Introduction
Temperature is one of the principal environmental factors that directly influences seed germination responses and determines the fraction of seeds that will germinate in a given population as well as their emergence times (Baskin and Baskin, Reference Baskin and Baskin2004; Bewley et al., Reference Bewley, Hilhorst, Bradford and Nonogaki2013). The thermal time model relates developmental processes to the thermal energy received by the system. Knowing cardinal temperatures [base (T b), optimal (T o) and ceiling (T c) temperatures] is essential in the context of the model. For example, T b values can be used to predict the time necessary for a fraction (g) of a population of seeds to germinate within a given thermal regime (Batlla and Benech-Arnold, Reference Batlla and Benech-Arnold2015; Seal et al., Reference Seal, Barwell, Flowers, Wade and Pritchard2018; Picciau et al., Reference Picciau, Pritchard, Mattana and Bacchetta2019).
When seeds are exposed to environmental temperatures (T) above T b (with zero germination rates) until T o (the infra-optimal temperature range), the germination rate (GR) increases with T. Thus, this increase in GR is linearly associated with temperature (García-Huidobro et al., Reference García-Huidobro, Monteith and Squire1982; Cardoso and Pereira, Reference Cardoso and Pereira2009). As such, within a given temperature range, germination of a given fraction occurs when the thermal time (θg) required for the germination of that fraction has been accumulated. This response can be described by the equation θg = (T−T b) tg. In addition, subsequent seed germination may be predicted in relation to thermal time accumulation (heat sum, °Cd).
Seeds can germinate within a temperature range that characterizes their thermal germination niche (Donohue et al., Reference Donohue, Casas, Burghardt, Kovach and Willis2010). The germination niche breadth of a species can influence its ecological amplitude and geographic distribution (Donohue et al., Reference Donohue, Casas, Burghardt, Kovach and Willis2010; Müller et al., Reference Müller, Albach and Zotz2017; Daibes and Cardoso, Reference Daibes and Cardoso2018), with species with broad germination niches being expected to occupy larger geographical areas than species with more narrow germination niches. Some studies have demonstrated a relationship between species distribution and thermal germination niche (Orrù et al., Reference Orrù, Mattana, Pritchard and Bacchetta2012; Porceddu et al., Reference Porceddu, Mattana, Pritchard and Bacchetta2013; Marques et al., Reference Marques, Atman, Silveira and de Lemos-Filho2014; Picciau et al., Reference Picciau, Pritchard, Mattana and Bacchetta2019).
The genus Polygonum (Polygonaceae) comprises 200 species distributed throughout the Americas and Asia (Cialdella and Brandbyge, Reference Cialdella, Brandbyge, Spichiger and Ramella2001). Most of the species are aquatic, occurring along the banks of lakes and rivers and in areas subjected to flooding and low winter temperatures (Melo, Reference Melo2008). Polygonum ferrugineum is an aquatic emergent herb, either floating or amphibious (Melo, Reference Melo2008), distributed throughout tropical America, Argentina, Paraguay and Uruguay (Aymard and Howard, Reference Aymard, Howard, Steryermark, Berry, Yatskievych and Holst2004). Although there is no information available concerning the dynamics of dormancy and germination of P. ferrugineum seeds, studies of other species of the genus, such as P. persicaria (Bouwmeester and Karssen, Reference Bouwmeester and Karssen1992), P. weyrichii var. alpinum and P. cuspidatum (Nishitani and Masuzawa, Reference Nishitani and Masuzawa1996) and P. aviculare (Batlla and Benech-Arnold, Reference Batlla and Benech-Arnold2003), have shown that overcoming dormancy occurs through the action of low winter temperatures.
Seed dormancy is a trait that allows the species to survive under unfavourable environmental conditions (Bewley et al., Reference Bewley, Hilhorst, Bradford and Nonogaki2013). Temperatures during seed development and maturation (maternal environment), as well as post-harvest environmental conditions, influence seed dormancy (Cochrane, Reference Cochrane2019). It has an important ecological role in guaranteeing seed germination only under conditions favourable to initial growth and seedling establishment (Baskin and Baskin, Reference Baskin and Baskin2004). Changes in dormancy levels imply changes in requirements for, or sensitivity to, light and fluctuating temperatures – conditions commonly responsible for terminating seed dormancy and promoting germination (Graeber et al., Reference Graeber, Nakabayashi, Miatton, Leubner-Metzger and Soppe2012; Baskin and Baskin, Reference Baskin and Baskin2014; Fernández Farnocchia et al., Reference Fernández Farnocchia, Benech-Arnold and Batlla2019). Information concerning seed germination and dormancy can be useful to investigations of species distributions and how species are adapted to specific habitats.
