and progression, cellular integrity and steroidogenesis in COCs. Moreover, these events should be partially orchestrated by the NOS/NO/cGMP signalling pathway. In this way, the aim of the present study was to assess the effects of NOS inhibition by addition of l-NAME (Moncada et al., Reference Moncada, Palmer and Higgs1991) on these parameters during nuclear maturation in COCs co-cultured in vitro with HSs.
Materials and methods
Unless otherwise noted, all reagents used in these experiments were obtained from Sigma-Aldrich Brasil Ltd (São Paulo, Brazil).
Isolation of follicular wall hemisections
Bovine ovaries were collected from adult crossbreed cows in different stages of the estrous cycle at a local abattoir immediately after slaughter. The ovaries were then carried to the laboratory within 2 h in sterile saline solution containing antibiotics (100 IU/ml potassium penicillin G and 50 µg/ml streptomycin sulfate) at 30°C. Follicles were isolated from the ovary, dissected from the stromal tissue, and were selected based on size (3–5 mm) and transparency (Leibfried and First, Reference Leibfried and First1979). These follicles were cut into equal halves (Richard and Sirard, Reference Richard and Sirard1996). The HSs were washed three times in washing medium (tissue culture medium 199 (TCM 199–HEPES), in addition to 0.4% fatty acid-free bovine serum albumin (BSA-FAF), 0.2 mM pyruvate, 100 IU/ml potassium penicillin G, and 50 µg/ml streptomycin sulfate. At 2 h before the addition of COCs, eight HSs were transferred to 4-well plates (NUNC, Rochester, NY, USA) containing 200 µl of maturation medium [TCM 199 supplemented with 0.4% BSA (fatty acid free), 0.5 μg/ml FSH (Follitropin-V; Bioniche Life Science Inc., Canada), 5 μg/ml LH (Lutropin-V, Bioniche Life Science Inc., Canada), 0.2 mM pyruvate, and antibiotics] for each of the treatments (Dubeibe et al., Reference Dubeibe, Caldas-Bussiere, Maciel, Sampaio, Quirino, Gonçalves, De Cesaro, Faes and Paes de Carvalho2017) and, according to the experiment, maturation medium was supplemented with 0.1, 0.5, 1.0, 2.5, 5.0 or 10.0 mM l-NAME. Controls were used without l-NAME supplementation, but were constituted by the addition of follicular HSs (+HSs) or not (–HSs).
Collection and cultivation of cumulus–oocyte complexes
Here 3- to 8-mm follicles were aspirated, and the collected COCs were immediately placed in TCM washing medium supplemented with 3-isobutyl-1-methylxanthine (IBMX; 0.5 mM) (Eppig and Downs, Reference Eppig and Downs1984). IBMX, a reversible broad-spectrum PDE inhibitor (Francis et al., Reference Francis, Blount and Corbin2011) was used to inhibit spontaneous resumption of meiosis in bovine oocytes during collection and handling of COCs (Dubeibe et al., Reference Dubeibe, Caldas-Bussiere, Maciel, Sampaio, Quirino, Gonçalves, De Cesaro, Faes and Paes de Carvalho2017). After selection, the diplotene-arrested oocytes were washed with TCM 199 medium to remove IBMX from the culture medium and were used for in vitro studies. The average time for collection and preparation of culture was 30 min. Only COCs with more than three layers of CCs and a homogeneous ooplasm (Leibfried and First, Reference Leibfried and First1979) were selected and transferred to plates containing the HSs. Cultivation was performed in an incubator at 38.5°C in an atmosphere containing 5% CO2 in air at 95% relative humidity for 22 h.
Assessment of nuclear maturation
Oocytes were denuded by repeated pipetting in a solution of phosphate-buffered saline (PBS) with 0.1% polyvinyl alcohol, subsequently mounted between a slide and a coverslip, fixed in a 3:1 solution of ethanol:acetic acid (Merck Industries SA, Rio de Janeiro, Brazil) for at least 22 h, and finally stained with 2% acetic orcein. The nuclear maturation stage of each oocyte was assessed through an inverted microscope (×400, NIKON – Eclipse TE300, Melville, Japan). Oocytes were classified as germinal vesicle (GV), metaphase I (MI), or MII (Fig. S1).
