Introduction
Intraindividual variability (IIV) is a measure of transient and rapid fluctuations or changes in an individual's performance during brief periods of time (e.g., trial-to-trial). IIV or inconsistency in neurocognitive speed is a known behavioral marker of aging-related cognitive decline (e.g., Fozard, Vercryssen, Reynolds, Hancock, & Quilter, Reference Fozard, Vercryssen, Reynolds, Hancock and Quilter1994; Hultsch, Strauss, Hunter, & MacDonald, Reference Hultsch, Strauss, Hunter and MacDonald2008; Li, Aggen, Nesselroade, & Baltes, Reference Li, Aggen, Nesselroade and Baltes2001) and greater inconsistency is found in individuals with dementia (e.g., Gorus, De Raedt, Lambert, Lemper, & Mets, Reference Gorus, De Raedt, Lambert, Lemper and Mets2008; Hultsch, MacDonald, Hunter, Levy-Bencheton, & Strauss, 2000), mild cognitive impairment (e.g., Dixon et al., Reference Dixon, Garrett, Lentz, MacDonald, Strauss and Hultsch2007), following traumatic brain injury (e.g., Collins & Long, Reference Collins and Long1996; Stuss, Pogue, Buckle, & Bondar, Reference Stuss, Pogue, Buckle and Bondar1994) and Parkinson's disease (PD) (Burton, Strauss, Hultsch, Moll, & Hunter, Reference Burton, Strauss, Hultsch, Moll and Hunter2006; Camicioli, Weiler, de Frias, & Martin, Reference Camicioli, Weiler, de Frias and Martin2008; Crawford, Goodrich, Henderson, & Kennard, Reference Crawford, Goodrich, Henderson and Kennard1989; de Frias, Dixon, Fisher, & Camicioli, Reference de Frias, Dixon, Fisher and Camicioli2007).
The catecholaminergic modulation model (Li & Lindenberger, Reference Li and Lindenberger1999; Li, von Oertzen, & Lindenberger, Reference Li, von Oertzen and Lindenberger2006) is a theoretical framework that links inconsistency in performance to the noisier aging brain. The age-related decline in the concentration of catecholamines in the basal ganglia partly accounts for the greater inconsistency in performance in both normal aging and neurological disordered older adults, as compared to younger or healthy controls, respectively. In addition to deterioration of neurotransmitters, other neural correlates of IIV include changes in anatomical, functional, and neuromodulatory characteristics such as smaller corpus collosum area, increased brain activity, variability in BOLD activity, and decreased D2 binding potential (e.g., Anstey et al., Reference Anstey, Mack, Christensen, Li, Reglade-Meslin, Maller and Sachdev2007; Bellgrove, Hester, & Garavan, Reference Bellgrove, Hester and Garavan2004; Garrett, Kovacevic, McIntosh, & Grady, Reference Garrett, Kovacevic, McIntosh and Grady2010; MacDonald, Li, & Bäckman, Reference MacDonald, Li and Bäckman2009). In PD patients, cognitive impairment which is nonresponsive to levodopa is associated with cortical and striatal atrophy (Bouchard et al., Reference Bouchard, Malykhin, Martin, Hanstock, Emery, Fisher and Camicioli2008; Camicioli et al., Reference Camicioli, Gee, Bouchard, Fisher, Hanstock, Emery and Martin2009). These structural changes may indicate the presence of cortical pathology which might affect performance and predict progression to dementia in PD. Performance variability may be a reliable marker of incipient dementia in PD.
Age-related increases in inconsistency on various cognitive tasks have mainly been documented cross-sectionally in healthy and neurologically impaired older adults. Evidence of longitudinal changes in IIV in aging is sparse (see Bielak, Hultsch, Strauss, MacDonald, & Hunter, Reference Bielak, Hultsch, Strauss, MacDonald and Hunter2010; Deary & Der, Reference Deary and Der2005; Fozard et al., Reference Fozard, Vercryssen, Reynolds, Hancock and Quilter1994; Lövdén, Li, Shing, & Lindenberger, Reference Lövdén, Li, Shing and Lindenberger2007; MacDonald, Hultsch, & Dixon, Reference MacDonald, Hultsch and Dixon2003). However, no known study has examined longitudinal changes in IIV in patients with PD. In our cross-sectional work on PD, we found that two patient groups (treated and untreated PD), showed greater IIV in neurocognitive speed as compared to matched controls (Camicioli et al., Reference Camicioli, Weiler, de Frias and Martin2008; de Frias et al., Reference de Frias, Dixon, Fisher and Camicioli2007). de Frias and colleagues (2007) also reported stronger motor-cognitive coupling between inconsistency in neurocognitive speed and executive dysfunction in severe PD patients. Support for the link between tasks that recruit the prefrontal cortex and show IIV derive from functional imaging investigations demonstrating associations between inconsistency and brain activations (Bellgrove et al., Reference Bellgrove, Hester and Garavan2004; Kelly, Uddin, Biswal, Castellanos, & Milham, Reference Kelly, Uddin, Biswal, Castellanos and Milham2008). An over-arching goal in the present study is to extend our earlier findings to examine longitudinal (18-month) changes in mean rate and IIV in speed tasks for two groups of PD patients (with and without incipient dementia) and controls.