In view of the dormancy reported for the seeds of some species of the genus Polygonum, the aims of this study were to: (1) investigate temperature effects on dormancy and germination of P. ferrugineum seeds; (2) characterize the thermal limits [infra-optimal (T b) and supra-optimal (T c)] for germination of non-dormant seeds; and (3) model non-dormant seed germination using the thermal time (degrees-days) model.
Materials and methods
Species studied
P. ferrugineum Wedd. colonizes the margins of boggy or frequently flooded areas (Pott and Pott, Reference Pott and Pott2000); its reproductive phases (flowering and fruiting) occur from January to June in southeastern Brazil (Melo, Reference Melo2008). The study examined seed dormancy and germination of one population established in the Metropolitan Region of Belo Horizonte (MRBH; 19°00′–20°30′S, 43°15′–44°45′W), Minas Gerais State, Brazil. The area of MRBH covers 34 cities (9459.1 km2), among which is Belo Horizonte, an area with high urban density (937 m a.s.l.; 19°51′S, 43°58′W) (Fig. 1A). The regional climate is warm temperate (Cwb using the Köppen system), with hot summers, a well-defined dry season and rainfall generally concentrated from October to April (Austral spring/summer) (Martins et al., Reference Martins, Gonzaga, Santos and Reboita2018). The seeds of P. ferrugineum used in the present study were collected from racemes that still held flowers of more than 50 individual plants at the time of natural dispersal (March and May 2015) (Fig. 1B). Variation in the minimum temperature over the last 20 years (between 2000 and 2020) for Belo Horizonte are shown in Fig. 2.
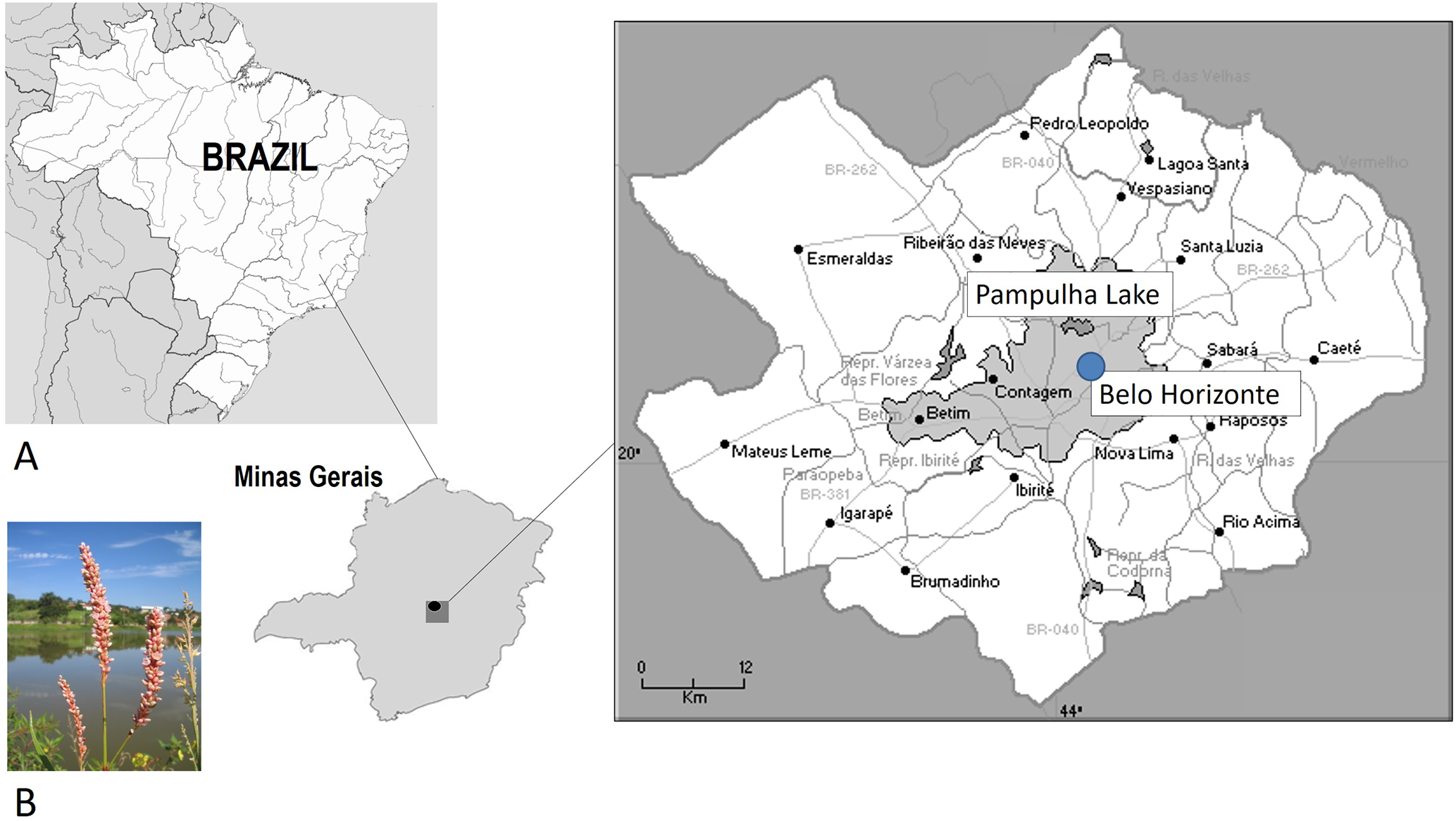
Fig. 1. Location of the collection area – Pampulha Lake, Belo Horizonte, Minas Gerais State, Brazil (A). Specimen of P. ferrugineum flowering (B).

Fig. 2. Variation of the minimum monthly temperatures in the last 20 years (2000–2020) of Belo Horizonte from local meteorological station – 83587Station (19°93′S, 43°93′W; 915.00 m a.s.l.).
Experimental design
