Introduction
The European eel Anguilla anguilla is an economically important species in Europe and globally (De Charleroy et al., Reference De Charleroy, Grisez, Thomas, Belpaire and Ollevier1990; Dekker, Reference Dekker, Aida, Tsukamoto and Yamaudi2003). During the past three decades, the European eel has declined drastically throughout its distribution (Dekker, Reference Dekker, Aida, Tsukamoto and Yamaudi2003). Habitat loss, climatic and oceanic changes, pollution, mortality due to river obstacles, overexploitation and parasites have been suggested as possible reasons for the decline (Dekker, Reference Dekker, Aida, Tsukamoto and Yamaudi2003; Kirk, Reference Kirk2003; Vogel, Reference Vogel2010; Pelster, Reference Pelster2015; Frisch et al., Reference Frisch, Davie, Schwarz and Turnbull2016).
European eel reproduction depends on a spawning migration of 5000–7000 km from the European coast to the Sargasso Sea in about 5 months. This long-distance journey causes great physiological stress on the eels including the swimbladder, a hydrostatic organ that provides neutral buoyancy (Sjöberg et al., Reference Sjöberg, Petersson, Wickström and Hansson2009; Pelster, Reference Pelster2015). Thus, any damage to swimbladder function could potentially interfere with the eel's ability to migrate and reproduce.
The nematode Anguillicoloides crassus (Kuwahara et al., Reference Kuwahara, Niimi and Itagaki1974) parasitizes the swimbladder of at least five eel species in addition to the European eel (Pratt et al., Reference Pratt, O'Connor, Stacey, Stanley, Mathers, Johnson, Reid, Verreault and Pearce2019) and is the most extensively studied parasite of anguillid eels (Lefebvre et al., Reference Lefebvre, Wielgoss, Nagasawa and Moravec2012). This nematode was accidentally introduced to Europe at the beginning of the 1980s by the importation of infected Japanese eels to Germany (Køie, Reference Køie1991). Transportation of eels for the global aquaculture trade has spread A. crassus throughout the world (ICES, 2010).
Numerous authors have suggested that the nematode A. crassus impairs swimbladder function and significantly compromises the success of the eels' spawning migration (Kirk, Reference Kirk2003; Pelster, Reference Pelster2015). Known pathological changes in eel swimbladder induced by A. crassus include lesions, haemorrhaging, infiltration of inflammatory cells, dilation of blood vessels, fibrosis, which increases the thickness of the swimbladder wall, the presence of granulocytes and macrophages around encysted parasite larvae, changes in epithelial cells and alteration of gas composition (Haenen et al., Reference Haenen, Grisez, De Charleroy, Belpaire and Ollevier1989, Reference Haenen, Van Banning and Dekker1994; Van Banning and Haenen, Reference Van Banning, Haenen, Perkins and Cheng1990; Molnár et al., Reference Molnár, Baska, Csaba, Glávits and Székely1993; Molnár, Reference Molnár1994; Molnár and Szèkely, Reference Molnár and Székely1995; Würtz and Taraschewski, Reference Würtz and Taraschewski2000; Knopf, Reference Knopf2006; Lefebvre et al., Reference Lefebvre, Fazio, Palstra, Székely and Crivelli2011, Reference Lefebvre, Wielgoss, Nagasawa and Moravec2012). Others have reported on the effects of A. crassus on the cellular and humoral immune response in A. anguilla swimbladder (Knopf et al., Reference Knopf, Naser, Van der Heijden and Taraschewski2000; Knopf, Reference Knopf2006; Terech-Majewska et al., Reference Terech-Majewska, Schulz and Siwichi2015) and on the physiological status of A. anguilla swimbladder in the presence of the nematode (Kelly et al., Reference Kelly, Kennedy and Brown2000; Sures et al., Reference Sures, Knopf and Kloas2001). The relationship between eel swimbladder and A. crassus infection has also been investigated by radiodiagnostic methods (Beregi et al., Reference Beregi, Molnár, Békési and Székely1998; Székely et al., Reference Székely, Molnar and Rácz2005), X-ray computerized tomography (Székely et al., Reference Székely, Molnár, Muller, Szabò, Romvávri, Hancz C and Bercsényi2004) and ultrasound scanning techniques (Frisch et al., Reference Frisch, Davie, Schwarz and Turnbull2016).
In addition to pathological changes caused by parasitization, the presence of A. crassus has been associated with a reduction in the distribution of oxygen to the swimbladder and changes in the mechanism of gas deposition, impeding swimbladder function (Würtz et al., Reference Würtz, Taraschewski and Pelster1996; Nimeth et al., Reference Nimeth, Zwerger, Würtz, Salvenmoser and Pelster2000; Pelster, Reference Pelster2015). Consequently, the nematode affects the vertical movement of its eel host and directly stresses the eel throughout migration, which could reduce the number of eels that reach the Sargasso Sea to spawn (Sprengel and Luchtenberg, Reference Sprengel and Luchtenberg1991; Kirk, Reference Kirk2003; Palstra et al., Reference Palstra, Heppener, Van Ginneken, Székely and Van den Thillart2007; Barry et al., Reference Barry, McLeish, Dodd, Turnbull, Boylan and Adams2014; Pelster, Reference Pelster2015). While parasitization by A. crassus is thought to be one reason for the decline of A. anguilla populations (Pelster, Reference Pelster2015), the mechanisms underlying the collapse are still uncertain, with other factors also likely contributing (Dekker, Reference Dekker, Aida, Tsukamoto and Yamaudi2003; Van Ginneken and Maes, Reference Van Ginneken and Maes2005).
