1. Introduction
Classic plate tectonics theory suggests that deformation occurs mainly along plate boundaries, whereas intraplate regions are rigid (Wilson, Reference Wilson1965; Morgan, Reference Morgan1968; McKenzie & Morgan, Reference McKenzie and Morgan1969). However, intracontinental deformation is also significant in the development of intraplate orogenic belts (Gordon & Stein, Reference Gordon and Stein1992; Gordon, Reference Gordon1998; Kearey et al. Reference Kearey, Klepeis and Vine2009, p. 92; Zheng et al. Reference Zheng, Chen, Dai and Zhao2015). In terms of geodynamics, a far-field consequence of continental collision or a flat-slab subduction is typically regarded as the driving forces for intracontinental deformation (Dickinson & Snyder, Reference Dickinson, Snyder and Matthews1978; Hendrix et al. Reference Hendrix, Graham, Carroll, Sobel, McKnight, Schulein and Wang1992; Wells et al. Reference Wells, Hoisch, Cruz-Uribe and Vervoort2012). Intracontinental deformation can be the result of intracontinental compression and extension (post-orogenic extension; Fossen, Reference Fossen2000) in the interior of plates.
Intracontinental compression contributes to the formation of the intracontinental orogens characterized by fold-and-thrust belts and regional décollement zones, with an absence of ophiolites, mélange and arc-related magmatism, for example the Cenozoic Tianshan orogen in central Asia (Tapponnier & Molnar, Reference Tapponnier and Molnar1979; Avouac et al. Reference Avouac, Tapponnier, Bai, You and Wang1993; Vincent & Allen, Reference Vincent, Allen, Hendrix and Davis2001; Li & Li, Reference Li and Li2007; Li et al. Reference Li, Wang, Jin, Dai, Liu, Zhou, Wang and Zhang2011; Chu et al. Reference Chu, Faure, Lin and Wang2012a, b; Wells et al. Reference Wells, Hoisch, Cruz-Uribe and Vervoort2012). Flexural basins (including foreland basin) develop in the frontal zone of the thrust faults and the sedimentary sequences in the basin are mainly controlled by nearby tectonic events (Lazauskiene et al. Reference Lazauskiene, Sliapua, Brazauskas, Musteikis, McCann and Saintot2003; McCann & Saintot, Reference McCann, Saintot, McCann and Saintot2003; Littke et al. Reference Littke, Bayer, Gajewski and Nelskamp2008). Intracontinental or syn-collisional extension commonly occur in the last phase of shortening or after orogenesis due to instabilities caused by factors such as the over-thickened orogenic crust and lithosphere, underplating, reduction in convergence rate and rapid erosion and/or deposition (Platt, Reference Platt1986; Séguret et al. Reference Séguret, Séranne, Chauvet and Brunel1989; Constenius, Reference Constenius1996; Fossen, Reference Fossen2000; Johnson et al. Reference Johnson, Webb, Graham, Hendrix, Badarch, Hendrix and Davis2001; Wang et al. Reference Wang, Zhang, Fan, Zhao, Zhang, Zhang, Zhang and Li2011b). Meanwhile, the intracontinental extensional structures are characterized by gravitational collapse and other extensional tectonics such as normal faults. Gravitational collapse in fold-and-thrust belts results from uplift of the thickened crust and the high gravitational potential of the belt (Séguret et al. Reference Séguret, Séranne, Chauvet and Brunel1989; Barnolas & Teixell, Reference Barnolas and Teixell1994; Constensius, 1996). The sediments in the collapse basin are therefore mainly derived from the adjacent fold-and-thrust belts and are characterized by high angularity and a large range in grain size, indicative of deposits transported from proximal areas. For example, the Jaca Basin in the southern Pyrenees is a foreland basin where collapses developed at the distal margin due to forebulge uplift (Barnolas & Teixell, Reference Barnolas and Teixell1994).
From Phanerozoic time, the South China Block displays complex patterns regarding early Palaeozoic, early Mesozoic and late Mesozoic tectonomagmatic evolution (Chu et al. Reference Chu, Faure, Lin, Wang and Ji2012c; Shu, Reference Shu2012; Wang et al. Reference Wang, Fan, Zhang and Zhang2013; Zhang et al. Reference Zhang, Guo, Wang, Li, Dong, Liu, He, Cheng, Lu and Yao2013; Li et al. Reference Li, Zhang, Dong and Johnston2014; Faure et al. Reference Faure, Chen, Feng, Shu and Xu2017). The Mesozoic intracontinental deformation in the South China Block is popular in research, with a fold-and-thrust belt, polylayered décollement system and even metamorphism. In addition, a series of NE-trending basins have developed in South China since Mesozoic time, such as the Yuan-Ma Basin (Chen et al. Reference Chen, Sun, Li, Wang, Peng, Xu and Li1992; Li et al. Reference Li, Zhang, Dong and Li2012a; Bai et al. Reference Bai, Jiang, Zhong and Xiong2015) and Jingzhou Basin (Zhang et al. Reference Zhang, Ma, Yang, Chen, Lei, Wang and Li2010), vital for discussing the Mesozoic tectonic evolution of the South China Block (Shu et al. Reference Shu, Zhou, Deng, Wang, Jiang, Yu and Zhao2009; Zhang et al. Reference Zhang, Ma, Yang, Chen, Lei, Wang and Li2010) (Fig. 1).

Fig. 1. Simplified geological map of South China and the tectonic location of the Yuan-Ma Basin (modified from Li et al. Reference Li, Zhang, Zhao, Johnston, Dong, Koppers, Miggins, Sun, Wang and Xin2017). YMB – Yuan-Ma Basin; FWMB – Fanjingshan-Wuling Mountain Belt; MPDS – Mesozoic polylayered décollement system; LMF – Longmenshan Fault; CBF – Cili-Baojing Fault; AXF – Anhua-Xupu Fault; CLF – Chenzhou-Linwu Fault.
Sedimentary records of basin-filling sediments are useful indicators of tectonic events (Greene et al. Reference Greene, Carroll, Hendrix, Graham, Wartes, Abbink, Hendrix and Davis2001; Vincent & Allen, Reference Vincent, Allen, Hendrix and Davis2001; McCann & Saintot, Reference McCann, Saintot, McCann and Saintot2003). The Xuefeng Orogenic Belt (the SW part of the Jiangnan Belt, Fig. 1) is interpreted as an early Mesozoic intracontinental orogen in the South China Block (Chu et al. Reference Chu, Faure, Lin and Wang2012a, c; Wang et al. Reference Wang, Zhang, Fan and Peng2005, Reference Wang, Fan, Zhang and Zhang2013). The Mesozoic polylayered décollement system is regarded as the fold-and-thrust belt of the Xuefeng Orogenic Belt, but the formation time, the phases and mechanism of the deformation and the décollements are still controversial (e.g. Yan et al. Reference Yan, Zhou, Song, Wang and Malpas2003; Mei et al. Reference Mei, Liu, Tang, Shen and Fan2010). The Yuan-Ma Basin, located between the Xuefeng Orogenic Belt and the Mesozoic polylayered décollement system, provides some useful information on the relationship between them, including the tectonic evolution of the South China Block (Fig. 1).
There have been fission-track dating analyses in the area. However, the existing complex age dataset does not elucidate the tectonic evolution (e.g. 165 Ma, Mei et al. Reference Mei, Liu, Tang, Shen and Fan2010; c. 100 Ma, Li & Shan, Reference Li and Shan2011). Although the tectonic evolution of the Yuan-Ma Basin has been addressed in many studies, the phases of deformation are still disputed. Many studies indicated that their research was only based on the deformation in the eastern segment of the Yuan-Ma Basin, regardless of the tectonic events in the western segment and with no existing isotopic geochronology data on the faulting and folding (Chen et al. Reference Chen, Sun, Li, Wang, Peng, Xu and Li1992; Li et al. Reference Li, Zhang, Dong and Li2012a; Bai et al. Reference Bai, Jiang, Zhong and Xiong2015). Study of the sedimentary sequences and detrital zircon ages can therefore provide valuable constraints on the deformation and tectonic evolution of the region. This paper reconstructs the development of the NE–NNE-trending Yuan-Ma Basin by presenting detailed structural cross-sections, sedimentary sequences and detrital zircon isotopic dating. We also discuss the provenance of the sediments with palaeocurrent data.