Assessment of cumulus cell plasma membrane integrity
After maturation, COCs were denuded mechanically, and oocytes were removed from the medium. The CCs were centrifuged (700 g) for 10 min and resuspended in PBS/5% fetal calf serum (FCS)-containing stains. Double staining with fluorescent markers Hoechst 33342 (excitation max at 346 nm and emission max at 460 nm), which labels cells with intact membranes (blue staining), and propidium iodide (excitation max at 632 nm and emission max at 493 nm), which labels cells with damaged membranes (red staining), was used to observe plasma membrane integrity of CCs (Viana et al., Reference Viana, Caldas-Bussiere, Paes de Carvalho, Dias, Lanes and Quirino2011; Dubeibe et al., Reference Dubeibe, Caldas-Bussiere, Maciel, Sampaio, Quirino, Gonçalves, De Cesaro, Faes and Paes de Carvalho2017). The cells were exposed to each of the markers for 5 min and then placed between a slide and a cover slip. The slides were analyzed (200 cells/treatment) under an epifluorescence microscope (×400 magnification, NIKON – Eclipse TE300, Melville, USA). The results were expressed as the proportion of cells with an intact or a damaged membrane.
Measurement of nitrate/nitrite concentrations
NO concentration was evaluated indirectly by measuring the nitrate/nitrite (NO3−/NO2−) concentrations and stable NO metabolites in the culture medium, as NO has a very short half-life. The maturation media containing the different treatments were centrifuged (2700 g for 5 min), stored (50 µl), and maintained at −20°C for later measurement using the Griess colorimetric reaction (Ricart-Jané et al., Reference Ricart-Jané, Llobera and López-Tejero2002). To transform NO3– into NO2–, the samples were incubated in 96-well plates with reducing solution [10 IU NO3– reductase enzyme diluted in ultrapure water + 900 µl of ultrapure water, 1000 µl of the NADPH cofactor (5 mg/ml) diluted in ultrapure water, 1000 µl of potassium phosphate buffer solution (0.5 M)] at 37°C for 16 h. The standard curve for NaNO3 was accessed through its dilution in TCM 199 medium at concentrations ranging from 0.5 to 100 µM. The reading was performed on a spectrophotometer (ELISA reader, BioTek, Winooski, VT, USA) at 540 nm. A scatterplot was obtained showing the absorbance values. The results are expressed in micromolar units.
Measurement of progesterone and 17β-estradiol concentrations
Culture media from the different treatments were centrifuged (2700 g for 5 min) and the supernatant stored and maintained at −20°C for later measurement of progesterone (P4) and 17β-estradiol (E2) concentrations. The samples were diluted 1:20 or 1:40 to measure the P4 concentration and 1:200 to measure the E2 concentration, both in distilled water. The chemiluminescence-automated system (Listed Laboratory Equipment 7 C20 UL, MH 15423, series 3975, DPC Immulite I) and commercial diagnostic set were used for quantitative measurement of P4 and E2 (Immulite 1000 Systems, Siemens Healthcare Diagnostics Products Ltd, Gwynedd, UK) (Faes et al., Reference Faes, Caldas-Bussiere, Viana, Dias, Costa and Escocard2009). High and low controls provided by the manufacturer were measured together with the samples in each experiment. The P4 and E2 concentrations are expressed in ng/ml.
Measurement of cGMP and cAMP concentrations
Intracellular concentrations of cGMP and cAMP were determined by enzyme immunoassay (EIA) using commercial kits (Cayman’s cGMP and cAMP EIA kits, Ann Arbor, MI, USA). Groups of 30 and 10 COCs of similar size were used for measurement of cGMP and cAMP, respectively (Bilodeau-Goeseels, Reference Bilodeau-Goeseels2007). After cultivation, COCs were washed in TCM 199–HEPES medium supplemented with 0.4% of BSA. To induce cell lysis, COCs were transferred to a solution of 0.1 M HCl (200 μl), kept for 20 min, and then vortexed for 1 min every 5 min during that period (four times). The samples were then centrifuged at 12,000 g for 5 min and the supernatant was stored at −20°C. All samples were acetylated to increase the sensitivity of the assay, according to the manufacturer’s recommendation. The concentration of the nucleotides was determined using a spectrophotometer (ELISA reader, BioTek, Winooski, USA) at 405 nm. The results from the spectrophotometer readings were transformed to fmol/COC.