Helminths are extraordinarily successful parasites because they can modulate the host immune response (Maizels et al., Reference Maizels, Smits and McSorley2018). In fish, the epithelia of gills, skin and the digestive tract form a physical barrier providing the first line of defence against infection (Koshio, Reference Koshio2016; Wang et al., Reference Wang, Lei, Gamil, Lagos, Yue, Schirmer, Mydland, Øverland, Krogdahl and Kortner2019). When this mucosal barrier is disturbed, resident immune cells are activated and tissue-specific leucocytes are recruited from the blood (Shi and Pamer, Reference Shi and Pamer2011; Tafalla et al., Reference Tafalla, Leal, Yamaguchi and Fischer2016; Salinas and Magadán, Reference Salinas and Magadán2017; Dezfuli et al., Reference Dezfuli, Castaldelli, Tomaini, Manera, DePasquale and Bosi2020). One of the most important sites of damage induced by adult and larval (L2) A. crassus is towards the inner-most layer of the swimbladder, which is formed by gas gland cells (Pelster, Reference Pelster, Hochachka and Mommsen1995; Maina, Reference Maina2000). This report includes a comparison between gas gland cells in infected vs uninfected swimbladders.
In many fishes, macrophages (Mulero et al., Reference Mulero, Noga, Meseguer, Garcia-Ayala and Mulero2008; Grayfer et al., Reference Grayfer, Kerimoglu, Yaparla, Hodgkinson, Xie and Belosevic2018; Mosberian-Tanha et al., Reference Mosberian-Tanha, Landsverk, Press, Mydland, Schrama and Overland2018) and mast cells (MCs) (Buchmann, Reference Buchmann2012; Secombes and Ellis, Reference Secombes, Ellis and Roberts2012; Galindo-Villegas et al., Reference Galindo-Villegas, Garcia-Garcia and Mulero2016; Dezfuli et al., Reference Dezfuli, Castaldelli, Tomaini, Manera, DePasquale and Bosi2020) are active in the immune response against parasites. Several authors have reported the occurrence of granulocytes and macrophages/macrophage aggregates (MAs) in eel swimbladders infected with A. crassus (Molnár et al., Reference Molnár, Baska, Csaba, Glávits and Székely1993; Molnár, Reference Molnár1994; Molnár and Szèkely, Reference Molnár and Székely1995; Würtz and Taraschewski, Reference Würtz and Taraschewski2000; Knopf, Reference Knopf2006) but no details have been provided previously about the type of granulocyte.
A set of extrinsic and intrinsic ganglia regulates the inflation/deflation of gas in the swimbladder, changing its volume in response to the fish's movements in the water column (Finney et al., Reference Finney, Robertson, McGee, Smith and Croll2006; Robertson et al., Reference Robertson, McGee, Dumbarton, Croll and Smith2007; Nilsson, Reference Nilsson2009). In addition to adrenergic and cholinergic pathways, other neurotransmitters have been documented in the nervous system components of the swimbladder, including met-enkephalin, substance P (SP), vasoactive intestinal peptide (VIP), neuropeptide Y (NPY), neuronal nitric oxide synthase (nNOS) and serotonin (5-hydroxytryptamine, 5-HT) (Lundin and Holmgren, Reference Lundin and Holmgren1984, Reference Lundin and Holmgren1989; Lundin, Reference Lundin1991, Reference Lundin1999; Schwerte et al., Reference Schwerte, Holmgren and Pelster1999; Finney et al., Reference Finney, Robertson, McGee, Smith and Croll2006; Robertson et al., Reference Robertson, McGee, Dumbarton, Croll and Smith2007; Nilsson, Reference Nilsson2009). This account represents the first immunohistochemistry survey on neuroendocrine components of eel swimbladders infected with A. crassus.
Materials and methods
A subpopulation of 32 A. anguilla with total length ranging from 34.5 to 63.6 cm (44.7 ± 1.1 cm, mean ± s.e.) and weighing from 60 to 500 g (162.1 ± 15. 2 g, mean ± s.e.) were collected in July 2019 in Lake Trasimeno (Central Italy, 43°9′11″N and 12°15′E) by professional fishermen of a local consortium using fyke nets. Trasimeno Lake is the largest lake in Italy, due to its shallowness. The eels were transferred alive to the consortium's facilities where they were euthanized with an overdose of MS222 (125 mg L−1, tricaine methanesulfonate, Sandoz, Basel, Switzerland). Once euthanized, the spinal cords were severed before the fish were dissected ventrally. The alimentary canal was removed to allow for the removal of the intact swimbladder from the eel's body.
Each swimbladder was opened longitudinally and several 15 × 15 mm pieces were excised and fixed in 10% neutral buffered formalin for 24 h. Thereafter, the samples were dehydrated through an alcohol series and then paraffin wax-embedded using a Shandon Citadel 2000 tissue processor. Multiple 5 μ m sections were taken from each tissue block, stained with Alcian Blue, or Haematoxylin and Eosin and/or Giemsa, and examined and photographed using a Nikon Microscope ECLIPSE 80i.
A panel of six rabbit polyclonal antibodies was chosen to study the neuroendocrine structures in swimbladders infected with A. crassus (Table 1). In our lab, we have frequently and successfully employed these antibodies to reveal nerve cell bodies and fibres (Dezfuli et al., Reference Dezfuli, Giari, Lorenzoni, Carosi, Manera and Bosi2018) and endocrine cells in the gut of numerous fish species (Dezfuli et al., Reference Dezfuli, Arrighi, Domeneghini and Bosi2000, Reference Dezfuli, Pironi, Giari, Domeneghini and Bosi2002; Bosi et al., Reference Bosi, Domeneghini, Arrighi, Giari, Simoni and Dezfuli2005a, Reference Bosi, Shinn, Giari, Simoni, Pironi and Dezfulib). The immunohistochemical reactions were made on sections of uninfected and infected eel swimbladder as reported in our previous work (Dezfuli et al., Reference Dezfuli, Giari, Lorenzoni, Carosi, Manera and Bosi2018). Negative controls were performed by incubating sections with: (a) phosphate-buffered saline instead of the primary antibody; or (b) preadsorbed antibody with its corresponding antigen as reported in Table 2. Sections were examined and photographed using a digital camera (Olympus Camedia C-5060, 5.1 Mp) and image analysis software (DP-software, Olympus, Milan, Italy). Evaluation of the frequency of the immunoreactive nervous and endocrine elements was based on subjective estimates after the examination of three sections from different regions for each uninfected and parasitized swimbladder.