2. Geological background
2.a. Tectonic setting
The South China Block, located in the SE segment of Eurasia, is a composite plate comprising the Yangtze Block in the NW and the Cathaysia Block in the SE, welded together during early Neoproterozoic time along the Jiangnan Belt (Charvet et al. Reference Charvet, Shu, Shi, Guo and Faure1996; Li, Reference Li1999; Li et al. 2009, Reference Li, Wang and Jahn2013b; Shu et al. Reference Shu, Faure, Yu and Jahn2011; Chu et al. Reference Chu, Faure, Lin and Wang2012a, c; Wang et al. Reference Wells, Hoisch, Cruz-Uribe and Vervoort2012; Zhang et al. Reference Zhang, Guo, Wang, Li, Dong, Liu, He, Cheng, Lu and Yao2013; Xia et al. Reference Xia, Xu, Niu and Liu2017) (Fig. 1). The South China Block is separated from the North China Block by the Qinling-Dabie Orogenic Belt to the north, from the Songpan-Garzê Block by the Longmenshan Fault to the NW (Fig. 1). The Yangtze basement rocks consist mainly of Archean TTG (tonalite, trondhjemite and granodiorite; 3.3–2.7 Ga) gneisses and Palaeoproterozoic gneisses (c. 2.0 Ga), tuff (1.7–1.5 Ga) and sedimentary rocks in the Kongling Complex (Peng et al. Reference Peng, Wu, Gao, Zhang, Wang, Liu, Gong, Zhou, Hu, Liu and Yuan2012; Guo et al. Reference Guo, Gao, Wu, Li, Chen, Hu, Liang, Liu, Zhou, Zong, Zhang and Chen2014; Cawood et al. Reference Cawood, Zhao, Yao, Wang, Xu and Wang2018), intruded by the Mesoproterozoic – Middle Neoproterozoic granites (Zhao & Cawood, Reference Zhao and Cawood2012; Cawood et al. Reference Cawood, Zhao, Yao, Wang, Xu and Wang2018). The Cathaysia basement rocks comprise Palaeoproterozoic–Neoproterozoic gneisses, granitoids and amphibolites, and are sparsely exposed in the Wuyishan, Yunkai and Hainan areas (Zhao & Cawood, Reference Zhao and Cawood2012). The basement rocks in the South China Block are unconformably overlain by late Neoproterozoic – Phanerozoic sedimentary rocks. The lower Palaeozoic strata include the Cambrian–Ordovician siliciclastics and carbonate and Silurian siliciclastics intruded by the Caledonian granites, and the upper Palaeozoic strata consist of Upper Devonian – Permian limestone, dolomite and minor sandstone, and siltstone (Wang et al. Reference Wang, Fan, Zhang and Zhang2013; Cawood et al. Reference Cawood, Zhao, Yao, Wang, Xu and Wang2018). During Middle Triassic time, the Indosinian Orogeny developed volumes of magma intruding to the South China Block; the Lower Triassic carbonates are overlain by the Upper Triassic – Middle Jurassic sandstone, siltstone, mudstone and conglomerate (Wang et al. Reference Wang, Fan, Zhang and Zhang2013). During Jurassic–Cretaceous time, the Yanshanian Orogeny strongly influenced the South China Block related to the westwards subduction of the palaeo-Pacific plate (Li & Li, Reference Li and Li2007; Zhang et al. Reference Zhang, Xu, Jia and Shu2009), and the rocks in the Cretaceous are predominantly sandstone, siltstone and mudstone.
The NE–NNE-trending Yuan-Ma Basin is a large Mesozoic terrestrial basin (35–65 km wide and c. 260 km long), located in the central South China Block in the transition region between the Fanjingshan-Wuling Mountain Belt to the NW and the Xuefeng Orogenic Belt to the SE (Figs. 1 and 2a). The upper Proterozoic and Cambrian – Lower Triassic strata mainly crop out in the large areas surrounding the basin. The Upper Triassic – Middle Jurassic clastic rocks are only exposed in the eastern segment of the basin, the Lower Cretaceous sedimentary rocks predominantly cover the whole basin, and the Upper Cretaceous rocks are only distributed in the vicinity of Mayang and Zhijiang areas (BGMRHN, 1988; Zhang et al. Reference Zhang, Ma, Yang, Chen, Lei, Wang and Li2010; Li et al. Reference Li, Zhang, Dong and Li2012a; Bai et al. Reference Bai, Jiang, Zhong and Xiong2015) (Fig. 2a). The age of these rocks is manifest by the fossils found here, such as Posidonia sp. and Ophiceras cf. demissum of Late Triassic age, Qiyangiaforlis and Pseudocardinia sp. of Jurassic age, and Eucypris-Quadracypris of Early Cretaceous age; dinosaur footprints of Late Cretaceous age were also found (Zeng, Reference Zeng1982; BGHRMN, 1988).

Fig. 2. Tectonic setting and geological background of the Yuan-Ma Basin. (a) Tectonic setting of the Yuan-Ma Basin and its adjacent regions (modified from Ma, Reference Ma2002; Li et al. Reference Li, Zhang, Dong and Li2012a). HCF – Huaihua-Chenxi Fault; XFOB – Xuefeng Orogenic Belt; FG – Fanjingshan Group; BG – Banxi Group; other abbreviations as for Figure 1. The location of (c) is shown and the location of red star is derived from Song et al. (Reference Song, Qin, Zhang, Wang and Han2014). (b) Sequence stratigraphic columns for the Yuan-Ma Basin: 1, Lower Triassic and Upper Triassic – Lower Jurassic; 2, Middle Jurassic; 3, Lower Cretaceous; 4, 5, eastern segment of the Yuan-Ma Basin. (c) Simplified geological map (modified from Geological Bureau of Hunan Province, 1965, 1971, 1972, 1973, 2000). Sampling locations are shown. (d) Schematic cross-section (A–B–C in (c)). Rectangle a: normal faulting of gravity-sliding fault (Fig. 3o); rectangle b: the strongly deformed Upper Triassic – Lower Jurassic and Permian – Lower Triassic klippe overlying Middle Jurassic rocks. F1 and F2 shown in (a) and (c), respectively.
2.b. Tectonic belts surrounding the Yuan-Ma Basin
The Xuefeng Orogenic Belt is separated from the Cathaysia Block to the SE by the Chenzhou-Linwu Fault and from the Fanjingshan-Wuling Mountain Belt to the NW by the Yuan-Ma Basin (Fig. 1). The early Mesozoic orogeny (245–195 Ma) created the Xuefeng Orogenic Belt, undergoing first folding and ductile deformation and then late orogenic magmatism (225–215 Ma) (Qiu et al. Reference Qiu, Zhang and Ma1998; Wang et al. 2005, Reference Wang, Fan, Zhang and Zhang2013; Li et al. Reference Li, Wang, Jin, Dai, Liu, Zhou, Wang and Zhang2011; Chu et al. Reference Chu, Faure, Lin and Wang2012a, b, c; Chu & Lin, Reference Chu and Lin2014; Faure et al. Reference Faure, Lin, Chu and Lepvrier2016, Reference Faure, Chen, Feng, Shu and Xu2017). The sedimentary rocks in the Xuefeng Orogenic Belt consist of Proterozoic slates, phyllites, tillites and metasedimentary rocks, Palaeozoic – Lower Triassic shallow marine sediments such as limestones and shales, and Middle Triassic – Cretaceous terrestrial sediments such as sandstones and conglomerates (BGMRHN, 1988).
The Fanjingshan-Wuling Mountain Belt is geologically composed of NE–SW-trending folds and faults that have experienced Jinning (middle Neoproterozoic), Caledonian, Indosinian and Yanshanian (Jurassic–Cretaceous) events (Zhou et al. Reference Zhou, Li, Wang and Liu2011). The Neoproterozoic basement rocks, including the Fanjingshan and Banxi groups, are predominantly exposed in the Fanjingshan Anticline (Fig. 2a); these slate, metasedimentary and volcanic rocks are overlain by a cover sequence of Cambrian–Ordovician carbonates. Yuan et al. (Reference Yuan, Sun, Zhou, Wang, Li, Zhang and Wo2010) demonstrated that the Fanjingshan-Wuling Mountain Belt was subjected to fast exhumation of the exposed folded basement during 137–100 Ma.