Experiments
Experiment I: Effect of inhibition of NOS with l-NAME on oocyte nuclear maturation, integrity of the plasma membrane of CCs, and NO3–/NO2–, progesterone and estradiol synthesis.
First, we aimed to assess the effects of maturation medium supplemented with l-NAME on nuclear maturation and CCs plasma membrane integrity in COCs co-cultured with HSs. Groups of 20 COCs were randomly cultured with eight HSs in 200 µl of maturation medium supplemented with 0.1, 0.5, 1.0, 2.5, 5.0 or 10.0 mM l-NAME. Two control groups were assessed along with the treatment groups: COCs cultured without HSs (control –HSs) in a conventional system, and COCs cultured in the presence of HSs (control +HSs) that was used to validate this oocyte maturation system. Oocyte nuclear maturation stage and CCs plasma membrane integrity were assessed after 22 h of culture. The experiment was repeated seven times.
Afterwards, to determine whether addition of l-NAME reduced NO production in cultured cells (COCs/HSs), nitrate/nitrite (NO3–/NO2–) concentrations were measured in the maturation medium as indirect indicators of NO concentration.
Finally, because NO affects steroid production in vitro (Faes et al., Reference Faes, Caldas-Bussiere, Viana, Dias, Costa and Escocard2009) and because steroids may be involved in the resumption and progression of meiosis (Tsafriri et al., Reference Tsafriri, Cao, Ashkenazi, Motola, Popliker and Pomerantz2005; Fair and Lonergan, Reference Fair and Lonergan2012), we assessed the effect of l-NAME on production of steroids P4 and E2 by COCs and HSs. Steroid concentrations were measured in the medium from the same samples as that for NO3–/NO2–.
Experiment 2: Effect of the inhibition of NOS with l-NAME on intracellular production of nucleotides cGMP and cAMP in COCs
The effect of l-NAME on the intracellular concentrations of cGMP and cAMP was assessed in COCs. By considering the results from the first experiment, COCs were cultured during the first hours of IVM (1, 3 and 6 h) with HSs (control +HSs) and with supplemental 1.0 mM l-NAME in the presence of HSs. cGMP and cAMP concentrations were measured in COCs immediately after their removal from follicles (0 h of culturing, immature COCs) and the results were compared with those obtained in the group control +HSs and l-NAME. This experiment was repeated four times. The experimental design of Experiments 1 and 2 is represented in Fig. 1.

Figure 1. Experimental design of the oocytes co-cultured with follicular wall hemisections (HSs). On the left side of the chart the first steps before IVM are represented, and include the obtaining of cumulus–oocyte complexes (COCs) and follicular hemisections (HSs) from 3–8 mm and 3–5 mm follicles, respectively (A). Twenty COCs were co-cultured with 8 HSs in 4-well dishes. Experiments 1 and 2 are depicted on the right side (B). Experimental groups, assessments and cultivation time vary between experiments. Control +HSs represents COCs co-cultured in the presence of HSs, and without additional supplementation with l-NAME. Control −HSs represents COCs cultured in the absence of HSs, and without additional supplementation of l-NAME.
Statistical analyses
Data consistency analysis and descriptive statistics (PROC UNIVARIATE, PROC MEANS) (SAS, 2011) were performed. Data transformation (log +1) and arcsin were performed for the variables that showed a coefficient of variation higher than 30% (McDonald, Reference McDonald2014). The effects of the different treatments on the variables studied were evaluated by analysis of variance. Means were compared using the SNK test at a 5% probability (PROC GLM) (SAS, 2011).
Results
Experiment I: Effect of the inhibition of NOS with l-NAME on oocyte nuclear maturation, integrity of the plasma membrane of cumulus cells, and NO3–/NO2–, progesterone and oestradiol production
Supplementation of maturation medium with 1.0 mM l-NAME significantly increased the percentage of oocytes that reached MII compared with the control +HSs (P < 0.05) (Fig. 2). No differences in the percentage of oocytes reaching MII after 22 h of culture were observed between the control +HSs and the other treatments (P > 0.05).