Table 1. Rabbit polyclonal antibodies used in the present study, their source, dilution and results in eel swimbladders uninfected and infected with Anguillicoloides crassus

CGRP, calcitonin gene-related peptide; NPY, neuropeptide Y; nNOS, neuronal-nitric oxide synthase; 5-HT, 5-hydroxytriptamine (serotonin); SP, substance P; VIP, vasoactive intestinal peptide; UN, uninfected; INF, infected.
Frequencies of endocrine cells and nerve cell bodies and fibres immunoreactive to the indicated antibodies were relatively quantified by the symbols: +++: high presence, ++: medium presence, +: low presence, and −: no presence.
Table 2. Blocking peptides and amine used for preabsorption in the negative control reactions.

CGRP, calcitonin gene-related peptide; NPY, neuropeptide Y; nNOS, neuronal nitric oxide synthase; 5-HT, 5-hydroxytriptamine (serotonin); SP, substance P; VIP, vasoactive intestinal peptide; R. T., room temperature.
For transmission electron microscopy (TEM), numerous 7 × 7 mm pieces of infected and uninfected swimbladders were fixed and embedded according to the methods reported previously (Dezfuli et al., Reference Dezfuli, Giari, Lorenzoni, Carosi, Manera and Bosi2018). Portions of 10 infected and five uninfected swimbladders were fixed in cold 2.5% glutaraldehyde in 0.1 m sodium cacodylate buffer for 2.5 h and routinely processed for scanning electron microscopy (SEM). Observation was done with a Cambridge Stereoscan 360 at an acceleration voltage of 20 kV.
Results
Sixteen eels out of 32 (50%) harboured A. crassus in their swimbladder and the intensity of infection ranged from 2 to 17 adult nematodes per organ (6.9 ± 1.6, mean ± s.e.). In six heavily infected swimbladders, adults and over 200 L2 larvae co-occurred (see further). During autopsy, uninfected swimbladders had thin walls and appeared transparent with different regions of the organ easily recognizable (Fig. 1A). Swimbladders with adult A. crassus were darker and less transparent, with large nematodes visible through the organ's wall (Fig. 1B). In very heavily parasitized swimbladders (Fig. 1C), the organ was even darker and the surface in some parts was distended by large nematodes that occupied the entire luminal space of the organ (Fig. 1C). Upon excision of infected swimbladders, long (several millimetres), black worms inside the lumen were visible to the naked eye.
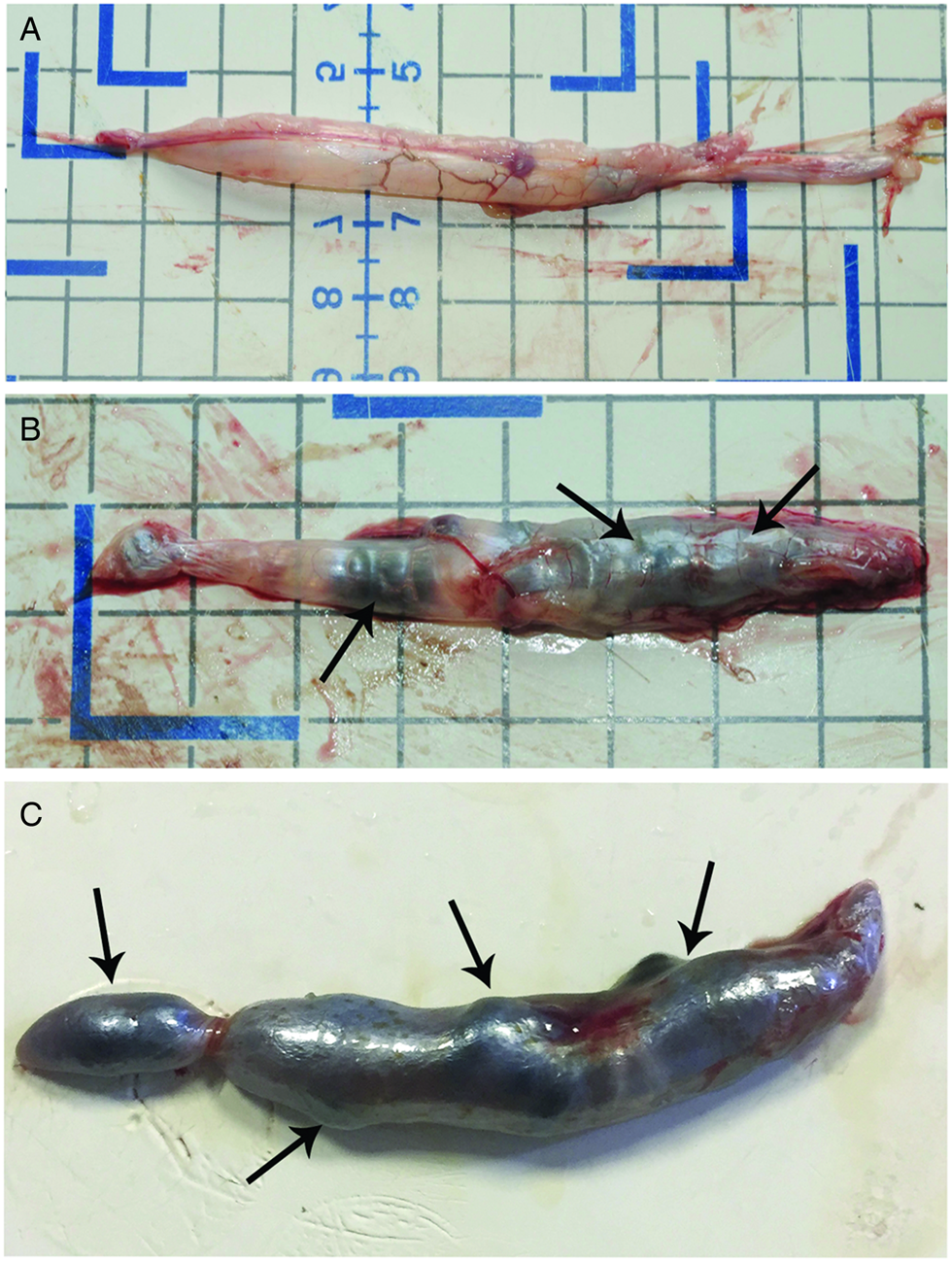
Fig. 1. Photos after necropsy of Anguilla anguilla. (A) Uninfected swimbladder, note transparency allowing one to see inside the organ. (B) Swimbladder with some preadult and adult Anguillicoloides crassus, worms (arrows) occupied most of the organ's lumen. (C) Very heavily infected swimbladder appeared very dark with a thick wall, large adult A. crassus occupied the entire luminal space and distended some parts of the organ surface (arrows).