2.c. Faults associated with the Yuan-Ma Basin
The major faults controlling the development of the Yuan-Ma Basin in the peripheral areas, from west to east, are the Cili-Baojing, Huaihua-Yuanling and Anhua-Xupu faults, which all dip to the SE (Wang et al. Reference Wang, Zhang, Fan and Peng2005; Zhang et al. Reference Zhang, Ma, Yang, Chen, Lei, Wang and Li2010; Bai et al. Reference Bai, Jiang, Zhong and Xiong2015) (Fig. 2a). The NE-trending Cili-Baojing Fault, located between the Mesozoic polylayered décollement system (as the thin-skinned structure to the NW) and the Xuefeng Orogenic Belt (as the thick-skinned structure to the SE), extends downwards to a basal detachment and underwent transtensional and tensional tectonic events at 131–120 and 119–86 Ma, respectively (Yan et al. Reference Yan, Zhou, Song, Wang and Malpas2003; Xie et al. Reference Xie, Yang and Ma2006; Qi et al. Reference Qi, Zhai and Zhang2009; Tang et al. Reference Tang, Yan, Wang and Zhang2011). The NNE–NE-trending Huaihua-Yuanling Fault, located along the SE margin of the Yuan-Ma Basin as the frontal fault of the Xuefeng Basal Thrust Belt (Ding et al. Reference Ding, Guo, Liu and Zhai2007), which comprises the Huaihua-Yuanling, Anhua-Xupu and other secondary faults (Fig. 2a), represents a strong compression in the field and strongly affected the formation of the Yuan-Ma Basin (Ding et al. Reference Ding, Guo, Liu and Zhai2007; Li et al. Reference Li, Zhang, Dong and Li2012a; Bai et al. Reference Bai, Jiang, Zhong and Xiong2015). The NE-striking Anhua-Xupu Fault underwent Late Triassic – Middle Jurassic sinistral strike-slipping and thrusting, late Middle Jurassic thrusting and late Early Cretaceous (120–91 Ma) extension (Yang et al. Reference Yang, Yang and Ma2004; Zhang et al. Reference Zhang, Ma, Yang, Chen, Lei, Wang and Li2010; Bai et al. Reference Bai, Zhong, Jia, Xiong and Huang2016).
3. Stratigraphic sequences and structural features within the Yuan-Ma Basin
3.a. Stratigraphic sequences
3.a.1. Eastern segment
The stratigraphic sequences in the eastern segment of the basin comprise predominantly Upper Triassic – Lower Jurassic clastic rocks, a coal-bearing formation and Middle Jurassic clastic rocks (Figs 2b1, b2, 3b–j). The Permian – Lower Triassic limestones are unconformably overlain by the Upper Triassic – Lower Jurassic layers (Fig. 3b, c). Thin coal-bearing shales occur at the bottom of the layers within the intensively deformed greyish-green sandstones, which experienced intensive deformation compared with the overlying layers. The overlying strata consist mainly of terrigenous sediments composed of (in ascending order): thick greyish-green sandstones and siltstones; thick grey greywackes; and thick interbedded layers between thin greyish-green sandstones and brick-red sandstones, siltstones and mudstones (Fig. 2b1, b2). The Middle Jurassic rocks comprise (ascending stratigraphic order): brick-red sandstones, c. 20 m thick conglomerates interbedded with thin pelitic siltstones, abundant pebbly sandstones and molasse-like formations (Figs. 2b2 and 3g–j). The gravel clasts in the conglomerates are in an arenaceous and calcareous matrix, rounded with small numbers of angular clasts, and composed mainly of limestones, cherts, metaquartzite, siliceous rocks and sandstones (Fig. 3g, h). The conglomerates that underlie the Permian – Lower Triassic klippe in the east segment of the basin are characterized by subangular–rounded clasts in an arenaceous matrix, which indicates that these deposits represent another sedimentary cycle (Fig. 3i). At the top of the Middle Jurassic rocks, the molasse-like formation is composed of sub-rounded–rounded gravel clasts of limestones, dolomites, cherts and sandstones of variable sizes, with arenaceous and argillaceous matrix (Fig. 3j).

Fig. 3. Field photographs from eastern to western segments of the Yuan-Ma Basin: (a) extensively deformed Permian – Lower Triassic limestone; (b) the unconformity between Permian–Lower Triassic and Upper Triassic – Lower Jurassic strata; (c) extensively deformed coal-bearing formation and sandstone within Upper Triassic – Lower Jurassic strata; (d–f) Upper Triassic – Lower Jurassic thrust faults and fault-related folds; (g, h) thick conglomerate in the Middle Jurassic layers; (i) Permian – Early Triassic klippe overlying Middle Jurassic rocks; (j) Middle Jurassic molasse-like formation; (k–n) weakly deformed Lower Cretaceous rocks in the central area of the basin; (o) normal fault developed at the western side of the Yuan-Ma Basin; (p) breccia in the normal fault belt; (q) the thrust fault developed at the front zone of the gravity-sliding fault in the western segment of the Yuan-Ma Basin; and (r) the unconformity between Neoproterozoic–Cambrian slate and limestone and upper Lower Cretaceous sandstone. P – Permian; T1, T2, T3 – Lower, Middle, Upper Triassic; J1, J2 – Lower, Middle Jurassic; K1 – Lower Cretaceous; Pt3 – Neoproterozoic; ϵ, Cambrian.
3.a.2. Central area
In the central area of the basin, the attitudes of the Lower Cretaceous rocks become near-flat in Mayang areas (Fig. 3m, n). The Neoproterozoic layers are sporadically exposed in the central area. The pre-Cretaceous rocks are unconformably overlain by the Lower Cretaceous rocks which consist of brick-red terrigenous deposits including pebbly sandstones, sandstones, siltstones, pelitic siltstones and mudstones, suggesting that deposition occurred during several sedimentary cycles in an oxidizing environment (Fig. 2b3).
3.a.3. Western segment
On the western side of the basin, the Neoproterozoic–Cambrian layers largely crop out surrounding the basin, and are composed of extensively deformed slates, metasandstones and limestones (Figs 2b4, b5, 3q, r). The Lower Cretaceous rocks in the western segment of the basin are predominantly composed of brick-red sandstones and siltstones (Fig. 3q, r). In particular, the fault breccias occur in the western segment and are composed of angular clasts of limestones, sandstones and slates in a poorly sorted arenaceous matrix (Fig. 3o, p).

Fig. 4. Representative cathodoluminescence (CL) images of the concordant detrital zircons. Age data are single spot 206Pb/238U ages for those younger than 1000 Ma and 207Pb/206Pb ages for those older than 1000 Ma. Red circles indicate the location of the analysis. CL images are also annotated with sample names and analysis numbers.

Fig. 5. Plot of age versus Th/U ratio for the concordant detrital zircons. Legend: 1, JFYM-01; 2, JFYM-03; 3, JFYM-04; 4, JF-46; 5, JF-45; 6, JF-44-1; 7, JF-43; 8, JF-40; 9, JF-38; 10, JF-37; 11, JF-47; 12, JFYM-06; 13, JFYM-07; 14, JFYM-08.
3.b. Structural features
A variety of structures have developed within the Yuan-Ma Basin and its periphery, such as NE–SW-, NNE–SSW- and E–W-trending normal faults, secondary strike-slip faults in the inner area and western segment of the basin, and NE–SW-trending thrust faults in the eastern segment of the basin (Fig. 2c). Overall, the basin is characterized by intensive deformation controlled by thrust faults in the east, and relatively weak deformation controlled by normal faults in the west.
In the eastern segment of the basin, the Permian – Lower Triassic limestones develop extensive folds and have a weak unconformity with the underlying layers (Fig. 3a–c). The Upper Triassic – Lower Jurassic layers underwent compressional evolution, manifest by the series of thrusts in the layers (Figs 2d3, 3d–f). The Middle Jurassic strata are weakly deformed, but a WNW-verging thrust fault transported the Permian – Lower Triassic limestones over the Middle Jurassic clastic rocks to generate a klippe, which indicates ESE–WNW-trending compression (Figs 2d3, 3i). In the central area of the basin, the deformation is relatively weak and resulted in low-angle strata and a series of minor normal and thrust faults that developed after basin formation (Fig. 3k–n). In the western segment of the basin, one gravity-sliding fault strongly controlled the late stage of the basin (Fig. 2d1, 3o–q). In Figures 2d1 and 3q, the root zone of the fault with a 5 m thick breccia belt cuts the extensively deformed Neoproterozoic–Cambrian layers and the front zone of the fault with a 2.5 m thick breccia belt thrusts these layers over the bottom of the Lower Cretaceous (145–116 Ma) layers; these are covered by the syn-tectonic upper Lower Cretaceous layers (116–100 Ma) (Fig. 3r, the same location as in Fig. 2d1). This thrust therefore occurred at c. 116 Ma. In Figure 3o, the normal fault with an c. 1.2 m thick breccia belt cuts the Lower Cretaceous strata in the hanging wall and the Neoproterozoic–Cambrian layers in the footwall.