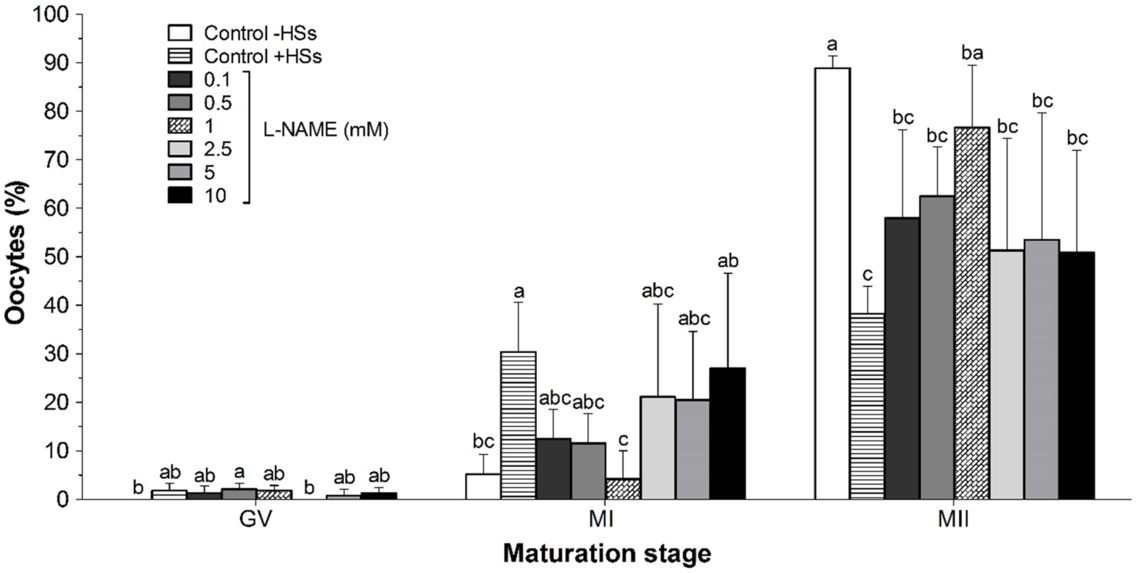
Figure 2. Effect of l-NAME on the nuclear maturation of bovine COCs co-cultured with eight follicular wall HSs for 22 h. Values are expressed as a mean ± standard deviation (SD) for seven replicates. Twenty oocytes per treatment were analyzed in each replicate (n = 140) from 1120 oocytes. Different letters within each maturation stage (same column) indicate significant differences (P < 0.05). GV: germinal vesicle; HSs, hemisections; control –HSs: control without follicular HSs; control +HSs: control with follicular HSs; l-NAME: Nω-nitro-l-arginine methyl ester hydrochloride; MI: metaphase I; MII: metaphase II.
We detected that treatment with 1.0 mM l-NAME increased the percentage of CCs that presented intact plasma membrane when compared with controls –HSs and +HSs (P < 0.05). The other COCs groups supplemented with l-NAME demonstrated similar proportions of CCs with intact membranes as the control group +HSs (P > 0.05) (Fig. 3). Both controls also showed a similar percentage of CCs with intact membranes (P > 0.05).

Figure 3. Effect of different concentrations of l-NAME on the plasma membrane integrity of cumulus cells (CCs) of COCs (n =20) co-cultured with eight follicular wall HSs were measured for 200 cells by repetition. Values represent the mean ± standard deviation (SD) of six replicates (n = 6000 cells). Different letters within the columns indicate significant differences (P < 0.05). COCs: cumulus–oocyte complexes; HSs, hemisections; control –HSs: control without follicular HSs; control +HSs: control with follicular HSs; l-NAME, Nω-nitro-l-arginine methyl ester hydrochloride.
All l-NAME concentrations investigated in this experiment reduced (P < 0.05) the concentrations of NO3–/NO2– in the culture medium to similar levels (P > 0.05) when compared with control +HSs (Fig. 4). Moreover, the values in treated groups were higher than in the control –HSs group (P < 0.05).

Figure 4. Effect of different concentrations of l-NAME on nitrate and nitrite (µM) synthesis in the presence of bovine COCs (n =20) and eight follicular wall HSs. Values represent the means ± standard deviation (SD) of six replicates. Different letters within the columns indicate significant differences (P < 0.05). COCs: cumulus–oocyte complexes; HSs: hemisections; control –HSs: control without follicular HSs; control +HSs: control with follicular HSs; l-NAME, Nω-nitro-l-arginine methyl ester hydrochloride.