The eel swimbladder consists of four distinct layers (Fig. 2A), with wall architecture similar to the anterior intestine, from which the swimbladder is derived. We use the terminology suggested by Fänge (Reference Fänge1953) for swimbladder layers. The innermost layer facing the swimbladder lumen is the mucosa; it has a simple cuboidal epithelium containing gas gland cells, which form folds and the lamina propria, rich in blood vessels. External to the mucosa is the muscularis mucosae. The third layer is the lamina submucosa with its loose network of connective elements, and the fourth layer is the lamina serosa, which forms the external covering of the swimbladder.

Fig. 2. Histological sections of A. anguilla swimbladder stained with Giemsa. (A) Sagittal section of uninfected swimbladder, note thin epithelium (arrow), M = lamina mucosa, mm = muscularis mucosae, SM = lamina submucosa, S = lamina serosa, Giemsa stain, scale bar = 50 μ m. (B) Transverse section of heavily infected swimbladder, hyperplasia of the main layers is remarkable, papillose aspect of folds (arrows) and nematode eggs in the lumen are evident (arrowheads), M = lamina mucosa, mm = muscularis mucosae, SM = lamina submucosa, scale bar = 50 μ m. (C) Infected swimbladder, abnormal dilation of blood vessels (arrows) visible far from nematode, an L3 (asterisk) in submucosa is surrounded with loose connective tissue (arrow heads), M = lamina mucosa, mm = muscularis mucosae, SM = lamina submucosa, scale bar = 100 μ m. (D) Higher magnification of dilated vessels (asterisks) of mucosal layer, A. crassus larvae (arrow heads), mm = muscularis mucosae, SM = lamina submucosa, scale bar = 20 μ m. (E) Intestine of an adult A. crassus is filled with eel erythrocytes (asterisk), numerous nematode larvae (arrow heads) are near the swimbladder epithelium, scale bar = 100 μ m. (F) An adult nematode (big asterisk), feeding activity of numerous larvae (arrows) eroded epithelium of the swimbladder folds (small asterisks) and residues of gas gland cells among larvae are visible, Giemsa stain, scale bar = 50 μ m.
Observations of histological sections of uninfected (Fig. 2A) and infected (Fig. 2B) swimbladders revealed remarkable structural differences. In uninfected organs, the epithelium was very thin (Fig. 2A). In contrast, swimbladders harbouring A. crassus showed hyperplasia of the lamina mucosa, submucosa and muscularis mucosae (Figs 2B and C). One of the most evident changes was the proliferation of epithelial cells and swimbladder folds that assumed a papillose aspect (Figs 2B and C). Dilation of blood vessels in the mucosal layer was common, far from any nematodes (Fig. 2C) and near parasite eggs (Fig. 2B) and larvae (Fig. 2D). In sections of some infected swimbladders, L2 and/or L3 larvae were observed in the lamina submucosa; often the larva was surrounded by loose connective tissue and host mononuclear cells (Fig. 2C). The intestines of adult nematodes were frequently filled with host erythrocytes and the adult worms often occupied most of the luminal space of the swimbladder (Fig. 2E). The presence and feeding activity of numerous larvae induced epithelial erosion; the residue of gas gland cells was seen among the larvae (Fig. 2F).
The adult nematodes induced inflammation in the swimbladder; numerous MCs were seen scattered among the gas gland cells and in the lamina propria near the blood vessels (Fig. 3A). Frequently, in the lamina propria, MCs were in degranulation (Fig. 3B); indeed, these cells were found around encapsulated larvae in the submucosal layer. MAs occurred among gas gland cells (Fig. 3C) and in the lamina propria close to the MCs and blood vessels (Fig. 3D). MAs contained azure-brownish pigments (Figs 3C and d) and in some cases were observed in the lamina serosa. In very heavily infected swimbladders, the supranuclear region of the gas gland cells was vacuolized (Figs 3B and D).

Fig. 3. Histological sections of A. anguilla swimbladder stained with Giemsa. (A) Arrow shows a mast cell among gas gland cells, numerous mast cells (arrow heads) around dilated blood vessel (asterisk), some mast cells are in degranulation (curved arrows), scale bar = 50 μ m. (B) Occurrence of macrophage aggregates (arrows) among gas gland cells, sections of larvae of A. crassus (arrow heads) in the lumen, scale bar = 100 μ m. (C) Macrophage aggregates in lamina propria (arrows) near mast cells (curved arrows), see vacuolization of apical part of gas gland cells (arrow heads), scale bar = 5 μ m.
Sections of infected/uninfected swimbladders were observed also by SEM. Figure 4A shows a luminal space occupied by a single adult female. Often adult worms were accompanied by numerous L2 larvae attached to the epithelium and/or free in the lumen and surrounded by secretion and host erythrocytes (Fig. 4B). In infected swimbladder, dilation of the blood vessels increased the wall thickness (Figs 4C and D) which ranged from 290 to 392 μ m (Fig. 4D). The walls of swimbladders not infected with A. crassus varied in thickness from 120 to 170 μ m (Fig. 4E).