PD is a common neurodegenerative movement disorder in older adults, and is associated with an increased risk of dementia (Aarsland, Zaccai, & Brayne, Reference Aarsland, Zaccai and Brayne2005). Early and accurate detection of the onset of dementia in PD would facilitate clinical management of the disorder. Known risk factors for PD with incipient dementia (PDID) include older age, male gender, visual hallucinations, and duration of symptoms (Galvin, Pollack, Morris, Reference Galvin, Pollack and Morris2006; Hobson & Meara, Reference Hobson and Meara2004). Ventricular enlargement, reflecting cortical atrophy, also occurs in PD patients with incipient dementia (Camicioli et al., Reference Camicioli, Sabino, Gee, Bouchard, Fisher, Hanstock and Martin2011). We examine whether concurrent and longitudinal changes in average latency and level of IIV are greater in incipient PDID than (a) PD without dementia or (b) controls. In a related cross-sectional study, Burton et al. (Reference Burton, Strauss, Hultsch, Moll and Hunter2006) showed that AD patients were more inconsistent than non-demented PD patients and controls. Our study is the first to examine these issues comparatively in PDID and PD (non-demented) patients, as well as controls.
Specifically, we addressed two main issues. First, we compared longitudinal (18-month) changes in mean rate of neurocognitive speed in controls, as well as PD and PDID. Since reduced speed is a common hallmark of parkinsonism, older PD patients would be expected to show increasingly slower performance over time than controls. We expected neurological complication of incipient dementia to exacerbate this slowing trend over time. Second, we compared longitudinal changes of IIV in neurocognitive speed among the three groups. We hypothesized that (a) PD patients would show an increase in variability compared to controls and (b) greater 18-month longitudinal changes in IIV for those PD patients in an incipient dementia period than for those PD patients who remained stable and non-demented at a later (36-month) follow-up assessment.
Method
Participants
Original sample
At Time (T1), there were 45 PD patients (26 men and 19 women) with a clinical diagnosis of idiopathic PD. All PD participants met UK brain bank criteria for idiopathic PD (Gibb & Lees, Reference Gibb and Lees1988), with the exception of allowing patients with symmetrical disease, without tremor and with upgoing plantar responses. All PD patients and controls showed no evidence of clinical dementia and all attained scores above 26/30 on the Mini-Mental Status Examination (MMSE) (Folstein, Folstein, & McHugh, Reference Folstein, Folstein and McHugh1975), and 125 or above (cutoff score is 123) on the Dementia Rating Scale (DRS) (Brown et al., Reference Brown, Rahill, Gorell, McDonald, Brown and Sillanpaa1999) at baseline. The PD patients ranged from 65 to 84 years (M = 71.3; SD = 4.5); the mean level of education was 14.0 years (SD = 3.0). The PD patients were recruited from the Movement Disorders Clinic at the University of Alberta or via advertisement in the Parkinson's Society of Alberta newsletter. In addition, at baseline there were age, sex, and education-matched control volunteers (27 men and 20 women). The controls ranged from 65 to 84 years (M = 71.4; SD = 4.9); the mean level of education was 14.9 years (SD = 3.6). The controls were recruited from the University of Alberta General Medicine Clinics and as acquaintances of other volunteers or respondents to local advertisements. Clinical depression was screened and participants were excluded from the study if their depression could not be managed by drug treatment. The study was approved by the University of Alberta Health Ethics review board and performed in accordance with the Helsinki declaration.