After collection, perianths were manually removed in the dark and the seeds (10% water content) stored in glass jars at room temperature (~25°C) for no more than 7 d. Recently harvested seeds (control) were subjected to germination tests in Petri dishes containing a double layer of filter paper moistened with distilled water. Germination tests were performed in germination chambers (three replicates of 30 seeds) at 10, 15, 20, 25 and 30 ± 1°C, under a 12-h photoperiod (30 μmol m−2 s−1) or continuous dark, to quantify initial sensitivity to temperature stimuli. For the dark treatments, the Petri dishes were wrapped in aluminium foil and subsequently placed in opaque polyethylene bags and evaluated under a green safety light (Duarte and Garcia, Reference Duarte and Garcia2015). Germination was evaluated daily (once every 24 h) for 30 consecutive days with the criterion for germination being primary root emergence.
For stratification treatments, five nylon bags containing approximately 100 seeds (to guarantee the number of seeds for germination tests) were buried 5 cm below the surface in dark pots containing sterile sand that had been previously dried at 70°C for 3 d. Stratification treatments were performed at 10, 15 and 20 ± 1°C. The pots were irrigated to saturation and subsequently sealed. Each pot was left to drain for 48 h to determine its field capacity. The pots were then weighed at irregular intervals during the storage period, adding water as necessary to regain their original weight and field saturation levels (Duarte and Garcia, Reference Duarte and Garcia2015). Bags containing seeds were exhumed after 7, 14 and 28 d of wet stratification from each temperature regime for the evaluation of germination. Stratified seeds were incubated at 10, 15, 20, 25 and 30 ± 1°C (three replicates of 30 seeds). Germination tests were conducted and evaluated as described previously for the control treatment. A scheme of the experimental design is provided in Fig. 3.

Fig. 3. Schematic representation of the experimental design of seed stratification and germination.