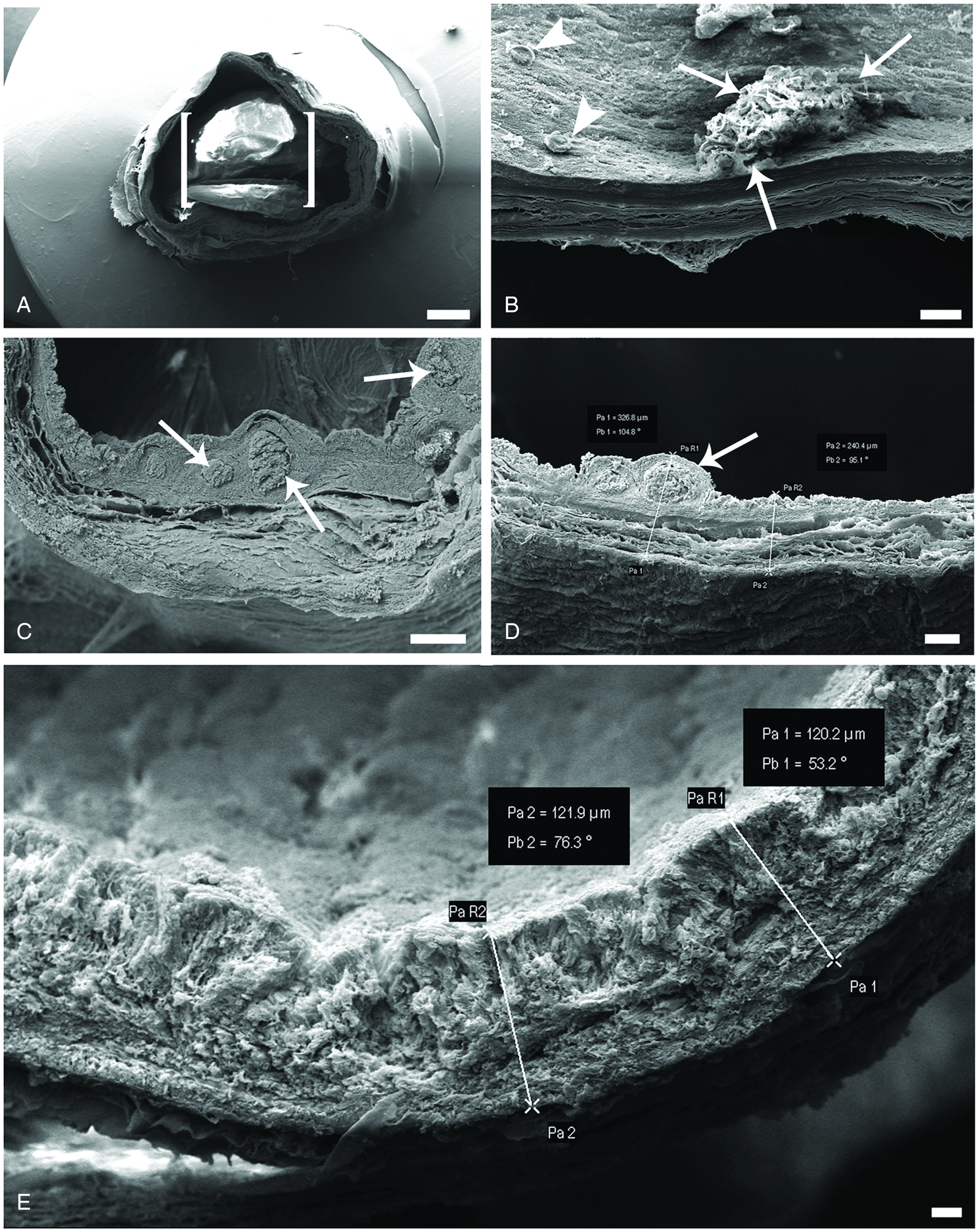
Fig. 4. Scanning electron micrographs of A. anguilla swimbladder. (A) Image of about 7 mm in length of infected swimbladder, one single adult A. crassus (parenthesis) occupied most luminal space of this portion, scale bar = 1 mm. (B) Some single larvae (arrow heads) and numerous larvae covered with secretion (arrows) are attached to the epithelium of swimbladder, scale bar = 100 μ m. (C) Heavily infected swimbladder, image of dilated blood vessels (arrows), scale bar = 200 μ m. (D) Micrograph shows piece of infected swimbladder, with dilated vessels (arrows) and increased thickness of swimbladder wall, scale bar = 100 μ m. (E) Image of uninfected swimbladder, note thinner wall, scale bar = 20 μ m.
TEM revealed ultrastructural differences between infected and uninfected swimbladders. In organs with no A. crassus, gas gland cells were intact, cuboidal in shape, with the nucleus with euchromatin and heterochromatin apposed to the nuclear envelope, with short microvilli on the luminal side, well-developed basolateral labyrinths (Fig. 5A) and clear apical junctional complexes (Fig. 5B). Within the cytoplasm, very few electron-dense vesicles and some round-oval mitochondria with normal cristae (not shown) were observed. In lightly infected swimbladders, short microvilli persisted, translucid vesicles were frequent within the cytoplasm of gas gland cells, and empty spaces were common between the cells; nonetheless, basolateral labyrinths assumed a vertical position between cells (Fig. 5C). Moreover, the presence of MCs among gas gland cells and within the lamina propria was common (respectively Figs 5C and D). In heavily infected swimbladders, epithelial dysplasia was noticed, gas gland cells assumed a bubble-shaped aspect and their supranuclear region showed intense vacuolization (Figs 5E and F). In swimbladders with several worms, the nuclei changed shape, had more heterochromatin and some were necrotic, and the mitochondria were much more electron-dense with short undefined cristae (not shown). Between gas gland cells, abundant empty spaces and the lack of a distinct apical junctional complex were common and labyrinths moved to the top of the epithelium (Fig. 5F). Indeed, in heavily parasitized swimbladders, gas gland cell degeneration was remarkable and the MCs were scattered among the epithelial cells residue (Fig. 5F).