There was no difference in the P4 concentration between all the l-NAME concentrations and control +HSs (P > 0.05), but all of them differed from control –HSs (P < 0.05). E2 concentration was also not different between treatments with l-NAME and control +HSs (P > 0.05), but there was difference between 2.5 mM l-NAME and control –HSs (P < 0.05). Finally, addition of HSs (+HS) increased the P4 and E2 concentrations compared with the control without HSs (–HSs) (P < 0.05; Table 1).
Table 1. Effects of adding different concentrations of l-NAME on the progesterone and 17β-estradiol (ng/ml) production by bovine COCs (n = 20) and eight follicular wall HSs evaluated in the culture medium after 22h in vitro maturation

Values represent the means ± standard deviation (SD) of six replicates. a,b Different lowercase letters in the same column mean difference (P < 0.05) between treatments. Control −HSs: control without follicular HSs; control +HSs: Control with follicular HSs.
Experiment 2: Effect of the inhibition of NOS with l-NAME on intracellular production of cGMP and cAMP in COCs
cGMP concentrations decreased in the control +HSs group after 1 h of culture (P < 0.05), remaining stable at 3 h (P > 0.05) and decreasing at 6 h (P < 0.05). cGMP concentrations in the groups treated with 1.0 mM l-NAME decreased after 1 h of culture (P < 0.05) and remained stable (P > 0.05) from 1 to 6 h (P < 0.05). At 3 h of culture, cGMP concentrations were lower in the group treated with 1.0 mM l-NAME than in the control +HSs group (P < 0.05) (Fig. 5).

Figure 5. Effect of the addition of 1.0 mM l-NAME on the kinetics of cGMP production (fmol/COC) in bovine COCs co-cultured with eight follicular wall HSs during the maturation in vitro. Values are expressed as the mean ± standard deviation (SD) of four replicates. Thirty COCs/treatment/h (n =120) were analyzed for in total, 840 COCs. The asterisk shows a significant difference between treatments exclusively at 3 h of culture (P < 0.05). Different lowercase letters denote significant differences between time in the control culture (P < 0.05). Different uppercase letters denote significant differences between time in the culture of l-NAME group (P < 0.05). HSs: hemisections; cGMP: cyclic guanosine monophosphate; COCs: cumulus–oocyte complexes; l-NAME: Nω-nitro-l-arginine methyl ester hydrochloride.
cAMP concentrations in COCs of the control +HSs group and the COCs treated with 1.0 mM l-NAME for 1 h were similar to the cAMP concentrations in immature COCs (0 h of culturing) (P > 0.05). An increase in cAMP was detected after 3 h of culture (P < 0.05) followed by a decrease at 6 h in the control +HSs but not in the 1.0 mM l-NAME treatment group (P < 0.05). At this time, cAMP concentrations were similar to those observed after 1 h of culture and 0 h of culturing in the control +HSs group (P > 0.05). After 1 h of culture, the cAMP concentrations were higher in the group treated with 1.0 mM l-NAME (P < 0.05) (Fig. 6).

Figure 6. Effect of 1.0 mM l-NAME on the kinetics of cAMP production (fmol/COC) in bovine COCs co-cultured with eight follicular wall HSs during the maturation in vitro. Values represent the mean ± standard deviation (SD) of four replicates. Ten COCs/treatment/h (n = 40) were analyzed in 280 COCs. The asterisk shows a significant difference between treatments exclusively at 1 h of culture (P < 0.05). Different lowercase letters denote significant differences between time in the control culture (P < 0.05). Different uppercase letters denote significant differences between time in the culture of l-NAME group (P < 0.05). cAMP: cyclic adenosine monophosphate; COCs: cumulus–oocyte complexes; HSs: hemisections; control +HSs: control with follicular HSs; l-NAME: Nω-nitro-l-arginine methyl ester hydrochloride.
Discussion
This study applied an in vitro co-culture system with COCs and HSs to mimic the intrafollicular microenvironment found in vivo, in which oocytes resume meiosis after the LH surge. However, in this model, the signalling interactions that need cell–cell contact between granulosa cells and CCs were not maintained. NO is somewhat lipophilic and possesses six- to eight-fold higher solubility in non-polar solvents (Shaw and Vosper, Reference Shaw and Vosper1977) and lipid membranes when compared with water, readily entering in the cells (Ignarro, Reference Ignarro2000). Hence, NO produced by CCs and follicular wall cells does not use any transport that needs cell–cell contact to reach the oocyte and CCs. In this way, this culture setting may provide promising results on the role of signalling substances secreted by follicular wall cells that reach the COC by the follicular fluid.