GR for different percentage fractions was obtained as the reciprocal of time (t) taken by a given percentage fraction to germinate (g), that is GRg = 1/tg, in days−1 (Daibes and Cardoso, Reference Daibes and Cardoso2018). Times for different percentage fractions (g = 20–80%) were estimated by interpolating the interval in which a given percentage was found in the cumulative germination curves against time (d).
Two models were applied to describe non-dormant seed germination of P. ferrugineum under different temperatures: a linear regression model (LR-model) and a probit model (P-model). The LR-model was used to calculate cardinal temperatures (T b, T o and T c) from GR data while the P-model was used to calculate thermal time (θ) requirements from percentage germination (%G).
The RL-model was performed by obtaining thermal parameters from the linear regression lines of GR for different germination fractions (g = 20–80%). Base and ceiling temperatures (T b and T c) in the RL-model were calculated by the x-axis intersecting point (y = 0) of the regression lines of both infra- and supra-optimal ranges for the different GRg values (Daibes and Cardoso, Reference Daibes and Cardoso2018). Values of GRg corresponding to a given percentage were plotted against temperature (T) and a regression line plotted for each percentage fraction along the temperature range (García-Huidobro et al., Reference García-Huidobro, Monteith and Squire1982; Covell et al., Reference Covell, Ellis, Roberts and Summerfield1986). The base temperature (T b) was calculated as the point in which the regression line of the GRg for different percentage fractions (g = 50, 60, 70 and 80%) touches the x-axis (y = 0), being the T b a single value. The GR lines in the supra-optimal range showed a linear and nearly parallel pattern among these different percentage fractions (g = 20, 30 and 40%), with T c (<50) being the median among the fractions. Optimal temperature was calculated considering the equations of the linear regressions of the GRs of the fractions 20, 30 and 40% of the infra and supra-optimal ranges, which were matched to obtain the values on the abscissa of the graph. Optimal temperatures were determined based on GR40 because there was no germination above the 40% fraction at 30°C. Thus, germination was partitioned into infra- and supra-optimal ranges below and above the optimum, respectively (Daibes and Cardoso, Reference Daibes and Cardoso2018).
The P-model was conducted to linearize and quantify the relation of germination percentages as a function of thermal time. Germination percentages (%G) were transformed to probits (Finney, Reference Finney1971). Linear regression was used to express probit(g) as a function of log θ for the infra-optimal temperature range (Hardegree, Reference Hardegree2006). The following equation was used to describe cumulative germination responses of seeds to temperature T (Covell et al., Reference Covell, Ellis, Roberts and Summerfield1986; Daibes and Cardoso, Reference Daibes and Cardoso2018):

where K is an intercept constant when thermal time (θg) is zero, T is the temperature, T b is the base temperature and tg is the time needed for a fraction (g) (20–80%) of seeds to germinate in infra-optimal range. The σ is the standard deviation of the response to θg (i.e., the reciprocal of the slope) and represents the sensitivity of the population to θg (Covell et al., Reference Covell, Ellis, Roberts and Summerfield1986). Thus, the flatter the slope of the fitted line, the greater the variation in response to thermal time among individual seeds (Daws et al., Reference Daws, Lydall, Chmielarz, Leprince, Matthews, Thanos and Pritchard2004). On a plot of probit(g) against log θg, the median thermal time required for seed germination of the population (g = 50%; θ50 INFRA) corresponds to the thermal time when probit(g) = 5 (Finney, Reference Finney1971; Orrù et al., Reference Orrù, Mattana, Pritchard and Bacchetta2012; Daibes and Cardoso, Reference Daibes and Cardoso2018).
The assumptions of the parametric analyses were tested through the Kolmogorov–Smirnov and Bartlett tests (Sileshi, Reference Sileshi2012). Final germination percentages (%G) were arcsine transformed prior to statistical analysis (Piepho, Reference Piepho2003). Germination responses at different temperatures to each light regime were evaluated by one-way analysis of variance (ANOVA) with paired comparisons by Tukey's Honest Significant Difference (HSD) test (P < 0.05) (Sileshi, Reference Sileshi2012). Statistical analyses were performed using Statsoft Statistica v 6.0 software.