3.c. Sample collections
Nineteen samples were collected from the Yuan-Ma Basin, including six from the eastern segment, three from the western segment and ten from the central area. A total of 14 samples were analysed and the analytical data and sample descriptions are provided in online Supplementary Tables S1 and S2 (available at http://journals.cambridge.org/geo) and in Table 1, respectively. Sampling locations are shown in Figure 2c. The conglomerate samples (JYFM-3, JFYM-4 and JF-46) were collected from the Middle Jurassic strata (Fig. 3g–j). Sample JFYM-5, collected from the Middle Jurassic rocks, is a tectonic breccia comprising angular clasts of limestone, sandstone and chert in an arenaceous and calcareous matrix (Fig. 3i). Sample JFYM-6, collected from the normal fault zone in the western segment of the basin, is a limestone breccia comprising angular sandstone and slate clasts in an arenaceous and ferruginous matrix; the mineral content is dominated by calcite and a few mica (Fig. 3o, p). All other samples were collected from Lower Cretaceous rocks with the exception of samples JFYM-1 and JFYM-8; the latter were collected from Upper Triassic – Lower Jurassic and Neoproterozoic–Cambrian strata, respectively. Sample JFYM-1 is a greywacke composed of quartz, perthite, microcline, plagioclase, albite and micas (mostly developed along fractures) (Fig. 3c). Sample JFYM-8 is a purple-red sandstone composed of quartz, plagioclase and mica.
Table 1. Sample descriptions and detrital zircon U-Pb age data from Yuan-Ma Basin, Central South China

4. Detrital zircon analysis
4.a. Analytical methods
Zircon grains were separated from sandstones and conglomerates by using conventional techniques, including heavy-liquid and magnetic separation, and hand-picking under a binocular microscope. The zircon grains were then mounted in epoxy disks, polished to expose their cores and coated with gold prior to analysis. Zircons were imaged under transmitted and reflected light, and cathodoluminescence (CL) images were obtained to reveal internal structures and select the optimal sites for U–Pb dating and trace-element analyses.
Analyses of zircon grains were performed using an Agilent 7500a inductively coupled plasma mass spectrometry (ICP-MS) instrument at the State Key Laboratory of Geological Processes and Mineral Resources, China University of Geosciences, Wuhan, China. The spot size and laser frequency were 32 μm and 10 Hz, respectively. Zircon 91500 and GJ-1 were used as external standards for calibration of U–Pb ages. NIST 610 was analysed as an external standard for calibration of trace-element contents. Details of the laboratory procedure are provided by Liu et al. (2008, Reference Liu, Gao, Hu, Gao, Zong and Wang2010a, b). The U–Th–Pb isotopic ratios used for zircon 91500 are from Wiedenbeck et al. (Reference Wiedenbeck, Allé, Corfu, Griffin, Meier, Oberli, Quadt, Roddick and Spiegel1995). Trace-element analyses of zircon were calibrated with Si as an internal standard. Each analysis incorporated c. 20–30 s of gas blank and 50 s of sample data acquisition.
Data from individual samples are shown in Table 1. Age data are plotted as single-spot 206Pb/238U ages for zircons younger than 1000 Ma and 207Pb/206Pb ages for zircons older than 1000 Ma (He et al. Reference He, Klemd, Zhang, Zong, Sun, Tian and Huang2015), and the full dataset is provided in online Supplementary Table S1.
4.b. Analytical results
4.b.1. Cathodoluminescence images, Th/U ratios and rare Earth elements of detrital zircons
CL images of representative detrital zircon are shown in Figure 4 with their corresponding ages. The zircons are mostly euhedral, subhedral or sub-rounded, and 20–180 μm long (Fig. 4). The Th/U ratio can be used to distinguish igneous and metamorphic zircons (Hoskin & Ireland, Reference Hoskin and Ireland2000) (Fig. 5). In Figure 5, most of the analysed zircons (c. 975 grains were useful for dating) yield Th/U ratios of 0.01–4.43. The Th/U ratios of 806 grains (82.7% of the total grains) are greater than 0.4, and the ratios of 13 grains (1.3%) are less than 0.1. All trace-element plots of the analysed zircons show distinct enrichment in heavy rare Earth elements (HREE) and depletion in light rare Earth elements (LREE). The REE patterns (Fig. 6) exhibit steeply rising slopes from LREE to HREE, and positive Ce anomalies and negative Eu anomalies. The zircon CL characteristics, Th/U ratios and REE patterns indicate that most of the zircon grains are magmatic in origin (Hoskin & Ireland, Reference Hoskin and Ireland2000; Hoskin & Schaltegger, Reference Hoskin, Schaltegger, Hanchar and Hoskin2003).

Fig. 6. Chondrite-normalized REE patterns obtained from the concordant single zircons for all analysed samples.
4.b.2. Detrital zircon ages
The four detrital zircon samples JFYM-01, 03, 04 and JF-46 from the eastern segment of the Yuan-Ma Basin demonstrate several major age clusters. The significant age clusters are at 2700–2300 Ma (11%), 900–700 Ma (31%) and 300–150 Ma (15% of the total zircons), and minor clusters are present at 3500–2550 Ma (2%), 2550–2300 Ma (10%), 2300–2100 Ma (3%), 1900–1700 Ma (8%), 1700–1100 Ma (4%), 1100–900 Ma (6%), 700–600 Ma (2%) and 600–300 Ma (8%).
The seven detrital zircon samples JF-37, 38, 40, 43, 44-1, 45 and 47 in the central segment of the Yuan-Ma Basin show several major age clusters. The significant age clusters are mainly at 900–700 Ma (26%), 600–350 Ma (12%) and 300–150 Ma (22% of the total zircons), with minor age groups at 3600–2700 Ma (1%), 2700–2300 Ma (6%), 2300–2000 Ma (2%), 2000–1700 Ma (9%), 1700–1100 Ma (6%), 1100–900 Ma (7%), 700–600 Ma (1%), 350–300 Ma (3%), 150–120 Ma (3%) and 120–100 Ma (2%).
The two detrital zircon samples JFYM-06 and -07 in the western segment of the Yuan-Ma Basin show several major age clusters. The significant age clusters are at 900–700 Ma (28%), 500–350 Ma (13%) and 350–150 Ma (14%), with minor age clusters at 3600–2600 Ma (3%), 2600–2300 Ma (5%), 2300–1900 Ma (4%), 1900–1600 Ma (7%), 1600–1050 Ma (9%), 1050–900 Ma (9%), 700–500 Ma (7%) and 120–100 Ma (1%). The youngest zircon of 570 ± 6 Ma in sample JFYM-08 is older than the youngest zircons in the other samples, although it also has the same age clusters at 2600–2300 and 900–600 Ma as the two other samples.
4.c. Interpretation of zircon U–Pb age data
Observations of the sedimentary rocks in the field, together with analysing detrital zircon age clusters, are best to constrain the sources and the upper age limits of the sediments (Hanchar & Hoskin, Reference Hanchar and Hoskin2003; Cawood et al. Reference Cawood, Hawkesworth and Dhuime2012). On the eastern segment of the basin, the youngest age of the analytical detrital zircons is 173 ± 2.2 Ma (JFYM-01), which indicates that the reactivation of the thrust faults, that is, Xuefeng Basal Thrust Belt (F1 in Fig. 2a) in the east is younger than 173 ± 2.2 Ma. In the western segment, the youngest detrital zircon ages from sample JFYM-06 in the fault zone and JFYM-07 in the hanging wall are 120 ± 1.3 Ma and 116 ± 1.7 Ma, respectively, which define the upper limit of the gravity-sliding fault (Fig. 2d1). The central area samples yield the youngest age of 101 Ma, which defines the lower limit of the gravity-sliding fault because the syn-tectonic upper Lower Cretaceous rocks overlay the fault and the whole Yuan-Ma Basin.