Longitudinal samples
We constructed two longitudinal data sets. First, after an 18-month interval, 75 participants returned and completed all relevant tasks at a second wave of testing. Returnees included 43 (of 47) controls and 32 (of 45) PD patients. One of the returning PD patients scored above .5 on the Clinical Dementia Rating scale (CDR) (Morris, Reference Morris1993) and was excluded from further analyses (leaving 31 dementia-free PD returnees). All PD patients were rated as between Hoehn and Yahr stages I–III (mild to severe). Since there were only three severe cases (Hoehn and Yahr all stage III) and no cases in stage IV, severity was not examined. Regarding attrition, there was no significant group (PD, controls) by longitudinal status (18-month continuers, drop outs) interaction on age or education levels. The second longitudinal data set was constructed as follows. At the subsequent (and final) 36-month follow-up, all 31 remaining PD patients returned and 10 were diagnosed with either dementia (n = 7) or cognitive impairment (n = 3). For our analyses of T1-to-T2 change, we labeled these 10 participants as Parkinson's disease with incipient dementia (PDID). Specifically, because they were diagnosed with significant cognitive impairment or dementia at T3 and were dementia-free at T2, they were incipient dementia PD participants at T2. They were examined in comparison to the remaining PD group and controls at T1 and T2. Although comparable data for controls at T3 are currently unavailable, the presence of potential pre-impairment participants at T2 would produce more conservative tests of our hypotheses.
Assessment
All participants were examined by a neurologist with expertise in aging and PD. The participant and an informant were independently interviewed at each time of measurement to determine if cognitive impairment was present. The assessment of cognitive impairment was based on consensus from a CDR interview by the physician (with the patient) and a trained research assistant (with an informant and reviewed by the physician) complemented by formal MMSE, Frontal Assessment Battery (FAB; Dubois, Slachevsky, Litvan, & Pillon, Reference Dubois, Slachevsky, Litvan and Pillon2000), and DRS assessment. Exclusion criteria at baseline included the presence of an unstable medical illness (e.g., active or recent cancer, symptomatic coronary artery disease, renal failure), an illness (other than PD) that could affect thinking or memory (e.g., symptomatic chronic pulmonary disease, epilepsy), or medications that could directly affect cognition (e.g., centrally acting anti-cholinergic medications). General health was graded using the Cumulative Illness Rating Scale (CIRS; Parmelee, Thuras, Katz, & Lawton, Reference Parmelee, Thuras, Katz and Lawton1995). The Hachinski Ischemic Score was used to rate risk factors for vascular dementia (Rosen, Terry, Fuld, Katzman, & Peck, Reference Rosen, Terry, Fuld, Katzman and Peck1980). Severity of cognitive impairment was graded using the CDR (Morris, Reference Morris1993). Motor function was assessed with the Unified Parkinson's Disease Rating Scale (UPDRS) (Gancher, Reference Gancher1997) and the Hoehn and Yahr staging (Hoehn & Yahr, Reference Hoehn and Yahr1967). Additional assessments included the MMSE (Folstein et al., Reference Folstein, Folstein and McHugh1975), the FAB, the DRS (Brown et al., Reference Brown, Rahill, Gorell, McDonald, Brown and Sillanpaa1999), the National Adult Reading Test-Revised (NART-R) (Blair & Spreen, Reference Blair and Spreen1989), and the Geriatric Depression Scale (GDS; 15-item screening version) (Yesavage, Reference Yesavage1988). All participants had blood tests to rule out reversible causes of cognitive impairment, including a complete blood count, glucose, urea nitrogen, creatinine, electrolytes, liver enzymes, thyroid stimulating hormone, vitamin B12, and folate levels at baseline. All participants with PD were examined in the on state of the dosing interval (i.e., when medication was effective, 1 to 3 hr after taking their medication). Because of age and practical concerns (e.g., frailty, ability to tolerate withdrawal) and the desire to examine best cognitive function, patients were not examined in the off state. Rather, patients retrospectively rated their on and off symptoms using the activities of daily living UPDRS-II scale (Goetz, LeWitt, & Weidenman, Reference Goetz, LeWitt and Weidenman2003).
Dementia, which was defined as cognitive impairment in two domains with functional impairment due to cognitive decline, was diagnosed based on both patient assessment and informant report, but did not necessitate memory impairment (i.e., modified from the DSM-IV, which was available at the time of study inception). In addition, dementia was diagnosed if patients declined cognitively across two waves as follows: (a) in two or more domains on the CDR, (b) 3 points on the modified MMSE, or 6 points on the DRS (version 2) (i.e., greater than 1 SD change). These values are beyond what would have been expected on the basis of the reliability of the instruments. In addition, the operationalization of PDID is supported by neuroimaging data (Camicioli et al., Reference Camicioli, Sabino, Gee, Bouchard, Fisher, Hanstock and Martin2011). That is, the group with PDID showed the greatest atrophy of gray matter relative to PD and controls, which supported the notion that PDID are undergoing brain degeneration. Participants were grouped together in the incipient dementia (PDID) group for T1 and T2 analyses if they had significant cognitive decline or dementia at T3.