Results
The freshly harvested seeds of P. ferrugineum (control) germinated under both light (49.3; 33.3%) and dark conditions (49.3; 22.7%) at 10 and 15°C, respectively, showing no germination at 20, 25 and 30°C. Stratification at both 10 and 15°C for 7 d was efficient at alleviating dormancy, as indicated by subsequent germination in all temperatures tested (i.e., 20 and 25°C) under both light and dark conditions (Table 1). Seeds stratified at 10°C for 7 d had 75% germination at all incubation temperatures in the dark; this, therefore, was considered the most efficient treatment for alleviating dormancy of P. ferrugineum seeds. Conversely, stratification at 20°C for 7 d was not efficient at alleviating dormancy, with subsequent ~50% germination only at 10°C. Seed germination decreased after stratification at 15 and 20°C for periods longer than 7 d, particularly in light conditions (Table 1).
Table 1. Final germination percentages (mean ± SD) of P. ferrugineum seeds at different temperatures for each pre-treatment of cold stratification – 10, 15 and 20°C for 7, 14 and 28 d

Data are the mean of three replicates. For each conditions of the light, the seed lots at different temperatures were compared using one-way ANOVA, followed by post hoc Tukey's Honest Significant Difference (HSD) test was carried out; the same letters are not statistically different (P > 0.05).
Based on the germination data (Fig. 4A), the construction of the thermal time model took the following assumptions into account: (1) 50% of newly dispersed seeds showed express dormancy at 10 and 15°C; (2) stratification at 10°C for 7 d maximizes and increases the temperature range for germination under dark condition; (3) after 14 d of stratification at 10°C, seeds lose the ability to germinate at higher temperatures; and (4) stratification at 15°C, despite increasing the temperature range for germination, has less effect than stratification at 10°C.

Fig. 4. Germination (%) curves as function of time (d) of the Belo Horizonte population after cold stratification (7 d at 10°C) and incubation at constant temperatures (10–30°C) (A). Base (T b), optimal (T o) and ceiling (T c (<50)) temperatures calculated by linear regression for the gemination rate of the fraction 40% of the infra (r 2 = 0.954) and supra-optimal (r 2 = 0.985) ranges (B).
Thermal parameters of germination were calculated from assumption (2) utilizing the LR-model. GR40 had a linear relationship with temperature, increasing up to an optimal point, as well as decreasing for the supra-optimal range (Fig. 4B). T b was 6.3°C, T c (<50) was 32.8°C ± 0.82, and T o was 20.6°C ± 0.12. The relationship between the logarithm of thermal time (log θ) and germination on a linear scale (expressed in probit) for the studied lot of seeds of P. ferrugineum explained more than 90% of the variation in their germination responses (Fig. 5A). For the population in Belo Horizonte, 44.4°Cd were needed to reach 50% germination (θ50 INFRA) in the infra-optimal range (Fig. 5B).

Fig. 5. Germination of P. ferrugineum seeds on the probit scale as a function of log (θ), according to the equation: log (T−T b).tg. Thermal time to attain 50% germination (dashed line) [θ50, corresponds to probit(g) = 5] was calculated assuming the base temperature for gemination of 6.3°C (A). Seed germination (%) for three temperatures (10, 15 and 20°C) in the infra-optimal range as a function of thermal time requirements (θ, °Cd) showing the normal distribution of θ predicted by the probit model (B).
Discussion
Low-temperature stratification (10°C for 7 d) alleviated dormancy and increased the thermal range permissive for germination of P. ferrugineum seeds. This behaviour characterizes non-deep physiological dormancy (sensu Baskin and Baskin, Reference Baskin and Baskin2004) and demonstrates that recently harvested seeds of P. ferrugineum have conditional physiological dormancy (PD), as has been reported for other species (e.g., Carta et al., Reference Carta, Probert, Puglia, Peruzzi and Bedini2016; Oliveira et al., Reference Oliveira, Diamantino and Garcia2017). According to Reynolds (Reference Reynolds1984), P. confertiflorum and P. douglasii require stratification at 3°C and incubation between 5 and 15°C in the dark to initiate germination. Seeds of P. lapathifolium require stratification at 3°C, and when they are incubated between 3.5 and 18.5°C, they attain 74% germination under continuous light (Milberg et al., Reference Milberg, Andersson and Thompson2000). Batlla and Benech-Arnold (Reference Batlla and Benech-Arnold2003) demonstrated that low temperatures alleviate dormancy of P. aviculare seeds, amplifying the thermal range permissive to germination. Among seeds with PD, environmental factors related to seasonality, such as temperature and humidity, can alter the depth of dormancy (Duarte and Garcia, Reference Duarte and Garcia2015) and seed sensitivity to other factors such as light (Batlla and Benech-Arnold, Reference Batlla and Benech-Arnold2005; Finch-Savage and Leubner-Metzger, Reference Finch-Savage and Leubner-Metzger2006). Environmental conditions during seed maturation and post-dispersal can also determine dormancy levels and germination responses (Finch-Savage and Leubner-Metzger, Reference Finch-Savage and Leubner-Metzger2006; Kagaya et al., Reference Kagaya, Tani and Kachi2011; Penfield and MacGregor, Reference Penfield and MacGregor2017; Oliveira and Garcia, Reference Oliveira and Garcia2019).