5. Discussion
5.a. Potential source areas of the Yuan-Ma Basin
The exhumation history indicated by the sedimentary rocks, especially the sandstone composition in the basin, can provide a powerful tool in the interpretation of the uplift and erosional history of the adjacent orogenic belt (Hendrix et al. Reference Hendrix, Graham, Amory and Badarch1996; Hendrix, Reference Hendrix2000).
5.a.1. Provenance of detrital zircons
The detrital zircon U–Pb ages of the Upper Triassic – Cretaceous rocks are consistent with the magmatism that occurred in the Xuefeng Orogenic Belt and the Fanjingshan-Wuling Mountain Belt (BGMRHZ, 1988; Chen et al. 2006, Reference Chen, Chen, Huang, Ding and Sun2007; Zhou & Li, Reference Zhou and Li2000; Li & Li, Reference Li and Li2007; Chu et al. Reference Chu, Faure, Lin, Wang and Ji2012c; Wang et al. Reference Wang, Fan, Zhang and Zhang2013), and the zircons are mainly derived from these two surrounding areas. The detrital zircon data show significant age clusters at 3600–2700 Ma, 2700–2300 Ma, 2300–1900 Ma, 1900–1700 Ma, 1700–1050 Ma, 1050–900 Ma, 900–700 Ma, 500–350 Ma, 300–150 Ma, and 120–100 Ma (Fig. 7).

Fig. 7. Relative probability density diagrams of the concordant detrital zircons for all samples. The age group of 400–90 Ma is shown in the inset figures.
The 120–100 Ma detrital zircons include subsidiary age clusters at 116–101 Ma and 120–116 Ma derived from samples in the central and western segments of the Yuan-Ma Basin, respectively. Song et al. (Reference Song, Qin, Zhang, Wang and Han2014) suggested that the rhyolitic porphyry with the age of 98.9 ± 1.3 Ma was exposed in the NE of the Fanjingshan Anticline (red star in Fig. 2a). In the western segment of the Yuan-Ma Basin, the small age cluster at 120–116 Ma defines the upper limit of the gravity-sliding fault and is related to the late Early Cretaceous magmatism that occurred along the Fanjingshan Anticline. The 120–100 Ma detrital zircons were therefore derived from the denudation of the Fanjingshan-Wuling Mountain Belt.
The 300–150 Ma detrital zircons have age clusters at 298–173 Ma in the eastern segment, 300–154 Ma in the central area and 298–210 Ma in the western segment of the basin. This age group can be divided into three age groups – 300–260 Ma, 260–210 Ma and 200–160 Ma (Fig. 7) – consistent with late Palaeozoic, Triassic (Indosinian) and Jurassic (Yanshanian) magmatism, respectively, in the SE of the Yuan-Ma Basin (Wang et al. 2003, 2007, Reference Wang, Fan, Zhang and Zhang2013; Chen et al. 2006, Reference Chen, Chen, Huang, Ding and Sun2007). Moreover, massive granites of age 300–150 Ma have been widely reported in the Xuefeng Orogenic Belt (Wang et al. 2005, 2007, Reference Wang, Zhang, Fan, Zhao, Zhang, Zhang, Zhang and Li2011b, Reference Wang, Fan, Zhang and Zhang2013; Chen et al. Reference Chen, Chen, Huang, Ding and Sun2007), suggesting that the detrital zircons were mainly sourced from the Xuefeng Orogenic Belt.
The detrital zircons aged 500–350 Ma have age clusters at 490–350 Ma in the eastern segment, 496–371 Ma in the central area and 479–366 Ma in the western segment of the basin. These detrital zircons are consistent with earliest late Palaeozoic and early Palaeozoic magmatism. The upper Palaeozoic magmatic rocks crop out in the SE South China Block, and the early Palaeozoic magmatism acts as the product of a post-early Palaeozoic orogeny exposed in the east side of the Anhua-Xupu Fault (Chu et al. Reference Chu, Faure, Lin, Wang and Ji2012c; Zhang et al. Reference Zhang, Wang, Zhang, Fan, Zhang and Zi2012; Charvet, Reference Charvet2013; Wang et al. Reference Wang, Fan, Zhang and Zhang2013) (Fig. 2). Consequently, the Palaeozoic magmatic rocks of the Xuefeng Orogenic Belt represent the most likely provenance for these detrital zircons. This age cluster of detrital zircons was likely derived from the lower Palaeozoic magmatic rocks of the Xuefeng Orogenic Belt as a result of the intracontinental orogeny as a far-field response to the assembly of the Australian–Indian Plate with the Cathaysia Block (Wang et al. Reference Wang, Zhang, Fan, Zhang, Chen, Cawood and Zhang2010b, Reference Wang, Zhang, Fan, Zhao, Zhang, Zhang, Zhang and Li2011b, Reference Wang, Fan, Zhang and Zhang2013; Zhang et al. Reference Zhang, Guo, Wang, Li, Dong, Liu, He, Cheng, Lu and Yao2013).
The detrital zircons of age 900–700 Ma have clusters at 898–706 Ma in the eastern segment, 887–702 Ma in the central area and 898–709 Ma in the western segment of the basin. These detrital zircon ages are consistent with the 900–850 Ma syn-collisional magmatism, 850–800 Ma post-collisional magmatism and 800–600 Ma post-orogeny extension-related magmatism that occurred in the western segment of the Xuefeng Orogenic Belt during late Neoproterozoic time (Wang et al. Reference Wang, Zhao, Zhou, Liu and Hu2008a, b; Shu, Reference Shu2012; Zhang et al. Reference Zhang, Guo, Wang, Li, Dong, Liu, He, Cheng, Lu and Yao2013; Cawood et al. Reference Cawood, Zhao, Yao, Wang, Xu and Wang2018). The Neoproterozoic mafic-ultramafic rocks and granites of the Fanjingshan area are intruded into the Fanjingshan Group, and related to collision between the Yangtze and Cathaysia blocks and the intracontinental rifting that resulted from the breakup of Rodinia (BGMRGZ, 1987; Zhou et al. Reference Zhou, Wang and Qiu2009; Wang et al. Reference Wang, Griffin, Yu and O’Reilly2010a; Wang, Reference Wang2012; Xue et al. Reference Xue, Ma and Song2012). The SW segment of the Xuefeng Orogenic Belt represents the potential source area of the Neoproterozoic detrital zircons in the eastern segment of the Yuan-Ma Basin. In contrast, the Fanjingshan-Wuling Mountain Belt is the potential source area of the detrital zircons in the western segment, although a few zircons were also derived from the Xuefeng Orogenic Belt. The detrital zircons in the central area were sourced from both the Xuefeng Orogenic Belt and the Fanjingshan-Wuling Mountain Belt.
The detrital zircons aged 1700–1050 Ma and 1050–900 Ma were characterized by scattered ages yield subgroupings of 1698–1062 Ma and 994–905 Ma in the eastern segment, 1695–1065 Ma and 1031–907 Ma in the central area, and 1672–1081 Ma and 1040–904 Ma in the western segment of the basin (and 1528–1339 Ma and 971–928 Ma from sample JFYM-08). These detrital zircon ages are consistent with early Neoproterozoic and Mesoproterozoic – late Palaeoproterozoic magmatism (e.g. Shu et al. Reference Shu, Faure, Yu and Jahn2011; Shu, Reference Shu2012; Li et al. Reference Li, Lin, Xing, Davis, Davis, Xiao and Yin2013a). The remaining detrital zircon ages of 3600–1700 Ma are consistent with derivation from the Archean and Palaeoproterozoic rocks in the Kongling Complex, a part of basement of the Yangtze Block.
5.a.2. Rock fragments and palaeocurrent analyses
Thin-sections of each sample were prepared (Fig. 8). The abundant rock fragments identified in thin-section are mainly chert, quartz schist, marble, slate, andesite, acid volcanic rocks and metapelite (Fig. 8). The rock fragments from the eastern segment of the Yuan-Ma Basin are mainly chert, slate, shale and andesite, whereas marble and sandstone are dominant in fragments from the western segment (Fig. 8j–r). In the central area of the basin, the rock fragments are mostly chert, slate, quartz schist, acid volcanic rocks and metapelite (Fig. 8a–i).