Measures and Procedure
We used tasks, measures, and procedures adapted from previous research (Camicioli et al., Reference Camicioli, Weiler, de Frias and Martin2008; Dixon et al., Reference Dixon, Garrett, Lentz, MacDonald, Strauss and Hultsch2007; de Frias et al., Reference de Frias, Dixon, Fisher and Camicioli2007).
Reaction Time Tasks
Simple reaction time (SRT) and three two-choice reaction time (CRT-2, CRT-4, and CRT-8) tasks were administered. The instructions emphasized speed of performance. Participants responded to stimuli by pressing keys on a custom designed response console. Responses were recorded in milliseconds. In the SRT task, participants were presented with a warning stimulus (III) followed by a signal stimulus (+) in the middle of the screen. Participants were instructed to press a key with their preferred hand as quickly as possible when the signal stimulus appeared. A total of 50 test trials were administered with 10 randomly arranged trials presented at each of the five intervals separating the warning and signal stimuli (500, 625, 750, 875, and 1000 ms). The measures used were the latencies of the 50 test trials. For the three CRT tasks, a 3 × 3 grid matching the arrangement of keys on the response console was displayed on the screen. This array was used to instrument two-, four-, and eight-choice RT trials. The center square, corresponding to the center key in the response keypad, served as the home key for the participant's preferred forefinger. Each block of 10 trials required the participant to attend to two, four, or eight squares. A warning stimulus was presented, followed (after a delay of 1000 ms) by the appropriate two-, four-, or eight-square matrix. Twenty trials were administered at each level of choice. The measures used were the latencies of all trials averaged across conditions.
Data Preparation
Outliers were trimmed to missing as extremely slow or fast responses might reflect errors (e.g., accidental key press, task interruption). A lower bound for legitimate responses as based on prior research (de Frias et al., Reference de Frias, Dixon, Fisher and Camicioli2007) was set for each task at 150 ms. An upper bound was based on computing the mean and standard deviation separately for each of the groups and dropping any trials exceeding the mean by three or more standard deviations. The number of trials dropped across the entire Persons × Trials data matrix was small (approximately 2%). Missing values were imputed for the outlier trials using a regression procedure in which missing value estimates were based on the relationships among responses across trials. Missing values were imputed using data from all available individuals and trials. Dropping outliers and imputing missing data reduces variability thereby providing a conservative approach to examining this issue.
IIV was indexed by computing the intraindividual standard deviation (ISD), a method used in previous research (Dixon et al., Reference Dixon, Garrett, Lentz, MacDonald, Strauss and Hultsch2007; de Frias et al., Reference de Frias, Dixon, Fisher and Camicioli2007). The ISD was computed separately across each task to examine inconsistency. Group differences in average level of performance, and also systematic changes over time (trials) associated with practice represent potential confounds for the analysis of IIV. For example, greater IIV in PD patients than normal older adults as evidenced by an ISD computed on raw scores may reflect the fact that PD patients are on average slower than normal aging adults. To control for these potential confounds, we partialed out the effects associated with group and trials and their interaction from the data before computing ISDs. Specifically, we restructured raw latency trials for each speed measure into a person–trial data matrix and separately regressed on (patient-control status) group and trial, and their higher order interactions. This procedure produced residual scores that were independent of group differences in speed or accuracy of performance and systematic variation attributed to practice effects. These purified Z-scores were then converted to T-scores (with a score of 50 as the average for the sample), allowing for comparisons between the size of scores between groups. Raw mean RT and standard deviation values (in ms) for each group by wave of measurement is presented in Table 1. Alpha levels of p < .05 were specified as the threshold to indicate statistical significance.
Table 1 Raw mean RT and standard deviation values (in milliseconds) for each group by wave of measurement
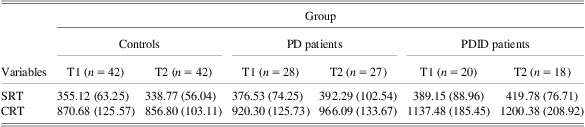
Note. Values in parentheses are standard deviations. RT = reaction time; SRT = simple reaction time (SRT); CRT = two-choice reaction time; PD = Parkinson's disease; PDID = Parkinson's disease with incipient dementia.
Results
Preliminary Analyses
Table 2 shows the cognitive impairment and health status of the PD patients and controls who participated over the 18-month period. There were no significant group differences over time on either status.
Table 2 Cognitive impairment and health status of the PD patients and controls who participated over the 18-month period
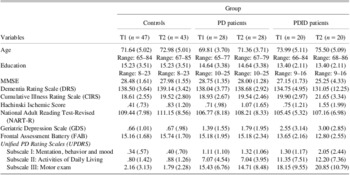
Notes. Values in parentheses are standard deviations. The group by time interactions were not statistically significant based on a MANOVA. T1 = Time 1; T2 = Time 2; PD = Parkinson's disease; PDID = Parkinson's disease with incipient dementia; MMSE = Mini-Mental State Examination.