We found that the addition of 1.0 mM l-NAME promoted cell cycle progression (MI to MII stage). The reduction in NO concentration in the culture medium confirmed that the treatment with 1.0 mM l-NAME decreased its synthesis and the cGMP concentration in COCs. The decline of the NO concentration in preovulatory follicles is an essential prerequisite for the resumption of nuclear maturation in rodents in vivo (Nakamura et al., Reference Nakamura, Yamagata, Sugino, Takayama and Kato2002; Sela-Abramovich et al., Reference Sela-Abramovich, Galiani, Nevo and Dekel2008; Tripathi et al., Reference Tripathi, Khatun, Pandey, Mishra, Chaube, Shrivastav and Chaube2009). Therefore, our observations reinforce that the NOS/NO/cGMP pathway is also functional in bovine COCs and regulates meiotic progression in vitro, in the experimental model used in the present study.
Matta et al. (Reference Matta, Caldas-Bussiere, Viana and Quirino2002) and Schwarz et al. (Reference Schwarz, Pires, Adona, De Bem and Leal2008) demonstrated that the addition of l-NAME (10–7, 10–5, and 10–3 M) to the culture medium reduced the rates of MII of bovine oocytes under spontaneous maturation conditions. These results are opposed to our findings in which the addition of 1.0 mM l-NAME increased the percentages of oocytes in MII when compared with control +HSs. It shows that different culture systems, i.e. those that allow for spontaneous nuclear maturation compared with others that block resumption and/or progression of meiosis, may activate different signalling pathways during IVM. In this experimental model, HSs added with COCs may be altering oocyte maturation in vitro, as these secrete steroid hormones (which are liposoluble when not bound to carrier proteins), protein hormones, growth factors and extracellular vesicles (EVs). These EVs can carry and transfer molecules that include mRNAs, microRNAs and proteins among granulosa cells, CCs and oocyte (del Collado et al., Reference Del Collado, Andrade, Meirelles, Silveira and Perecin2018). This could be the reason why we observed similar results to those obtained in mice in vivo after the addition of 1.0 mM l-NAME (Nakamura et al., Reference Nakamura, Yamagata, Sugino, Takayama and Kato2002; Sela-Abramovich et al., Reference Sela-Abramovich, Galiani, Nevo and Dekel2008; Tripathi et al., Reference Tripathi, Khatun, Pandey, Mishra, Chaube, Shrivastav and Chaube2009). Therefore, these results suggested that addition of HSs in the culture system mimics the events that occur in vivo when we evaluate the action of l-NAME on in vitro nuclear maturation.
All concentrations of l-NAME decreased the nitrate/nitrite concentrations in the culture medium, although concentrations were similar among all treated groups after 22 h of culture. l-NAME is a competitive NOS inhibitor that selectively inhibits the production of NO by eNOS and iNOS, mainly by its stronger inhibitory effect on eNOS (Moncada et al., Reference Moncada, Palmer and Higgs1991). Similar results were also observed by Matta et al. (Reference Matta, Caldas-Bussiere, Viana, Faes, Paes de Carvalho, Dias and Quirino2009) in which the use of 1 mM and 10 mM aminoguanidine (AG), selective inhibitor of iNOS isoform, showed no difference in NO3–/NO2– concentration. In addition, Dubeibe et al. (Reference Dubeibe, Caldas-Bussiere, Maciel, Sampaio, Quirino, Gonçalves, De Cesaro, Faes and Paes de Carvalho2017) did not observe any change in NO concentration at 7 and 22 h of culture when adding 2.5, 5.0, and 10.0 mM l-arginine. These results demonstrated that, over a given range, by adding both NOS inhibitors and its precursor, i.e. l-arginine, NO concentrations do not change in the culture medium, leaving this concentration at optimal levels for maintaining cell integrity, probably due to a compensatory feedback between the NOS isoforms.
cGMP concentrations in COCs treated with l-NAME that stimulated the nuclear maturation were markedly reduced after 3 h of culture when compared with the concentrations observed in the control +HSs group. NO stimulates the production of cGMP via activation of the soluble guanylate cyclase enzyme (Denninger and Marletta, Reference Denninger and Marletta1999). Therefore, the reduction in NO production associated with the addition of l-NAME may have induced low cGMP synthesis and may represent one of the possible pathways for meiotic resumption and progression as described in rodents (Pandey et al., Reference Pandey, Tripathi, PremKumar, Shrivastav and Chaube2010; Tiwari and Chaube, Reference Tiwari and Chaube2017).