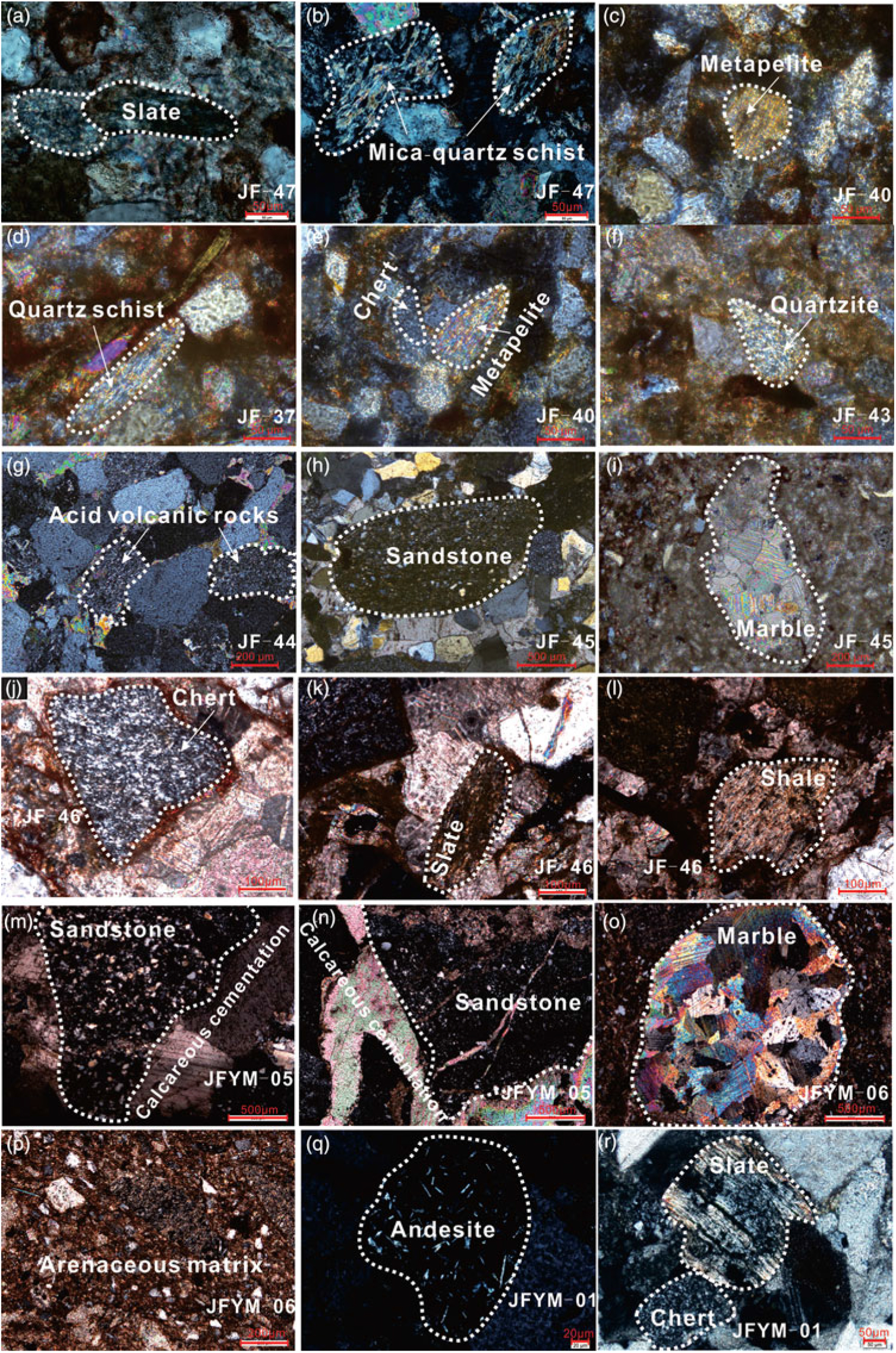
Fig. 8. Microphotographs of rock fragments in samples: (a) slate of sample JF-47; (b) mica-quartz schist of sample JF-47; (c) metapelite of sample JF-40; (d) quartz schist of sample JF-37; (e) chert and metapelite of sample JF-40; (f) quartzite of sample JF-43; (g) acid volcanic rocks of sample JF-44; (h) sandstone of sample JF-45; (i) marble of sample JF-45; (j) chert of sample JF-46; (k) slate of sample JF-46; (l) shale of sample JF-46; (m) sandstone of sample JFYM-05; (n) sandstone of sample JFYM-05; (o) marble of sample JFYM-06; (p) arenaceous matrix of sample JFYM-06; (q) andesite of sample JFYM-01; and (r) chert and slate of sample JFYM-01.
Sandstones with parallel and cross-bedding and thin imbricated conglomerates occur in the whole basin. In Figure 9, the palaeoflow directions are perpendicular to the orogenic belts in the two sides of the basin. The palaeocurrent directions of the Upper Triassic – Lower Jurassic strata in Chenxi area (located close to the Huaihua-Chenxi Fault) were mostly aligned SE–NW (Fig. 9), suggesting that the basin sediments were sourced from the Xuefeng Orogenic Belt. Palaeoflow directions in the Middle Jurassic layers in the south of Chenxi Town were aligned SE–NW and locally E–W, indicating that sediments in the Yuan-Ma Basin were also derived from the Xuefeng Orogenic Belt. In the Lower Cretaceous strata, it is evident that the palaeoflow directions in the eastern segment of the basin were aligned SE–NW, E–W and locally NE–SW, indicating that the sediments in the basin were supplied from geological bodies in the east, that is, the Xuefeng Orogenic Belt. The palaeocurrent directions in the western segment of the basin were aligned NW–SE, E–W and locally SW–NE, suggesting that the basin sediments were sourced from the Fanjingshan-Wuling Mountain Belt. Importantly, based on the palaeocurrent data in the Lower Cretaceous layers, it is evident that during Cretaceous time the depocentre of the basin was at Mayang and Tanxi areas, very close to the western segment of the basin. These features show that the basin grew to the west during Late Triassic – Early Cretaceous time, during which period the sediments were mainly derived from the Fanjingshan-Wuling Mountain Belt. Further, the palaeoflow directions, namely the NW-directed flow that was nearly perpendicular to the orogenic belts and the NE–SW-aligned direction of the palaeoflow that was parallel to the trends of the basin, are significant features of the flexural basin.

Fig. 9. Palaeocurrent directions plotted on the tectonic framework of the study area, measured from cross-bedding and imbricated conglomerate. The directions measured from imbricated conglomerate have been rotated 180°. Red – Late Triassic – Early Jurassic; Blue – Middle Jurassic; Yellow – Early Cretaceous. Abbreviations as for Figure 3.
Synthesizing the potential source area of detrital zircons, palaeocurrent data and rock fragments, and combining with the lithology column of the Xuefeng Orogenic Belt and the Fanjingshan-Wuling Mountain Belt, the sediments in the eastern segment of the Yuan-Ma Basin were predominantly supplied from the Xuefeng Orogenic Belt and in the western segment mainly from the Fanjingshan-Wuling Mountain Belt (Figs 8–10).

Fig. 10. The potential source areas of sediments deposited in the Yuan-Ma Basin at different sedimentary stages (the Xuefeng Orogenic Belt and Fanjingshan-Wuling Mountain Belt). The lithological column of the sedimentary rocks from the Xuefeng Orogenic Belt and the Fanjingshan-Wuling Mountain Belt are modified from BGMRGZ (1987) and BGMRHN (1988). Abbreviations as for Figure 3.
5.b. Timing of the Yuan-Ma Basin and deformation stage constraints in central South China
Numerous studies have suggested that the evolution of sedimentary basins is primarily controlled by tectonic events, and is a useful indicator of the evolution of the adjacent orogenic belt (e.g. Hendrix et al. Reference Hendrix, Graham, Amory and Badarch1996; Hendrix, Reference Hendrix2000; McCann & Saintot, Reference McCann, Saintot, McCann and Saintot2003; Cawood et al. Reference Cawood, Hawkesworth and Dhuime2012). Our detailed field investigations, detrital zircon analyses and palaeocurrent analyses provide constraints on the tectonic processes, formation times and evolution of the Yuan-Ma Basin. The formation of the Yuan-Ma Basin had undergone three stages of tectonic evolution (Fig. 11): the Late Triassic – Early Jurassic flexural basin, the Middle–Late Jurassic flexural basin, and the late Early Cretaceous extensional basin.