A group (3: PDID, PD, controls) by task complexity (3: CRT2, CRT4, CRT8) by time (2: T1, T2) repeated measures ANOVA was conducted on the CRT average rate and IIV scores. There were no significant interactions with task therefore subsequent analyses for task were based on two complexity levels (simple, complex) by combining the CRT2, CRT4, and CRT8 scores for average rate and IIV, separately.
Issue 1: Longitudinal Change in Rate of Response Speed
A group (3: PDID, PD, controls) by task complexity (2: simple, complex) by time (2: T1, T2) repeated measures MANOVA was conducted on the mean latency scores. Only significant interactions are reported. The time by group interaction was significant, F(2,71) = 24.28, p < .001, η2 = .41. Based on the 95% confidence interval around the means, the controls became significantly faster over time overall (T1 M = 48.15; SE = .67; T2 M = 46.40; SE = .76), whereas the PD (T1 M = 47.45; SE = .92; T2 M = 51.08; SE = 1.04) and PDID (T1 M = 51.72; SE = 1.12; T2 M = 58.04; SE = 1.28) groups became slower over time overall (see Figure 1). The task by group interaction was significant, F(2,71) = 9.24, p < .001, η2 = .21. Based on the 95% confidence interval around the means, task complexity did not moderate performance for controls (simple M = 47.40; SE = .86; complex M = 47.15; SE = .64). However, the PD group (simple M = 50.67; SE = 1.18; complex M = 47.85; SE = .87) was less affected by task complexity (appearing more like controls) than the PDID group (simple M = 52.54; SE = 1.44; complex M = 57.22; SE = 1.06) (see Figure 2). The results were unchanged covarying for age and gender.
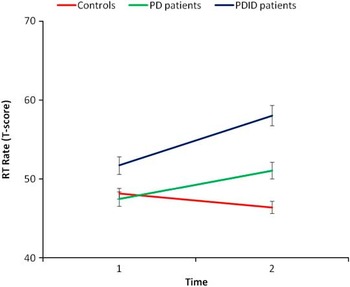
Fig. 1 Over the 18-month interval, the Parkinson's disease (PD) and PD with incipient dementia (PDID) groups performed slower on average on reaction time (RT), whereas healthy older adults improved.
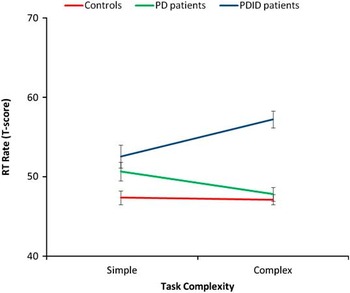
Fig. 2 The Parkinson's disease (PD) group performed faster on average with increasing task complexity whereas the PD with incipient dementia (PDID) group performed slower.
To check the potential effect of overall motor impairment (UPDRS-III) as measured at baseline in accounting for the two significant interactions, we computed a repeated measures multivariate analysis of covariance (MANCOVA) for the PD and PDID groups only. The results indicated that after controlling for UPDRS-III at baseline, the group by task interaction remained significant (p < .01) but the time interaction did not (p = .28).
A logistic regression model was conducted with the two 18-month change (using difference scores) in mean rate scores in the same model. All three combinations of group membership were then compared (i.e., controls vs. PD patients, controls vs. PDID patients, and PD vs. PDID patients) in three separate models (see Table 3). Change in mean rate in CRT was a significant predictor of group membership between PDID vs. controls: (Exp β) = 2.32; 95% CI = 1.23–4.35 (sensitivity = 71%; specificity = 97%). The positive predictive value (PPV) was 91% and the negative predictive value (NPV) was 90%. Change in mean rate in CRT was also a significant predictor of group membership between PD vs. controls: (Exp β) = 4.01; 95% CI = 1.82–8.8 (sensitivity = 95%; specificity = 97%). The PPV is 95% and the NPV is 97% (see Table 3). No other effects were significant.
Table 3 Sensitivity and specificity rates of logistic regression models for predicted probability values of .50 for each group pairing
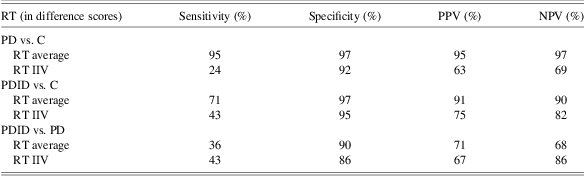
Note. C = controls; PPV = positive predictive value; NPV = negative predictive value; RT = reaction time; PD = Parkinson's disease; PDID = Parkinson's disease with incipient dementia.