Fig. 5. Transmission electron microscopy micrographs of A. anguilla swimbladder. (A) Uninfected swimbladder, note normal aspect of three gas gland cells (asterisks), short microvilli (arrow heads) and lack of empty spaces between cells, arrows show basolateral labyrinths, scale bar = 3 μ m. (B) Uninfected swimbladder, high magnification of apical junctional complex (arrows) between adjacent gas gland cells, scale bar = 0.2 μ m. (C) Slightly infected swimbladder, between gas gland cells (asterisks), empty spaces and translucid vesicles in cytoplasm are visible, displacement of basolateral labyrinths (arrows) in vertical position and two mast cells (curved arrows) among gas gland cells are evident, scale bar = 2.6 μ m. (D) Occurrence of mast cells with electron-dense granules within the lamina propria, degranulation (curved arrows) is visible, scale bar = 2 μ m. (E) Epithelium of heavily infected swimbladder, note intense vacuolization-degeneration of apical part of gas gland cells, necrotic nucleus (arrow) and A. crassus larva (curved arrow) in swimbladder lumen, scale bar = 5.2 μ m. (F) Epithelium of heavily infected swimbladder, gas gland cells (asterisks) vacuolization-degeneration, absence of distinct apical junctional complex and basolateral labyrinths are visible, a mast cell (curved arrow) among the residue of gas gland cells, scale bar = 2.7 μ m.
In uninfected swimbladders, the nervous components were not immunoreactive to antibodies to NPY, 5-HT and SP (Table 1, Figs 6A and b). In contrast, they showed a positive reaction to antibodies to calcitonin gene-related peptide (CGRP), nNOS and VIP (Table 1, Fig. 6C) in single small ganglia and slender nerve fibres interspersed in the submucosa (Fig. 6C). In infected organs, we observed large ganglia with several anti-CGRP immunoreactive neurons, and a high number of positive nerve fibres (Figs 6D and E). Several neurons and nerve bundles were immunoreactive to the antibody to NOS, both under the mucosa (Fig. 6F) and close to the blood vessels (Fig. 6G). Ganglia with anti-5-HT immunoreactive neurons were seen in the walls of infected swimbladders (Fig. 6H). Anti-VIP immunoreactive neurons in large ganglia and numerous nerve fibres were observed in the connective tissue of the submucosa layer (Fig. 6I) and close to the blood capillaries (Fig. 6J) of the swimbladder. In the epithelium of uninfected swimbladders, endocrine cells were not observed to react to any of the antibodies used (Table 1). Conversely, a high number of endocrine cells immunoreactive to the antibodies to 5-HT and NPY were observed in the epithelium of very heavily infected swimbladders (Table 1, Fig. 7).

Fig. 6. Nervous components of the eel swimbladder uninfected (A–C) and infected with Anguillicoloides crassus (D–J). (A) A ganglion with no immunoreactive neurons (arrows) to the anti-5-HT. Scale bar = 50 μ m. (B) Neurons (arrows) and nerve fibres (arrowhead) with negative immunoreactivity to the anti-NPY. Scale bar = 100 μ m. (C) A little ganglion with three neurons (arrow) positive to the anti-VIP surrounded by unreactive nervous fibres (arrowhead); ep, epithelium; rm, rete mirabile. Scale bar = 100 μ m. (D) A large ganglion with several neurons (arrows) immunoreactive to anti-CGRP; arrowhead shows a negative bundle of nervous fibres. Scale bar = 100 μ m. (E) Region of the swimbladder wall with a high number of anti-CGRP immunoreactive nervous fibres (arrowheads). Scale bar = 100 μ m. (F) A group of neurons (arrows) and nervous fibres (arrowhead) immunoreactive to anti-n-NOS; the oedematous epithelium shows numerous blood capillaries (asterisk); parasite larvae (curved arrows) are in the lumen with tissue debris and erythrocytes. Scale bar = 100 μ m. (G) Two bundles of nerve fibres (arrowheads) and a little neuron (arrow) immunoeactive to anti-n-NOS close to a blood vessel (asterisk). Scale bar = 50 μ m. (H) A ganglion with neurons (arrows) immunoreactive to anti-5-HT; curved arrows show blood capillaries in the epithelium. Scale bar = 50 μ m. (I) Several neurons (arrows) immunoreactive to anti-VIP in a ganglion within the submucosal layer of the swimbladder wall, epithelium (ep). Scale bar = 100 μ m. (J) Many nerve fibres immunoreactive to anti-VIP (arrowheads) close to the blood vessels (asterisks). Scale bar = 100 μ m.

Fig. 7. Endocrine cells belonging to the diffuse endocrine system in the epithelium of the eel swimbladder infected with Anguillicoloides crassus. (A) Several endocrine cells immunoreactive to anti-5-HT (arrows); asterisk indicates a dilated blood vessel. Scale bar = 200 μ m. (B) High magnification shows three endocrine cells positive to anti-5-HT. Scale bar = 20 μ m. (C) Numerous endocrine cells (arrows) immunoreactive to anti-NPY; note erosion of lamina mucosa due to action of parasite larvae (curved arrows); asterisk indicates a blood vessel. Scale bar = 200 μ m. The inset shows a high magnification of the immunoreactive endocrine cells. Scale bar = 50 μ m.
Discussion
In this study, 50% of eels sampled from Lake Trasimeno were infected with the nematode A. crassus, with some swimbladders completely filled with an impressive number of large adults and a few hundred larvae. Under natural conditions, the presence of so many A. crassus larvae in the swimbladder could be a considerable stressor for eels (Sures et al., Reference Sures, Knopf and Kloas2001).
In eels, gas gland cells are responsible for initiating gas secretion and play a crucial role in the metabolism of swimbladder tissue (Pelster, Reference Pelster, Hochachka and Mommsen1995; Würtz et al., Reference Würtz, Taraschewski and Pelster1996; Barry et al., Reference Barry, McLeish, Dodd, Turnbull, Boylan and Adams2014). Thus, understanding the effects of parasites on these critical cells will contribute to our understanding of the subsequent effects on migration. Previously, Würtz and Taraschewski (Reference Würtz and Taraschewski2000) had provided the only ultrastructural survey of the effects of A. crassus on eel swimbladder gas gland cells. The results reported here support those earlier findings and further our understanding with several new observations, including vacuolization of the apical part of the gas gland cells and thus their disintegration, necrotic nuclei of some disintegrating cells, displacement of the basal labyrinth towards the top of the epithelium, the presence of many empty spaces among gas gland cells and a lack of defined apical junctional complexes. The combination of larval A. crassus feeding on tissue and inducing degeneration of the gas gland cells (Kirk, Reference Kirk2003, current study) and the presence of adult worms in the lumen reduce the gas-secreting capacity of gas gland cells and swimbladder wall elasticity (respectively Würtz et al., Reference Würtz, Taraschewski and Pelster1996; Würtz and Taraschewski, Reference Würtz and Taraschewski2000; Barry et al., Reference Barry, McLeish, Dodd, Turnbull, Boylan and Adams2014).