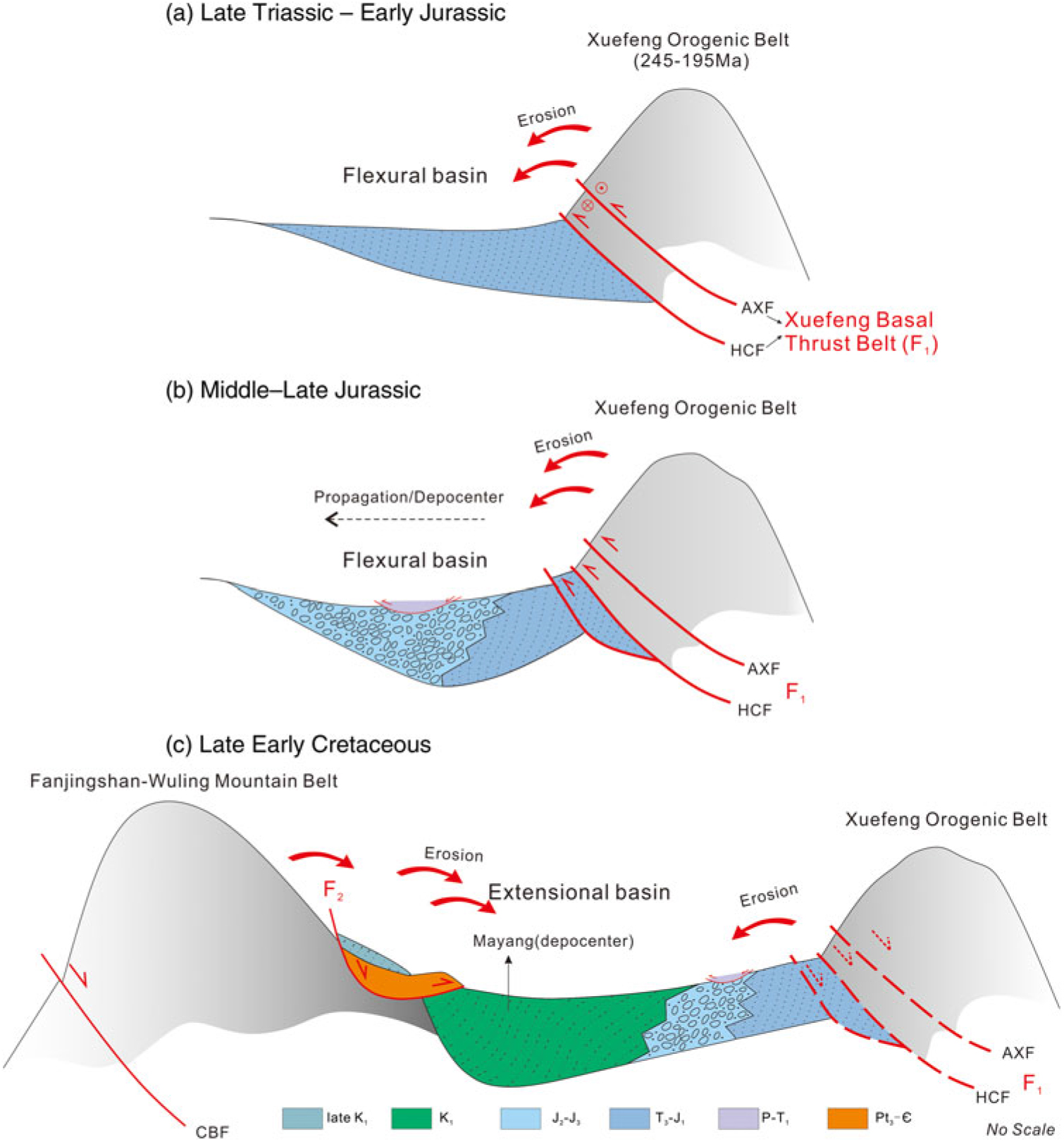
Fig. 11. Proposed model for the Late Triassic – Early Cretaceous evolution of the Yuan-Ma Basin. (a) The first stage of evolution resulted in the flexural basin, and the SE-dipping Xuefeng Basal Thrust Belt (F1) controlled the initial formation of the Yuan-Ma Basin during Late Triassic – Early Jurassic time. (b) The second stage of evolution involved a flexural basin controlled by WNW-vergent compression during Middle–Late Jurassic time. (c) The third stage of evolution generated an extensional basin controlled by the SE-dipping gravity-sliding fault (F2) during late Early Cretaceous time. Abbreviations as for Figure 3.
5.b.1. First stage of the Yuan-Ma Basin
A regional unconformity occurred between the Permian and Lower Triassic strata and the Upper Triassic – Lower Jurassic strata, suggesting that a tectonic event influenced this area at this time. This tectonic event, evidenced by the extensively deformed Permian – Lower Triassic limestones (Fig. 3a), is probably consistent with the NW–SE shortening Indosinian intracontinental orogeny developed in the Xuefeng Orogenic Belt (244–195 Ma, Wang et al. Reference Wang, Zhang, Fan and Peng2005; 245–215 Ma, Chu et al. Reference Chu, Lin, Faure, Wang and Ji2012b). The Upper Triassic – Lower Jurassic coal-bearing formation unconformably overlies the Permian – Lower Triassic dolomite and represents the time of maximum subsidence (Ettensohn, Reference Ettensohn2005), which means it began to form the flexural basin and deposited the sediments in the basin (Fig. 11a). In the field, the colour of the clastic rocks gradually changes from greyish-green to brick-red upwards through the stratigraphy, implying that the depositional environment regressed from a marine to a terrestrial environment, varying between reducing and oxidizing environments, suggesting that a tectonic event influenced this area (Fig. 2b1, b2). During Late Triassic – Early Jurassic time, the NE-trending and SE-dipping Anhua-Xupu Fault caused transpression (sinistral oblique thrusting) and had a strong influence on the Yuan-Ma Basin (Wang et al. Reference Wang, Zhang, Fan and Peng2005; Zhang et al. Reference Zhang, Ma, Yang, Chen, Lei, Wang and Li2010; Bai et al. Reference Bai, Zhong, Jia, Xiong and Huang2016). Due to the activities of the faults in the east and the erosion of the Xuefeng Orogenic Belt, the syn-tectonic sediments became the sedimentary detritus in the basin during Late Triassic – Early Jurassic time.
For the tectonic implication, the orogenies in South China and its surroundings occurred universally during Middle Triassic – Early Jurassic time (Fig. 12), for example: the 240–220 Ma collision along Qinling-Dabie Orogenic Belt (Wang et al. Reference Wang, Zhou, Liu, Li and Yang2018); the 240–208 Ma deformation in Longmenshan tectonic belt (Yan et al. 2011, Reference Yan, Zhou, Qiu, Wells, Mu and Xu2018); and the 245–195 Ma orogeny in Xuefeng Orogenic Belt (Wang et al. Reference Wang, Zhang, Fan and Peng2005; Chu et al. Reference Chu, Faure, Lin and Wang2012a, b, c). In central South China, the early Mesozoic intracontinental orogeny created the Xuefeng Orogenic Belt and terminated the marine sedimentary environment in South China. The reactivation of the Anhua-Xupu Fault and formation of the Yuan-Ma Basin were constrained by the diachronous progressive intracontinental deformation that occurred in the west of the Xuefeng Orogenic Belt during Late Triassic – Early Jurassic time (Wang et al. Reference Wang, Zhang, Fan and Peng2005; Zhang et al. Reference Zhang, Guo, Wang, Li, Dong, Liu, He, Cheng, Lu and Yao2013; Li et al. Reference Li, Santosh, Zhao, Zhang and Jin2012b).

Fig. 12. Three stages of tectonic evolution in other areas of South China. D1: early Mesozoic orogeny in South China and its surroundings (240–200 Ma collision along the Qinling-Dabie Orogenic Belt, Wang et al. Reference Wang, Zhou, Liu, Li and Yang2018; 240–208 Ma deformation in Longmenshan tectonic belt, Yan et al. 2011, Reference Yan, Zhou, Qiu, Wells, Mu and Xu2018; 245–195 Ma orogeny in Xuefeng Orogenic Belt, Wang et al. Reference Wang, Zhang, Fan and Peng2005; Chu et al. Reference Chu, Faure, Lin and Wang2012a, b, c). D2: Middle–Late Jurassic subduction of the west palaeo-Pacific plate( e.g. 180–160 Ma, Zhou & Li, Reference Zhou and Li2000). D3: Late Early Cretaceous intracontinental extension as a result of the subduction direction changes of the palaeo-Pacific plate (e.g. 130–120 Ma extensional tectonics in Wugongshan, Faure et al. Reference Faure, Sun, Shu, Monié and Charvet1996; Li et al. Reference Li, Zhang, Dong and Johnston2014).