ROC analyses were conducted by generating the predicted probabilities associated with each group comparison from the logistic regression model. The area under the ROC curve (AUC) comparing PD and controls was .71 (p < .01; 95% CI = .55, .86) for SRT, and .98 (p < .001; 95% CI = .95, 1.00) for CRT. The AUC comparing PDID and controls was .76 (p < .01; 95% CI = .59, .92) for SRT, and .96 (p < .001; 95% CI = .91, 1.00) for CRT. The AUC comparing PDID and PD groups was .59 (p > .05; 95% CI = .39, .79) for SRT, and .63 p > .05; 95% CI = .40, .85) for CRT.
Issue 2: Longitudinal Change in Inconsistency
A group (3) by task complexity (2) by time (2) repeated measures MANOVA was conducted on the mean ISD scores. Similar to Issue 1 above, only significant interactions will be presented. The time by group interaction was significant, F(2,71) = 6.03, p < .01, η2 = .15. Based on the 95% confidence interval around the means, the PDID patients (T1 M = 7.58; SE = .37; T2 M = 9.22; SE = .45) were more inconsistent over time, whereas the controls (T1 M = 6.12; SE = .22; T2 M = 6.09; SE = .27) and PD patients (T1 M = 6.55; SE = .30; T2 M = 7.14; SE = .37) did not change in inconsistency over time (see Figure 3). The task by group interaction was significant, F(2,71) = 3.79, p < .05, η2 = .10. Based on the 95% confidence interval around the means, the PD patients’ level of inconsistency (simple M = 6.77, SE = .32; complex M = 6.92, SE = .38) was unaffected by task complexity. However, the controls’ (simple M = 6.42; SE = .24; complex M = 5.79; SE = .28) and the PDID patients’ (simple M = 7.98; SE = .40; complex M = 8.82; SE = .46) level of inconsistency were more affected by task complexity (see Figure 4).
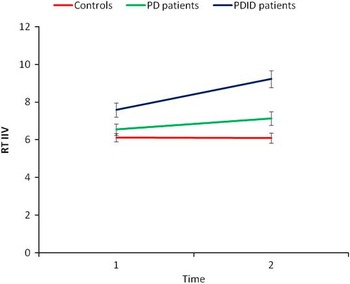
Fig. 3 Parkinson's disease with incipient dementia (PDID) patients became more inconsistent over time whereas the PD patients and healthy older adults remained at baseline levels of inconsistency. RT IIV = reaction time intraindividual variability, or individual standard deviation.
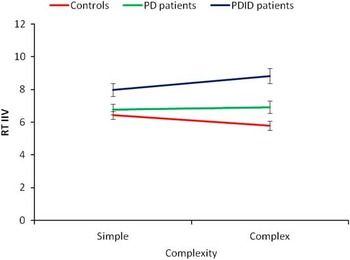
Fig. 4 PDID patients’ and healthy older adults’ level of inconsistency averaged over time was moderated by task complexity. RT IIV = reaction time intraindividual variability, or individual standard deviation.
A repeated measures MANCOVA was conducted for the PD and PDID patients only, to examine the potential effect of overall motor impairment (UPDRS-III) at baseline in accounting for the two significant interaction effects. Neither interaction was significant after controlling for overall motor impairment, indicating that task performance is related to overall progression of PD.
A logistic regression model was conducted with the two 18-month change (using difference scores) in IIV scores in the same model. As before, all three combinations of group membership were then compared in three separate models. Change in IIV in SRT ((Exp β) = 1.38; 95% CI = 1.05–1.83) was a significant predictor of group membership between PDID and controls. The sensitivity was 24% and the specificity was 92%. The PPV was 63% and the NPV was 73% (see Table 3). No other effects were significant.
ROC analyses were conducted by generating the predicted probabilities associated with each group comparison from the logistic regression model. The area under the ROC curve (AUC) comparing PD and controls was .65 (p > .05; 95% CI = .50, .79) for SRT, and .52 (p > .05; 95% CI = .35, .68) for CRT. The AUC comparing PDID and controls was .69 (p < .05; 95% CI = .53, .85) for SRT, and .66 (p > .05; 95% CI = .47, .85) for CRT. The AUC comparing PDID and PD groups was .57 (p > .05; 95% CI = .36, .77) for SRT, and .62 p > .05; 95% CI = .42, .81) for CRT.