Most previous reports on the cellular immune response in the eel swimbladder–A. crassus system dealt with the presence of granulocytes and macrophages around larvae in the wall of the stomach, intestine or swimbladder (Haenen et al., Reference Haenen, Grisez, De Charleroy, Belpaire and Ollevier1989; Molnár et al., Reference Molnár, Baska, Csaba, Glávits and Székely1993; Molnár, Reference Molnár1994; Würtz and Taraschewski, Reference Würtz and Taraschewski2000; Knopf, Reference Knopf2006). Two very important components of the innate immune system are MAs and MCs; in this report, we have documented high numbers of the above components in the mucosal layer of heavily infected swimbladders. Therefore, below we will examine in turn MAs and MCs.
Phagocytosis is the primary defence mechanism of all metazoan organisms (Grayfer et al., Reference Grayfer, Kerimoglu, Yaparla, Hodgkinson, Xie and Belosevic2018). MAs are groups of pigmented phagocytes primarily found in kidney, spleen and liver; MAs are reported in more than 130 fish species and are active in immune defences as well as in normal physiological processes (Steinel and Bolnick, Reference Steinel and Bolnick2017). The intestine possesses the largest pool of MAs, which is essential for epithelial renewal (Bain and Mowat, Reference Bain and Mowat2014). The presence of MAs in infected eel swimbladders has been reported previously (Molnár, Reference Molnár1994; Würtz and Taraschewski, Reference Würtz and Taraschewski2000; Knopf, Reference Knopf2006; Lefebvre et al., Reference Lefebvre, Wielgoss, Nagasawa and Moravec2012), but no insight was provided on a reason for their occurrence in this organ and Munoz et al. (Reference Munoz, Peñalver, Ruiz de Ybañez and Garcia2015) reported a decrease in the number of MAs in eel swimbladders harbouring A. crassus, compared with uninfected controls. In the current study, we saw numerous MAs in the mucosal layer of infected swimbladders, and one interesting finding of this study was the presence of intraepithelial MAs. In some cases, intraepithelial MAs occurred very close to nematode larvae and eggs adhered to the gas gland cell surface. It has been reported that turtle MAs are aggressive phagocytes, attacking bacteria, fungi and helminth eggs in vitro (Johnson et al., Reference Johnson, Schwiesow, Ekwall and Christiansen1999). Recently in the intestine of Chelon ramada, a high number of intraepithelial macrophages engulfing spores of Myxobolus mugchelo (Myxozoa) was observed by Dezfuli et al. (Reference Dezfuli, Castaldelli, Tomaini, Manera, DePasquale and Bosi2020). MAs regulate inflammatory response and protect mucosa against pathogens and scavenge dead cells and foreign debris (Estensoro et al., Reference Estensoro, Mulero, Redondo, Álvarez-Pellitero, Mulero and Sitjà-Bobadilla2014). Because feeding activity of larval and adult A. crassus damage epithelial cells of eel swimbladder, intraepithelial MAs might be necessary to phagocytose the residues of damaged gas gland cells.
In heavily infected eel swimbladders, numerous MCs were encountered in the mucosal layer, among gas gland cells and in lamina propria close to blood vessels. To regulate inflammation and coordinate an appropriate response against pathogens, in all vertebrates MCs are strategically placed at perivascular sites (Secombes and Ellis, Reference Secombes, Ellis and Roberts2012; Dezfuli et al., Reference Dezfuli, Giari, Lorenzoni, Carosi, Manera and Bosi2018). By degranulation, MCs release their granules (Bosi et al., Reference Bosi, DePasquale, Manera, Castaldelli, Giari and Dezfuli2018; Dezfuli et al., Reference Dezfuli, Castaldelli, Tomaini, Manera, DePasquale and Bosi2020), which contain a wide spectrum of inflammatory and immunomodulatory mediators such as α-N-acetyl-galactosamine (Dezfuli et al., Reference Dezfuli, Bo, Lorenzoni, Shinn and Giari2015), piscidins (Salger et al., Reference Salger, Reading and Noga2017), histamine (Gomez Gonzalez et al., Reference Gomez Gonzalez, Cabas, Montero, Garcia Alcazar, Mulero and Garcia Ayala2017) and serotonin (Da Silva et al., Reference Da Silva, Simões and Gutierre2017). There is a dearth of reports on the co-occurrence of MCs and MAs in the epithelium of infected organs of fish. In this survey, we documented a high number of MCs and several MAs in the epithelium and in the rest of the mucosal layer of highly infected eel swimbladders. The same phenomenon was encountered in the intestines of Silurus glanis harbouring an acanthocephalan (Dezfuli et al., Reference Dezfuli, Manera, DePasquale, Pironi and Giari2017) and in the gut of mullet heavily infected with a myxozoan (Dezfuli et al., Reference Dezfuli, Castaldelli, Tomaini, Manera, DePasquale and Bosi2020). Our previous and current results strongly favour the hypothesis that eels use these two types of immune cells to face the extensive attack by A. crassus on the swimbladder mucosal layer.