cGMP inhibits cAMP metabolism through the action of phosphodiesterase 3A (PDE3A), which is the most active PDE in bovine oocytes (Sasseville et al., Reference Sasseville, Albuz, Côté, Guillemette, Gilchrist and Richard2009) and this shows similar affinities for both cGMP and cAMP (Francis et al., Reference Francis, Blount and Corbin2011). As the rate of cGMP hydrolysis by PDEA3 is 10% that of cAMP, cGMP can compete with and inhibit cAMP breakdown at the catalytic site. Therefore, changes in cGMP level directly interfered with cAMP breakdown and thereby regulated cAMP signalling (Francis et al., Reference Francis, Blount and Corbin2011). For this reason, we evaluated intra-oocyte cAMP concentrations in parallel with those of cGMP.
cAMP concentrations in COCs from control and supplemented with 1.0 mM l-NAME groups gradually increased from the first hour of culture, peaked at 3 h and remained stable until 6 h. The presence of gonadotropins (especially FSH) in the maturation medium may have led this observation. Luciano et al. (Reference Luciano, Modina, Vassena, Milanesi, Lauria and Gandolfi2004) found that the addition of gonadotropins and fetal serum to the maturation medium influenced intracellular cAMP concentration during the first hours of culture. Moreover, FSH activates membrane receptors and G proteins in target cells, which can induce cAMP production by activating adenylate cyclase (Luciano et al., Reference Luciano, Lodde, Beretta, Colleoni, Lauria and Modina2005).
The addition of substances that stimulate cAMP synthesis in in vitro maturation systems prevents meiotic resumption (Bilodeau et al., Reference Bilodeau, Fortier and Sirard1993). Nevertheless, changes in cAMP concentrations in the COC may not represent the mechanism by which HSs inhibit nuclear maturation, as lower cAMP concentrations were observed during the first hour of culture in the control +HSs group. Similar levels of cAMP were also detected in the group of COCs treated with 1.0 mM l-NAME in subsequent hours. These alterations in nucleotide concentrations are different from those observed in rodents (Tiwari and Chaube, Reference Tiwari and Chaube2017). However, we and others (Schwarz et al., Reference Schwarz, Pires, Mesquita, Chiaratti and Leal2014; Botigelli et al., Reference Botigelli, Schwarz, Zaffalon, Del Collado, Castro, Fernandes and Leal2017; Dubeibe et al., Reference Dubeibe, Caldas-Bussiere, Maciel, Sampaio, Quirino, Gonçalves, De Cesaro, Faes and Paes de Carvalho2017) have also observed the lack of correlation between cGMP and cAMP concentration during meiosis resumption in bovines. Recently, using this same experimental model, we showed that this increase in cAMP in the COC, which we also observed here, is due to increase of the production by CCs, causing a crescent decreasing in its concentration in the oocyte (Torres et al., Reference Torres, Caldas-Bussiere, Nogueira, Dubeibe, Paes de Carvalho, Dias and Souza2015). This would justify the fact that we did not observe a temporal relationship between the two nucleotides in the resumption of meiosis. More studies are needed to confirm the reproducibility of this model in bovines, as described in rodents (Pandey et al., Reference Pandey, Tripathi, PremKumar, Shrivastav and Chaube2010; Tiwari and Chaube, Reference Tiwari and Chaube2017) to better understand the role of these nucleotides in the two compartments, somatic and germinal, during bovine nuclear maturation in vitro.
NO produced by HSs does not need cell–cell contact to reach the oocyte and/or CCs, but the nucleotides produced by granulosa cells require gap junctions to reach the CCs and oocyte. Therefore, the higher concentration of cGMP observed in COCs from control +HSs may have been due to lower phosphodiesterase activity that degrades cGMP, unlike l-NAME-treated COCs. Further studies are needed to evaluate whether the inhibition of meiosis progression that occurred in the control +HSs was due to NO increase in the culture medium after the addition of follicular wall HSs or whether this effect occurred via maintenance of the high levels of cGMP caused by inhibition of phosphodiesterase 5 (PDE5A) present in bovine oocytes (++) and CCs (+) (Schwarz et al., Reference Schwarz, Pires, Mesquita, Chiaratti and Leal2014). Moreover, it could have occurred due to NO action on nitrosylation of proteins involved in the cell cycle of bovine oocytes. This hypothesis is supported by the findings of Romero-Aguirregomezcorta et al. (Reference Romero-Aguirregomezcorta, Santa, García-Vázquez, Coy and Matás2014), who demonstrated that protein S-nitrosylation participates in the pathway through which NO exerts its effect on porcine IVM.