Discussion
We examined longitudinal (2-wave, 18-month) changes in two indicators of neurocognitive speed performance (average rate and IIV) in non-demented PD patients, controls, and PD patients with incipient dementia (PDID). As supported by (a) our previous inconsistency results with PD patients (de Frias et al., Reference de Frias, Dixon, Fisher and Camicioli2007), (b) theoretical models (Li & Lindenberger, Reference Li and Lindenberger1999) applied to PD, and (c) previous studies IIV in normal aging and cognitive impairment, we predicted both relatively greater slowing and inconsistency over time for the PDID group, followed by the PD and control groups. We addressed two main research issues. First, we examined whether the three groups had similar two-wave trajectories of mean rate (speed) performance. We found that the controls became faster over time, perhaps due to practice effects, whereas both the PD and PDID groups became slower. Of interest, recent research shows that practice effects may be an early marker of incipient cognitive decline (Cooper, Lacritz, Weiner, Rosenberg, & Cullum, Reference Cooper, Lacritz, Weiner, Rosenberg and Cullum2004; Duff et al., Reference Duff, Beglinger, Schultz, Moser, McCaffrey, Haase and Paulsen2007; Suchy, Kraybill, & Franchow, Reference Suchy, Kraybill and Franchow2011). The apparent reduced or lack of practice effects in our study for PD or PDID patients may be attributed to a compromised ability to benefit from practice, at least at 18-month intervals when motor declines may cancel potential practice-related improvements. The lack of learning effect could reflect dopaminergic loss as seen in all PD patients. In addition, greater novelty effects on a motor task was found among older adult cognitive decliners relative to nondecliners (Suchy et al., Reference Suchy, Kraybill and Franchow2011), likely due to vulnerable cognitive reserves. We also found that the groups differed in how task complexity affected their performance. Task complexity did not moderate the average rate of controls, but did affect the performance of the PD and PDID groups. To address the second main issue, we examined the three groups on inconsistency. Our 18-month longitudinal data showed that the PDID patients became more inconsistent over time, whereas the stable PD patients and controls remained at baseline levels of inconsistency. Greater task complexity moderated inconsistency, such that the groups become more different from each other with task complexity. Within groups, the controls were less inconsistent, the PD remained unaffected, and the PDID group became more inconsistent with advancing complexity.
To explore these results further, we conducted several follow-up analyses. First, we examined the potential effect of overall motor impairment (UPDRS-III) at baseline in accounting for the significant group by time interaction effects. The group by time interaction for average rate was not significant, along with the interactions for inconsistency after covarying for UPDRS-III at baseline and these findings were also found using UPDRS-III at T3. Change in average rate and inconsistency may have been partly accounted for by dopaminergic deficits in motor pathways and disease progression. Second, we compared the relative effectiveness of IIV and mean rate in distinguishing change between PD, PDID, and controls. Examining ROC models, we found that the predictive validity of change in mean rate or IIV on membership into PD vs. PDID groups was not supported by the AUC. However, the logistic regression analyses showed that the PDID and PD groups were more likely to show slower mean rate of performance (in CRT) over time than the controls. The ability to differentiate the controls from PD and PDID groups was further supported by the ROC model. The logistic regression analyses showed that the PDID group was more likely to show greater inconsistency (in SRT) over time than the controls, and the ability to differentiate these two groups was further supported by the AUC.
Overall, these results extend related earlier work. For example, we found that PD patients (both treated and untreated groups) were slower and more inconsistent than controls, and that PD disease severity was a moderator of IIV (Camicioli et al., Reference Camicioli, Weiler, de Frias and Martin2008; de Frias et al., Reference de Frias, Dixon, Fisher and Camicioli2007). The present study extends these findings by showing that changes in mean rate and inconsistency are greatest in PD patients with incipient dementia. Clinically, this suggests that neurocognitive speed deficits may be early markers of PDID, perhaps distinguishing those with preclinical dementia from those who remain stable in non-demented status. Theoretically, these results call attention to the well-known fact that PD patients may differentially develop early signs of dementia, and even common concurrent assessment techniques may not be sensitive to preclinical changes. Accordingly, in single-occasion studies, some dementia-free PD patients may in fact be in the very early—and difficult to detect—preclinical phases, thus affecting the overall group average performance on other assessment tests. For both clinical and research reasons, it is important to identify those PD patients with incipient dementia: Systematic assessment of neurocognitive speed may provide important markers. Is there a difference in effectiveness between the two markers we tested? Our study shows that change in IIV is a more sensitive marker compared to change in mean rate in differentiating the longitudinal profiles of PDID from PD in the preclinical phase. One clinical implication of these results is that individuals with PDID who show greater inconsistency over time may be especially targeted for selection into cognitive-enhancing therapies.