The results of the present study demonstrated that there is an established pattern in the genus Polygonum, which is a requirement for stratification at low temperatures to overcome/alleviate dormancy and promote germination. Marques et al. (Reference Marques, Atman, Silveira and de Lemos-Filho2014) defined germination niche breadth as the amplitude of germination cues to which a seed is responsive. These authors showed that generalist bromeliads germinate readily across different environments and so have broad germination niches, while specialist bromeliads that respond only to a restricted set of cues have narrow niches. About 50% P. ferrugineum seeds that did not undergo stratification germinated only at 10 and 15°C, thus presenting a narrow thermal niche for germination. However, non-dormant seeds have a broader thermal niche for germination (10–30°C) but must go through specific low-temperature conditions to alleviate dormancy.
Seeds of P. ferrugineum do not encounter restrictions to germination with respect to water availability as they are aquatic or sub-aquatic plants. In general, T b is constant within a given population (Ellis et al., Reference Ellis, Hong and Roberts1987; Pritchard and Manger, Reference Pritchard and Manger1990), although its value can vary between populations (Ellis et al., Reference Ellis, Hong and Roberts1987; Daws et al., Reference Daws, Lydall, Chmielarz, Leprince, Matthews, Thanos and Pritchard2004). The T b of non-dormant P. ferrugineum seeds could explain the species’ distribution in the coldest regions of Brazil (in the South and Southeast and higher altitudes). The thermal characteristics of P. ferrugineum seeds impede their recruitment in regions experiencing high temperatures. According to Pott and Pott (Reference Pott and Pott2000), the occurrence of species of Polygonum in warm localities may reflect vegetative reproduction, as is known to occur in other species of the genus, such as P. lapathifolium (Costa et al., Reference Costa, Martins, Rodella and Rodrigues-Costa2011).
The estimated thermal time for the freshly dispersed P. ferrugineum seeds was θ50 INFRA = 44.4°Cd. Batlla and Benech-Arnold (Reference Batlla and Benech-Arnold2003) reported a θ50 INFRA of 67°Cd for P. aviculare seeds. Differences in thermal time requirements (such as °Cd) are correlated with the environmental parameters of the localities where seeds develop, such as latitude (Daws et al., Reference Daws, Lydall, Chmielarz, Leprince, Matthews, Thanos and Pritchard2004), longitude (Orrù et al., Reference Orrù, Mattana, Pritchard and Bacchetta2012) and altitude (Arana et al., Reference Arana, Gonzalez-Polo, Martinez-Meier, Gallo, Benech-Arnold, Sánchez and Batlla2016). Values for T b and σ, for example, can increase or decrease with increasing latitude (Daws et al., Reference Daws, Lydall, Chmielarz, Leprince, Matthews, Thanos and Pritchard2004). Based on data obtained in this study, P. ferrugineum seeds have a narrow thermal germination niche. Consequently, P. ferrugineum is expected to occur in colder environments, for example, at high altitudes, higher temperatures decrease the probabilities of alleviate dormancy and the ability of their seeds to germinate.
Financial support
This study received financial support by Fundação de Amparo à Pesquisa no Estado de Minas Gerais (FAPEMIG). Q.S.G. received research productivity grant from Conselho Nacional de Desenvolvimento Científico e Tecnológico (CNPq, Brazil).