5.b.2. Second stage of the Yuan-Ma Basin
During Middle–Late Jurassic time, the basin continuously grew to the west in the form of a flexural basin and sedimentary detritus was mainly derived from the Xuefeng Orogenic Belt (Figs 9, 11b). Through the field investigation, the Upper Triassic – Lower Jurassic layers underwent extensive deformation and developed large numbers of NNE-trending faults and fault-related folds (Fig. 3d–f). During Middle Jurassic time, the Anhua-Xupu and Huaihua-Yuanling faults both represented compression; at the same time, the tectonic system changed from the early Mesozoic orogeny to the subduction of the palaeo-Pacific plate (Ding et al. Reference Ding, Guo, Liu and Zhai2007; Zhang et al. Reference Zhang, Xu, Jia and Shu2009; Bai et al. Reference Bai, Zhong, Jia, Xiong and Huang2016), which led to the development of the thick conglomerates in the basin. In the field, the faults juxtaposed the Permian – Lower Triassic limestones over the Middle Jurassic conglomerates; the sedimentary hiatus, characterized by the unconformity between the Middle Jurassic and the Lower Cretaceous layers, occurred during Middle–Late Jurassic time, suggesting that the thrusting of the faults, the growth of the basin and the depocentre of the syn-tectonic sediments shifted westwards during Middle–Late Jurassic time (Fig. 3i, j).
Geodynamically, the oceanic plate subduction can provide the driving force for the formation of intracontinental contractional tectonics, for example, the Precordillera thin-skinned thrust belt and thick-skinned Pampeanas Ranges in the Andes (e.g. Jordan et al. Reference Jordan, Isacks, Allmendinger, Brewer, Ramos and Ando1983), and thin-skinned Sevier thrust belt and thick-skinned Laramide province in the western USA (e.g. Lacombe & Bellahsen, Reference Lacombe and Bellahsen2016). During 180–160 Ma, the tectonic domain changed from palaeo-Tethys oceanic sea closing to palaeo-Pacific plate subduction (Zhang et al. Reference Zhang, Xu, Jia and Shu2009). At this time, the palaeo-Pacific plate was being subducted beneath the Eurasia plate at a very low (flat-slab) angle (Zhou & Li, Reference Zhou and Li2000; Wang, Reference Wang2006; Wang et al. Reference Wang, Zhou and Li2011a, Reference Wang, Tsai, Zhou, Qiu and Sun2017; Kiminami & Imaoka, Reference Kiminami and Imaoka2013) (Fig. 12). The far-field effect of the NW subduction of the palaeo-Pacific plate created a 1300 km wide intracontinental orogenic belt characterized by NNE-trending folds and associated thrusts and NE-trending fold-and-thrust belt (MPDS in Fig. 1) (Li & Li, Reference Li and Li2007; Lin et al. Reference Lin, Wang and Chen2008; Zhang et al. Reference Zhang, Xu, Jia and Shu2009; Wang et al. Reference Wang, Tsai, Zhou, Qiu and Sun2017). The contractional event at this stage of the Yuan-Ma Basin therefore resulted from the intracontinental contraction deformation in South China.
5.b.3. Third stage of the Yuan-Ma Basin
During late Early Cretaceous time (120–100 Ma), the gravity-sliding fault controlled the growth of the basin and the sedimentary detritus was predominantly sourced from the Fangjingshan-Wuling Mountain Belt (Figs 9–11c). Some studies indicate that the Cili-Baojing Fault represented sinistral strike-slipping and thrusting during 190–130 Ma and extension during 120–100 Ma, which led to the uplift of the Fanjingshan-Wuling Mountain Belt and the development of extensional structures in the east side of the Fanjingshan-Wuling Mountain Belt (Xie et al. Reference Xie, Yang and Ma2006; Qi et al. Reference Qi, Zhai and Zhang2009). In the field, a gravity-sliding fault apparently occurred in the western segment of the basin characterized by normal faulting in the root zone and thrusting in the front zone (Fig. 2d1), which was consistent with the extensional setting during 120–100 Ma and controlled the final stage of the basin. The date of the gravity-sliding fault is c. 116 Ma. The syn-tectonic sediments, which were mainly sourced from the Fanjingshan-Wuling Mountain Belt and, to a lesser extent, from the Xuefeng Orogenic Belt, were deposited in the basin. It is also evident that the gravity-sliding fault is overlain by the syn-tectonic upper Lower Cretaceous rocks (Fig. 3r). Over the large scale, the intracontinental extension in eastern China is recorded by normal faults, metamorphic core complex and rift basins during Cretaceous time (e.g. Wang et al. Reference Wang, Zhou and Li2011a). For instance, the generation of Wugongshan dome was associated with several stages of extension during 130–120 Ma (Faure et al. Reference Faure, Sun, Shu, Monié and Charvet1996; Li et al. Reference Li, Zhang, Dong and Johnston2014) (Fig. 12). Some studies indicate that the increasing dip angle of subduction slab or the roll-back of the downgoing plate could explain extension of the lithosphere mantle and the oceanwards younging of Cretaceous extension-related magmatism (e.g. Zhou & Li, Reference Zhou and Li2000; Kiminami & Imaoka, Reference Kiminami and Imaoka2013; Li et al. Reference Li, Zhang, Dong and Johnston2014). This stage is therefore probably related to the subduction direction changes of the palaeo-Pacific plate triggered on extensional tectonics over the whole of eastern China (e.g. Sun et al. Reference Sun, Ding, Hu and Li2007; Wang et al. Reference Wang, Zhou and Li2011a, Reference Wang, Zhou, Liu, Li and Yang2018).
6. Conclusions
Analysis of stratigraphy and field structures indicates that the two early stages of the Yuan-Ma Basin evolution were controlled by the thrust fault in the east of the basin. The third stage of basin evolution involved the gravity-sliding fault that was dominant in the western segment of the basin during late Early Cretaceous time. The detrital zircon data yield significant age clusters at 3600–2700 Ma, 2700–2300 Ma, 2300–1900 Ma, 1900–1700 Ma, 1700–1050 Ma, 1050–900 Ma, 900–700 Ma, 500–350 Ma, 300–150 Ma and 120–100 Ma. The major age clusters are 900–700 Ma, 500–350 Ma and 300–150 Ma, as well as a minor age cluster at 120–100 Ma, which provide insights into the timing of tectonic activity in the Yuan-Ma Basin and sediment provenance. Stratigraphic sequences, detrital zircon data and palaeocurrent data suggest that the sediments of the Yuan-Ma Basin were sourced from the Xuefeng Orogenic Belt in the SE and the Fanjingshan-Wuling Mountain Belt in the NW. The evolution of the Yuan-Ma Basin was mainly controlled by three stages of tectonic activity. The first stage occurred in the eastern segment of the basin during Late Triassic – Early Jurassic time in a flexural basin setting. The second stage discontinuously occurred in the eastern segment of the basin during Middle–Late Jurassic time with a flexural basin shape. The third stage developed in the western segment of the basin during late Early Cretaceous time in an extensional basin. These three tectonic stages are connected to the diachronous progressive intracontinental deformation of central South China, the Middle–Late Jurassic subduction of the palaeo-Pacific Plate and the subduction direction changes of the palaeo-Pacific plate, respectively.
Author ORCIDs
Ma Huimin, 0000-0002-1439-3410
Acknowledgements
We greatly appreciate the constructive comments and suggestions from the editor Professor Olivier Lacombe, the reviewer Professor J Charvet and an anonymous reviewer. This study was financially supported by the National Natural Science Foundation of China (Grant No. 41430316). We thank Professor ZC Hu and Doctor PY Han for their assistance with processing age data during the laboratory analyses and Professor DF He for helpful discussions.
Supplementary material
To view supplementary material for this article, please visit https://doi.org/10.1017/S0016756819000451.