A higher percentage of CCs with intact plasma membranes was observed after 1.0 mM l-NAME was added. As the NO3–/NO2– concentrations in the culture medium were similar between treatments, these findings suggest that 1.0 mM l-NAME adequately inhibited at least one of the NOS isoforms (eNOS or iNOS, or both) and selectively acted on the signalling pathway linked to plasma membrane integrity. Other studies have illustrated the role of NO in ensuring the integrity of the plasma membrane of oocytes and spermatozoa using NO donors (Leal et al., Reference Leal, Caldas-Bussiere, Paes de Carvalho, Viana and Quirino2009; Viana et al., Reference Viana, Caldas-Bussiere, Paes de Carvalho, Dias, Lanes and Quirino2011) or NO synthesis inhibitors (Leal et al., Reference Leal, Caldas-Bussiere, Paes de Carvalho, Viana and Quirino2009; Matta et al., Reference Matta, Caldas-Bussiere, Viana, Faes, Paes de Carvalho, Dias and Quirino2009). NO can bind and therefore inhibit free radicals that cause lipid peroxidation (e.g. O2− and ONOO–). Therefore, under appropriate concentrations, the NOS/NO system can protect the plasma membrane from damage from an environment characterized by nitrosative or oxidative imbalance (Rubbo et al., Reference Rubbo, Darley-Usmar and Freeman1996).
The importance of NO as a modulator of steroidogenesis has been demonstrated in bovine granulosa cells (Basini et al., Reference Basini, Baratta, Ponderato, Bussolati and Tamanini1998; Faes et al., Reference Faes, Caldas-Bussiere, Viana, Dias, Costa and Escocard2009). In contrast, we did not detect any changes in steroid (P4 and E2) concentrations in the medium after 22 h of culture with any of the l-NAME concentrations tested compared with control +HSs. Our results show that after the addition of HSs with or without l-NAME, an increase of 28.4-fold in the P4 concentration was observed, although there was an increase of 1.4-fold in the E2 concentration. This steroid profile is different from a preovulatory follicle, in which the E2 concentration increases and the P4 concentration remains low with a slight increase before ovulation (Bleach et al., Reference Bleach, Glencross, Feist, Groome and Knight2001) counting as a possible pathway to maintain the partial meiosis arrest observed in this in vitro culture system.
In conclusion, our data show that, using this IVM system that partially blocks meiosis, the NOS/NO/cGMP pathway is also functional in bovine COCs and regulates meiotic progression in vitro, similar to that observed in rodents in vivo, and different to the traditional IVM of bovine oocytes (–HSs). Follicular wall cells appear to be the major producers of NO at levels that are not favourable to the integrity of CCs. Finally, HSs are directly responsible for the major production of steroid hormones and NO seems to not influence this production.
Supplementary material
To view supplementary material for this article, please visit https://doi.org/10.1017/S0967199420000234
Acknowledgements
The authors thank Carla Sobrinho Paes de Carvalho, Bruna Lomba Dias for technical support.
Author contributions
Diego Fernando Dubeibe conducted the experiment with the collaboration of Valter Luiz Maciel Jr and Wlaisa Vasconcelos Sampaio. Data were analyzed by Professor Celia Raquel Quirino. Márcia Resende Faes measured the hormone levels. Maria Clara Caldas-Bussiere is the senior author. All authors participated in the experimental design and preparation of the manuscript.
Financial Support
We would like to thank Rio de Janeiro Research Foundation (FAPERJ) (grant nos. E-26/103.080/2011 and E-26/101.453/2011) for financial support. The Coordenação de Aperfeiçoamento de Pessoal de Nível Superior – Brasil (CAPES) – Finance Code 001, financed this study in part.
Conflict of Interest
None of the authors has any conflict of interest to declare.
Ethical Standards
Not applicable.