Our findings also extend those of cross-sectional studies showing slower speed and greater inconsistency in aging groups with increased neuropathology, but not for groups with somatic or peripheral disease (Hultsch et al., Reference Hultsch, MacDonald, Hunter, Levy-Bencheton and Strauss2000; Whitehead, Dixon, Hultsch, & MacDonald, Reference Whitehead, Dixon, Hultsch and MacDonald2011). For example, recent studies have reported exaggerated inconsistency in neurocognitive and attention performance in older individuals with mild cognitive impairment (Dixon et al., Reference Dixon, Garrett, Lentz, MacDonald, Strauss and Hultsch2007) and mild dementia (Duchek et al., Reference Duchek, Balota, Tse, Holtzman, Fagan and Goate2009; Gorus et al., Reference Gorus, De Raedt, Lambert, Lemper and Mets2008). A study by Burton et al. (Reference Burton, Strauss, Hultsch, Moll and Hunter2006) reported greater slowness and IIV for a PD group than controls, but the AD group performed worse in both aspects of neurocognitive speed. Notably, all these studies show that IIV discriminates between normal aging and early-to-severe cognitive impairment. The present results supplement this perspective by showing that changes in inconsistency and general slowness are especially marked in PD patients with incipient dementia, as compared even to parallel PD patients with no incipient dementia. The pathology of advanced PD is marked by symptoms that are not responsive to dopaminergic therapy, such as dementia. The mechanisms underlying greater IIV in PDID may be due to a decrease in catecholamines (Li & Lindenberger, Reference Li and Lindenberger1999), cholinergic deficits (Klein et al., Reference Klein, Eggers, Kalbe, Weisenbach, Hohmann, Vollmar and Hilker2010), or cortical pathology (Camicioli et al., Reference Camicioli, Sabino, Gee, Bouchard, Fisher, Hanstock and Martin2011).
Several limitations of the present study can be noted. First, most of our PD patients were using dopaminergic medications for treatment of symptoms, but our previous research (Camicioli et al., Reference Camicioli, Weiler, de Frias and Martin2008) shows that untreated PD patients were also more inconsistent in neurocognitive speed than controls. All participants with PD were tested in the on state of the dosing interval, a period noted for improved motor functioning. Both medication use and time of testing may serve to minimize potential differences between patients and controls. Second, our PD group size became smaller over time; however, reliable group differences and changes were detected. Third, a younger PD group was not included in the study design. Such a comparison group would be useful to examine whether greater IIV is specific to aging and PD or a function of disease pathology. Fourth, errors were not collected to examine error rates across groups, leaving the possibility that some participant groups may have expressed speed/accuracy tradeoffs, making them appear faster. Fifth, our measures included visual reaction time tasks and therefore our results may not generalize to other modalities (e.g., auditory reaction time tasks). Sixth, as T3 data for controls is not currently available, 36-month trajectories cannot be examined. Conceivably, some controls may have declined by T3, but any pre-impairment condition would provide for a more conservative test of the hypotheses of group differences.
Several strengths of the study can also be noted. First, the three-wave longitudinal design permitted a unique test of non-demented PD patients compared to PDID patients. Such a preclinical group is an important addition to the literature. Second, our study demonstrates the clinical utility of neurocognitive inconsistency as a potential clinical symptom for the early identification of incipient dementia in PD. Third, all participants were well characterized and strict exclusionary criteria were applied. Although some studies have begun to examine longitudinal changes in neurocognitive performance in healthy control samples (e.g., Bielak et al., Reference Bielak, Hultsch, Strauss, MacDonald and Hunter2010; Lövdén et al., Reference Lövdén, Li, Shing and Lindenberger2007; MacDonald et al., Reference MacDonald, Hultsch and Dixon2003), ours is the first to date to focus on longitudinal changes in neurocognitive speed and inconsistency in PD and PDID groups.
In conclusion, our study shows that longitudinal profiles of both mean rate of neurocognitive speed and IIV distinguish between PDID and healthy aging, and IIV distinguishes PD and PDID (incipient dementia). Future research may examine the neural correlates of changes in mean rate and IIV to illuminate the mechanisms underlying the bifurcation between those PD patients who become demented from those who remain dementia-free.
Acknowledgments
Richard Camicioli was supported by an operating grant from the Canadian Institutes of Health Research (CIHR) (MOP-67132). Roger Dixon was supported by grants from the U.S. National Institute on Aging (R37 AG008235) and the Canada Research Chairs program. We thank Nancy Fisher for data collection of the RT tasks, which were adopted from the Victoria Longitudinal Study. The information in this manuscript and the manuscript itself has never been published either electronically or in print. No conflicts of interest exist.