The autonomic nervous system regulates the inflation/deflation of gas in the swimbladder (Finney et al., Reference Finney, Robertson, McGee, Smith and Croll2006; Robertson et al., Reference Robertson, McGee, Dumbarton, Croll and Smith2007; Nilsson, Reference Nilsson2009; Dumbarton et al., Reference Dumbarton, Stoyek, Croll and Smith2010). In uninfected swimbladders, nerve fibres were thin and few in number and intramural ganglia were small, consisting of one to three neurons, and they were sometimes negative and in several occasions immunoreactive to anti-CGRP, -VIP and -nNOS. Accounts on the positive reaction of the nerve cell bodies of the fish swimbladder to VIP and nNOS have been reported previously (Lundin and Holmgren, Reference Lundin and Holmgren1984, Reference Lundin and Holmgren1989; Lundin, Reference Lundin1991; Schwerte et al., Reference Schwerte, Holmgren and Pelster1999; Finney et al., Reference Finney, Robertson, McGee, Smith and Croll2006; Robertson et al., Reference Robertson, McGee, Dumbarton, Croll and Smith2007). The immunoreactivity to anti-CGRP antibody reported here is the first for nerve cell bodies and fibres in a fish swimbladder. In fish, CGRP is involved in the control of gut peristalsis, by an inhibitory effect on the intestinal smooth muscle (Ceccotti et al., Reference Ceccotti, Giaroni, Bistoletti, Viola, Crema and Terova2018), in vasodilatation (Shahbazi et al., Reference Shahbazi, Holmgren and Jensen2009) and in the regulation of body fluid homoeostasis. Several studies have shown the immunoreactivity of the nervous components of the fish swimbladder to NPY, 5-HT and SP as neurotransmitters (Lundin and Holmgren, Reference Lundin and Holmgren1984, Reference Lundin and Holmgren1989; Lundin, Reference Lundin1991; Finney et al., Reference Finney, Robertson, McGee, Smith and Croll2006; Robertson et al., Reference Robertson, McGee, Dumbarton, Croll and Smith2007; Pereira et al., Reference Pereira, Rodrigues de Freitas, Cardoso de Oliveira, Santos Costa, Andrés Vigliano and Vieira Rosa2017). We failed to find these neuromodulators in neurons of the uninfected swimbladders. Regarding the absence of NPY in swimbladders of adult zebrafish, Robertson et al. (Reference Robertson, McGee, Dumbarton, Croll and Smith2007) stated that, ‘….cells noted in this study had either disappeared by adulthood, or that they continued to be present but did not contain detectable levels of NPY’. Thus, the above suggestion could be true also for uninfected eel swimbladders, that is, probably in the absence of the parasite, the eel swimbladder does not produce a detectable quantity of NPY.
In infected swimbladders, large ganglia were noticed in the submucosa and close to smooth muscle fibres, with neurons immunoreactive to anti-CGRP, -nNOS, -VIP and -5-HT antibodies. The network of nerve fibres immunoreactive to these antibodies was dense, especially in layers rich with blood vessels. The increase in the number of nNOS-immunoreactive neurons was related to the synthesis of nitric oxide, and it was reported as a strong vasodilator of the blood vessels in the eel swimbladder (Schwerte et al., Reference Schwerte, Holmgren and Pelster1999). With regard to VIP, it has a vasodilatory effect, increasing the flow through the gas gland cells (Lundin and Holmgren, Reference Lundin and Holmgren1984; Schwerte et al., Reference Schwerte, Holmgren and Pelster1999; Finney et al., Reference Finney, Robertson, McGee, Smith and Croll2006) and thus increasing the swimbladder volume. Moreover, VIP causes the relaxation of smooth muscle in swimbladders of eel and zebrafish (respectively, Lundin, Reference Lundin1991; Finney et al., Reference Finney, Robertson, McGee, Smith and Croll2006), whereas 5-HT activated smooth muscle contraction in the eel swimbladder (Lundin, Reference Lundin1991). Herein, in swimbladders harbouring A. crassus, changes to the pattern of intramural nervous system with contrasting signals were reported for the first time. Based on the known roles of these compounds in swimbladders of eels and other bony fish, we hypothesize that the observed differences are attributable to the occurrence of A. crassus.
In eel swimbladders harbouring several adults and numerous larvae of A. crassus, many endocrine cells immunoreactive to anti-NPY and -5-HT antibodies were observed within the epithelium. In contrast, no endocrine cells positive to the above antibodies were observed in the epithelium of swimbladders with very few adults and with no larvae in the lumen. About 90% of the body's 5-HT is produced by enterochromaffin cells in the gut, which reveals the importance of this amine in gut function (Wang et al., Reference Wang, Sharkey and McKay2018). Lundin and Holmgren (Reference Lundin and Holmgren1989) reported the expression of 5-HT in the swimbladder epithelial cells of four fish species. Serotonin is one of the most important mediator molecules in inflammation caused by helminths (Dezfuli et al., Reference Dezfuli, Arrighi, Domeneghini and Bosi2000, Reference Dezfuli, Pironi, Giari, Domeneghini and Bosi2002; Bosi et al., Reference Bosi, Domeneghini, Arrighi, Giari, Simoni and Dezfuli2005a; Wang et al., Reference Wang, Sharkey and McKay2018). Recently, Gonzáles-Stegmaier et al. (Reference Gonzáles-Stegmaier, Villarroel-Espíndola, Manríquez, López, Monrás, Figueroa, Enríquez and Romero2017) have demonstrated the pro-inflammatory effects of NPY in the SHK-1 cell line and head kidney of the Atlantic salmon Salmo salar. In heavily infected swimbladder of eels, the occurrence of numerous positive cells to anti-NPY and -5-HT antibodies might suggest their involvement in inflammatory response against helminths, as they are active in immune cells recruitment and host local defence (Bosi et al., Reference Bosi, Domeneghini, Arrighi, Giari, Simoni and Dezfuli2005a; Gonzáles-Stegmaier et al., Reference Gonzáles-Stegmaier, Villarroel-Espíndola, Manríquez, López, Monrás, Figueroa, Enríquez and Romero2017; Dezfuli et al., Reference Dezfuli, Giari, Lorenzoni, Carosi, Manera and Bosi2018; Wang et al., Reference Wang, Sharkey and McKay2018).
Acknowledgements
We would like to thank Dr M. Manera from the University of Teramo (Italy) for helpful comments on an initial draft of the ms. We are indebted to the Centre of Electron Microscopy of the University of Ferrara for technical assistance.
Financial support
This study was supported by grants from the University of Ferrara to B.S.D. (FAR 2019).
Conflict of interest
None.
Ethical standards
This study was conducted in accordance with the national law (D.L. 4 March 2014, n. 26) and was approved by the Ferrara University Ethical Committee (TLX n. 2/2018).